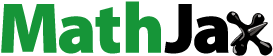
Abstract
Eu3+ doped MLa2O4 (M = Mg, Ca, Sr) nanophosphors were synthesized by a rapid facile gel combustion route. Luminescence properties of these prepared nanophosphors were analyzed by their excitation and emission spectra. The excitation spectrum consisted of some peaks in the 350–410 nm range due to the f–f transitions. The emission spectra of prepared nanophosphors had transitions of Eu3+ ions i.e. 5D0 → 7F0 (580 nm), 5D0 → 7F1 (594–596 nm), 5D0 → 7F2 (614–618, 628–629 nm), and 5D0 → 7F3 (650–651 nm). The main emission peak was observed at 614–618 nm of 5D0→7F2 transitions of Eu3+ ions. The enhancement in optical properties was observed when materials were reheated at higher temperatures. The nanostructural morphology was confirmed with scanning as well as transmission electron microscopy. The prepared materials were having size in the range of 10–50 nm. X-ray powder diffraction (XRD) technique was used to determine the crystal structure and phase of the prepared phosphor materials. XRD measurements revealed that the crystallinity of MLa2O4 materials increased with increasing the sintering temperature. The prepared materials had bright red emitting optical properties that could be suitably applied in various display devices.
Public Interest Statement
Europium-doped mixed oxide has received particular attention due to their efficient optical properties. These materials have bright red emission under the UV light source. Generally all the prime colors are important for the generation of the full color. This prepared series of compounds emit red luminescence (614–618 nm) that could be proficiently used for the generation of the white light for the various optoelectronic display applications.
1. Introduction
Technologically, rare earth-doped oxides nanophosphors are the strong motivators for the research due to their outstanding thermochemical and optoelectronic properties (Gouteron, Michel, Lejus, & Zarembowitch, Citation1981; Morais, Scalvi, Cavalheiro, Tabata, & Oliveira, Citation2008). The electronic structure of trivalent europium ion when used as an activator has a non-degenerate ground state 7F0 which is well separated from the first excited 5D0 multiplet (Venkatramu et al., Citation2010), provide unique properties to various host lattices (Garcı́a-Hipólito et al., Citation2003; Joffin, Dexpert-Ghys, Verelst, Baret, & Garcia, Citation2005; Quan, Wang, & Lin, Citation2005). The emission spectrum of Eu3+ ion is generally not affected by the influence of the surrounding due to the shielding effect of 5s, 5p electrons (Zhang, Lü, Xiu, & Wang, Citation2007). Hence, due to this excellent property of Eu3+ ion, it is used as an activator for the synthesis of red phosphor materials having outstanding properties (Cong et al., Citation2008; Davolos, Feliciano, & Pires, Citation2003; Dhanaraj, Geethalakshmi, & Jagannathan, Citation2004; Fragoso, de Mello Donegá, & Longo, Citation2003; Joly, Chen, Zhang, & Wang, Citation2007; Sun, Qi, Lee, & Lee, Citation2004). These Eu3+ ions-doped materials have been used as various display materials (Rambabu, Khanna, & Rao, Citation1998; Rambabu, Mathur, & Buddhudu, Citation1999; Ronda, Citation1997; Tanner & Wong, Citation2004). Thermoluminescence, electroluminescence, and cathodoluminescence properties of these materials are significantly applied in radiation detector, display material as field emission and electroluminescent display (FED, ELD) and cathode ray tube (CRT) (Oshio et al., Citation1999; Ravichandran, Roy, & White, Citation1997; Shea, McKittrick, & Lopez, Citation1996).
La2O3 is renowned as an outstanding host lattice for rare earth-doped oxide phosphors due to their good photoluminescence properties and moderately low cost. RE ions-activated metal lanthanates phosphors having general formula MLn2O4 (where M = Sr, Ba, and Ln = Y, La, Gd) have been proved outstanding phosphor materials (Yang, Xiao, Ding, Yang, & Wang, Citation2009; Zhou, Shi, & Gong, Citation2007). Different synthetic methods such as spray pyrolysis (Medina, Orozco, Hernandez, Hernandez, & Falcony, Citation2011), precipitation (Gunawidjaja, Diez-y-Riega, & Eilers, Citation2015), sol–gel Pechini method (Méndez et al., Citation2010), glycine nitrate solution combustion synthesis (Shang, Jiang, Shang, Li, & Zhao, Citation2011), etc. are used for the preparation of lanthanates-based phosphor materials. In our present work, we have synthesized and investigated the luminescence properties of these nanocrystalline MLa2O3:Eu3+ (M = Sr, Ca, Mg) phosphors with facile rapid combustion synthesis process. The prepared phosphors are having very fine particle size because of the released heat energy and gases of exothermic reaction of fuel and metal nitrates (Lou & Chen, Citation2008). The synthesized series of phosphors are characterized by PL, X-ray powder diffraction (XRD), SEM, and TEM analysis. The synthesized nanomaterials are having better optical properties than the La2O3:Eu3+ that could be effectively used in various display devices.
2. Experimental details
2.1. Syntheses of nanomaterials
High-purity chemicals [Eu(NO3)3.6H2O], [M(NO3)2].xH2O, (M = Sr, Ca, Mg), [La(NO3)3.6H2O] and hexamethylenetetramine [C6H12N4] were taken as raw materials. Nanophosphors having general formula MLa(2-z)O4:zEu3+ (where z = 1–5 mol%) were synthesized (Singh et al., Citation2015) by combusting a hydrated concentrated mixture having a considered stoichiometric amount of metal nitrates and hexamethylenetetramine as fuel. The amount of hexamethylenetetramine fuel was calculated by considering oxidizing and reducing valencies (Ekambaram & Patil, Citation1997). All these chemicals were mixed with deionized water and heated on hot plate till a semisolid paste was prepared. This gel mixture was then subjected to muffle furnace set already at 500°C. The material underwent rapid dehydration followed with degradation and production of numerous gases. These combustible gases ignited and burnt rapidly leaving a fluffy white colored fine powder. The solid powder obtained was reheated at 750 and 950°C for 1 h to study its effect on materials (Figure ). The equation for the synthesis of this series of nanophosphor is shown as:
Figure 1. A schematic presentation for the synthesis of Eu3+-activated MLa2O4 (M = Sr, Ca, Mg) nanophosphors.
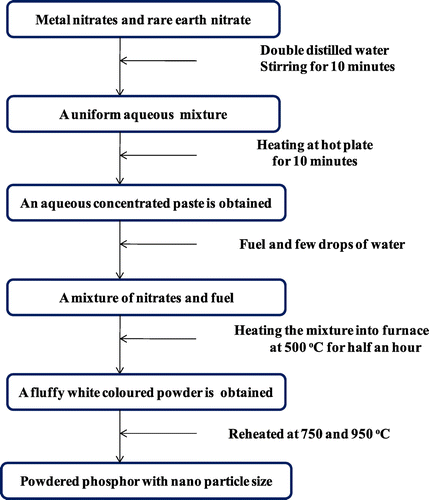
where M may be Sr/Ca/Mg, x is the number of moles of water in accordance to the metal nitrates and z is the number of moles of Europium nitrates (z varies from 1 to 5 mol%).
2.2. Instrumentation
Nanophosphors were characterized by X-ray diffraction profiles of the samples by the use of Rigaku Ultima IV X-ray diffractometer with Cu–Ka radiation for 2θ = 20–80°. Transmission electron micrographs (TEM) were taken using the Hitachi F-7500 transmission electron microscope. Scanning electron microscopy was carried out using JEOL JSM-6360LV scanning electron microscope. Emission and excitation spectra were measured with a Fluorimeter SPEX Fluorolog 1680 (USA) equipped with the SPEX 1934 D phosphorimeter having Xenon lamp as excitation source at room temperature in the UV–Visible region. All the characterizations were done at room temperature.
3. Results and discussion
3.1. Optical characterization
MLa2O4:Eu3+ (M = Mg, Ca, Sr) materials show different peaks (350–410 nm range) in their excitation spectra. These excitation lines are due to 7F0,1→5D4 (360–362 nm), 7F0,1→ 5L7 (377–380 nm), and 7F0,1→ 5L6 (394–396 nm) transitions (f–f) of Eu3+ ion. The 7F0,1 to 5L6 at 394–396 nm is observed as the strongest absorption for the synthesized materials. The emission spectra of MLa2O4:Eu3+ upon excitation with 395 nm show a series of emission lines in visible red region as shown in Figures . These emission lines are assigned to 5D0 →7FJ (J→0–3) transitions of the 4f6 configuration of Eu3+ ion present in their lattices. All the emission peaks are positioned in 560–660 nm range and the most intense emission band is observed at around 613–618 nm ascribed to the 5D0→7F2 transition of Eu3+ ion for prepared series of nanophosphors (MLa2O4:Eu3+). The orange emission is observed at 595 nm which appears due to the magnetic dipole 5D0→7F1 transition of europium ion and this transition rarely varies with the crystal field strength (Volanti et al., Citation2009). The emission at 614–616 and 628–629 nm is ascribed to the electric dipole transition of 5D0→7F2 transition of Eu3+, and this transition depends on the crystal field (Liu & Wang, Citation2007) of the prepared lattice. As excitation and emission overlay graphs (Figure ) are showing the maximum intensity of emission in Eu3+-doped SrLa2O4 lattice.
Figure 2. Photoluminescence spectra of SrLa2O4:Eu3+ excited at 395 nm. (a) Temperature variation (b) Concentration variation.
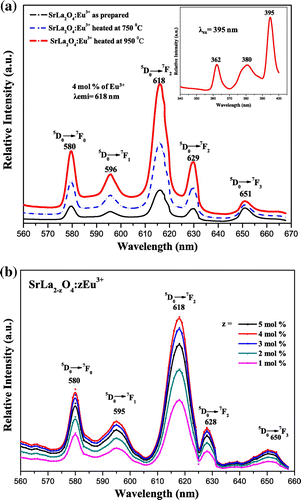
Figure 3. Photoluminescence spectra of CaLa2O4:Eu3+ excited at 395 nm. (a) Temperature variation (b) Concentration variation.
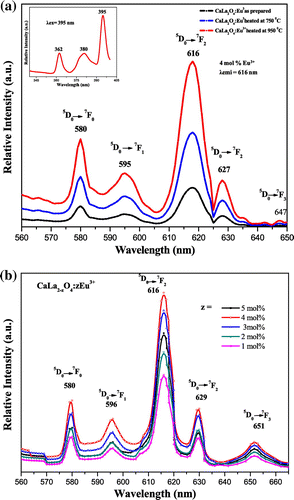
Figure 4. Photoluminescence spectra of MgLa2O4:Eu3+ excited at 395 nm. (a) Temperature variation (b) Concentration variation.
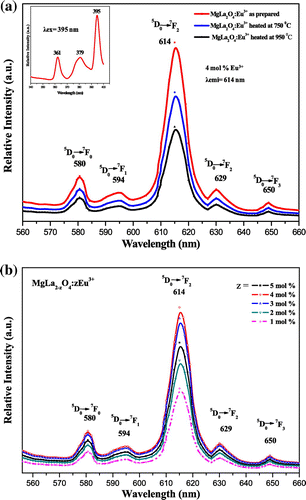
Figure 5. Photoluminescence spectra of La2O3:Eu3+ and MLa2O4:Eu3+ (M = Sr, Ca, Mg) nanophosphor showing variation of metal ions in host lattice.
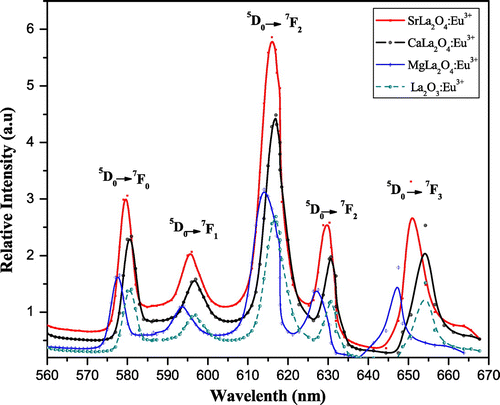
Luminescence properties of nanophosphors frequently depend on activator concentration and crystallinity of the material. Luminescence intensity varies with Eu3+ ions concentration in MLa2O4:Eu3+ samples (z = 1–5 mol%), synthesized at 500°C which are presented in Figures (b), (b), (b). It is analyzed that emission intensity in prepared host lattice increased with the increase of europium ion concentration and reached at maximum when z is doped up to 4 mol% in the host lattice. Further increase in activator concentration results in overdoping, which with further increase of non-radiative energy transfer between adjacent Eu3+ ions, resulting in lowering of their luminescence intensity (Blasse & Grabmaier, Citation1994). Figures (a), (a), (a) show the emission spectra of MLa2O4 doped with 4 mol% of europium ions, synthesized at 500°C, sintered at 750 and 950°C temperatures, upon excitation at 395 nm. The increase in the intensity of emission spectral lines is accredited to the increase of crystallinity of nanophosphors particles with the increase of sintering temperature.
The powders sintered at high temperatures show better red emission and this intensity of red color also increased with increase in dopant concentration. The best sample is observed when concentration of dopant is taken 4 mol% and calcined at 950°C. This is well described by color coordinates given in Table . The ratio R of the intensities I(5D0→7F2)/I(5D0→7F1) provides the percentage purity of the red luminescent color of phosphors. As confirmed by XRD, an additional phase La2O3 along with the main MLa2O4 phase is also present. These two phases in the lattice resulted into crystal field splitting of 5D0→7F2 into two lines at 613–618 nm and 628–629 nm. Figures reveal that the intensity of 5D0→7F2 transitions is more; as a result, R > 1 is obtained. So the red color is more prominent in MLa2O4:Eu3+ phosphors than the La2O3:Eu3+.
Table 1. Color co-ordinates of phosphors at varying concentrations and at varying calcination temperatures
CIE invented (λ),
(λ), and
(λ) [three color corresponding functions] subsequent to three primary colors i.e. blue, green, and red. By the use of these three functions, calorimetric coordinates (x, y) are calculated from the tristimulus value as shown in Equations (1) and (2).
(1)
(1)
(2)
(2)
The calculated values of color co-ordinates are shown in Table corresponding to red color of the visible region on the color gamut calculated from the emission spectra of MLa2O4:Eu3+ nanophosphor prepared at various annealing temperatures and at different concentrations. The color co-ordinates of MLa2O4:Eu3+ (0.04 mol) materials calcined at 950°C are shown in color triangle in Figure . Samples heated at 750 and 950°C show the CIE co-ordinates in the intense red color region of the color triangle.
3.2. Morphological study of the phosphor
The morphology of Eu3+-doped MLa2O4 nanoparticles was studied by scanning electron micrographs and transmission electron micrographs. The surface morphology of Eu3+ (4 mol%)-doped MLa2O4 nanoparticles prepared by present facile combustion method were studied using SEM. SEM photomicrographs of MLa2O4:Eu3+ samples are presented in Figures (a, b, c). The SEM micrographs showed that as-synthesized phosphors have spherical agglomerated nanocrystalline particles. As in combustion synthesis, numerous gasses evolved within very short period that generally produced nanosized materials with pores and voids. So it is analyzed from SEM images that the powders show large voids, cracks, and pores. The TEM Figures (a, b, c) are showing highly agglomerated nanoparticles with an average size of ~10–50 nm. TEM analysis has provided further additional microstructural details of these nanostructures. Furthermore, the crystallite size according to Scherrer’s equation is much closer to TEM observation.
3.3. X-ray diffraction study
The powder XRD patterns of the MLa2O4:Eu3+ (M = Sr, Ca, Mg) nanophosphors prepared by facile combustion synthesis at 500°C, calcined at 750 and 950°C, are shown in Figures . By using JCPDS data, multiphase components are observed at 500°C because of the occurrence of cubic La2O3 phase attributed as JCPDS No. 005-0602. In SrLa2O4:Eu3+, numerous supplementary peaks consequent to Sr(NO3)2 phase (JCPDS No. 004-0310) are also found. The XRD patterns of SrLa2O4:Eu3+ powder sintered at 750 and 950°C are assigned to SrLa2Ox phase matched with JCPDS card No. 042-0343. XRD pattern of sintered CaLa2O4 nearly matched with SrLa2O4 but no further records are found for CaLa2O4 and MgLa2O4. The as-prepared CaLa2O4 and MgLa2O4 samples also showed many additional peaks for M(NO3)2 and cubic La2O3 phase. But with the increase of calcination temperature, these additional peaks diminished. So it appears that 500°C was not an adequate temperature for the degradation of M(NO3)2 to prepare pure MLa2O4 phase. It is observed from XRD graphs that the intensities of peaks increased with increase of sintering temperature, which specify the improvement of crystallinity and also assign the increase in size of particles. With the increase of temperature of calcination, the intensity of XRD pattern gets increased and full with half the maximum of peaks slightly decreased. All this indicated the enhancement in crystalline nature of the prepared compounds. Eu3+ ions (0.95 A°) replaced La3+ (1.061 A°) ions in the host lattices instead of Mg2+ (0.72 A°), Ca2+ (0.99 A°) and Sr2+ (1.12 A°) ions. Here due to both size and ionic charge issues, it is difficult for Eu3+ to replace M2+ ions, though size of Ca2+ is nearly same to Eu3+, but here also charge compensation issue predominate. Because the differences in sizes of ionic radii of La3+ and Eu3+ is small and the charges of both ions are also same. Therefore, Eu3+ ions easily substitute La3+ ions.
The average crystallite sizes (D) are calculated using Scherrer’s formula from the line broadening obtained from the X-ray powders.
where, “K” is constant, “λ” is wavelength of X-rays, and “β” is FWHM.
Tables provided the detailed description related to size of particles and phase of prepared phosphors.
Table 2. Detailed description of size and phase of particle of SrLa2O4:Eu3+
Table 3. Detailed description of size and phase of particle of CaLa2O4:Eu3+
Table 4. Detailed description of size and phase of particle of MgLa2O4:Eu3+
4. Conclusions
Structurally and morphologically well-defined nanophosphor compounds were efficiently prepared by present facile rapid gel combustion process. XRD patterns showed the presence of multiphase components at 500°C, but on increasing sintering temperature, crystallinity of phosphors increased and single phase of phosphors was obtained at 750 and 950°C. Crystallite size of MLa2O4:Eu3+ phosphor particles were found below 50 nm as calculated from XRD using Scherrer equation. On increasing sintering temperature, sizes of particles were increased, which showed enhancement in the crystallinity of phosphors. Moreover, SEM and TEM characterization confirmed nanocrystalline size of prepared particles. Photoluminescence spectra obtained for all MLa2O4:Eu3+ phosphors had dominant red emission peak at 613–618 nm. The photoluminescence intensity of all the prepared nanomaterials was found to be increased with the increasing of the sintering temperature. Intensity of luminescence of SrLa2O4:Eu3+ nanophosphor was found maximum when sintered at 950°C. All prepared nanomaterials had tremendous red photoluminescence properties so they could be made functional in different optoelectronic display applications.
Additional information
Funding
Notes on contributors
Devender Singh
Devender Singh is presently working as an assistant professor (Stage-III) of Chemistry at Maharishi Dayanand University, Rohtak, Haryana. He has completed his MSc (2001) and PhD (2005) in Inorganic Chemistry under the supervision of Prof. Ishwar Singh and Prof. Sang Do Han (KIER, S. Korea) from the Maharshi Dayanand University, Rohtak, India. His current research interest focuses on light-emitting materials and their fabrication for optoelectronic display applications. He has published more than 50 research papers in well-reputed international journals. Presently he is a principal investigator for the Major Research Project funded by the University Grant Commission, New Delhi.
References
- Blasse, G., & Grabmaier, B. C. (1994). Luminescent materials. Berlin: Springer.10.1007/978-3-642-79017-1
- Cong, Y., Li, B., Lei, B., Wang, X., Liu, C., Liu, J., & Li, W. (2008). Enhancement of luminescence intensity and increase of emission lifetime in Eu3+-doped 3CdO–Al2O3–3SiO2 amorphous system. Journal of Luminescence, 128, 105–109. doi:10.1016/j.jlumin.2007.05.016
- Davolos, M. R., Feliciano, S., & Pires, A. M. (2003). Solvothermal method to obtain europium-doped yttrium oxide. Journal of Solid State Chemistry, 171, 268–272. doi:10.1016/S0022-4596(02)00174-3
- Dhanaraj, J., Geethalakshmi, M., & Jagannathan, R. (2004). Eu3+ doped yttrium oxysulfide nanocrystals—Crystallite size and luminescence transition(s). Chemical Physics Letters, 387, 23–28. doi:10.1016/j.cplett.2004.01.079
- Ekambaram, S., & Patil, K. C. (1997). Synthesis and properties of Eu2+ activated blue phosphors. Journal of Alloys and Compounds, 248, 7–12. doi:10.1016/S0925-8388(96)02622-9
- Fragoso, W. D., de Mello Donegá, C., & Longo, R. L. (2003). Luminescence and energy transfer in La2O3–Nb2O5–B2O3:M3+ (M=Bi, Eu, Dy) glasses. Journal of Luminescence, 105, 97–103. doi:10.1016/S0022-2313(03)00113-3
- Garcı́a-Hipólito, M., Hernández-Pérez, C. D., Alvarez-Fregoso, O., Martı́nez, E., Guzmán-Mendoza, J., & Falcony, C. (2003). Characterization of europium doped zinc aluminate luminescent coatings synthesized by ultrasonic spray pyrolysis process. Optical Materials, 22, 345–351. doi:10.1016/S0925-3467(02)00346-4
- Gouteron, J., Michel, D., Lejus, A. M., & Zarembowitch, J. (1981). Raman spectra of lanthanide sesquioxide single crystals: Correlation between A and B-type structures. Journal of Solid State Chemistry, 38, 288–296. doi:10.1016/0022-4596(81)90058-X
- Gunawidjaja, R., Diez-y-Riega, H., & Eilers, H. (2015). Synthesis and characterizations of spherical Eu:La2O3 and related core/shell nanoparticles. Powder Technology, 271, 255–261. doi:10.1016/j.powtec.2014.11.021
- Joffin, N., Dexpert-Ghys, J., Verelst, M., Baret, G., & Garcia, A. (2005). The influence of microstructure on luminescent properties of Y2O3: Eu prepared by spray pyrolysis. Journal of Luminescence, 113, 249–257. doi:10.1016/j.jlumin.2004.10.021
- Joly, A. G., Chen, W., Zhang, J., & Wang, S. (2007). Electronic energy relaxation and luminescence decay dynamics of Eu3+ in Zn2SiO4:Eu3+ phosphors. Journal of Luminescence, 126, 491–496. doi:10.1016/j.jlumin.2006.09.004
- Liu, X., & Wang, X. (2007). Preparation and luminescence properties of BaZrO3: Eu phosphor powders. Optical Materials, 30, 626–629. doi:10.1016/j.optmat.2007.02.032
- Lou, X., & Chen, D. (2008). Synthesis of CaWO4:Eu3+ phosphor powders via a combustion process and its optical properties. Materials Letters, 62, 1681–1684. doi:10.1016/j.matlet.2007.09.066
- Medina, D. Y., Orozco, S., Hernandez, I., Hernandez, R. T., & Falcony, C. (2011). Characterization of europium doped lanthanum oxide films prepared by spray pyrolysis. Journal of Non-Crystalline Solids, 357, 3740–3743. doi:10.1016/j.jnoncrysol.2011.07.021
- Méndez, M., Carvajal, J. J., Cesteros, Y., Aguiló, M., Díaz, F., Giguère, A., … Marsal, L. F. (2010). Sol–gel pechini synthesis and optical spectroscopy of nanocrystalline La2O3 doped with Eu3+. Optical Materials, 32, 1686–1692. doi:10.1016/j.optmat.2010.02.018
- Morais, E. A. D., Scalvi, L. V. A., Cavalheiro, A. A., Tabata, A., & Oliveira, J. B. B. (2008). Rare earth centers properties and electron trapping in SnO2 thin films produced by sol–gel route. Journal of Non Crystalline Solids, 354, 4840–4845. doi:10.1016/j.jnoncrysol.2008.04.029
- Oshio, S., Kitamura, K., Shigeta, T., Horii, S., Matsuoka, T., Tanaka, S., & Kanda, T. (1999). Firing technique for preparing a BaMgAl10O17:Eu2+ phosphor with controlled particle shape and size. Journal of the Electrochemical Society, 146, 392–399. doi:10.1149/1.1391620
- Quan, Z. W., Wang, L. S., & Lin, J. (2005). Synthesis and characterization of spherical ZrO2:Eu3+ phosphors by spray pyrolysis process. Materials Research Bulletin, 40, 810–820. doi:10.1016/j.materresbull.2005.02.006
- Rambabu, U., Khanna, P. K., & Rao, I. C. (1998). Fluorescence spectra of Eu3+-doped lanthanide oxybromide-based powder phosphors. Materials Letters, 34, 269–274. doi:10.1016/S0167-577X(97)00183-3
- Rambabu, U., Mathur, A., & Buddhudu, S. (1999). Fluorescence spectra of Eu3+ and Tb3+-doped lanthanide oxychloride powder phosphors. Materials Chemistry and Physics, 61, 156–162. doi:10.1016/S0254-0584(99)00122-4
- Ravichandran, D., Roy, R., & White, W. B. (1997). Synthesis and characterization of so–gel derived hexa-aluminate phosphors. Journal of Materials Research, 12, 819–824. doi:10.1557/JMR.1997.0119
- Ronda, C. R. (1997). Recent achievements in research on phosphors for lamps and displays. Journal of Luminescence, 72–74, 49–54. doi:10.1016/S0022-2313(96)00374-2
- Shang, C., Jiang, H., Shang, X., Li, M., & Zhao, L. (2011). Investigation on the luminescence improvement of nanosized La2O3/Eu3+ phosphor under charge-transfer excitation. The Journal of Physical Chemistry C, 115, 2630–2635. doi:10.1021/jp108608f
- Shea, L. E., McKittrick, J., & Lopez, O. A. (1996). Synthesis of red-emitting, small particle size luminescent oxides using an optimized combustion process. Journal of the American Ceramic Society, 79, 3257–3265. doi:10.1111/j.1151-2916.1996.tb08103.x
- Singh D., Tanwar V., Bhagwan S., Nishal V., Sheoran S., Kadyan S., … Kadyan P. S. (2015). Synthesis and optical characterization of europium doped MY2O4 (M = Mg, Ca, and Sr) nanophosphors for solid state lightening applications. Indian Journal of Materials Science, 2015, 845065, 8. doi:10.1155/2015/845065
- Sun, Y., Qi, L., Lee, M., & Lee, B. I. (2004). Photoluminescent properties of Y2O3:Eu3+ phosphors prepared via urea precipitation in non-aqueous solution. Journal of Luminescence, 109, 85–91. doi:10.1016/j.jlumin.2004.01.085
- Tanner, P. A., & Wong, K. L. (2004). Synthesis and spectroscopy of lanthanide ion-doped Y2O3. The Journal of Physical Chemistry B, 108, 136–142. doi:10.1021/jp035583o
- Venkatramu, V., Giarola, M., Mariotto, G., Enzo, S., Polizzi, S., Jayasankar, C. K., … Speghini, A. (2010). Nanocrystalline lanthanide-doped Lu3Ga5O12 garnets: Interesting materials for light-emitting devices. Nanotechnology, 21, 175703–175714. doi:10.1088/0957-4484/21/17/175703
- Volanti, D. P., Rosa, I. L. V., Paris, E. C., Paskocimas, C. A., Pizani, P. S., Varela, J. A., & Longo, E. (2009). The role of the Eu3+ ions in structure and photoluminescence properties of SrBi2Nb2O9 powders. Optical Materials, 31, 995–999. doi:10.1016/j.optmat.2008.11.006
- Yang, J., Xiao, S., Ding, J., Yang, X., & Wang, X. (2009). Preparation and photoluminescence properties of SrY2O4:Yb3+, Er3+ powders. Journal of Alloys and Compounds, 474, 424–427. doi:10.1016/j.jallcom.2008.06.158
- Zhang, H., Lü, M., Xiu, Z., & Wang, S. (2007). Synthesis and photoluminescence properties of a new red emitting phosphor: Ca3(VO4)2:Eu3+; Mn2+. Journal of Materials Research Bulletin, 42, 1145–1152. doi:10.1016/j.materresbull.2006.09.002
- Zhou, L., Shi, J., & Gong, M. (2007). Synthesis and luminescent properties of phosphor. Journal of Physics and Chemistry of Solids, 68, 1471–1475. doi:10.1016/j.jpcs.2007.03.014