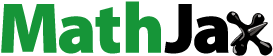
Abstract
Increasing temperature and frequency of drought associated with climate variability and change and low soil fertility are factors that handicap sustained rice (Oryza sativa L) production in the tropics. In this study, the growth and yield of rice (Legon 1 rice variety) were investigated under three temperature regimes T1 = 33 °C, T2 = 34 °C, and T3 = 36 °C, two water regimes W1 = continuous flooding and W2 = intermittent irrigation, and two nitrogen application rates: (N1 = 0 kg N ha−1 and N2 = 90 kg N ha−1) at the Soil and Irrigation Research Centre, of the University of Ghana, Ghana. The experimental design was split-plot with temperature treatments as main plots and water and nitrogen applications as subplots with 4 replicates. The rice plants under high temperatures (T3 & T2) produced significantly lower biomass, nutrient uptake, and grain yield. Furthermore, the intermittent irrigation (W2) and nitrogen application (N2) significantly increased the percentage of yield components, grain yield, and nutrient uptake, The intermittent irrigation saved between 20 and 33% of irrigation water. The grain yield under the intermittent irrigation was 36, 24, and 15 g pot−1 for T1, T2, and T3, respectively, compared with 22, 14, and 8 g pot−1 for T1, T2, and T3 under the full continuous irrigation conditions. The uptake of nutrients was lower under the increased temperature with the rate of decline higher under nitrogen fertilization. The potential decline in grain quality due to reduced nutrient uptake poses a challenge for human nutrition under warmer climates.
1. Introduction
Rice (Oryza sativa), is a major staple in many tropical countries. Despite several policy interventions, self-sufficiency in rice production continues to be a challenge in these countries. The percent import of rice in Ghana was 50% in 2019 (MoFA-IFPRI, Citation2020), while that of Rwanda is somewhat less, i.e. 40% in 2018 (Wigboldus et al., Citation2021). The success of achieving higher local production of rice rests on several factors, among which climate has become a major handicap. Climate change, which manifests as increasing temperature and frequency of extreme rainfall events such as drought and floods (Furtak & Wolińska, Citation2023; MacCarthy et al., Citation2023) is a major threat to crop production, especially in tropical rainfed agricultural systems. Data from Ghana shows that over the past 40 years, the temperature rose by 1 °C (Mawunya & Adiku, Citation2013). Increasing temperatures may adversely impact plant phenology, quality, and quantity of production and as well yields (MacCarthy et al., Citation2021). Models have demonstrated the negative effects of climate change on the yields of major crops in tropical regions (Rezaei et al., Citation2023). According to Parkes et al. (Citation2022) ambient temperatures beyond the optimum often lead to heat stress, which would modify the crop’s lifecycle and negatively affect production (Dubey et al., Citation2018). The decrease in crop yield would depend on the intensity and duration of the stress (Nadeem et al., Citation2019).
Apart from temperature effects, water and nutrient availability are also major determinants of crop growth and yield (Fahad et al., Citation2017). With regard to the tropics, rainfall at several locations in Ghana have shown decreasing trends, with increasing frequency of dry spells (Adiku, Citation2019; Gbangou et al., Citation2020). Even in those locations showing no significant reduction in seasonal rainfall, there is evidence of shifts in the within-season distribution. Early season rains have declined in favour of late season rainfall that has significantly increased (Adiku, Citation2019). A reduction in rainfall under climate change would influence soil moisture storage and availability, leading to water stress (Serdeczny et al., Citation2017). Water stress is the most dominant factor causing the yield reduction in rice plants (Venkatesan et al., Citation2005) because rice naturally has high water demand. Water stress is particularly damaging to morphological and physiological development, reducing rice yields due to a decrease in the number of tillers, panicles, grain, and seed weight (Dabi & Khanna, Citation2018), which is exacerbated by concurrent temperature stress (Wiebbecke et al., Citation2012). Under the condition of water stress, roots are incapable of taking up many nutrients from the soil (da Silva et al., Citation2011).
Furthermore, the low inherent fertility of tropical soils coupled with the low fertilizer input are major causes of poor crop yields in the tropics (MacCarthy et al., Citation2018). A typical crop response to nutrient deficiency stress is a reduction in growth and rate of development (Sanderson et al., Citation1997). Nitrogen (N) is one of the most significant biological components for plants since it is part of amino acids, proteins, genetic materials, pigments, and other key organic molecules (Tang et al., Citation2019). Nitrogen deficiency also impacts a host of physiological processes such as photosynthesis (Ghannoum et al., Citation2005; Makino et al., Citation2003), enzyme activity (Xu et al., Citation2015), the ratio of enzyme activity to electron transport activity under N deficiency (Mu & Chen, Citation2021), among others. The combination of increased soil and environmental temperature, drought and nutrient deficiency may have a greater interactive impact on the phenological, morphological, and other growth traits on rice plants (Dubey et al., Citation2018) than the sole factors. Indeed, it is conceivable that increasing temperature would increase evaporation rates and soil desiccation, reducing the uptake of nutrients such as N, that are taken up in the transpiration stream.
In the face of the challenges, the development of appropriate water and nutrient management practices become important in ensuring sustained growth and yields of rice. The water deficit problem can be remedied by irrigation, but this has not been achieved in many resource poor developing countries. The total arable land under irrigation in Ghana is less than 2% (Gross & Pennink, Citation2018; Mendes et al., Citation2014). Yet, water saving technologies such as “intermittent” instead of full-scale irrigation, that have been proposed in the literature have not been adequately assessed in Ghana, especially under climate change conditions. According to Pascual and Wang (Citation2016), intermittent irrigation, instead of full-scale irrigation led to water savings between 55% and 74% than continuous flooding. Singh et al. (Citation2005) reported that irrigation could be withheld for about 7–15 days after rice sprouting, depending on soil texture. Deep rooting enabled by delayed irrigation, reduces leaching of nitrogen and makes seedlings resistant to drought. Hence, water and nutrient management may constitute two key controls that could offset climate change impact on rice growth. Yet, studies in most of the Sub-Saharan African countries on the effects of climate change on rice yield and the types of management required to minimize the impact, are still minimal. Thus, whereas the literature shows the results on rice response to sole factors such as temperature, water stress, and nutrient deficiency (Anim, Citation2017; Chaturvedi, Citation2005; Tuong et al., Citation2005; Xiong et al., Citation2018; Yang et al., Citation2017), the combined effect of these abiotic factors on rice growth and development has not received sufficient attention.
This study evaluates the growth, yield and uptake of N, P and K of rice (Legon 1 rice) under varied temperature, watering regimes and N application managements conditions.
2. Material and method
2.1. Description of the experimental area
The experiment was conducted under 3 different temperature chambers created at the Soil and Irrigation Research Centre (SIREC) - Kpong, University of Ghana, which lies within a major rice-growing zone of Ghana. The centre is situated in the lower Volta basin in the Coastal Savannah Agro-ecological zone at a latitude of 6° 09′ N and longitude 0° 04′ E with an altitude of 22 m above sea level. The soil used was derived from alluvial material weathered from hornblende gneiss (Brammer, Citation1955), and classified as Typic Calciustert (Amatekpor et al., Citation1993). It is locally known as the Amo series, which are normally deep black soils. The temperature varies between 22.1 and 33 °C with an average air temperature of 27.2 °C. The relative humidity ranges between 70% and 100% at night and 20% to 65% during the day.
2.2. Construction of temperature chambers and measured weather variables
Three temperature chambers were constructed to house the experiments of this study. The chambers comprised of wooden frames of dimension 4.78 m (length) 1.24 m (width) and 1.13 m (height) covered by transparent polythene sheets ().
The temperatures in the chambers were not controlled but varied from one another according to the number of windows created in the side of the polythene sheets to allow wind inflow and outflow. Weather parameters (temperature, humidity) were monitored and recorded by Bio-Temp In and Out Thermo-Hygrometer, (Model: LIEGE6753, Luxemburg) five times each day (6 am, 9 am, 12 noon, 3 pm and 6 pm) and averaged to estimate minimum, maximum and mean temperatures. The temperature measurements showed that one chamber had an average of 33 °C (T1), another had 34 °C (T2), and the last one had 36 °C (T3), though the actual diurnal temperatures varied largely. The dynamics in the average temperature over the growth period is illustrated in .
The vapour pressure deficit (VPD) within the chambers were determined as (Berry et al., Citation1945):
(1)
(1)
With SVP defined as (Monteith & Unsworth, Citation2013):
(2)
(2)
Where SVP is Saturation Vapour Pressure, T is temperature.
2.3. Chamber experiments
The soils used for the experiment were sampled at a depth of 0–20 cm and air-dried and sieved through a 5 mm mesh and potted into small plastic buckets of 22 cm diameter and 18 cm height, to a bulk density of 1.3 g cm−3. The lower ends of the buckets were not perforated so that drainage was prevented. After potting, each pot held 6 kg of sieved soil, which were pre-saturated with 2.5 L of water prior to seeding with rice. The rice variety was Legon 1 rice obtained from SIREC. This variety is largely cultivated in lowland zones in Ghana, having a medium duration of around 125 days, and a potential yield of about 6.00 tons per ha. Twenty-one-days old seedlings were transplanted with three seedlings per pot. For the first 12 days following transplanting, all the pots received watering at 500 mL and kept in a large screenhouse before transferring to the temperature chambers. At the transfer date, the seedlings were thinned to one plant per pot and applied with a basal phosphorus fertilizer at the equivalent of 60 kg P2O5 ha−1 and K fertilizer at 60 kg K2O ha−1 from KCl.
Superimposed on the three temperature environments were two water treatments: (i) continuous flooding, W1, and (ii) intermittent irrigation, W2. The water application to the intermittent irrigation treatments were determined from a drop in the water level in piezometers that were installed in each pot. The piezometers were constructed from PVC pipes with holes drilled on the sides. For the continuous flooding, about 2 cm water level above the soil was maintained. Water treatments commenced three (3) days after the pots were transferred to the chambers. Two nitrogen application rates, zero kg N ha−1 and 90 kg N ha−1 were imposed on the temperature and water treatments, giving two water and two nitrogen and three temperature chambers. There were ten replicates of each treatment, giving forty experimental units in each chamber, or 120 units for all three chambers. The experiment was laid out as a split-plot design.
2.4. Data collection
The quantity of water applied to each treatment unit was recorded on a daily basis. As indicated, watering was done to restore the water to predetermined levels in the pots with the aid of the piezometer. The plant phenology was determined as the number of days to 50% of the plants reached a particular development stage. Observations were made on days to (i) 50% flowering, and (ii) 50% maturity. Data were also collected on the plant height and number of tillers. Two destructive samplings were carried out; first was at flowering with 3 pots and the second harvest was at maturity with 4 pots per treatment. Root and shoot dry matter were determined at maturity after oven-drying for three days at 70 °C. Data on yield parameters such as the number and weight of panicles, grain number and weight, and the percentage of grain-filling were also collected. The Water Use Efficiency (WUE) for each treatment as defined in Nyasapoh et al. (Citation2022) was calculated as:
(3)
(3)
2.5. Plant analysis and nutrient uptake
Tissue N, P and K concentrations in the rice straw and grain were determined. For this, a sample of finely milled plant samples were digested in H2SO4. and subsequently steam-distilled into 2% boric acid to determine N concentration according to the Kjeldahl Digestion method. A mixed indicator containing methyl red and methylene blue were used for colour development in the distillate and then titrated against 0.01 M HCL solution (Bremner, Citation1965). Total P was determined by using double acid wet digestion method with a 2 : 1 (v/v) nitric : perchloric acids ratio (AOAC, Citation1990) and analysed colorimetrically on a Cole Parmer 83057-01 (USA) spectrophotometer using ascorbic acid for colour development. Total K was determined by the double acid wet digestion method with 2 : 1 (v/v) nitric : perchloric acids ratio and analysed on a Perkin Elmer Analyst 800 (USA) atomic absorption spectrometer. The nutrient uptake was then calculated by multiplying the nutrient concentration with dry matter weight (MacCarthy et al., Citation2020) and expressed as mg of nutrient per pot.
2.6. Statistical analysis
Quantitative data were subjected to Analysis of Variance (ANOVA) using GenStat statistical software (12th edition, 2009), and mean differences were compared using the Duncan Multiple Range Test at a 5% significance level. To determine the effect of temperature on the uptake of the nutrients (N, P and K) in straw and grain, Analysis of Covariates was used. Temperature was the quantitative covariate, while water and nitrogen were categorical variables. Microsoft Excel (Office 2013) was used for data entry, and the graphical representation of data was with GraphPad Prism (version 5.00.288) and Sigma Plot (version 10.0).
3. Results
3.1. Chamber weather conditions during the experiment
shows the average daily temperature and humidity patterns during the growth period of the plants (i.e., December to February 2020) for the different temperature chambers. The maximum temperatures for T1 and T2, reached 34 °C and 36 °C, respectively, whereas that of T3 reached 39 °C. However, the mid-day temperature in T3 could reach values as high as 49.5 °C. Throughout the experiment, the highest temperatures coincided with the reproductive (flowering and maturity) stages of the plants.
Figure 3. Average daily (a) temperature (°C) and (b) humidity in the growth chambers throughout the experiment.
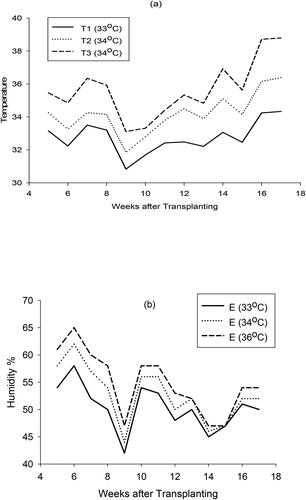
The Relative Humidity (RH) also varied throughout the experiment for the three different chambers (), with the RH decreasing from the beginning of the experiment to the end of the study. As the chambers were enclosed with polythene sheets, the free-flow of wind that would transport vapour away was prevented. Therefore, the T1 chamber with the lowest temperature had a relatively higher RH than the T2 and T3. However, the combination of temperature and RH, as expressed by the VPD, showed that in general T1 had an average VPD of about 2.5 kPa while T2 and T3 had 3.0 and 3.4 kPa, respectively, especially towards the end of the growing period. Higher VPD would imply a drier atmosphere and will exact more transpiration from the plants.
3.2. Soil water and evapotranspiration
All the full-flooding water treatments (W1) received a total of 37.0 L per pot (equivalent of 1000 mm) of water applied under the high N (N2) application, irrespective of chamber temperature (). Under the low N (N1) application (), the total water applied under T1 was 28.9 L per pot (744 mm). In the case of the intermittent irrigation W2, water application varied with the temperature and humidity conditions of the chambers. Irrespective of N application rate, a total of 30.3, 28.3 and 25.6 L of water were applied to pots under T3, T2 and T1, respectively (). These correspond to 800, 744 and 674 mm, respectively. Thus, the higher VPD environment (T3 and T2) received more water application than the lower VPD chamber (T1). In all cases, the intermittent irrigation showed a water saving of between 20% (T3) to 33% (T1) over the full-scale irrigation.
3.3. Effects of the different treatments on dry matter yield
The growth environment significantly influenced straw yield with T1 (lowest temperature) recording the highest straw yield. Straw yield increased in all chambers over time, with the highest values recorded at the maturity stage (). In general, straw yield was highest for plants under T1 with high N and intermittent water application (W2). The effect of nitrogen application was significant in influencing straw yield in 12 WAT (weeks after transplanting). The effect of water management also significantly influenced straw yield across the 3 development periods. Shifting from continuous flooding, (W1), to intermittent flooding, (W2), resulted in a 20% straw yield increased at 14 WAT. Thus, though less water was applied, shoot growth was not adversely impacted.
Figure 5. Dry yield of shoot and roots of rice at different growth stages grown under different temperature, water and nitrogen fertilizer management. T1, T2 and T3 are growth chamber temperatures at 33, 34 and 36 °C, respectively. W1, W2, are intermittent watering and continous flooding, respectively, while N1 and N2 are control and 90 kg N ha−1, respectively.
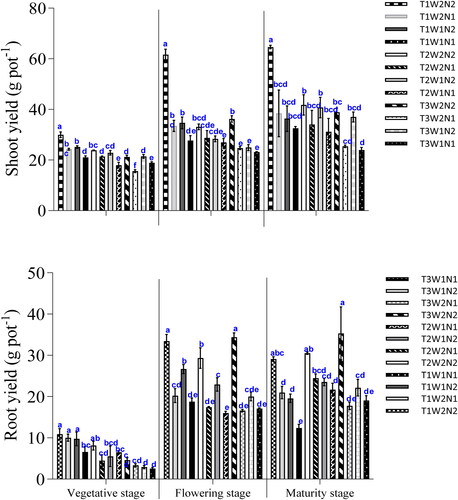
Root growth was enhanced for plants under the low temperature chamber T1 (). Increasing temperature to 34°C (T2) and 36°C (T3) resulted in a reduction in root dry weight per pot by 18% in T2 and 23% in T3 at 14 WAT. The interaction between water and nitrogen also influenced dry root yield significantly (p < .05) at 14 WAT.
3.4. Treatment effects on tiller production and yield components
The number of tillers were not significantly influenced by temperature in the growth chambers (). The highest tiller numbers were recorded for T1W2N2 treatment, which corresponded to the low temperature, intermittent water and high N application. The application of nitrogen resulted in an increased number of tillers (p < .05). Plants that received intermittent watering performed better than those under continuous flooding, resulting in an increased number of tillers by 15%, 26%, and 28% at the 8th, 12th, and 14th WAT, respectively ().
Table 1. Mean tiller numbers at 14 WAT under varying temperature, water, and nitrogen treatments. W1, W2, are intermittent watering and continous flooding, respectively, while N1 and N2 are control and 90 kg N ha−1, respectively.
In the case of panicle numbers, the temperature environments did not significantly influence the number of panicles produced per pot (). Water management significantly influenced the number of panicles produced per pot. A shift from continuous flooding to intermittent irrigation resulted in a 13% increase in panicle numbers. The application of nitrogen also significantly influenced the number of panicles per pot with an increase in the number of panicles by 111% relative to the control. The interaction between water and nitrogen also significantly influenced the number of panicles per pot. The growth temperature significantly (p < .05) influenced panicle yield with the highest recorded for T1 (33 °C). In the other two (2) chambers, T2 and T3, panicle yields were 25% and 28% respectively lower than those in T1. Intermittent watering (W2) increased panicle yield by 28% over the continous flooding treatment (W1). As with the other parameters, nitrogen also influenced panicle yield significantly (p < .05) leading to a 77% increase in panicle yield over the treatment with no N application. The interactions between water and nitrogen as well as the growth environment also influenced panicle yield.
Figure 6. Panicle number and yield of irrigated rice under the different temperature, nitrogen and water treatments. T1, T2, T3, are temperatures at 33, 34 and 36°, respectively. W1, W2, are intermittent watering and continous flooding respectively, while N1 and N2 are control and 90 kg N ha−1.
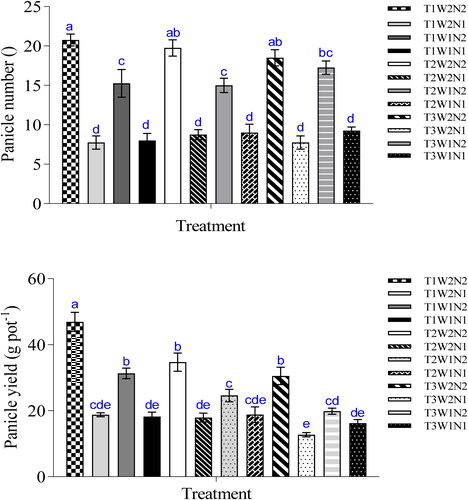
Grain yield was significantly (p < .05) influenced by chamber temperature with T1 recording the highest grain yield (). The other two chambers T2 and T3, recorded grain yields that were lower than those in T1 by 27 and 56% respectively. Water management also significantly influenced grain yield. A shift from continuous flooding to intermittent irrigation resulted in a 45% increase in grain yield. The application of nitrogen fertilizer also significantly increased grain yield by 138%. The effect of chamber environments significantly (p < .05) influenced the proportion of filled-grains. The highest proportion of filled grains was recorded in T1. This reduced by 12 and 32% with increasing temperature in T2 and T3, respectively, relative to T1. Also, the application of fertilizer significantly affected the proportion of filled-grains by 25%, compared to the control. The interactions between the growth environment and nitrogen fertilizer, as well as that between water and nitrogen fertilizer significantly (p < .05), influenced the proportion of filled-grains.
Figure 7. Grain-filled number, grain yield and water use efficiency of irrigated rice under the different temperature, nitrogen and water treatments. T1, T2, T3, are temperatures at 33, 34 and 36°, respectively. W1, W2, are intermittent watering and continous flooding respectively, while N1 and N2 are control and 90 kg N ha−1.
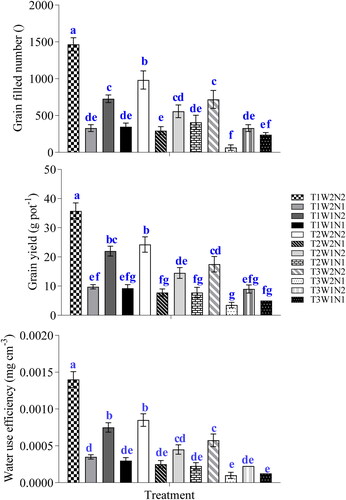
3.5. Effects of water and nitrogen management on water use efficiency (WUE)
There was a significant (p < .05) difference in the interaction among temperature, water, and nitrogen treatments. The combination of temperature and water treatments significantly (p < .05) influenced WUE. The highest WUE was recorded under W2 (intermittent irrigation) and the least value recorded under W1 (). Both temperature and watering regime significantly (p < .001) impacted WUE. Intermittent irrigation produced a higher WUE than full irrigation. The interaction between temperature and nitrogen was also significant (p < .05), however, nitrogen application resulted in better water use efficiency.
3.6. Effects of water and nitrogen management on nutrient uptake
summarizes the effects of temperature, water, and nitrogen management on the uptake of N, P, and K in both grains and straw. The grain N uptake was highest for T1 and decreased by 40% in T2 and 70% in T3 relative to that obtained in T1. Also, N uptake in straw was highest in T1, and declined under increasing temperatures by 33% in T2 and 50% in T3. The effect of water management also influenced N uptake in the grain significantly (p < .05). Intermittent irrigation resulted in an increase in N uptake by 129%. The N uptake in straw under intermittent irrigation was 44% higher than those under continuous flooding. The application of nitrogen fertilizer also influenced N uptake significantly (p < .05). Nitrogen uptake increased by 553% over the control across the three temperature environments. Interactions among temperature environment, water, and fertilizer also influenced N uptake significantly (p < .05).
Table 2. Mean Grain and Straw N, P and K uptake under different temperature, water and nitrogen treatments.
With regard to P uptake, environments T2 and T3 recorded about 37% and 46% less P uptake compared with T1. Water management also had a significant influence on P uptake with intermittent irrigation recording 247% increased P uptake relative to continuous irrigation. The uptake of P in straw was not influenced by water management. However, the application of nitrogen increased P uptake in the grain by 78% relative to the control. Significantly (p < .05), N also influenced the uptake of P in straw with an increase of 38% relative to the control. The interaction effects of temperature × water, temperature × nitrogen, water × nitrogen all influenced the uptake of P.
The uptake of K in grain was influenced (p < .05) by the combination of temperature and water treatments. The highest K uptake was recorded under T1W2 and the least uptake value recorded under the T3W2. Temperature treatments significantly (p < .05) affected the uptake of K in the grain with mean values of 170.5, 102.8, and 47.9 mg/pot in T1, T2, and T3 respectively. The effect of water treatments showed a significant (p < .05) effect on the K uptake in grain. Intermittent irrigation produced the highest K uptake in grain compared to continous irrigation. The interaction between temperature and nitrogen treatments was significant (p < .001), however, plants that received nitrogen performed better compared to the control in all different temperature environments. The highest K uptake in grain was observed under the combination of intermittent irrigation and nitrogen application. Uptake of K in the straw in the other two growth environments T2 and T3 were 45% and 73% respectively lower than the uptake in T1. Continuous flooding resulted in lower K uptake compared to those under intermittent irrigation by 116%. The interaction between water and nitrogen significantly influenced the uptake of K in straw.
The relationship between nutrient uptake and the environmental temperature under various water and nitrogen management are illustrated in . Generally, the effect of temperature in reducing the uptake of nutrient in both grain and straw was highest under intermittent irrigation condition when nitrogen was applied. The rate of decline in the uptake of nutrients was generally higher under nitrogen application.
Figure 8. Relationship between nutrient (N, P and K) uptake and environmental temperature under different nitrogen and water management practices in a greenhouse. CFNO, IIRNO, CFN1 and IIRN1 are continuous flooding with no nitrogen application, intermittent irrigation with no nitrogen application, continuous flooding with nitrogen application and intermittent irrigation with nitrogen application.
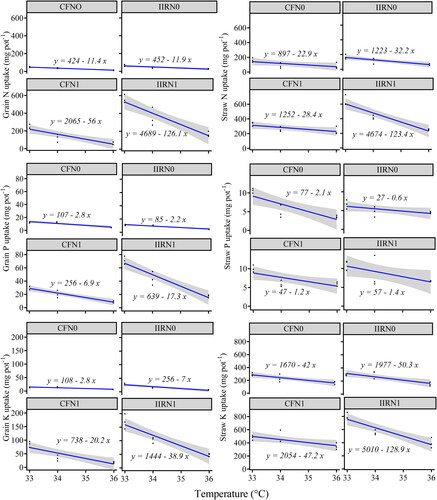
4. Discussion
4.1. Influence of treatment on growth and yield of rice
Temperature and water stress effect on plants manifest in different ways. Under each of these stress conditions, plants processes such as physiology, biochemical, phenology are distrupted or alterted to tolerate the stress with potential consequecies for yield. From the development view, increasing temperature would accelerate development and shorten the life cycle. In this study, there was no clear temperature effect on phenology, and hence was not presented. However, extreme temperatures are known to cause a reduction in tiller numbers and subsequent reduction rice yield (Li et al., Citation2003). Thangasamy et al. (Citation2012) showed that tiller production is a key agronomic trait in rice and is very sensitive to temperature. Several earlier studies (Krishnan et al., Citation2011; MacCarthy et al., Citation2020; Oh et al., Citation2023) found that elevated temperature decreased plant tiller number. The impact of increasing temperatures on tiller numbers was not significant in this study, however, panicle yield reduced significantly as a result of the decline in the proportion of filled grains under higher temperature environment (MacCarthy et al., Citation2020).
Findings from this study also showed a reduction in the above ground biomass under high temperatures. Generally, temperatures beyond of optimum would adversely affect aboveground biomass accumulation as enzymes required to catalyze photosynthesis become less efficient, thereby slowing the rate of photosynthesis. Additionally, plants under go physiological adjustments such as regulating stomatal conductance in response to heat stress and this also negatively affect photosynthesis, and hence, biomass and yield. The Legon 1 rice variety recorded lower dry shoot yield under the high temperature (T3) compared to the ambient temperature (T1). This was consistent with the results of studies conducted by Zhang et al. (Citation2013) who found a reduction in biomass under hot night temperature than lower night temperature. Dubey et al. (Citation2018) also observed that excessive temperature reduced the dry biomass significantly. Similarly, Cheng et al. (Citation2009) reported a reduction in plant height, number of tillers, and total biomass for rice cultivars under high temperature.
Nitrogen application increased the number of tillers as reported by Zhou et al. (Citation2022) and hence, straw and grain yields. Chaturvedi (Citation2005) also reported significant dry matter accumulation with increased N fertilizer application in rice production. We also observed in this study that treatments that received nitrogen had significantly high number of panicles and percent filled grain, and grain yield than the control. This is in agreement with previous studies by (MacCarthy et al., Citation2020; Rezaei et al., Citation2009), who reported that nitrogen fertilizer application increased plant height, panicles per meter square, harvest index, filled grain and grain yield.
The interactive effect among watering regime, nitrogen management and temperature influenced rice yield. In general, our observation showed that intermittent irrigation (W2), nitrogen application (N2) and low temperature (T1) produced the highest rice yield. The increasing temperature was detrimental to grain yield. Previous studies by Cassman et al. (Citation1998) and Liu et al. (Citation2019) indicated that water and N management play an important role in plant growth and their tolerance to temperature stress. Also, proper nitrogen management can mitigate the damaging effects of high temperature on crops. It has been reported that unlike continuously flooded conditions, intermittent irrigation can increase grain yield (Peng & Bouman, Citation2007; Yang et al., Citation2007; Zhang et al., Citation2013) and also is more tolerant to extreme temperature stress (Wichelns, Citation2016). Waraich et al. (Citation2012) indicated that crops with proper nutrition are healthy and, hence, can withstand stresses incurred during the growing season.
Intermittent irrigation has proven in this study to be superior to continuous flooding. In one way, it saved water which is increasing becoming scarce. In another way, the plant appeared to be hardened along the growth period, and tolerated temperature stress better. It is our view that a well designed rice production system on the basis of intermittent irrigation and high N application would be a technology that can sustain rice yields even under extreme climate that is likely under climate change. This not withstanding, the efficiency of nitrogen fertilization will reduce under a warming climate, though it would increase absolute yield.
4.2. Influence of treatments on nutrient uptake
Nitrogen is an important component of protein in a crop. Thus, its uptake in adequate quantities together with other nutrients is important for the quality of the harvested crop. Our study showed a decline in the uptake of nitrogen, phosphorous and potassium in the higher temperature environments. Similarly, nutrient uptake were generally low under water and nitrogen stress. These have a negative implication on the protein content of both grain and straw, and thus grain quality. A number of studies have illustrated that the uptake of nutrients, particularly nitrogen positively influences grain protein content (Lan et al., Citation2021; Wan et al., Citation2023). Thus, the drastic reduction in the uptake of nitrogen suggests the protein content of the grain will be significantly reduced under projected increases in temperature associated with climate change. Till date, empirical data is scarce on the impact of extreme temperature, water and nutrient stress on the growth, yield and quality of rice grain and straw, particularly in sub-Sahara Africa. Thus, this study provides information that will also be useful in calibrating and evaluating various crop models on their sensitivity to simulating the interaction of these three stress factors on yield and the quality of yield, particularly for rice which is now a staple crop in the region. This work therefore contributes to filling this knowledge gap.
5. Conclusion
The main aim of this research was to investigate the performance of rice under varying temperatures, water and nitrogen management conditions, with the view of understanding how rice would perform under climate change. The study has shown that increased temperatures resulted in yield decline regardless of water and nitrogen treatments. The combination of intermittent irrigation and recommended nitrogen improved grain yield significantly compared to the continuous flooding in all the growing temperature conditions. The uptake of nutrients also generally declined with increased temperatures with the magnitude of reduction in the nutrients higher under higher fertilization compared to those unfertilized, thereby suggesting a potential reduction in grain and straw quality under warmer climate, thus, posing a challenge to human nutrition. Even though there are significant decline in rice yield stemming from temperature stress, the benefits from the adoption of intermitent irrigation technology and nitrogen fertilizer management could reduce the adverse temperature effects on grain yield.
About the author and the study
This research paper forms part of an MPhil. Thesis work of the first author, Miss Pauline Niyomugabo, which was supervised by the co-authors, Prof. Dilys Sefakor MacCarthy and Prof. Samuel Godfried Kwasi Adiku. It was a greenhouse study conducted at Soil and Irrigation Research Centre of the University of Ghana, Ghana.
Rice is a vital crop for billions of people around the world and its productivity under adverse effect of climate change is a pressing issue especially in the tropics. Thus, this study explored the effects of extreme temperatures, water availability, and nitrogen levels on the growth and yield of rice. The study advanced the understanding on how rice response to increasing heat, erratic rainfall, and related nitrogen demands. By gleaning insights into crop-climate interactions, we hope our work could enhance breeding and agronomic strategies to ensure food security amid changing climate.
Acknowledgements
The lead author acknowledges the support of the staff of the Soil and Irrigation Research Centre, and the Department of Soil Science in carrying out experiment and laboratory analysis.
Disclosure statement
No potential conflict of interest was reported by the author(s).
Data availability statement
The data that support the findings of this study are available from the corresponding author, [author initials], upon reasonable request.
Additional information
Funding
References
- Adiku, S. (2019). Climate Change: Variability and Biophysical Landuse in Ghana. Afram Publications (Ghana) Limited.
- Amatekpor, J. K., Oteng, J. W., & Agyiri, P. (1993). Field tour guide: Technical Center for Agricultural and Rural Co-operation (CTA). Proceedings of the Sustaining Soil Productivity in Intensive African Agriculture.
- Anim, J. J. (2017). Evaluation of growth and yield of rice (Oryza sativa L.) under different fertilizer management options in three growing ecologies. University of Ghana.
- AOAC. (1990). Official methods, association of official analytical chemists. (12th ed.). AOAC.
- Berry, F. A., Bollay, E., & Beers, N. R. (1945). Handbook of meteorology. McGraw-Hill.
- Brammer, H. (1955). Detailed survey of the Kpong Pilot Irrigation Area. Gold Coast Department of Soil and Landuse Survey. Memoir No. 1, 1–15.
- Bremner, J. M. (1965). Total nitrogen. In A. G. Norman (Ed.), Methods of soil analysis: Part 2 chemical and microbiological properties. SSAA. https://doi.org/10.2134/agronmonogr9.2.c32
- Cassman, K. G., Peng, S., Olk, D. C., Ladha, J. K., Reichardt, W., Dobermann, A., & Singh, U. (1998). Opportunities for increased nitrogen-use efficiency from improved resource management in irrigated rice systems. Field Crops Research, 56(1-2), 7–39. https://doi.org/10.1016/S0378-4290(97)00140-8
- Chaturvedi, I. (2005). Effect of nitrogen fertilizers on growth, yield and quality of hybrid rice (Oryza sativa). Journal of Central European Agriculture, 6(4), 611–618.
- Cheng, W., Sakai, H., Yagi, K., & Hasegawa, T. (2009). Interactions of elevated [CO2] and night temperature on rice growth and yield. Agricultural and Forest Meteorology, 149(1), 51–58. https://doi.org/10.1016/j.agrformet.2008.07.006
- da Silva, E. C., Nogueira, R., da Silva, M. A., & de Albuquerque, M. B. (2011). Drought stress and plant nutrition. Plant Stress, 5(1), 32–41.
- Dabi, T., & Khanna, V. K. (2018). Effect of climate change on rice. Agrotechnology, 7(2), 2–7.
- Dubey, A. N., Verma, S., Goswami, S. P., & Devedee, A. K. (2018). Effect of temperature on different growth stages and physiological process of rice crop-a review. Bulletin of Environment. Pharmacology and Life Sciences, 7(11), 129–136.
- Fahad, S., Bajwa, A. A., Nazir, U., Anjum, S. A., Farooq, A., Zohaib, A., Sadia, S., Nasim, W., Adkins, S., Saud, S., Ihsan, M. Z., Alharby, H., Wu, C., Wang, D., & Huang, J. (2017). Crop production under drought and heat stress: Plant responses and management options. Frontiers in Plant Science, 8, 1147. https://doi.org/10.3389/fpls.2017.01147
- Furtak, K., & Wolińska, A. (2023). The impact of extreme weather events as a consequence of climate change on the soil moisture and on the quality of the soil environment and agriculture–A review. CATENA, 231, 107378. https://doi.org/10.1016/j.catena.2023.107378
- Gbangou, T., Ludwig, F., Van Slobbe, E., Greuell, W., & Kranjac-Berisavljevic, G. (2020). Rainfall and dry spell occurrence in Ghana: Trends and seasonal predictions with a dynamical and a statistical model. Theoretical and Applied Climatology, 141(1-2), 371–387. https://doi.org/10.1007/s00704-020-03212-5
- Ghannoum, O., Evans, J. R., Chow, W. S., Andrews, T. J., Conroy, J. P., & von Caemmerer, S. (2005). Faster Rubisco is the key to superior nitrogen-use efficiency in NADP-malic enzyme relative to NAD-malic enzyme C4 grasses. Plant Physiology, 137(2), 638–650. https://doi.org/10.1104/pp.104.054759
- Gross, E., & Pennink, C. (2018). Impact evaluation of the sustainable water fund (FDW) Integrated water management and knowledge transfer in Sisili Kulpawn basin (FDW/12/GH/02) in the Northern Region of Ghana Final report December 2018. Retrieved March 11, 2024, from https://www.government.nl/binaries/government/documenten/reports/2020/04/30/evaluation-of-projects-co-financed-by-the-sustainable-water-fund-fdw/1++Ghana+IWAD+final+report.pdf
- Krishnan, P., Ramakrishnan, B., Reddy, K. R., & Reddy, V. R. (2011). High-temperature effects on rice growth, yield, and grain quality. Advances in Agronomy, 111, 87–206.
- Lan, Y., Sui, X., Wang, J., Duan, Q., Wu, C., Ding, C., & Li, T. (2021). Effects of nitrogen application rate on protein components and yield of low-gluten rice. Agriculture, 11(4), 302. https://doi.org/10.3390/agriculture11040302
- Li, X., Qian, Q., Fu, Z., Wang, Y., Xiong, G., Zeng, D., Wang, X., Liu, X., Teng, S., Hiroshi, F., Yuan, M., Luo, D., Han, B., & Li, J. (2003). Control of tillering in rice. Nature, 422(6932), 618–621. https://doi.org/10.1038/nature01518
- Liu, K., Deng, J., Lu, J., Wang, X., Lu, B., Tian, X., & Zhang, Y. (2019). High nitrogen levels alleviate yield loss of super hybrid rice caused by high temperatures during the flowering stage. Frontiers in Plant Science, 10, 357. https://doi.org/10.3389/fpls.2019.00357
- MacCarthy, D. S., Akponikpe, P. B. I., Akinseye, F. M., Ly, M., Timpong-Jones, E. C., Hathie, I., & Adiku, S. G. K. (2023). Modelling the impact of climate change on agriculture in West Africa. Nendel, C. (Ed.). Modelling climate change impacts on agricultural systems, pp. 667–712, Burleigh Dodds Science Publishing, Cambridge, UK. http://dx.doi.org/10.19103/AS.2022.0115.21
- MacCarthy, D. S., Darko, E., Nartey, E. K., Adiku, S. G., & Tettey, A. (2020). Integrating biochar and inorganic fertilizer improves productivity and profitability of irrigated rice in Ghana, West Africa. Agronomy, 10(6), 904. https://doi.org/10.3390/agronomy10060904
- MacCarthy, D. S., Hathie, I., Freduah, B. S., Ly, M., Adam, M., Ly, A., Nenkam, A., Traoré, P. S., & Valdivia, R. (2021). Potential impacts of agricultural intensification and climate change on the livelihoods of farmers in Nioro, Senegal, West Africa. https://agritrop.cirad.fr/602251
- MacCarthy, D. S., Kihara, J., Masikati, P., & Adiku, S. G. K. (2018). Decision support tools for site-specific fertilizer recommendations and agricultural planning in selected countries in Sub-Sahara Africa. In A. Bationo, D. Ngaradoum, S. Youl, F. Lompo, & J. O. Fening (Eds.), Improving the profitability, sustainability and efficiency of nutrients through site specific fertilizer recommendations in West Africa Agro-Ecosystems (pp. 265–289). Springer International Publishing. https://doi.org/10.1007/978-3-319-58792-9_16
- Makino, A., Sakuma, H., Sudo, E., & Mae, T. (2003). Differences between maize and rice in N-use efficiency for photosynthesis and protein allocation. Plant & Cell Physiology, 44(9), 952–956. https://doi.org/10.1093/pcp/pcg113
- Mawunya, F. D., & Adiku, S. G. K. (2013). Implications of climate change for Agricultural Productivity in Ghana: An Agrometeorological perspective. Ghana Policy Journal, 5 (4), 7–26.
- Mendes, D. M., Jackson, D., Altozano, A. G., & Paglietti, L. (2014). Ghana: Irrigation market brief. Food and Agriculture Organization of the United Nations.
- MoFA-IFPRI. (2020). Ghana’s rice market. MoFA-IFPRI Market Brief No, 2, 800.
- Monteith, J., & Unsworth, M. (2013). Principles of environmental physics: Plants, animals, and the atmosphere. Academic press.
- Mu, X., & Chen, Y. (2021). The physiological response of photosynthesis to nitrogen deficiency. Plant Physiology and Biochemistry, 158, 76–82. https://doi.org/10.1016/j.plaphy.2020.11.019
- Nadeem, M., Li, J., Yahya, M., Sher, A., Ma, C., Wang, X., & Qiu, L. (2019). Research progress and perspective on drought stress in legumes: A review. International Journal of Molecular Sciences, 20(10), 2541. https://doi.org/10.3390/ijms20102541
- Nyasapoh, J. B. A., Adiku, S. G. K., MacCarthy, D. S., & Yanore, S. A. (2022). Mode of biochar application to vertisols influences water balance components and water use efficiency of maize (Zea mays L.). West African Journal of Applied Ecology, 30(1), 1–12.
- Oh, D., Ryu, J.-H., Jeong, H., Moon, H.-D., Kim, H., Jo, E., Kim, B.-K., Choi, S., & Cho, J. (2023). Effect of elevated air temperature on the growth and yield of paddy rice. Agronomy, 13(12), 2887. https://doi.org/10.3390/agronomy13122887
- Parkes, B., Buzan, J. R., & Huber, M. (2022). Heat stress in Africa under high intensity climate change. International Journal of Biometeorology, 66(8), 1531–1545. https://doi.org/10.1007/s00484-022-02295-1
- Pascual, V. J., & Wang, Y.-M. (2016). Impact of water management on rice varieties, yield, and water productivity under the system of rice intensification in Southern Taiwan. Water, 9(1), 3. https://doi.org/10.3390/w9010003
- Peng, S., & Bouman, B. A. M. (2007). Prospects for genetic improvement to increase lowland rice yields with less water and nitrogen. In J. H. J. Spiertz, P. C. Struik, & H. H. van Laar (Eds.), Scale and Complexity in Plant Systems Research: Gene-Plant-Crop Relations (pp. 251–266). Springer.
- Rezaei, E. E., Webber, H., Asseng, S., Boote, K., Durand, J. L., Ewert, F., Martre, P., & MacCarthy, D. S. (2023). Climate change impacts on crop yields. Nature Reviews Earth & Environment, 4(12), 831–846. https://doi.org/10.1038/s43017-023-00491-0
- Rezaei, M., Vahed, H. S., Amiri, E., Motamed, M. K., & Azarpour, E. (2009). The effects of irrigation and nitrogen management on yield and water productivity of rice. World Applied Sciences Journal, 7(2), 203–210.
- Sanderson, M. A., Stair, D. W., & Hussey, M. A. (1997). Of perennial forages to stress. Advances in Agronomy, 59, 171.
- Serdeczny, O., Adams, S., Baarsch, F., Coumou, D., Robinson, A., Hare, W., Schaeffer, M., Perrette, M., & Reinhardt, J. (2017). Climate change impacts in Sub-Saharan Africa: From physical changes to their social repercussions. Regional Environmental Change, 17(6), 1585–1600. https://doi.org/10.1007/s10113-015-0910-2
- Singh, S., Sharma, R. K., Singh, G., Singh, S. S., Singh, U. P., Gill, M. S., Jat, M. L., Sharma, S. K., Malik, R. K., & Josan, A. S. (2005). Direct seeded rice: A promising resource conserving technology. Rice-Wheat Consortium for Indo-Gangetic Plains. NASC Complex. 11–12.
- Tang, J., Sun, B., Cheng, R., Shi, Z., Luo, D., Liu, S., & Centritto, M. (2019). Effects of soil nitrogen (N) deficiency on photosynthetic N-use efficiency in N-fixing and non-N-fixing tree seedlings in subtropical China. Scientific Reports 9(1), 4604. https://doi.org/10.1038/s41598-019-41035-1
- Thangasamy, S., Bolaños-Villegas, P., & Jauh, G. Y. (2012). Branching pattern: An inevitable trait for rice production. In Yishihiro Liu & Liliana Froyen (Eds.), Rice: Production, consumption and health benefits. Nova Science Publishers, Inc. https://ir.sinica.edu.tw/handle/201000000A/13359
- Tuong, P., Bouman, B. A. M., & Mortimer, M. (2005). More rice, less water—Integrated approaches for increasing water productivity in irrigated rice-based systems in Asia. Plant Production Science, 8(3), 231–241. https://doi.org/10.1626/pps.8.231
- Venkatesan, G., Selvam, M. T., Swaminathan, G., & Krishnamoorthi, S. (2005). Effect of water stress on yield of rice crop. International Journal of Ecology & Development, 3(3), 77–89.
- Wan, C., Gao, L., Wang, J., Lei, X., Tao, J., Feng, B., & Gao, J. (2023). Effects of nitrogen fertilizer on protein synthesis, accumulation, and physicochemical properties in common buckwheat. The Crop Journal, 11(3), 941–950. https://doi.org/10.1016/j.cj.2023.01.002
- Waraich, E. A., Ahmad, R., Halim, A., & Aziz, T. (2012). Alleviation of temperature stress by nutrient management in crop plants: A review. Journal of Soil Science and Plant Nutrition, 12(2), 221–244. https://doi.org/10.4067/S0718-95162012000200003
- Wichelns, D. (2016). Managing water and soils to achieve adaptation and reduce methane emissions and arsenic contamination in Asian rice production. Water, 8(4), 141. https://doi.org/10.3390/w8040141
- Wiebbecke, C. E., Graham, M. A., Cianzio, S. R., & Palmer, R. G. (2012). Day temperature influences the male-sterile locus ms9 in soybean. Crop Science, 52(4), 1503–1510. https://doi.org/10.2135/cropsci2011.08.0410
- Wigboldus, S., Guijt, J., & Garcia-Campos, P. (2021). Rwanda’s journey towards sustainable food systems: The processes and practices that made a difference. Food & Agriculture Org.
- Xiong, Q., Tang, G., Zhong, L., He, H., & Chen, X. (2018). Response to nitrogen deficiency and compensation on physiological characteristics, yield formation, and nitrogen utilization of rice. Frontiers in Plant Science, 9, 1075. https://doi.org/10.3389/fpls.2018.01075
- Xu, Z., Jiang, Y., & Zhou, G. (2015). Response and adaptation of photosynthesis, respiration, and antioxidant systems to elevated CO2 with environmental stress in plants. Frontiers in Plant Science, 6, 701. https://doi.org/10.3389/fpls.2015.00701
- Yang, J., Liu, K., Wang, Z., Du, Y., & Zhang, J. (2007). Water-saving and high-yielding irrigation for lowland rice by controlling limiting values of soil water potential. Journal of Integrative Plant Biology, 49(10), 1445–1454. https://doi.org/10.1111/j.1672-9072.2007.00555.x
- Yang, Z., Zhang, Z., Zhang, T., Fahad, S., Cui, K., Nie, L., Peng, S., & Huang, J. (2017). The effect of season-long temperature increases on rice cultivars grown in the central and southern regions of China. Frontiers in Plant Science, 8, 1908. https://doi.org/10.3389/fpls.2017.01908
- Zhang, Y., Tang, Q., Peng, S., Zou, Y., Chen, S., Shi, W., Qin, J., & Laza, M. R. C. (2013). Effects of high night temperature on yield and agronomic traits of irrigated rice under field chamber system condition. Australian Journal of Crop Science, 7(1), 7–13.
- Zhou, W., Yan, F., Chen, Y., & Ren, W. (2022). Optimized nitrogen application increases rice yield by improving the quality of tillers. Plant Production Science, 25(3), 311–319. https://doi.org/10.1080/1343943X.2022.2061538