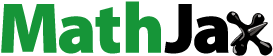
Abstract
The nature of dark matter (DM) is one of the most intriguing questions in particle physics. DM can be postulated to be part of a hidden sector whose interactions with the visible matter are not completely decoupled. The discovery of a fundamental scalar particle compatible with the Higgs boson predicted by the Standard Model paves the way for looking for DM with novel methods. An overview of the searches looking for a hidden sector in exotic Higgs decays and for invisible decays of the Higgs boson within the ATLAS experiment is presented. Prospects for searches with Large Hadron Collider data at a center-of-mass energy of 13 TeV are summarized.
Public Interest Statement
The nature of dark matter is one of the most intriguing questions in modern physics. The discovery of a fundamental particle compatible with the Higgs boson predicted by the Standard Model was announced at the CERN laboratory in Geneva in July 2012. The newly found Higgs boson particle paves the way for looking for dark matter with novel methods for the experiments at CERN. An overview of the searches conducted so far by the ATLAS collaboration is reviewed in this contribution.
1. Introduction
There is an overwhelming evidence for dark matter (DM) in the universe but its particle nature and the form of its non-gravitational interactions are unknown. Experiments at hadron colliders traditionally search for DM by looking at collisions with a large missing transverse momentum and some high- object, as for example, a parton emitted as initial-state radiation, a vector boson, a photon, or a top quark pair. Legacy results of the ATLAS experiment using 8 TeV proton–proton collisions have been recently published and found no evidence of DM candidates (ATLAS Collaboration, Citation2014a,Citation2014b,Citation2015a,Citation2015b,Citationin press).
The newly discovered Higgs boson (ATLAS Collaboration, Citation2012a; CMS Collaboration, Citation2012) is the last missing piece of the Standard Model (SM) and offers new exciting opportunities for looking for DM at the Large Hadron Collider (LHC). The experimental investigation of this new fundamental state has just begun and the Higgs boson may decay to non-SM particles. When including constraints from observing the Higgs boson to SM decay channels, branching ratios to non-SM states as high as are allowed (Curtin, Citation2014). Projections of the precise measurements of the Higgs boson properties at the high-luminosity LHC, a project for delivering about 3000 fb
of 14 TeV proton collisions by 2030, predict a precision on the cross-section of the
decay channel of
(ATLAS Collaboration, CitationXXXX). Therefore, Higgs boson branching fractions of
into exotic decay modes are not only still allowed by existing data, but will remain reasonable targets for the next decade. In addition, the Higgs boson has an extremely narrow width in the SM,
MeV, meaning that even a small coupling to a non-SM light state can easily open up new sizable decay modes.
Recent searches within the ATLAS experiment looking for exotic Higgs decays are reviewed. The searches may also be interpreted in other theoretical contexts. Three searches are detailed: a search looking for displaced vertices, a search looking for displaced lepton-jets, and a search looking for invisible decays of the Higgs boson. Only the third analysis requires some missing transverse momentum in the search strategy. If not stated otherwise, the searches used 20.3 fb of data collected in proton–proton collisions at
TeV.
2. The ATLAS detector
ATLAS is a general purpose detector, designed for studying proton–proton collisions at the LHC. It consists of three main components almost covering the entire solid angle and centered around the nominal interaction point: the inner detector tracking system, the calorimeters, and the muon system. The inner detector is immersed in a solenoidal magnetic field and the magnetic field in the muon system is generated by three large toroidal magnets. A detailed description of the ATLAS detector has been published elsewhere (ATLAS Collaboration, Citation2008).
3. Displaced decays in the hadronic calorimeters
The search looks for two displaced decays of neutral particles to jets in the hadronic calorimeters (ATLAS Collaboration, Citation2015c). The model considered is a hidden sector weakly coupled to the SM through a communicator particle. The communicator is chosen to be a SM-sector scalar boson mixing with the hidden sector scalar boson
, which decays back into detectable SM particles. The search considers communicator masses between 100 and 900 GeV and includes a search for exotic decays of the Higgs boson. A hidden sector naturally includes DM candidates. The specific benchmark model is a Hidden Valley model where the lightest particles,
, form a isospin triplet of pseudoscalar particles. The process studied is therefore the Higgs boson decaying to two
particles. The
decay is via Yukawa coupling and therefore proportional to the fermion masses of the decay products.
The lifetime of the is unconstrained and can give rise to displaced decays within the ATLAS volume. If the decay is inside the hadronic calorimeter or near the outer edge of the electromagnetic calorimeter, a jet with an unusual energy deposition is reconstructed.
Two jets are searched for with three characteristic properties: a narrow radius, the absence of reconstructed tracks matched to the jet, and little or no energy deposited in the portion of the electromagnetic calorimeter matched to the jet. These properties are employed for both the triggering and the search strategy. Details on the dedicated trigger can be found in (ATLAS Collaboration, Citation2013a). The distribution of the number of tracks associated to the jets and of the logarithmic ratio of the energy deposited in the hadronic calorimeter to that in the electromagnetic calorimeter is shown in Figure for signal and background events.
Figure 1. Distribution of the number of tracks (left) and the logarithmic ratio of the energy deposited in the hadronic and in the electromagnetic calorimeters (right). Notes: The dashed (dotted) histogram is for jets originating from decays in the hadronic calorimeter (inner detector). The filled histogram is for multi-jet data events used for estimating the background in the signal region.
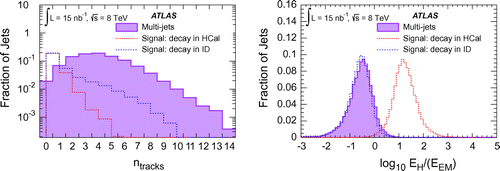
Figure 2. Observed 95% confidence level limits on the exotic Higgs decays to pairs. Note: The production cross-section is taken to be 19.0 pb.
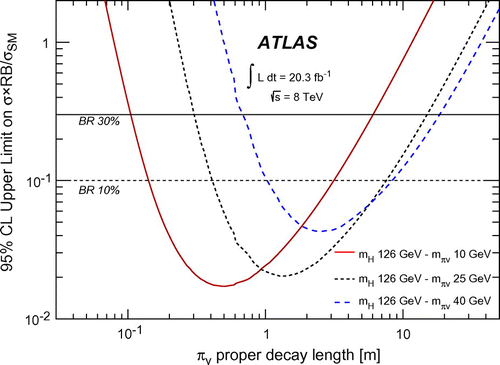
The background composition is mainly composed of multi-jet events. Backgrounds due to cosmic ray and beam–halo interactions are also considered. The multi-jet background is calculated deriving the probability that a jet passes the trigger and the analysis selection. A correction to account for two-jet correlations is applied by evaluating this probability separately for leading and sub-leading jets. The final background prediction is events in the signal region with an uncertainty dominated by the statistics in a control sample selected by the dedicated trigger employed in the search. The number of observed events in data is 24 and is compatible with the expected background. The 95% confidence level upper limits on the Higgs boson branching ratio to a
pair are derived as a function of the
lifetime and shown in Figure . For a
mass of 25 GeV, lifetimes between 0.3 m and 14.99 m are excluded assuming a 30% branching ratio of the Higgs boson and between 0.41 m and 7.57 m are excluded assuming a 10% branching ratio.
4. Displaced lepton-jets
The search looks for displaced lepton-jets within the ATLAS detector volume (ATLAS Collaboration, Citation2014c). Lepton-jets are defined as collimated jet-like objects containing a mixture of electron, muon, and pion pairs. This signature arises when a light neutral particle, that is highly boosted in the laboratory frame, decays.
The dark photon, , is the vector gauge boson corresponding to an additional sector to the SM characterized by
symmetry. A mixing parameter
is introduced providing a natural connection between the SM and the hidden sector. The
parameter controls both the lifetime and the decay branching ratios of the dark photon. Due to their weak couplings, dark photons can travel a measurable distance before decaying to lepton-jets.
The search adopts a generic definition of lepton-jet. Model-independent simulated samples of a dark photon decaying to a lepton-jet and a hidden scalar decaying to two dark photons, each decaying to a lepton-jet are studied. Dark photons with different kinematic properties and with decays to electrons, muons, and pions are considered. The optimal size of the search cone is found to be around the lepton-jet line of flight.
Three categories are built as shown in the schematic pictures of Figure . A lepton-jet of TYPE0 is built if at least two tracks in the muon spectrometer and no jets are found in the same cone. The lepton-jet is of TYPE1 if there are at least two tracks in the muon spectrometer and only one jet in the same cone and of TYPE2 if a jet with electromagnetic fraction less than 0.4 is found with no muons in the same cone. TYPE0 lepton-jets are sensitive to decays to muons beyond the pixel detector and up to the first trigger plane of the muon spectrometer. TYPE1 lepton-jets are sensitive to
decays to both muon pair and electron/pion pair in the same cone beyond the pixel detector to the end of the hadronic calorimeter. TYPE2 lepton-jets are sensitive to
decays to electron/pion pairs in the hadronic calorimeter.
Figure 3. Schematic pictures of the lepton-jet classification according to the decay products. Notes: Three flavors are considered: lepton-jets formed of only muons (TYPE0), a mixed scenario with both muons and a jet (TYPE1), or only a jet (TYPE2).
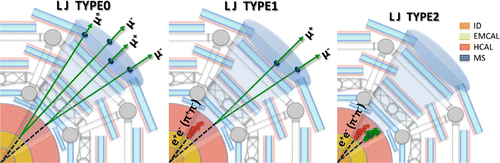
Events are selected asking for at least two lepton-jet candidates. Additional requirements are imposed to further suppress the background. Cuts are applied on the jet width, the jet timing, the isolation with respect to tracks, and the between the two candidates. A total of 119 events survive all the cuts in the full data sample for all possible combinations of lepton-jet types.
The main backgrounds are multi-jet production and cosmic ray muons traversing the detector in coincidence with a proton–proton interaction. Events in empty bunch crossings are employed to estimate a background due to cosmic rays of events. The multi-jet background is evaluated using a data-driven method and estimated in
events. The majority of the background is for the events with two TYPE2 lepton-jets.
Figure 4. Parameter space exclusion plot for dark photons as a function of the kinetic mixing parameter and of the mass. Notes: Shown are existing 90% CL exclusion regions from beam-dump or fixed-target experiments, as well as low-energy electron colliders, astrophysical observations, and data on the anomalous magnetic moments of the electron and the muon. The plot includes the ATLAS exclusion limit in a previously unexplored region, looking at Higgs boson decays to displaced lepton-jets. The NNLO gluon fusion Higgs production cross-section is used and decay branching ratios to dark photons of 5, 10, 20, and 40% are assumed.
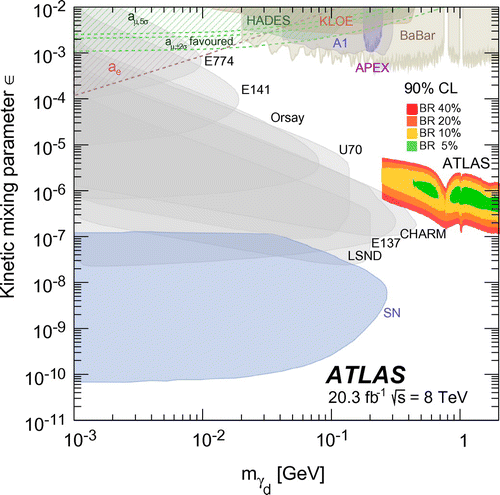
Data are interpreted in the context of non-SM Higgs boson decays to lepton-jets. Two benchmarks are considered. The Higgs boson decays to a pair of hidden fermions, each decaying to a dark photon and to a lighter hidden fermion (). The second model considers an additional step in the decay chain of the hidden sector resulting in four dark photon decays to lepton-jets (
). The number of events in data is 119 and is compatible with the expected background. Upper limits on the Higgs boson decay branching ratio to lepton-jets are set as a function of the
mean lifetime. Assuming a 10% branching ratio, dark photons are excluded in the range between 14 and 140 mm for the process
and between 15 and 260 mm for the process
. The result is also interpreted as exclusion contours in the kinetic mixing parameter
and the
mass plane, as shown in Figure . Masses are considered in the interval between 0.25 and 1.5 GeV and assuming Higgs boson branching ratios to lepton-jets from 40 to 5%. The ATLAS result, assuming a non-SM decay of the Higgs boson, is able to exclude a previously unexplored region of the parameter space.
5. Invisible decay
The search looks for a Higgs boson produced in association with a boson and decaying into two particles with very small interaction cross-sections with SM particles (ATLAS Collaboration, Citation2014d). Similar to other searches presented in this contribution, the analysis strategy is also sensitive to additional scalar bosons. The search employed the full data-set at both 7 and 8 TeV.
Events are required to pass the single-lepton or lepton-pair triggers. The invariant mass of the selected dilepton system is then required to be consistent with the leptons originating from a boson decay. The missing transverse momentum after the dilepton mass requirement is shown on the left plot in Figure . The data are consistent with the expected background based on simulated samples for all but the multi-jet background. Additional cuts are imposed when looking at data such as a requirement on the missing transverse momentum to be greater than 90 GeV and a requirement on the azimuthal separation between the dilepton system and the missing transverse momentum. After all selection criteria, the main background is the production of
and
as shown in the right plot in Figure .
Background is estimated with data-driven techniques and data are found compatible with the expected background. Limits are set on the cross-section times branching ratio for a scalar boson decaying to invisible particles anywhere in the mass range between 110 and 400 GeV. For the Higgs boson, an upper limit of 75% at 95% confidence level is derived on the branching ratio to invisible particles.
Figure 5. Distribution of the missing transverse momentum for the events with the invariant mass of the dilepton system consistent with the leptons originating from a boson decay (left) and after the full selection (right).
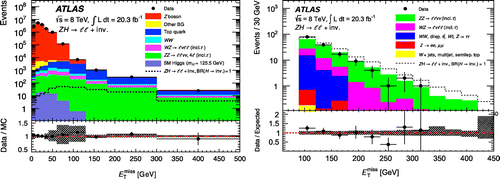
Figure 6. Limits on the DM–nucleon scattering cross-section at 90% confidence level, extracted from the branching ration limit in a Higgs portal scenario and compared to results from direct-search experiments. The results from the direct-search experiments do not depend on the assumptions of the Higgs portal scenario.
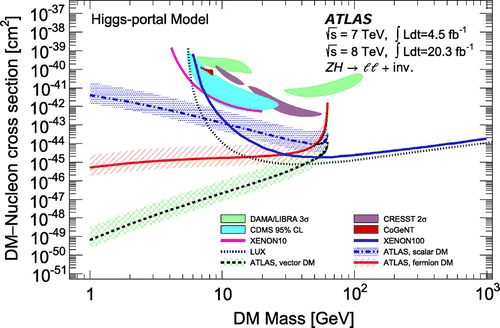
Within the context of a Higgs portal scenario, an upper limit on the DM–nucleon scattering cross-section is reported. Figure shows the 90% confidence level upper limits on the DM–nucleon scattering cross-section for three model variants in which a single DM candidate is considered and is either a scalar, a vector, or a Majorana fermion. Spin-independent results from direct-search experiments are also shown. Within the constraints of the considered scenario, the results at low-mass DM candidates are stronger than the ones obtained from direct-search experiments.
6. Conclusion and prospects
The Higgs boson can provide a unique window onto light-hidden sectors, which naturally include DM candidates. Recent results by the ATLAS collaboration are reviewed in this contribution where events with unconventional signatures are searched for and interpreted in the context of exotic Higgs decays. A search for Higgs boson decaying to particles escaping the detection is also reviewed.
Much more searches targeting this phenomenology can be conceived, some are ongoing within the collaboration and others are being planned for the upcoming LHC run. For example, displaced decays can be searched for with techniques based on sub-detectors different than the hadronic calorimeter, as ATLAS did with the search at TeV looking at displaced vertices in the muon spectrometer (ATLAS Collaboration, Citation2012b). The search employed a dedicated reconstruction technique (ATLAS Collaboration, Citation2014e). Dark photon searches can be conducted also looking at prompt lepton-jets. The feasibility of this search is demonstrated by another ATLAS search at
TeV (ATLAS Collaboration, Citation2013b). Dark photons with higher masses should be also searched for in exotic Higgs decays. Finally, searches for invisible Higgs bosons are being conducted in other production channels such as via vector boson fusion.
The imminent LHC run at a center-of-mass energy of 13 TeV will provide unprecedented opportunities for looking into exotic Higgs boson decays. The Higgs boson is uniquely sensitive to the potential existence of new weakly coupled particles that could explain the existence of DM.
Additional information
Funding
Notes on contributors
Andrea Coccaro
Andrea Coccaro is a member of the ATLAS experiment at the CERN Large Hadron Collider. His research interests in the past years mainly focused on the searching for new physics by looking at displaced decays of long-lived neutral particles. He is particularly interested in looking for exotic decays of the Higgs boson particle. The Higgs boson can provide a unique window onto light-hidden sectors, which would explain the existence of DM. The research reported in this contribution is conducted by the ATLAS collaboration with key contributions by the author.
Notes
This article is associated with the From Higgs to Dark Matter 2014 meeting in Geilo, Norway.
On behalf of the ATLAS Collaboration.
References
- ATLAS Collaboration. (2008). The ATLAS experiment at the CERN Large Hadron Collider. Journal of Instrumentation, 3, S08003.
- ATLAS Collaboration. (2012a). Observation of a new particle in the search for the standard model Higgs boson with the ATLAS detector at the LHC. Physical Letters B, 716, 1–29.
- ATLAS Collaboration. (2012b). Search for a light Higgs boson decaying to long-lived weakly-interacting particles in proton–proton collisions at √s = 7 TeV with the ATLAS detector. Physical Review Letters, 108, 251801.
- ATLAS Collaboration. (2013a). Triggers for displaced decays of long-lived neutral particles in the ATLAS detector. Journal of Instrumentation, 8, P07015.
- ATLAS Collaboration. (2013b). A search for prompt lepton-jets in pp collisions at √s = 7 TeV with the ATLAS detector. Physical Letters B, 719, 299–317.
- ATLAS Collaboration. (2014a). Search for dark matter in events with a Z boson and missing transverse momentum in pp collisions at √s = 8 TeV with the ATLAS detector. Physical Review D, 90, 012004.
- ATLAS Collaboration. (2014b). Search for dark matter in events with a hadronically decaying W or Z boson and missing transverse momentum in pp collisions at √s = 8 TeV with the ATLAS detector. Physical Review Letters, 112(4), 041802.
- ATLAS Collaboration. (2014c). Search for long-lived neutral particles decaying into lepton jets in proton–proton collisions at √s = 8 TeV with the ATLAS detector. Journal of High Energy Physics, 1411, 088.
- ATLAS Collaboration. (2014d). Search for invisible decays of a Higgs boson produced in association with a Z boson in ATLAS. Physical Review Letters, 112, 201802.
- ATLAS Collaboration. (2014e). Standalone vertex finding in the ATLAS muon spectrometer. Journal of Instrumentation, 9, P02001.
- ATLAS Collaboration (2015a). Search for new phenomena in events with a photon and missing transverse momentum in pp collisions at √s = 8 TeV with the ATLAS detector. Physical Review D, 91(1), 012008.
- ATLAS Collaboration (2015b). Search for dark matter in events with heavy quarks and missing transverse momentum in pp collisions with the ATLAS detector. European Physical Journal C, 75, 92.
- ATLAS Collaboration. (2015c). Search for pair-produced long-lived neutral particles decaying to jets in the ATLAS hadronic calorimeter in pp collisions at √s = 8 TeV. Physical Letters B, 743, 15–34.
- ATLAS Collaboration. (XXXX). Projections for measurements of Higgs boson signal strengths and coupling parameters with the ATLAS detector at a HL-LHC, ATL-PHYS-PUB-2014-016.
- ATLAS Collaboration. (in press). Search for new phenomena in final states with an energetic jet and large missing transverse momentum in pp collisions at s = 8 TeV with the ATLAS detector. arXiv:1502.01518.
- CMS Collaboration. (2012). Observation of a new boson at a mass of 125 GeV with the CMS experiment at the LHC. Physical Letters B, 716, 30–61.
- Curtin, D., Essig, R., Gori, S., Jaiswal, P., Katz, A., Liu, T., ... Zhong, Y.-M. (2014). Exotic decays of the 125 GeV Higgs boson. Physical Review D, 90, 075004.