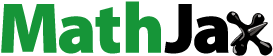
Abstract
Nickel-doped barium nanohexaferrites with the chemical formula BaNixFe(12-x)O19 are prepared using the chemical co-precipitation technique. The calcination of the samples is done at 800°C for 4 h. In order to carry out the structural analysis that includes the crystallographic and the morphological investigations, X-ray diffraction and TEM are carried out to govern the phase, structure and the size of the crystal. By the use of Debye–Scherrer equation, the size of the crystallite is calculated to be in the range of 38–100 nm. TEM results reveal the magnetoplumbite structure of the hexaferrites. The M–H curve obtained using the Vibrating Sample Magnetometer computes the magnetic parameters like saturation magnetization, coercivity, remanence and verifies the behaviour of hexaferrites as hard magnetic materials. Further, the effect of variation in calcination temperature is also investigated on the values of magnetic parameters of hexaferrites. In order to carry out the investigations on the electrical properties of hexaferrites, the two-probe method and parallel plate capacitor set-up are used. The values of relative permittivity, dielectric loss and loss tangent are computed as a function of frequency. The values of DC electrical resistance for pure and nickel-doped barium nanohexaferrites are evaluated from the slope of I–V graphs.
Public Interest Statement
The main aim of the work is to study the effect of reducing the size of the barium hexaferrite to nanoscale on its structural, magnetic and electrical properties. Barium nanohexaferrites are highly resistive materials with hard magnetic properties and find their applications in permanent magnets, magnetic recording media and high-frequency microwave devices.
1. Introduction
M-type barium nanohexaferrites and its doped nanocomposites are highly resistive ferrimagnetic compounds having iron as their main constituent (Valenzuela, Citation2012). These magnetically hard materials are broadly being explored by the researchers for their significant magnetic and electrical properties like large magneto-crystalline anisotropy, high coercivity, excellent corrosion resistance and chemical stability (Castro, Corrêa, Paulim Filho, Rivas Mercury, & Cabral, Citation2015; Ounnunkad, Citation2006; Wu & Yang, Citation2007). The M-type hexagonal ferrites cover a wide arena of technological applications. Owing to their high resistivity and high permeability, these nanomaterials are used in permanent magnets, magneto optical devices, magnetic recording media and high-frequency microwave devices, especially in the development of microwave absorbers (Cho & Kim, Citation1999; Pullar, Citation2012).
The dielectric and the magnetic behaviour of ferrites are subjective to several factors like synthesis technique, chemical composition, calcination temperature, crystallite size and the type of dopant used (Cernea et al., Citation2016; Din et al., Citation2014; Iqbal & Farooq, Citation2010; Mallick, Shepherd, & Green, Citation2007a). The effect of frequencies on the electrical behaviour offers much valued information about the charge carriers which in turn helps to explicate the mechanisms responsible for charge transport phenomena and its dielectric behaviour (Cernea et al., Citation2016).
However, endeavours are being made to alter the structure of barium nanohexaferrites and to analyse the effect of such structural modifications on their magnetic and electrical behaviour. These characteristics can be reformed by doping the pure form of barium hexaferrites with the divalent ions of transition metal elements like nickel which replaces the Fe ions because of their comparative ionic radius and electronic configuration (Gordani, Ghasemi, & Saidi, Citation2014; Li et al., Citation2015; Mallick et al., Citation2007a; Mallick, Shepherd, & Green, Citation2007b; Vinnik et al., Citation2015). The magnetic properties are a result of the occupancy of iron ions at the spin up and spin down positions of the crystal structure which gets modified due to the replacement with nickel ions. Also, the alteration in electronic properties with the addition of nickel occurs due to the change in behaviour of electrons that hop from octahedral to tetrahedral positions in the lattice.
Different techniques available for the synthesis of barium nanohexaferrites include the sol–gel (Kanagesan et al., Citation2014), ball milling (Ding, Yang, Miao, McCormick, & Street, Citation1995), hydrothermal (Giri, Samanta, Maji, Ganguli, & Bhaumik, Citation2005), combustion method (Ganjali, Ganjali, Eskandari, & Aminzare, Citation2013) and chemical co-precipitation (Iqbal & Ashiq, Citation2008; Kumar, Chitkara, Sandhu, Mehta, & Kumar, Citation2015; Radwan, Rashad, & Hessien, Citation2007). In this work, chemical co-precipitation technique is used to synthesize pure and nickel-doped barium nanohexaferrites since it is a reliable method that offers much control over the chemical composition and the crystallite size of the material. The measurement of electrical properties includes the DC electrical resistance from the I–V characteristics and the complex dielectric permittivity, dielectric loss and loss tangent with frequency as a parameter (Cernea et al., Citation2016; Iqbal & Farooq, Citation2010). Further, the magnetic properties involve the investigation of M–H curve for measurement of saturation magnetization (Ms), remanence (Hr) and coercivity (Hc).
2. Method of synthesis
Samples of pure barium nanohexaferrites (BaFe12O19) and doped samples of nickel barium nanohexaferrites (BaNixFe(12-x)O19) with x = 0.01, 0.05, 0.1 for nickel concentration to be 1, 5 and 10%, respectively, are synthesized using the chemical co-precipitation method followed by calcination at 800°C for 4 h. A part of the sample of pure barium nanohexaferrite is calcined at 600°C and is named as Pure600 and the other calcined at 800°C is named as Pure800. For pure sample, stoichiometric quantities of barium chloride di-hydrated (BaCl2·2H2O: Fisher Scientific, Purity min. 99%) and ferric chloride hexa-hydrated (FeCl3·6H2O: SD Fine Chemical, Purity min. 97%) having Fe/Ba molar ratio of 12:1 are mixed homogeneously in distilled water with continuous stirring for a few hours to form a consistent and homogeneous solution. An alkaline solution of 10 M NaOH is added drop by drop to this salt solution while the pH is checked continuously and the stirring is sustained. With the further addition of NaOH, the pH value increases and the burette is closed as soon as the pH of 12 is achieved (Iqbal & Ashiq, Citation2008). The stirring is still continued to maintain the homogeneity of the mixture. At this point, dark brown precipitates are formed which are further filtered out and washed many times using de-ionized water and later washed with ethanol to get rid of the excess amount of chlorine and other by-products. Drying of the washed precipitates is done in an oven at 100°C for 24 h in order to properly remove the water from it. The dried samples are then grinded properly to prepare the powdered form. Further, in a muffle furnace, the calcination at 800°C for 4 h is done followed by a final grinding.
For the nickel-doped barium nanohexaferrites, calcined at 800°C, a similar synthesis route is followed in which stoichiometric amount of NiCl2·6H2O (SDFCL; Purity: 97%) is used as an additional precursor at the first stage. The selection of 800°C as the calcination temperature for Ni-doped samples is done based on the results obtained for pure barium nanohexaferrite calcined at 800 and 600°C.
The prepared samples are characterized for their crystallography using the powder X-ray diffraction (XRD) technique. For morphological characterization using TEM, the sample under test is prepared by sonicating the powdered sample with ethanol for 10–15 min. The study of magnetic properties using VSM involves the use of powdered sample directly. Further, the two-probe method makes use of the pellet which is prepared from the powdered sample using a pellet making tool with a pressure of 10 tonnes. The pellet is kept between the two copper electrodes in series with a variable voltage source and a digital nano-ammeter which measures the value of currents for varied values of voltages.
3. Results and discussion
3.1. Crystallographic and morphological structure
The XRD is used to determine the XRD patterns of the pure and nickel-doped barium nanohexaferrites. This is achieved using the PAN-Analytic XRD tool X’PERT Pro using CuKα (0.154 nm) radiation at room temperature. The specimen length is kept as 10 mm and the range of the scattering angle is between 10 and 80° with a step size of 0.0170°. The XRD patterns are shown in Figures and .
Figure 1. XRD patterns presenting the phase of crystal structure of pure barium nanohexaferrites on varying the calcination conditions.
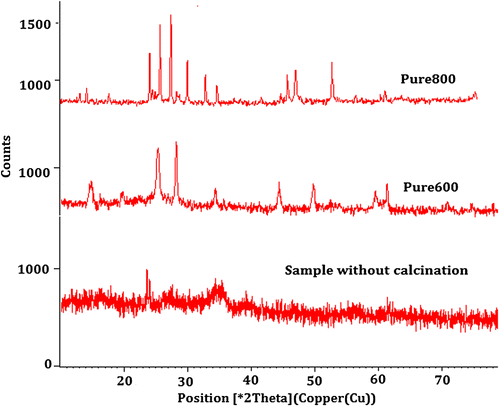
Figure 2. XRD patterns presenting the nickel-doped structure of pure barium nanohexaferrite with different values of nickel concentration.
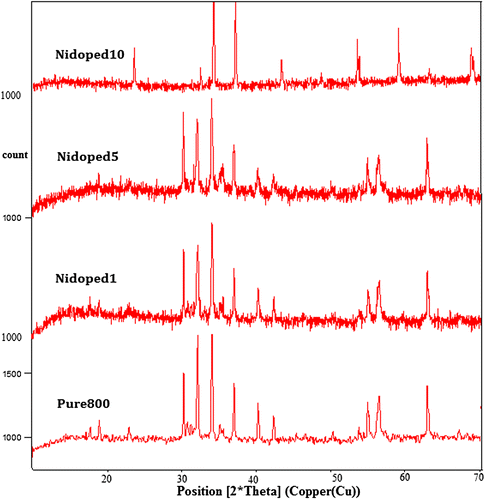
Figure shows the effect of calcination in which the pure barium nanohexaferrite shows a phase transition from amorphous to crystalline state. Also, the crystallinity increases as the temperature is increased and finally at a temperature of 800°C for sample named Pure800, hexaferrite phase formation takes place. The diffused pattern in XRD results is related to the existence of haematite phase (Fe2O3) which can be associated with the results as reported by Mossbauer spectra in previous research (Ding et al., Citation1995). According to the Mossbauer spectra, no hexaferrite phase is observed for the samples calcined at temperatures below 750°C. Further, Figure shows the effect of doping with nickel on pure barium nanohexaferrites. By the use of Debye–Scherrer equation, the size of the crystallite is computed and listed in Table .
Table 1. Size calculation of synthesized samples for calcination duration of 4 h using Debye–Scherrer equation
As observed, the steady increase in the size of the crystallite with increasing concentration of nickel is due to the substitution of Fe ions by Ni ions of comparatively larger ionic radius. Also, the increase in the size of pure barium nanohexaferrite with the increasing calcination temperatures from 600 to 800°C is also observed.
Figures and show the TEM results of morphological characterization of pure and nickel-doped barium nanohexaferrites that verify its magnetoplumbite hexagonal shape. The TEM results also confirm the nanosize of the crystals. Also, a variation in size and hexagonal shape of nickel-doped samples is observed from their TEM micrographs.
3.2. Magnetic properties
The magnetic hysteresis loop of pure form of barium nanohexaferrites for both the samples calcined at 600 and 800°C is as shown in Figures and . On comparing the hysteresis loop for Pure600 and Pure800, a significant change in the hysteresis loop is observed.
The pure barium nanohexaferrite when calcined at 600°C results in a small value of Ms (<10 emu/g) and a moderate magnetic Hc (~2 KOe). Such small value of Ms for Pure600 is a result of the presence of more amorphous haematite phase as compared to the hexaferrite phase in Pure800 which can also be verified from the peaks of diffraction pattern (Ding et al., Citation1995). Moreover, the haematite phase shows anti-ferromagnetic behaviour which leads to the reduced magnetic properties (Willis & Rooksby, Citation1952). Further, when the temperature of calcination is increased from 600 to 800°C, which is considered as an appropriate temperature for the hexaferrite phase, a significant increase in the value of both Ms and Hr and magnetic coercivity Hc is observed.
Figure shows the hysteresis loop for nickel as a dopant for the barium nanohexaferrite in which there is an increase in Ms and Hr but reduction in Hc. This reduced Hc is a result of change in axis of magnetization which disturbs the uniaxial anisotropy of pure barium nanohexaferrites, whereas the increased value of Ms accounts to the increase in size of the crystallite for nickel-doped barium nanohexaferrites as compared to pure barium nanohexaferrites as nickel might have replaced the Fe ions at the spin down position of the lattice. The magnetic parameters calculated from the hysteresis loop are summarized in Table .
Table 2. Magnetic parameters of pure and doped barium nanohexaferrite samples
3.3. Electrical properties
On placing a dielectric material in an alternating field, the reversal of the direction of the field leads to the change in the orientation of the dipoles. The dipole orientation follows the change in field direction easily at lower frequencies, whereas there is a lag in the change in orientation of the dipole in case of higher frequencies due to the inertial and the spatially oriented defects (Cernea et al., Citation2016; Mallick et al., Citation2007a). The complex formulation of the dielectric permittivity involves the storage of electromagnetic energy and the thermal conversion as a function of frequency as shown in Equation (1).(1)
(1)
where the real part () shows the storage of electromagnetic energy from the AC field and the imaginary part (
) shows the thermal conversion or the dielectric losses. The calculation of real and imaginary parts is done using a parallel plate capacitor set-up with LCR meter. The dielectric parameters such as dielectric constant
, dielectric loss
and loss tangent (tan δ) are calculated using Equations (2)–(4) in which t is the thickness of the pellet, C is the capacitance of the dielectric material, C° is free space capacitance, G is conductivity and A is the area of the plate.
(2)
(2)
(3)
(3)
(4)
(4)
Figure shows the real part of the complex dielectric permittivity as a function of frequency for pure and nickel-doped samples. It can be observed that at lower frequencies, the dielectric constant shows a large value in the range of 4 × 106 for pure and 1 × 106 for nickel-doped sample. With the increase in frequency, there is a significant decline in relative permittivity and it becomes almost constant when it reaches the kHz range.
Figure 8. Real part of the relative permittivity of pure and nickel-doped barium nanohexaferrites as a function of logarithm of frequency.
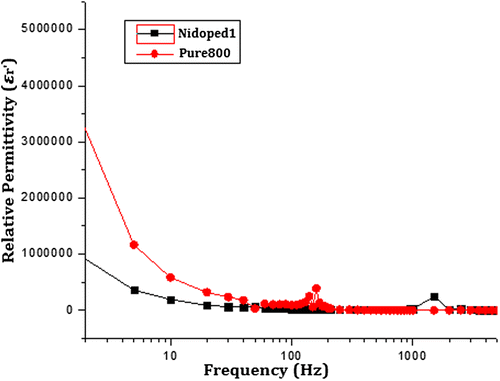
Similarly, Figure shows the imaginary component of relative permittivity as a function of frequency. Since the formula for dielectric loss includes frequency in the denominator, there is an inverse relationship between the two parameters which can be clearly observed from the graph.
Figure 9. Imaginary part of relative permittivity of pure and nickel-doped barium nanohexaferrites as a function of frequency.
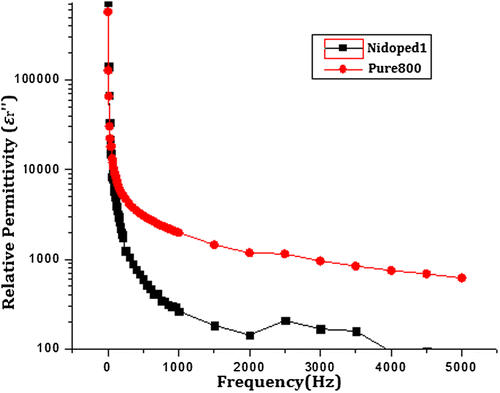
Also, the dielectric loss tangent, which is the ratio of the imaginary part to the real part, is plotted as a function of frequency in Figure . It can be observed that nickel-doped BaM shows higher loss tangent up to 0.4 at lower frequencies while the pure BaM starts with lower loss tangent and increases as the frequency increases.
Figure 10. Loss tangent of pure and nickel-doped barium nanohexaferrites as a function of frequency.
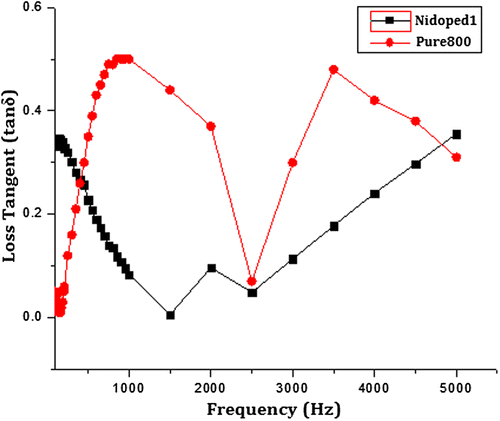
Moreover, the highly resistive behaviour of barium nanohexaferrites can be observed from the I–V characteristics drawn using the two-probe method as shown in Figure . The resistance of pure barium nanohexaferrites is calculated from the slope of the curve and is found to be ~2 MΩ, whereas the doping with nickel leads to an increase in DC resistance to ~6 MΩ. This variation in electrical and magnetic properties due to doping is possibly attributed to the different site occupancies by the dopant.
4. Conclusion
In the present investigations, crystallographic and morphological characteristics along with the frequency-dependent dielectric behaviour and magnetic characteristics of pure and nickel-doped barium nanohexaferrites synthesized using chemical co-precipitation technique are explored. From the crystallographic characterization, it is found that a phase transition from amorphous to hexaferrite phase occurs at a temperature of 800°C and also that there is an increase in the crystallite size as the calcination temperature is raised from 600 to 800°C. The magnetoplumbite hexagonal structure is verified from the TEM results. Further, the magnetic properties studied from the VSM confirm that barium nanohexaferrites are hard ferrites with coercivities up to 5 KOe. The use of nickel enhances the Ms while the Hc is reduced which is due to the reduced magneto-crystalline anisotropy as Ni ions replace the Fe ions in the crystal structure. Also, the investigations of the DC and AC electrical properties reveal higher resistance and lossy dielectric behaviour of the material. Such high resistance combined with larger dielectric losses at high frequencies and large magnetic Hc makes these materials useful for applications in permanent magnets and electromagnetic interference suppressors.
Additional information
Funding
Notes on contributors
Naini Dawar
Naini Dawar did her BTech in ECE from UIET, Kurukshetra University in 2013. Presently, she is pursuing her ME fellowship in ECE from Chitkara University, Punjab. Her areas of research include Nanoscience and Technology, Nanoferrites and their applications.
Mansi Chitkara
Mansi Chitkara obtained her PhD degree in Mechanical Engineering from PTU and ME degree in Industrial Materials & Metallurgy from PEC, Chandigarh. Her research area includes Nanomaterials, Nanoherbal materials: Synthesis and Characterization.
Inderjeet Singh Sandhu
Inderjeet Singh Sandhu obtained his PhD degree in Solid-State Physics and MSc degree in Physics from Kurukshetra University, Kurukshetra. His research areas include Nanomaterials, Nanoelectronics and Nanoelectrodynamics.
Jaspreet Singh Jolly
Jaspreet Singh Jolly completed his BTech in ECE from PTU, Punjab. His areas of research include nanoferrites: synthesis and characterization.
Shivani Malhotra
Shivani Malhotra completed her MTech from Punjab University, Punjab. She is currently pursuing PhD from Chitkara University, Punjab, and is working in the area of synthesis and characterization of nanohexaferrites.
References
- Castro, W. S., Corrêa, R. R., Paulim Filho, P. I., Rivas Mercury, J. M., & Cabral, A. A. (2015). Dielectric and magnetic characterization of barium hexaferrite ceramics. Ceramics International, 41, 241–246.10.1016/j.ceramint.2014.08.064
- Cernea, M., Negrea, R. F., Ciuchi, I. V., Baldisserri, C., Trusca, R., & Galassi, C. (2016). Dielectric characterization of BaxSr1−xFe12O19 (x=0.05−0.35) ceramics. Ceramics International, 42, 1050–1056.10.1016/j.ceramint.2015.09.029
- Cho, H. S., & Kim, S. S (1999). M-hexaferrites with planar magnetic anisotropy and their application to high-frequency microwave absorbers. IEEE Transactions on Magnetics, 35, 3151–3153.
- Din, M. F., Ahmad, I., Ahmad, M., Farid, M. T., Iqbal, M. A., Murtaza, G., … Khan, M. A. (2014). Influence of Cd substitution on structural, electrical and magnetic properties of M-type barium hexaferrites co-precipitated nanomaterials. Journal of Alloys and Compounds, 584, 646–651.10.1016/j.jallcom.2013.09.043
- Ding, J., Yang, H., Miao, W. F., McCormick, P. G., & Street, R. (1995). High coercivity Ba hexaferrite prepared by mechanical alloying. Journal of Alloys and Compounds, 221, 70–73.10.1016/0925-8388(94)01402-7
- Ganjali, M., Ganjali, M., Eskandari, M., & Aminzare, M. (2013). Effect of heat treatment on structural and magnetic properties of nanocrystalline SrFe12O19 hexaferrite synthesized by Co-precipitation method. Journal of Advanced Materials and Processing, 1, 41–48.
- Giri, S., Samanta, S., Maji, S., Ganguli, S., & Bhaumik, A. (2005). Magnetic properties of α-Fe2O3 nanoparticle synthesized by a new hydrothermal method. Journal of Magnetism and Magnetic Materials, 285, 296–302.10.1016/j.jmmm.2004.08.007
- Gordani, G. R., Ghasemi, A., & Saidi, A. (2014). Enhanced magnetic properties of substituted Sr-hexaferrite nanoparticles synthesized by co-precipitation method. Ceramics International, 40, 4945–4952.10.1016/j.ceramint.2013.10.096
- Iqbal, M. J., & Ashiq, M. N. (2008). Physical and electrical properties of Zr–Cu substituted strontium hexaferrite nanoparticles synthesized by co-precipitation method. Chemical Engineering Journal, 136, 383–389.10.1016/j.cej.2007.05.046
- Iqbal, M. J., & Farooq, S. (2010). Impact of Pr–Ni substitution on the electrical and magnetic properties of chemically derived nanosized strontium–barium hexaferrites. Journal of Alloys and Compounds, 505, 560–567.10.1016/j.jallcom.2010.06.073
- Kanagesan, S., Hashim, M., Kalaivani, T., Ismail, I., Rahman, N. A., & Hajalilou, A. (2014). Microwave sintering of Ni-Co doped barium strontium hexaferrite synthesized via sol-gel method. Journal of Chemical and Pharmaceutical Research, 6, 1210–1215.
- Kumar, K., Chitkara, M., Sandhu, I. S., Mehta, D., & Kumar, S. (2015). Photocatalytic and magnetic properties of Zn1−xCrxO nanocomposites prepared by a co-precipitation method. Materials Science in Semiconductor Processing, 30, 142–151.10.1016/j.mssp.2014.10.001
- Li, J., Zhang, H., Liu, Y., Li, Q., Ma, G., & Yang, H. (2015). Structural and magnetic properties of M-Ti (M = Ni or Zn) co-substituted M-type barium ferrite by a novel sintering process. Journal of Materials Science: Materials in Electronics, 26, 1060–1065.
- Mallick, K. K., Shepherd, P., & Green, R. J. (2007a). Dielectric properties of M-type barium hexaferrite prepared by co-precipitation. Journal of the European Ceramic Society, 27, 2045–2052.10.1016/j.jeurceramsoc.2006.05.098
- Mallick, K. K., Shepherd, P., & Green, R. J. (2007b). Magnetic properties of cobalt substituted M-type barium hexaferrite prepared by co-precipitation. Journal of Magnetism and Magnetic Materials, 312, 418–429.10.1016/j.jmmm.2006.11.130
- Ounnunkad, S. (2006). Improving magnetic properties of barium hexaferrites by La or Pr substitution. Solid State Communications, 138, 472–475.10.1016/j.ssc.2006.03.020
- Pullar, R. C. (2012). Hexagonal ferrites: A review of the synthesis, properties and applications of hexaferrite ceramics. Progress in Materials Science, 57, 1191–1334.10.1016/j.pmatsci.2012.04.001
- Radwan, M., Rashad, M. M., & Hessien, M. M. (2007). Synthesis and characterization of barium hexaferrite nanoparticles. Journal of Materials Processing Technology, 181, 106–109.10.1016/j.jmatprotec.2006.03.015
- Valenzuela, R. (2012). Novel applications of ferrites. Physics Research International.
- Vinnik, D. A., Zherebtsov, D. A., Mashkovtseva, L. S., Nemrava, S., Semisalova, A. S., Galimov, D. M., … Niewa, R. (2015). Growth, structural and magnetic characterization of Co-and Ni-substituted barium hexaferrite single crystals. Journal of Alloys and Compounds, 628, 480–484.10.1016/j.jallcom.2014.12.124
- Willis, B. T. M., & Rooksby, H. P. (1952). Crystal structure and antiferromagnetism in haematite. Proceedings of the Physical Society. Section B, 65, 950–954.10.1088/0370-1301/65/12/304
- Wu, K. H., & Yang, F. C. (2007). Synthesis and characterization of organically modified silicate/NiZn ferrite hybrid coatings. Acta Materialia, 55, 507–515.10.1016/j.actamat.2006.08.041