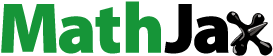
Abstract
New Higgs scalars with masses up to 10 GeV are predicted in models such as the NMSSM, and in scenarios with hidden sectors that couple weakly to the Standard Model. Searches at B factories have resulted in tight constraints on such models. We review the recent searches and new results, and discuss the future outlook for this physics.
Keywords:
Public Interest Statement
The Astronomers tell us that the universe is full of dark matter, but the particle physicists don’t know what it is. Perhaps the new particles will only be seen at the LHC. But perhaps they can be seen in data from existing particle accelerators if you look hard enough and cleverly enough. This note describes searches that have been made recently by two “B-factory” experiments in Japan and the USA, and what the results tell us.
1. The B factories
The B factories: PEP-II at Stanford and KEK-B at Tsukuba, were built in the 1990s as asymmetric electron–positron colliders with a centre-of-momentum system energy around 10 GeV, aimed at producing large numbers of and
mesons through the decay of the
. Together with their associated detectors, BABAR and Belle, their goal was firstly to establish whether CP violation, which at the time had only been observed in
mesons, also existed in the B system, and secondly, if that were the case, to measure its effects to high accuracy in many different channels, to establish whether the theoretical explanation and prediction of Kobayashi and Maskawa (Citation1973) that the effect was due to mixing between three quark generations, was correct and sufficient, or whether some further source of CP violation, perhaps, related to the cosmic matter–antimatter asymmetry, was needed.
This project was a spectacular success, and Kobayashi and Maskawa received the Nobel prize in 2008. As the press release put it, “As late as 2001, the two particle detectors BaBar at Stanford, USA and Belle at Tsukuba, Japan, both detected broken symmetries independently of each other. The results were exactly as Kobayashi and Maskawa had predicted almost three decades earlier” (Retrieved February 18, 2015, from http://www.nobelprize.org/nobel_prizes/physics/laureates/2008/press.html). A full account of the physics discoveries of both factories, and the unsuccessful attempt to find CP violation that could not be accommodated in the Kobayashi–Maskawa scheme, can be found in Bevan et al. (Citation2014).
Although B physics was their primary purpose, the factories also produced many results in other areas of particle physics: charm and physics in particular; these can also be found in Bevan et al. (Citation2014). The purpose of this note is to survey their impact on the subject of the conference: light Higgs bosons and dark matter candidates.
Although these machines are not at the “energy frontier” where most discoveries are made, they explore large new areas of the second “precision” frontier. The accurate measurement of CP violation in B mesons required high statistical precision from enormous samples of events. The design luminosities of seemed very challenging when they were set, as the CLEO-II ring at Cornell and only achieved
. That these goals were met and exceeded, with luminosities above
being regularly achieved by both machines, is a matter of pride for the machine builders. The handling of the enormous data samples also required a complete paradigm shift in the DAQ and data processing systems. This was made possible by the emergence of the “farm” of commodity computers as the replacement for the computer mainframe, and helped by the adoption of a modern computer language, C++, to finally break free of the domination of Fortran. This provided a useful and perhaps necessary intermediate system to meet the challenge of data handling at the LHC.
The data samples grew rapidly; the integrated luminosity is shown as a function of time in Figure .
The events are ideal for many sort of physics studies. As these are collisions, the interacting fundamental particles contain the full energy of the beam (unlike quarks in the LHC). There are no “spectator” effects, no multiple collisions and no “residual event”. The asymmetry of the collisions, the fact that the cms is moving in the lab at 0.5 c, though essential for B lifetime measurements, is not helpful in any other channel, but on the other hand, it is not a hindrance either. The detectors cover most, though not all, of
and comprise the usual layered structure of vertex chamber, tracking chamber, Cherenkov detector, electromagnetic calorimeter and hadron calorimeter/muon filter. The design was dictated by the needs of B physics program, and so the vertex detection is excellent, the electromagnetic calorimetry has very good energy resolution, and the
separation is exceedingly good. These features may be borne in mind when considering other physics analyses.
2. Dark matter particles
Astronomical observations of the motion of stars and galaxies have long suggested the existence of as yet unknown species of elementary particle. These are not part of the Standard Model, but the many Beyond-the-Standard-Model theories offer a wide range of candidates.
The reason these have evaded detection so far may be due to their having a large mass: dark fermions, particularly those predicted by supersymmetry, generally fall into this category, and to discover these an energy frontier machine is needed. Or the dark matter particle(s) may be light, but have not yet been observed due to their weak couplings. However, in many models, the dark matter particle(s) is part of a whole sector of new particles, some of which could be detected.
The dark photon is one such candidate. If, as proposed by Fayet (Citation1980), there is a sector of dark matter WIMP particles which do not decay to known particles due to conservation of some new charge, there can be a new gauge boson, the
. Kinetic mixing generates a small coupling
, between this dark photon and the conventional photon. Such a particle is not itself dark, despite the name, but provides a “portal”, interacting with both the dark and the normal sectors. This particular model has aroused interest recently as it provides a possible explanation for the excess of positrons reported by AMS (Aguilar et al., Citation2013): dark fermions annihilate to produce dark photons, which then decay to particle/antiparticle pairs. However, to fit this explanation, the mass of the dark photon must be small, below a few GeV, or it would also give a cosmic
excess, which is not seen.
In such a model the dark photon mass is generated by a Higgs mechanism, and there is a new Higgs particle, , the dark Higgs; this is also a “portal” particle. Similar particles occur in other models with two or more Higgs doublets, as a light scalar h or pseudoscalar
. The experimental searches are sensitive to all such particles: basically a result detects, or puts a limit on, the presence of a particle at a particular mass decaying into two fermions; this can then be interpreted in terms of the parameters of a particular model.
This note covers searches for particles through their production; there is also a wealth of evidence from indirect searches, particularly FCNC b decays, where virtual BSM particles can interfere with the . These decays, such as
and
, provide powerful constraints on the models, which can be found in Bevan et al. (Citation2014).
3. Searches
We consider searches in five channels: ISR production, decays, dark Higgsstrahlung, narrow gauge bosons and long-lived particles. Full details can be found in the references.
3.1. ISR production
If there is a dark photon (or other state coupling to electrons), with a mass below the cms energy, then initial state radiation down to that mass, as depicted in Figure , has a characteristic monochromatic photon as a signature, as well as any decay products from the particle itself. This enables a scan of the mass region for any possible or similar particle that decays to a state with a clear signature, such as two muons or two electrons.
BABAR has performed this analysis (Lees et al., Citation2014) and there is no sign of any peak in the range in either channel (apart from the well-known
and
resonances), as can be seen in Figure . There is a disagreement in the low-mass electron pairs, but this particular Monte Carlo is not tuned for this region. The Monte Carlo is not used in the extraction of the rests.
From this lack of signal, one can set upper limits set on the dark matter coupling parameter at the level on level
, depending on the mass of the
. The interesting point about this exclusion plot (Figure ) is that it excludes almost all of the remaining regions of parameter space favoured by
discrepancy.
3.2. Production in 
decays
The is narrow, due to Zweig’s rule, and rare decays can in principle be observed. The b and
quarks may produce a dark photon (or other particle), emitting a monochromatic photon to take away the excess energy, as depicted in Figure . The
decays to a fermion–antifermion pair. The object produced could be a dark photon or a NMSSM CP-odd Higgs: the difference lies in the couplings to the different channels.
Searches can be performed by running at the energy and looking for the channels in question. A refinement of this technique is to run at the
or
energy, and the radiative decay
provides a characteristic tag, in the form of the two slow pions, that show that a
has been formed. These events are then analysed for a
signal.
BABAR have performed a complete set of such tagged searches in the different channels, including invisible decays to light dark matter. The final analysis, has been recently completed (Lees et al., Citationin press). In this—as in all other such searches—there is no evidence for any signal and limits can be placed on the product branching ration, as shown in Figure .
The lack of any signal for a particle below 10 GeV does not rule out its existence: the result could be due to small coupling constants. However, the current results rule out large areas of NMSSM parameter space, and the limit , said to be “generally preferred” in the NMSSM, is effectively excluded.
3.3. Dark Higgsstrahlung
If the dark Higgs and the dark photon are both light, with , then the “Higgstrahlung” process through a virtual
,
, can be followed by
, as depicted in Figure . This gives three
particles in the final state, each decaying to a pair of fermions, generally written
although, if the fermion is a quark, the resulting particle is typically a pion.
BABAR and Belle have searched for such events (Lees et al., Citation2012; Jaegle et al., Citation2015), reconstructing all three pairs of particles (or two pairs, with a compatible missing mass) for the ,
and
decay possibilities (though not the
mode, due to large backgrounds). Results presented involve the parameter
, the ‘dark coupling constant’, as well as
and
and are presented differently by the two experiments, as shown in Figures and . There is no evidence for any signal, and again limits can be set on model parameters.
3.4. Dark Gauge Bosons
Further, non-Abelian symmetries will give rise to more (electrically neutral) gauge bosons: , which could be narrow with low mass, but that have evaded detection so far through the small size of the coupling constant.
These would be a pair produced in the reaction , and BABAR has searched for these in the leptonic decay modes
(Aubert et al., Citation2009). No signal is seen and the resulting limits on
are shown in Figure , where, as stated earlier,
is the coupling between the dark and the conventional photon and
is the coupling constant within the dark sector itself.
3.5. Long-lived dark Higgs searches
Belle also considers cases where the or the
particles produced in dark Higgsstrahlung have long lifetimes, travelling mm/cm before decay. Such lifetimes are possible if the coupling is small enough, and the low masses mean that dark channels are not available. This requires a separate analysis as the decays are not “prompt” and do not form part of the main vertex. Results (Jaegle, Citation2012) are still preliminary but a final analysis is expected soon. They are shown in Figure .
Figure 11. Belle limits on the production of long-lived dark photon and dark Higgs particles, considering different lepton channels.
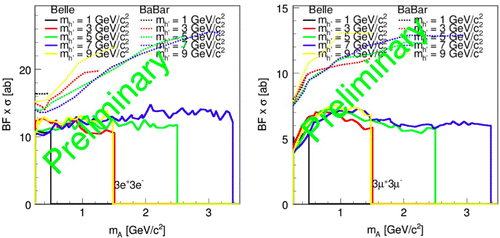
Such long-lived decays could result in many theories, and the particles could be produced by various mechanisms. BABAR Citation2015 has performed an inclusive search for “V” decays, , where
decays to
,
,
,
,
or
.
Efficiencies vary from 47% for 1 GeV and 3 cm to a few per mille at large mass and large
. Backgrounds are evaluated, systematic uncertainties folded in and the significance of any deviations calculated (avoiding known mass peaks, and low-mass threshold effects). There is no reportable evidence for a signal, and the results can be used to set 90% Bayesian limits on
efficiency, as shown in Figure .
Figure 12. BABAR limits on long-lived particle inclusive production, for different decay channels, and showing results from the and lower energies separately.
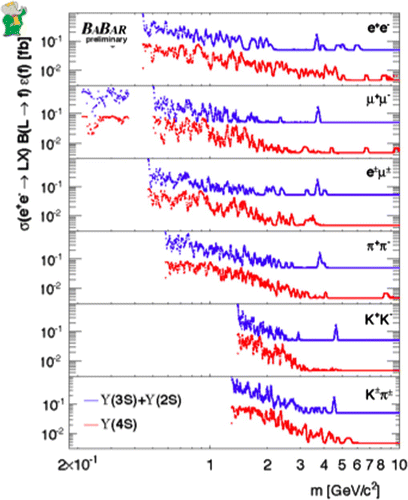
For any theory predicting such particles one can use these results, together with a table of experimental efficiencies, to put limits on the model parameters.
A second set of limits is obtained for events in which the remainder of the event contains a net strangeness, in a reaction which can be written as . Limits on the product of the branching ratios are of order
, depending on the mass and lifetime.
4. Outlook and conclusions
Both B factories have ceased taking data, but their enormous sample of high-quality data is still providing useful results, in areas of physics which were not considered when they were first proposed. The continuing thrust of data analysis by a new generation of postdocs and PhD students is a great success: despite the lack of “discoveries”, these measurements are playing a significant part in constraining BSM models. The experiments will welcome theorists with new ideas for objects to search for.
Although BABAR is in the past, Belle, which was shut down in 2010, will re-emerge with an upgraded detector, Belle2, and a greatly enhanced accelerator, SuperKEKB. The detector is scheduled to roll in this year, and take its first physics data in 2017. The design goal is to increase the statistics by a factor of 40—and the cynics should remember that B-factory design goals have regularly been met and exceeded. dark matter searches, including the Higgsstrahlung analysis, will continue as an important part of the physics programme.
Acknowledgements
The author would like to thank Abi Soffer (BaBar) and Gianluca Inguglia (Belle) for material.
Additional information
Funding
Notes on contributors
Roger Barlow
Roger Barlow is a particle physicist who worked on the TASSO and JADE electron–positron annihilation detectors at DESY, and then OPAL at CERN, before joining BaBar at SLAC, which produced the results on which this paper is based. He now works on LHCb. His main interests lie in the interpretation of data through statistics, and he has written a textbook and many papers on this topic. In recent years, his activities have broadened to include accelerator physics, and he led the project that successfully built EMMA, the first non-scaling fixed field alternating gradient accelerator, in 2011. He is now the director of the International Institute for Accelerator Applications at the University of Huddersfield, where he and his group work, among other projects, on the use of accelerators for proton therapy and thorium-fuelled ADSRs.
Notes
(i) Representing the BaBar Collaboration
References
- Aguilar, M., et al., (The AMS Collaboration). (2013). First result from the Alpha Magnetic Spectrometer on the International Space Station: Precision measurement of the positron fraction in primary cosmic rays of 0.5--350 GeV. Physical Review Letters, 110, 141102.
- Aubert, B., et al., (The BaBar Collaboration). (2009). Search for a narrow resonance in e+e- to four lepton final states( arXiv:0908.2821).
- Bevan, A., et al., (The BaBar Collaboration). (2014). Physics of the B factories. European Physical Journal C, 74, 3026.
- Fayet, P. (1980). Effects of the spin 1 partner of the goldstino (gravitino) on neutral current phenomenology. Physics Letters B, 95, 285–289.
- Jaegle, I. (2012). Search for the “Dark Photon” and the “Dark Higgs” at Belle. ( arXiv:1211.1403v2).
- Jaegle, I., et al., (The Belle Collaboration). (2015). Search for the dark photon and the dark Higgs boson at Belle. Physical Review Letters, 114, 211801. ( arXiv:1502.00084).
- Kobayashi, M., & Maskawa, T. (1973). CP-violation in the renormalizable theory of weak interaction. Progress of Theoretical Physics, 49, 652–657.
- Lees, J. P., et al., (The BaBar Collaboration). (2012). Search for low-mass dark-sector Higgs bosons. Physical Review Letters, 108, 211801.
- Lees, J. P., et al., (The BaBar Collaboration). (2014). Search for a dark photon in e+e-- collisions at BABAR. Physical Review Letters, 113, 201801.
- Lees, J. P., et al., (The BaBar Collaboration). (2015). Search for long-lived particles in e+e− collisions. Physical Review Letters, 114, 171801. ( arXiv:1502.02580v1).
- Lees, J. P., et al., (The BaBar Collaboration). (in press). Search for a light Higgs resonance in radiative decays of the Y(1S) with a charm tag. Physical Review D, 91, 071102(R). ( arXiv:1502.06019).