Abstract
This research shows that small animals, tardigrades (Milnesium tardigradum) in tun (dehydrated) state and Artemia salina cists (dried eggs) can tolerate the very high hydrostatic pressure of 7.5 GPa. It was really surprising that living organisms can survive after exposure to such a high pressure. We extended these studies to the extremely high pressure of 20 GPa by using a Kawai-type octahedral anvil press. After exposure to this pressure for 30 min, the tardigrades were soaked in pure water and investigated under a microscope. Their bodies regained metabolic state and no serious injury could be seen. But they were not alive. A few of Artemia eggs went part of the way to hatching after soaked in sea water, but they never grew any further. Comparing with the case of blue-green alga, these animals are weaker under ultra-high pressure.
Public Interest Statement
We found that small animals, water bares in dehydrated state and plankton eggs and plant seeds of white clover and moss spores can tolerate the very high pressure of 775,000 atmosphere for at least 1 h. We extended these studies to the extremely high pressure of 200,000 atmosphere. In the bodies of water bares, no serious injury could be seen. But they were not alive. A few of plankton Artemia eggs went part of the way to hatching after being soaked in sea water, but they never grew any further. But, it was really surprising that living organisms can survive or nearly survive after exposure to such ultra-high pressures. This pressure corresponds to that of the depth of 550–600 km below the earth’s surface. The results may give the possibility that a small creature can travel through the space in a large meteorite.
1. Introduction
A terrestrial tardigrade, Milnesium tardigradum, belongs to Tardigrada which appeared on earth in Cambrian period, is a small animal about 0.6 mm in length. This animal usually lives among mosses. When it rains, it becomes metabolically active, while when dried, it turns into a dehydrated state called “tun.” A tardigrade in the cryptobiotic (dehydrated) state is known to show strong tolerance to extreme environmental conditions (Beltrán-Pardo et al., Citation2013; Guidetti, Altiero, & Rebecchi, Citation2011; Welnicz, Grohme, Kaczmarek, Schill, & Frohme, Citation2011), such as exposure to low temperature, high radioactive radiation, to outer-space vacuum for 10 days (Jönsson, Rabbow, Schill, Harms-Ringdahl, & Rettberg, Citation2008) and to high hydrostatic pressure of 0.6 GPa (Seki & Toyoshima, Citation1998). The present author’s group extended the study of the pressure tolerance of this animal to a very high pressure of order several GPa (Hirokawa et al., Citation2009; Ono et al., Citation2008). They found all of the 20 tuns of tardigrades set in a high-pressure cell alive after exposure to 7.5 GPa for 3 h. They also found that tardigrades can tolerate this pressure for more than 12 h.
Artemia salina belonging to Crustacea in Arthropoda, is a kind of plankton and is commonly known as brine shrimp or sea monkey. This animal lives in inland saltwater lakes and the species has been conserved without any significant evolution since the Triassic period. Artemia cists (dried eggs in cryptobiotic state) are metabolically inactive and even in the case when the lakes are dried up, they remain alive for many years. Artemia cists are also known to show very strong tolerance to severe environmental conditions. We have also extended high-pressure study on Artemia eggs and found that they can survive exposure to 7.5 GPa for up to 72 h (Minami et al., Citation2010; Ono, Minami, et al., Citation2010). This pressure corresponds to that existing in the middle of the upper mantle, at the depth of 180 km from the surface of the earth.
These findings are quite in contrast to the well-known fact that proteins began to unfold around 0.3 GPa, where most bacteria and many other organisms die (Silva, Luan, Glaser, Voss, & Weber, Citation1992; Suzuki & Taniguchi, Citation1972; Weber & Drickamer, Citation1983). To obtain any hint to make this point clear, we extended high-pressure study to various living samples including plants (Mori, Yokota, & Ono, Citation2012; Nishihira et al., Citation2010, Citation2012; Ono, Mori, et al., Citation2010; Ono et al., Citation2012) and yeast (Shibata et al., Citation2014). All of these creatures investigated so far were alive after exposure to 7.5 GPa for at least 1 h. However, the question mentioned above is yet to be solved.
In the present investigation, the maximum pressure has been extended to an ultra-high pressure range, as high as 20 GPa, which corresponds to the pressure just above the lower mantle of the earth. It will be interesting to see whether tardigrades and Artemia can withstand such ultra-high pressures.
2. Experiment
The experimental procedure adopted here is the same as described in the reference (Ono, Citation2015; Ono et al., Citation2013, Citation2015). Tardigrades (M. tardigradum) used as living samples in the present study were collected from a field in the city of Okayama, Japan. At one time, 5–10 tardigrades were dehydrated into tun state. Artemia cysts samples were purchased from INVE Aquaculture, Ogden UT, USA. The tuns of tardigrades and a few dozens of Artemia eggs were sealed in a small Teflon capsule with a liquid pressure medium fluorinert (PC77, Sumitomo 3M). The inner diameter and the height of the capsules were 1.6 and 1.8 mm, respectively. An illustration of sample preparation and the configuration of the living samples in a Teflon capsule is shown in Figure . This capsule was put in the centre of an octahedron made of MgO with an edge length of 8.0 mm. To generate a pressure up to 20 GPa a Kawai-type two-stage octahedral anvil press installed at the Institute for Study of the Earth’s Interior (ISEI) of Okayama University was adopted. The pressure was increased to 20 GPa at the rate of 40 GPa/h, then kept for 30 min at the maximum pressure, and decreased down to ambient pressure at the rate of 20 GPa/h. Figure (a) and (b) show the MgO octahedron with Teflon capsule before and after exposure to the ultra-high pressure of 20 GPa for 30 min, respectively. It is seen in this figure that the dimension of the octahedron became about a half of its original size after the compression.
Figure 1. An illustration of the sample preparation.
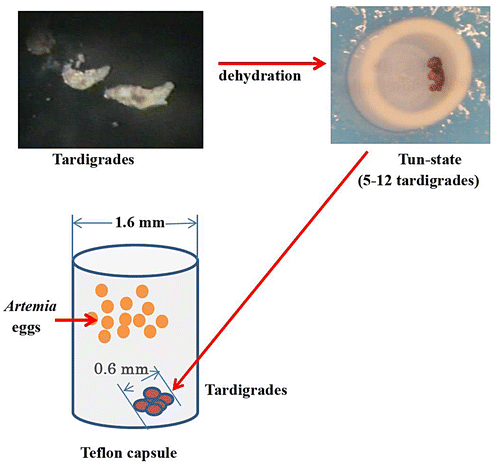
Figure 2. (a). The MgO octahedron with the Teflon capsule before compression. A few dozens of Artemia eggs are seen in the Teflon capsule which is being inserted in the centre hole of the octahedron and (b). after compression to 20 GPa for 30 min.
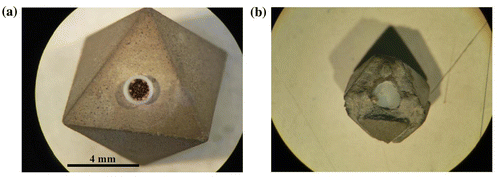
After the pressure was released, the Teflon capsule was brought out from the MgO high pressure cell, and the animal samples at the metabolic state were investigated under an optical microscope.
3. Results
3.1. Tardigrades after exposure to 20 GPa
The tuns of tardigrades after exposure to 20 GPa for 30 min were brought out of the Teflon capsule, and soaked in pure water. Tardigrades exposed to 20 GPa for 30 min (first run) are shown in Figure (a). The photo was taken 4 h after they were soaked in pure water. The one shown in the expanded scale in (b) looks almost alive. But, all of them were dead. No movement of their body and legs had been observed during the investigation till 3 days after they were soaked in water. Figure (a) shows five tardigrades after exposure to 20 GPa for 30 min (second run). Two of them are shown in an expanded scale in (b) and (c). These photos were taken 16 h after they were soaked in water. No serious injuries were seen in these bodies, but they all were not alive.
Figure 3. (a). Tardigrades after exposure to 20 GPa for 30 min (first run). The one on the right-hand side is shown in (b). in an expanded scale, where no serious injuries were seen.
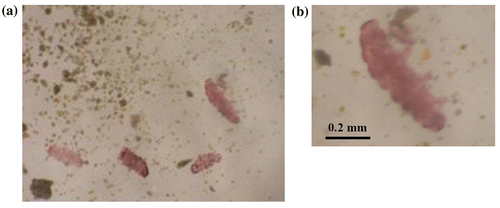
Figure 4. (a). Tardigrades exposed to 20 GPa for 30 min (second run). Two of them are shown in an expanded scale in (b). and (c). None of these five tardigrades could survive. However, no serious injuries could be seen in these two tardigrades.
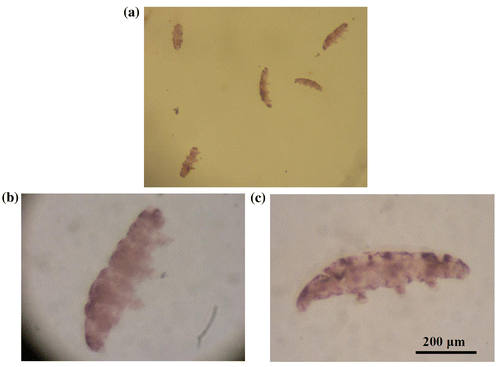
3.2. A. salina after exposure to ultra-high pressure
Artemia eggs exposed to 20 GPa for 30 min were also brought out of the Teflon capsule and soaked in sea water. The effect of high pressure on Artemia was investigated under a microscope by comparing the image of the control group which has not been exposed to high pressure. A few of Artemia eggs went part of the way to hatching after they were soaked in sea water. One of them is shown in Figure (a). This photo was taken 14 h after being soaked in sea water. For comparison, a hatching egg of Artemia is shown in Figure (b) as a control, which is not exposed to high pressure. In Figure (c) is shown the same Artemia egg that is shown in (a) 39 h after being soaked in sea water. At this moment, the control Nauplius (hatched larva) was already moving around as seen in (d). All of these Artemia eggs that went part of the way to hatching observed at the time 14 h after being soaked in sea water never grew any further. Other Artemia eggs exposed to 20 GPa did not go hatching. Some of them are shown in Figure . Thus, all the Artemia eggs that were exposed to 20 GPa could not tolerate this high pressure. Comparing with the case of blue-green alga (Ono et al., Citation2015), Artemia is weaker against ultra-high pressure.
Figure 5. (a). An Artemia cyst exposed to 20 GPa for 30 min and soaked in sea water. It seems that this cyst went part of the way to hatching, (b). The control which was not exposed to high pressure. (c). The Artemia egg shown in (a) 39 h after being soaked in sea water, and (d). a control Nauplius (hatched larva) shown for comparison.
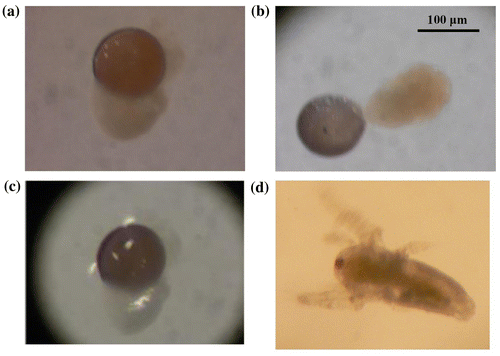
4. Discussion
It was shown by the present author’s group that all the living samples, both animals and plants that have been tested, could tolerate the high pressure of 7.5 GPa for at least 1 h. The animals tested were tardigrades in tun state and Artemia cysts, and the plants were spores of mosses, seeds of white clover Trifolium Lepens L. These results are incomprehensible, considering the fact that denaturation of proteins usually takes place below 1 GPa. To obtain any hints to this question, we have extended our experiment to the ultra-high pressure of 20 GPa.
In the case of tardigrades, we could not see any serious injuries in their bodies after exposure to 20 GPa for 30 min, but they all were not alive. This fact means that the cause of death is not a macroscopic injury of the bodies, but some other reasons such as choking, i.e., lack of Oxygen for breathing, braking some special molecules or DNAs. Under this ultra-high pressure, even in a solid material such as P2O5 the average atomic volume decreases about 40% (Brazhkin et al., Citation2015). The volume of a moss spore was estimated to decrease by about 60% on increasing pressure from ambient to 20 GPa (Ono, Citation2015). Under the ultra-high pressure of 20 GPa, the molecules and atoms that comprise the body are fixed too tightly to let the organisms breathe. Any microscopic injuries due to the existence of pressure gradient may also cause death.
On the other hand, in the case of Artemia, a few of high-pressure exposed eggs went part of the way to hatching. However, all of these eggs never grew any further. This fact implies that these eggs might be alive after exposure to the ultra-high pressure, but the cells which contribute to cell division might have serious injuries. This situation of the Artemia eggs which went part of the way to hatching is similar to the case of the moss Venturiella. As seen in the Figure (a) in the reference (Ono et al., Citation2013), a spore protonema of Venturiella after exposure to 20 GPa for 30 min went part of the way to full germination. But, this protonema did not grow any further. In this case, it is also considered that the cells which contribute to cell division might have serious injuries. It is well known that cells that contribute to cell division are weaker than normal cells under severe environmental conditions such as low temperature, high radioactive radiation, high pressure.
The high pressure tolerance of animals and plants investigated so far is illustrated in Figure . In this figure the data obtained by Koyama (Citation2010) for the cells of normal eel and deep sea eel at the metabolic state are also shown for comparison.
Figure 7. Pressure tolerance of animals and plants; tardigrade tuns for 12-h exposure[5], Artemia cists for 72 h (Minami et al., Citation2010; Ono, Minami, et al., Citation2010), white clover seeds for 2 h (Nishihira et al., Citation2012), moss Venturiella spores for 144 h (Ono, Mori, et al., Citation2010) and blue-green algae for 30 min (Ono et al., Citation2015).
![Figure 7. Pressure tolerance of animals and plants; tardigrade tuns for 12-h exposure[5], Artemia cists for 72 h (Minami et al., Citation2010; Ono, Minami, et al., Citation2010), white clover seeds for 2 h (Nishihira et al., Citation2012), moss Venturiella spores for 144 h (Ono, Mori, et al., Citation2010) and blue-green algae for 30 min (Ono et al., Citation2015).](/cms/asset/d271163f-f08d-4c4f-b1f4-67b6d8b448d7/oaph_a_1167575_f0007_oc.gif)
It is worth emphasizing that the sizes of Artemia eggs (100 μm in diameter), tardigrade tun (150 μm) and moss spores (30 μm) are in the range of the order 20–200 μm. On the other hand, the size of blue-green alga is as small as 2 μm. We reported that blue-green algae, Microcystis flos-aquae (Wittl.) Kirchn. tolerate the ultra-high pressure of 20 GPa for 30 min (Ono et al., Citation2015). The stronger tolerance of them than tardigrades, Artemia and moss spores seems due to their smaller size. This fact implies that pressure gradient also plays an important role in the high-pressure tolerance of living creatures.
5. Conclusion
It was shown from the present study that tardigrade tuns and Artemia cysts could not survive after exposure to the ultra-high pressure of 20 GPa for 30 min. However, no serious injuries could be seen in the bodies of tardigrades brought back to metabolic state. This fact means that the cause of death of tardigrades is not due to a macroscopic injury of the bodies. Possible cause of death could be lack of Oxygen for breathing, breaking some special molecules or DNAs or some other microscopic injuries.
In the case of Artemia, a few eggs went part of the way to hatching. However, all of these eggs never grew any further. This fact implies that these eggs might be alive after exposure to the ultra-high pressure, but the cells which contribute to cell division might have serious injuries. Comparing with the case of much smaller sized sample, blue-green alga, these animals are weaker under ultra-high pressure.
Acknowledgments
Fumihisa Ono wishes his sincere thanks to Dr. Masaya Sougawa for his kind help in generating high pressure. This paper presents a result of a joint research programme carried out at the Institute for Study of the Earth’s Interior, Okayama University.
Additional information
Funding
Notes on contributors
Fumihisa Ono
Fumihisa Ono has been engaged in research study on Physical properties of materials under high pressure. One day, he thought of putting small animals in the high-pressure cell which he has been using, and consulted a biologist. The first candidate was tardigrades in tun state. It was surprising that all of the 20 tardigrades could tolerate the very high pressure of 7.5 GPa for at least 6 h. The study has been extended to other plants and animals by joining Physics and Biology groups. All the specimens investigated so far were found alive after exposure to the high pressure for at least 1 h. Then a big question arose what mechanism made the living creatures so strong against high pressure. To solve this question discussion has been made among Okayama, Rome and Montpellier groups. The pressure has been extended to 20 GPa by joining Misasa group.
References
- Beltrán-Pardo, E., Jönsson, K. I., Wojcik, A., Haghdoost, S., Harms-Ringdahl, M., Bermúdez-Cruz, R. M., & Bernal Villegas, J. E. (2013). Effects of ionizing radiation on embryos of the tardigrade milnesium cf. tardigradum at different stages of development, PLoS ONE 8, p. e72098. doi:10.1371/journal.pone.0072098
- Brazhkin, V. V., Gromnitskaya, E. L., Danilov, I. V., Katayama, Y., Lyapin, A. G., & Popova, S. V. (2015). High pressure behavior of P 2 O 5 crystalline modifications: Compressibility, elastic properties and phase transitions. Materials Research Express, 2, 025201.10.1088/2053-1591/2/2/025201
- Guidetti, R., Altiero, T., & Rebecchi, L. (2011). On dormancy strategies in tardigrades. Journal of Insect Physiology, 57, 567–576.10.1016/j.jinsphys.2011.03.003
- Hirokawa, D. D., Iwata, K., Kawai, K., Koseki, S., Okuda, T., & Yamamoto, K. (2009). High hydrostatic pressure tolerance of four different anhydrobiotic animal species. Zoological Science, 26, 238–242.
- Jönsson, K. I., Rabbow, E., Schill, R. O., Harms-Ringdahl, M., & Rettberg, P. (2008). Tardigrades survive exposure to space in low Earth orbit. Current Biology, 18, R729–R731.10.1016/j.cub.2008.06.048
- Koyama, S. (2010). Molecular and cellular biological studies for deep-sea multicellular organisms under atmospheric pressure condition. Journal of Japanese Society for Extremophiles, 9, 106–115.10.3118/jjse.9.106
- Minami, K., Ono, F., Mori, Y., Takarabe, K., Saigusa, M., Matsushima, Y., ... Yamashita, M. (2010). Strong environmental tolerance of Artemia under very high pressure. Journal of Physics: Conference Series, 215, 012164, 4 p.10.1088/1742-6596/215/1/012164
- Mori, Y., Yokota, S., & Ono, F. (2012). Germination of vegetable seeds exposed to very high pressure. Journal of Physics: Conference Series, 377, 012055, 4 p.10.1088/1742-6596/377/1/012055
- Nishihira, N., Iwasaki, T., Shinpou, R., Hara, A., Ono, F., Hada, Y., ... Yamashita, M. (2012). Maintaining viability of white clover under very high pressure. Journal of Applied Physics, 111, 112619, 4.10.1063/1.4726241
- Nishihira, N., Shindo, A., Saigusa, M., Ono, F., Matsushima, Y., Mori, Y., ... Yamashita, M. (2010). Preserving life of moss Ptychomitrium under very high pressure. Journal of Physics and Chemistry of Solids, 71, 1123–1126.10.1016/j.jpcs.2010.03.018
- Ono, F. (2015). Moss spores tolerate ultra-high pressure. In K. Akasaka & H. Matsuki (Eds.), High pressure bioscience (Chapter 22, pp. 443–466). Dordrecht: Springer.
- Ono, F., Minami, K., Saigusa, M., Matsushima, Y., Mori, Y., Takarabe, K., ... Yamashita, M. (2010). Life of Artemia under very high pressure. Journal of Physics and Chemistry of Solids, 71, 1127–1130.10.1016/j.jpcs.2010.03.019
- Ono, F., Mori, Y., Sougawa, M., Takarabe, K., Hada, Y., Nishihira, N., ... Saini, N. L. (2012). Effect of very high pressure on life of plants and animals. Journal of Physics: Conference Series, 377, 012053, 6.10.1088/1742-6596/377/1/012053
- Ono, F., Mori, Y., Takarabe, K., Nishihira, N., Shindo, A., Saigusa, M., ... Yamashita, M. (2010). Strong environmental tolerance of moss Venturiella under very high pressure. Journal of Physics: Conference Series, 215, 012165, 4.10.1088/1742-6596/215/1/012165
- Ono, F., Nishihira, N., Hada, Y., Mori, Y., Takarabe, K., Saigusa, M., ... Ito, E. (2015). Strong tolerance of blue-green alga Microcystis flos-aquae to very high pressure. Journal of Physics and Chemistry of Solids, 84, 57–62.10.1016/j.jpcs.2014.08.008
- Ono, F., Nishihira, N., Sougawa, M., Hada, Y., Mori, Y., Takarabe, K., ... Saini, N. L. (2013). Distortion of spores of moss Venturiella under ultra high pressure. High Presuure Research, 33, 362–368.
- Ono, F., Saigusa, M., Uozumi, T., Matsushima, Y., Ikeda, H., Saini, N. L., & Yamashita, M. (2008). Effect of high hydrostatic pressure on to life of the tiny animal tardigrade. Journal of Physics and Chemistry of Solids, 69, 2297–2300.10.1016/j.jpcs.2008.04.019
- Seki, K., & Toyoshima, M. (1998). Preserving tardigrades under pressure. Nature, 395, 853–854.10.1038/27576
- Shibata, M., Torigoe, M., Matsumoto, Y., Yamamoto, M., Takizawa, N., Hada, Y., ... Ono, F. (2014). Tolerance of budding yeast Saccharomyces cerevisiae to ultra high pressure. Journal of Physics: Conference Series, 500, 102004, 5.10.1088/1742-6596/500/10/102004
- Silva, J. L., Luan, P., Glaser, M., Voss, E. W., & Weber, G. (1992). Effects of hydrostatic pressure on a membrane-enveloped virus; high immunogenicity of the pressure-inactivated virus. Journal of virology, 66, 2111–2117.
- Suzuki, K., & Taniguchi, Y. (1972). Effect of pressure on biopolymers and model systems. Symposia of the Society for Experimental Biology, 26, 103–124.
- Weber, G., & Drickamer, H. G. (1983). The effect of high pressure upon proteins and other biomolecules. Quarterly Reviews of Biophysics, 16, 89–112.10.1017/S0033583500004935
- Welnicz, W., Grohme, M. A., Kaczmarek, L., Schill, R. O., & Frohme, M. (2011). Anhydrobiosis in tardigrades-The last decade. Journal of Insect Physiology, 57, 577–583.