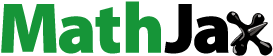
Abstract
Four heterobimetallic derivatives of the type, [R2(Cl)SnL]PdCl2 (R = n-Bu: 1)/[R3SnL]PdCl2 (R = Me: 2; n-Bu: 3; Ph: 4) where L = 4-(2-hydroxyethyl)piperazine-1-carbodithioic acid, have been synthesized by refluxing 4-(2-hydroxyethyl)piperazine-1-carbodithioic acid with R2SnCl2/R3SnCl in 1:1 M/L ratio and then stirring with PdCl2 at room temperature. The complexes have been characterized by various spectroscopic (IR, 1H and 13C NMR, and EI-MS) techniques and thermogravimetric analysis. FTIR data demonstrated the bidentate binding of dithiocarbamate group. The NMR data demonstrated 4-coordinated geometry in solution. The complex 4 exhibits the interaction with salmon sperm DNA (SS-DNA) with significant hypochromic effect and suggests intercalating mode of binding. The complex 2 was found most active for inhibition of alkaline phosphates enzyme (ALPs). The complexes 1–4 showed significant antimicrobial activities as compared to the inactive free ligand precursor. The in vitro hemolytic activity showed that average lysis of human red blood cells was significantly lower as compared to Triton-X 100 (positive control, 100% lysis).
Public Interest Statement
Heterobimetallic (Sn, Pd) complexes have been synthesized with 4-(2-hydroxyethyl)piperazine-1-carbodithioic acid and characterized by IR, 1H and 13C NMR, EI-MS, and TGA techniques. The complexes demonstrated the 4-coordinated geometry around Sn(IV) and Pd(II) in both the solid and solution states. The complexes exhibited an inhibition of alkaline phosphates enzyme (ALPs) and an interaction with salmon sperm DNA (SS-DNA). The complexes showed significant antimicrobial activities as compared to free ligand. The in vitro hemolytic activity showed that average lysis of human red blood cells was significantly lower as compared to Triton-X 100 (positive control, 100% lysis).
1. Introduction
Considerable attention has been focused on the synthesis of heterobimetallic complexes owing to their potential use in photochemical molecular devices and as light sensitive probes in biological systems (Balzani, Juris, Venturi, Campagna, & Serroni, Citation1996). It has been observed that heterobimetallic complexes containing both electron-deficient and electron-rich transition metal ions behave in a cooperative manner leading to an enhanced reactivity (Wark & Stephan, Citation1990). These systems are of great interest because of their structural diversities, molecular magnetism, and electrical conductivities (Robertson & Cronin, Citation2002). The presence of two metal ions in close proximity mimic the active site of several metalloenzymes and offers an opportunity to study the metal–proteins and metal–enzyme interactions (Magnus, Ton-That, & Carpenter, Citation1994). Chemistry of organotins attracts interest due to several applications in the form of potential antineoplastic and antituberculosis agents (Zhang, Song, Li, Liu, & Tang, Citation2007), PVC stabilizers (Arkış & Balköse, Citation2005), and antitumor drugs (Tabassum & Pettinari, Citation2006) as well as polymer catalysts (Angiolini et al., Citation2006). Numerous studies on organotin(IV) complexes have been carried out to explore their biological properties against bacterial, fungal strains, and cancer cells line (Hadjikakou & Hadjiliadis, Citation2009; Hadjikakou, Ozturk, Xanthopoulou, & Zachariadis, Citation2008). Recently, significant attention has been focused on the DNA binding properties of dithiocarbamate metal complexes among which Pd(II) and Pt(II) complexes of dithiocarbamates have gained special interest due-to-their potential antitumor properties (Islami-Moghaddam, Mansouri-Torshizi, Divsalar, & Saboury, Citation2009; Mansouri-Torshizi, Moghaddam, Divsalar, & Saboury, Citation2009). Currently, cis-platin is one of the most effective anticancer drug, however, there is an increase of interest in palladium(II) complexes containing N and S donor ligands in recent years, with the aim to synthesize antitumor drugs having a reduced toxicity with respect to cis-platin and analogs (Mansouri-Torshizi et al., Citation2011).
Keeping in view, wide scope and the applications of organotin(IV)/Pd(II) complexes, we extend our investigations from organotin chemistry (Hussain et al., Citation2011; Hussain et al., Citation2012; Shahzadi & Ali, Citation2008) to the synthesis and characterization of heterobimetallic (Sn, Pd) complexes with 4-(2-hydroxyethyl)piperazine-1-carbodithioic acid. The complexes have been characterized by various spectroscopic techniques and thermogravimetric analyses. DNA binding and alkaline phosphatase inhibition studies of the complexes were also carried out. The complexes were screened against various strains of bacteria/fungi and their minimal inhibitory concentrations were also evaluated. In vitro hemolytic activities were performed to check their possible toxic effects on human red blood cells.
2. Materials and methods
Dibutyltin dichloride, trimethyltin chloride, tributyltin chloride, triphenyltin chloride, palladium chloride, and carbon disulfide were purchased from Sigma-Aldrich (USA) and used without any further purification. 2-(1-Piperazinyl)ethanol was purchased from Merck (Germany). AR grade solvents of Merck (methanol) and Lab-scan (DMSO) origin were used. Methanol was dried before use by a standard procedure (Armarego & Chai, Citation2003). p-Nitrophenyl phosphate hexahydrate (pNPP), diethanolamine, and magnesium chloride were purchased from Sigma-Aldrich and used as such. Human serum was used as a source of alkaline phosphatase. Nutrient agar and potato dextrose agar were procured from Oxoid, Hampshire, UK.
The samples were taken in capillary tube and their melting points were recorded by using an electrochemical melting point apparatus Stuart SMP3 and are uncorrected. Infrared spectra were recorded by a Perkin-Elmer-100 FTIR spectrophotometer in the range of 4,000–250 cm−1. The percentage composition of C, H, N, and S were determined by using CHNS-932 elemental analyzer, Leco (USA). The 1H and 13C NMR spectral measurements were made at 300 and 75 MHz, respectively, by Bruker ARC 300 MHz-FT-NMR spectrometer. The EI mass spectrum was recorded using Thermo Fisher Exactive Orbitrap instrument. TGA spectra were obtained by TGA-7 Perkin-Elmer USA under nitrogen atmosphere.
The complexes were tested for their interaction with salmon sperm DNA (SS-DNA) (Sastri, Eswaramoorthy, Giribabu, & Maiya, Citation2003; Zhang et al., Citation2011) and alkaline phosphatase enzyme (ALPs) (Malik et al., Citation2011). The ligand and complexes were screened to check their in vitro response against various strains of bacteria (Escherichia coli, Bacillus subtilis, Staphlocuccus aureus, and Pasturella multocida) and fungi (Alternaria alternata, Trichoderma harzianum, and Aspergillus niger) by disc diffusion method (Clinical Laboratory Standards Institute [CLSI], Citation2007) as well as minimum inhibitory concentration (MIC) (Sarker, Nahar, & Kumarasamy, Citation2007). The activities were performed in an incubator (Sanyo, Germany) and sterilized in an autoclave (Omron, Japan). The minimum inhibitory concentrations were determined in a Micro Quant apparatus (BioTek, USA). Streptomycin and fluconazole were used as standard drugs for antibacterial and antifungal screening tests, respectively. The in vitro hemolytic bioassay (Sharma & Sharma, Citation2001) of the complexes was performed with human red blood cells.
2.1. Synthesis
2.1.1. Synthesis of 4-(2-Hydroxyethyl)piperazine-1-carbodithioic acid (HL)
2-(1-Piperazinyl)ethanol (1 mmol) and CS2 (1 mmol) were stirred together in methanol (30 mL) in a round bottom two necked flask (250 mL) for 0.5 h. The precipitates obtained were filtered off and dried in air.
2.1.2. General procedure for synthesis of complexes 1–4
4-(2-Hydroxyethyl)piperazine-1-carbodithioic acid (1 mmol) was dissolved in chloroform (150 mL) in a round bottom two necked flask (250 mL) with continuous stirring. Then R2SnCl2/R3SnCl (1 mmol) was added as a solid in portions and the solution was refluxed for 3 h. The reaction mixture was cooled at room temperature and then PdCl2 (1 mmol) solution in distilled water (25 mL) was added drop wise and reaction mixture was further stirred for 2 h with constant stirring resulting in the appearance of orange colored precipitates. The precipitates obtained were filtered off and dried in air. The complexes were recrystallized in acetone and pet.ether (2:1).
2.2. DNA interaction study
A solution of 0.2 g of salmon sperm DNA (SS-DNA) in double deionized water (100 mL) was prepared and kept at 4°C. DNA solution in 20 mM Tris-HCl (pH 7.4) gave the ratio of UV absorbance at 260 and 280 nm, A260/A280, of 1.86 which demonstrated that DNA was free from protein (Zhang et al., Citation2011). The DNA concentration was found to be 7.45 × 10−5 M via absorption spectroscopy at 260 nm using the molar absorption coefficient of 6,600 M−1 cm−1 (Sastri et al., Citation2003). From this stock solution, working solutions (10, 19, 27, 35, 42, 48, 54, 59, 64, and 69 μM) were prepared. Solution of the complex with a concentration of 2 × 10−6 M was prepared in 90% DMSO. The UV absorption titrations were performed by keeping the concentration of complexes constant (2 mM) and varying the concentration of DNA. Equivalent solutions of DNA were added to the complex and the reference solutions to eliminate the absorbance of DNA itself. Compound-DNA solutions were incubated at ambient temperature for 30 min before performing the measurements. Absorption spectra were recorded by using the cuvettes of 1 cm path length.
2.3. Alkaline phosphatase activity
The assay of alkaline phosphatase activity was carried out by reported method (Malik et al., Citation2011) with slight modification. A working substrate was prepared by mixing the four parts of reagent A (diethanolamine pH = 9.8, 2 mol/dm3 and magnesium chloride 0.5 mmol/dm3) and one part of reagent B (p-nitrophenyl phosphate 50 mmol/dm3). The substrate was incubated for five minutes at 25°C. In a cell cuvette, 2 mL of the substrate was taken and 40 μL of human serum having the activity of 165 IU/L was added. After incubation of 1 min, the absorbance was recorded to check the activity of the enzyme. ALP hydrolyzed the p-NPP and yellow colored p-nitrophenol was produced that absorbed at 405 nm. The assay of the alkaline phosphatase activity gets the advantage of the fact that the enzyme utilizes the non-biological substrate p-nitrophenyl phosphate and it is non-specific.
Then various amounts of complexes were added periodically from the 25 mM stock solution and again incubated for 3 min. Absorbance was recorded again 1, 2, 3, 4, and 5 min thereafter. At the end, the average was taken and the percentage inhibition was calculated.
2.4. Biological activities
Antibacterial and antifungal activities were determined by disc diffusion method (CLSI, Citation2007). Small filter paper discs (size, 9 mm), each soaked with 100 μL sample solution, were placed flat on a growth medium (nutrient agar for bacteria and potato dextrose agar for fungi) containing microbes and the Petri plates were incubated for 24 h at 37°C for bacterial growth and for 48 h at 28°C for fungal growth. The biologically active samples inhibited the bacterial/fungal growth to form the clear zones, which were measured by a zone reader. The ligand and the products were also evaluated for their MICs by a modified resazurin microtitre plate assay (Sarker et al., Citation2007). MIC values against fungal strains were noted using nutrient broth medium and resazurin indicator. For fungal strains sabouraud dextrose agar (SDA) medium was used and no indicator was involved.
2.5. Hemolytic activity
Three milliliter of heparinized human blood was freshly obtained from volunteers after consent. Then it was mixed gently, transferred into a 15 mL sterile polystyrene screw-cap tube and centrifuged for 5 min at 850 g. The resultant supernatant mass was poured off and the viscous pellet was washed three times with 5 mL of chilled (4°C) and sterile isotonic phosphate-buffered saline (PBS) of pH 7.4. The washed cells were suspended in 20 mL sterile chilled PBS and counted on a hemocytometer. The blood cell suspension retained on wet ice was diluted for each assay with sterile PBS to 7.068 × 108 cells ml−1. Aliquots of 20 μL of each compound solution were placed aseptically into 2 mL microfuge tubes. For each assay, 0.1% Triton-X 100 was used as a positive control (100% lysis) and PBS as a negative control (0% lysis). Then aliquot of 180 μL diluted blood cell suspension was aseptically placed into each 2 mL tube and gently mixed with a wide mouth pipette tip three times. The tubes were incubated with agitation (80 rpm) at 37°C for 35 min and then placed for 5 min on ice followed by centrifugation at 1,310 g for 5 min. Aliquots of 100 μL of supernatant were collected, placed into a sterile 1.5 mL microfuge tube and then diluted with 900 μL chilled and sterile PBS. All the tubes were maintained on wet ice after dilution. Absorbance was then noted on a microquant at 576 nm. The experiment was performed triplicate. Percent hemolysis was found by following formula (Sharma & Sharma, Citation2001):
3. Results and discussion
The complexes are stable in air having sharp melting points and are soluble in common organic solvents. The physical data are given in Table .
Table 1. Physical data of the complexes 1–4
3.1. IR spectroscopy
Infrared spectra of the ligand HL and the complexes 1−4 were recorded in the range of 4,000–250 cm−1. The most important bands are given in Table .
Table 2. IR data (cm−1) of complexes 1–4
A peculiar feature of the IR spectra is the appearance of a broad band at 3,231 and 2,573 cm−1 for υOH and υSH vibrations, respectively, in the free ligand HL. Disappearance of these bands confirm complexation due to deprotonation of –OH and –CSSH moieties for coordination with Sn(IV) and Pd(II), respectively. The υC–S band observed at 1,076 cm−1 in HL was shifted to lower frequency lie in the range of 980–985 cm−1 in the complexes 1–4, indicating the metal sulfur coordination, which is also supported by appearance of υPd-S bands in the range of 355–370 cm−1 (Singh, Fahmi, & Biyala, Citation2005). The observed υ(C–N) vibrations (1,429–1,593 cm−1) lie between the range as reported earlier (Fregona et al., Citation2003) for C–N single bonds (1,250–1,360 cm−1) and for C=N double bonds (1,640–1,690 cm−1). Thus, υ(N-CSS) mode has been shifted to higher frequencies in complexes relative to the HL indicating the appearance of a partial double bond character in CSS− anion, thus enabling its bidentate coordination with Pd(II) metal (Fregona et al., Citation2003). New bands were appeared in the range 471–502 cm−1 in the complexes 1–4 due to υSn–O vibrational mode and the bands in the range 522–542 cm−1 and at 254 cm−1 were assigned to υSn–C vibration in complexes 1–3 and triphenyltin(IV) complex 4, respectively.
3.2. 1H NMR spectroscopy
The 1H NMR data is given in Table . The signals were assigned by their distinct multiplicity patterns, resonance intensities, coupling constants, and tin satellites. The number of protons found by integration of peaks in the spectra agreed very well with those calculated from the expected composition.
Table 3. 1H NMR data of complexes 1–4
The singlet resonance occurring at 4.95 ppm and 4.49 ppm was assigned to the –SH and –OH protons, respectively, in the dithiocarbamate ligand (HL). The absence of these resonances ( –OH/ –SH) in the spectra of the complexes verified bimetallic coordination through deprotonated hydroxyl/dithiocarbamate moieties as also indicated by IR spectroscopy. The methyl protons in complex 2 appeared as singlet at 1.05 ppm with 2J(119Sn-1H) coupling constant of 55 Hz. The C−Sn−C bond angle (109°) calculated from 2J(119Sn-1H) value (Lockhart, Manders, & Holt, Citation1986) supports the tetrahedral geometry around tin(IV) in the complex 2. Despite the complex pattern of di- and tri-n-butyl fragments in the spectra, a clear triplet due to terminal methyl group was appeared at 0.85 ppm and 0.87 ppm for the complexes 1 and 3, respectively, with 3J(1H, 1H) = 7.2 Hz in each case. Ortho protons absorbed downfield as compared to meta and para protons in the triphenyltin(IV) complex 4 (Hussain et al., Citation2012).
3.3. 13C NMR spectroscopy
The assignments of –CSS group in investigated compounds is straightforward which is observed in the range of 201.5–202.6 ppm indicating the coordination of sulfur to the tin atom. Table lists the chemical shifts of 13C and tin-carbon coupling constants for complexes 1–4. The 13C NMR chemical shifts due to the phenyl groups are observed at positions comparable to other similar compounds (Al-Hayaly et al., Citation2005; Bonati & Ugo, Citation1967). Coordination of the tin atom in complexes 1–4 has been related to nJ(119Sn-13C) coupling constants. The nJ(119Sn-13C) coupling for complexes 2 and 3 is 522 and 478 Hz, respectively, which is indicative of 4-coordinated geometry (Bonati & Ugo, Citation1967) in solution state.
Table 4. 13C NMR data of complexes 1–4
3.4. Mass spectrometry
The electron ionization mass spectrum (EI-MS) was recorded for complex 1. The mass spectral data are given in Table . The mass spectrum of complex 1 showed no molecular ion (M+•) peak. The complex 1 loses an ethoxide ion to yield [C13H25N2PdS2SnCl3]+ fragment with m/z = 604 (2%). Primary fragmentation in complex 1 occurs by eliminating PdCl2 in the first step and two butene molecules in the next two steps to generate [C7H14ClN2OS2Sn]+ fragment at m/z = 361 (4%) which then releases H2 + HCl, CS2, Sn, CO, and CH3CHNH in different steps and ultimately gave the fragment [C3H6 N]+ at m/z = 56 (66%). Primary decompostion may also generate fragment [C5H9ClN2PdS2]+ at m/z = 302 (5%), which further split up into [PdCl]+ fragment at m/z = 143 (15%) and [CS2]+ fragment at m/z = 76 (100).
Table 5. Mass spectral data of complex 1
3.5. Thermogravimetric analysis
Thermogravimetric analysis (TGA) of the ligand HL and complexes 1, 2, and 4 were performed under N2 atmosphere to evaluate their degradation pattern and thermal stability. The theoretically calculated weight loss was compared with experimental results (Table ). The thermally decomposed data agreed well with the expected chemical composition of the ligand and the complexes. The thermal stability of the complexes was found greater than that of the respective ligand, since the increase in metal content makes a complex more resistant to decomposition (Kalia, Kaushal, Lumba, & Priyanka, Citation2008). The thermal decomposition resulted in the evolution of coordinated organotin(IV) moieties as reported earlier (Sharma, Kaistha, & Bhatt, Citation2003; Valla, Bakola-Christianopoulou, Akrivos, Kojic, & Bogdanovic, Citation2006).
Table 6. Thermal decomposition data of complexes 1, 2, and 4
3.6. DNA interaction study
For complexes 1–4, DNA binding parameters were evaluated by using absorption spectroscopy. It was found that only the complex 4 exhibits binding with DNA. There exists a single band in the absorption spectrum at 281.5 nm for complex 4. The UV spectrum of complex 4 (Figure ) showed significant hypochromic effect and suggests intercalating mode of binding. After 24 h, the spectrum was again taken and obtained the same results which confirmed the stability of drug–DNA complex.
Figure 1. Absorption spectra of 2 mM of complex 4 in the absence (a) and presence of 10 μM (b), 19 μM (c), 27 μM (d), 35 μM (e), 42 μM (f), 48 μM (g), and 54 μM (h) DNA.
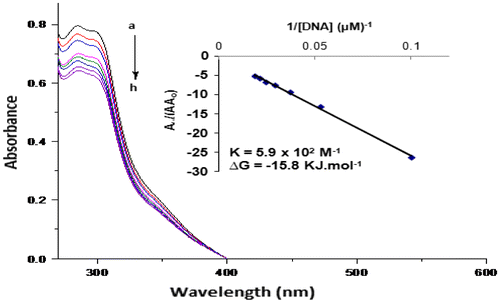
The intrinsic binding constant K for the investigated DNA active complex was calculated in order to compare binding strengths of complex–DNA and ligand–DNA by using Benesi–Hildebrand equation (Ahmad et al., Citation2007):
where K = binding constant; A0 = absorbance of the drug; A = absorbance of the drug and its complex with DNA; εG = absorption coefficient of the drug; and εH − G = absorption coefficient of the drug–DNA complex.
The association constant was obtained from the intercept-to-slope ratios of A0/(A−A0) vs. 1/[DNA] plots.
The binding constant was found to be 5.9 × 102 M−1 for complex 4. The Gibb’s free energy of complex 4 was determined by using the following equation:
where R is general gas constant (8.314 J K−1 mol−1) and T is the temperature (298 K). The Gibb’s free energy was found to be −15.8 for complex 4. The negative value suggests that the interaction of the compound with DNA is a spontaneous process.
3.7. Alkaline phosphatase activities (ALPs)
The ligand HL and complexes 1–4 were screened for their ALPs activity (Malik et al., Citation2011). Ligand was found totally inactive against the enzymatic activity, while complexes 1–4 rendered activity for inhibition of ALPs (Liu, Chou, & Humphreys, Citation1979). Inhibition of ALPs is because of blockage of active sites of the enzyme by the palladium ion. Actually, the Pd may replace the Zn or Mg metal of the enzyme and hence the enzyme fails to bind with the substrate. It may also be possible that enzyme binds with the palladium complex or palladium ion more efficiently rather than substrate. The exact mechanism is still unknown. Among the complexes 1–4, only complex 2 was the most active one due to smaller size of its organotin(IV) moiety. The complex 4 was found to be least active due to larger size of organotin moiety (Figure ).
3.8. Antimicrobial activities
The ligand and the complexes were screened to check their in vitro response against various strains of bacteria (E. coli, B. subtilis, S. aureus, and P. multocida) and fungi (A. alternata, G. lucidum, P. notatum, T. harzianum and A. niger) by disc diffusion method (CLSI, Citation2007) and measurement of MIC (Sarker et al., Citation2007). The data are given in Table .
Table 7. Antimicrobial activity data of complexes 1–4
The biological activity was found to depend mainly upon the absence/presence of organotin(IV) fragments in the complexes. The free ligand was found inactive against all the tested organisms as reported earlier (Hussain et al., Citation2015), whereas the complexes possessed comparable activity as reported in literature (Hussain et al., Citation2015; Rafiq et al., Citation2014) for other heterobimetallic complexes. The coordination of the ligand with chlorodi- or trialkyltin(IV) moities have appreciably enhanced the activities of complexes (Hussain et al., Citation2014). Antimicrobial activities varied according to the substitution pattern at tin because the function of the ligand is to support the transportation of active organotin(IV) moiety to the site of action where it is released by hydrolysis (Pellerito, Nagy, Pellerito, & Szorcsik, Citation2006).
3.9. Hemolytic activity
Hemolytic activity of the ligand and complexes 1–4 was performed because, even if a compound exhibits potent antimicrobial activities, its use is not recommended in medicine in the presence of these hemolytic effects. Thus, in vitro hemolytic bioassay of the complexes was performed with human red blood cells and the average lysis was reported with respect to the triton X-100 as positive control (100% lysis) and PBS as negative control (0% lysis). The results are given in Table . The complexes 1–4 exhibit lower hemolytic activity as compared to their free ligand HL, however, the activity was found significantly lower as compared to triton-X 100. The lowest (11.98%) and highest activity (57.41%) was found for the complex 4 and complex 2, respectively.
Table 8. Hemolytic activity of complexes 1–4
4. Conclusion
We have synthesized heterobimetallic complexes with 4-(2-hydroxyethyl)piperazine-1-carbodithioic acid. The ligand and complexes have been characterized by IR, NMR, mass spectrometry, and TGA. IR data show bidentate nature of dithiocarbamate group in complexes 1–4. NMR data show 4-coordinated geometry in solution. The complex 4 exhibits the interaction with salmon sperm DNA (SS-DNA) with hypochromic effect and suggests intercalating mode of binding. In alkaline phosphatase enzyme assay, only the complex 2 was found active. The complexes exhibited significant antimicrobial activities as compared to free ligand. The hemolytic activity shows that the complexes exhibit comparatively higher hemolytic activity as compared to the free ligand.
Cover image
Source: Authors.
Additional information
Funding
Notes on contributors
Saira Shahzadi
The group is engaged in diversified research fields such as material chemistry, bioinorganic chemistry, and synthesis of biodiesel. In bioinorganic chemistry, various organometallic and coordination complexes have been synthesized and characterized by various techniques. Their biological aspects including DNA interaction, enzyme inhibition, anticancer, and antidiabetic studies have been performed.
The biodiesel was synthesized from various non-edible oils and subsequently characterized by various analytical techniques. The catalytic properties of different organometallic compounds in the synthesis of biodiesel have also been investigated. Work is also being carried out for the fabrication of functionalized carbon nanotubes and their uses in polymer matrices to enhance the thermomechanical properties.
References
- Ahmad, M. S., Hussain, M., Hanif, M., Ali, S., & Mirza, B. (2007). Synthesis, chemical characterization and biological screening for cytotoxicity and antitumor activity of Organotin (IV) derivatives of 3,4-methylenedioxy 6-nitrophenylpropenoic acid. Molecules, 12, 2348–2363.10.3390/12102348
- Al-Hayaly, L. J., Buttrus, N. H., Tarq, F., & Al-Allaf, T. A. K. (2005). Trinuclear platinum group metals complexes with o-aminphenyl thiolatotin(IV). Journal of Applied Science (Jordon), 17, 64–70.
- Angiolini, L., Caretti, D., Mazzocchetti, L., Salatelli, E., Willem, R., & Biesemans, M. (2006). Cross-linked polystyrene resins containing triorganotin-4-vinylbenzoates: Assessment of their catalytic activity in transesterification reactions. Journal of Organometallic Chemistry, 691, 3043–3052.10.1016/j.jorganchem.2006.03.016
- Arkış, E., & Balköse, D. (2005). Thermal stabilisation of poly(vinyl chloride) by organotin compounds. Polymer Degradation and Stability, 88, 46–51.10.1016/j.polymdegradstab.2004.02.021
- Armarego, W. L. F., & Chai, C. L. L. (2003). Purification of laboratory chemicals (5th ed.). London: Butterworth Heinemann.
- Balzani, V., Juris, A., Venturi, M., Campagna, S., & Serroni, S. (1996). Luminescent and redox-active polynuclear transition metal complexes. Chemical Reviews, 96, 759–834.10.1021/cr941154y
- Bonati, F., & Ugo, R. (1967). Organotin(IV) N,N-disubstituted dithiocarbamates. Journal of Organometallic Chemistry, 10, 257–268.10.1016/S0022-328X(00)93085-7
- Clinical Laboratory Standards Institute. (2007). Agar dilution and susceptibility testing of camplobacter spp. Journal of Clinical Microbiology, 45, 2758–2759.
- Fregona, D., Giovagnini, L., Ronconi, L., Marzano, C., Trevisan, A., Sitran, S., … Bordin, F. (2003). Pt(II) and Pd(II) derivatives of ter-butylsarcosinedithiocarbamate. Journal of Inorganic Biochemistry, 93, 181–189.10.1016/S0162-0134(02)00571-8
- Hadjikakou, S. K., & Hadjiliadis, N. (2009). Antiproliferative and anti-tumor activity of organotin compounds. Coordination Chemistry Reviews, 253, 235–249.10.1016/j.ccr.2007.12.026
- Hadjikakou, S. K., Ozturk, I. I., Xanthopoulou, M. N., & Zachariadis, P. C. (2008). Synthesis, structural characterization and biological study of new organotin(IV), silver(I) and antimony(III) complexes with thioamides. Journal of Inorganic Biochemistry, 102, 1007–1015.10.1016/j.jinorgbio.2007.12.027
- Hussain, S., Ali, S., Shahzadi, S., Sharma, S. K., Qanungo, K., Altaf, M., & Evans, H. S. (2011). Synthesis, characterization, and semi-empirical study of Organotin(IV) complexes with 4-(Hydroxymethyl)piperidine-1-carbodithioic Acid: X-ray structure of Chlorodimethyl-(4-hydroxymethyl piperidine-1-carbodithioato-S,S′)tin(IV). Phosphorus, Sulfur, and Silicon and the Related Elements, 186, 542–551.
- Hussain, S., Ali, S., Shahzadi, S., Sharma, S. K., Qanungo, K., & Bukhari, I. H. (2012). Homobimetallic complexes containing Sn(IV) with acetylene dicarboxylic acid: Their syntheses and structural interpretation by spectroscopic, semi-empirical, and DFT techniques. Journal of Coordination Chemistry, 65, 278–285.10.1080/00958972.2011.648186
- Hussain, S., Ali, S., Shahzadi, S., Sharma, S. K., Qanungo, K., & Shahid, M. (2014). Synthesis, characterization, semi-empirical and biological activities of Organotin(IV) carboxylates with 4-piperidinecarboxylic acid. Bioinorganic Chemistry and Applications, 2014, 1–11.
- Hussain, S., Bukhari, I. H., Ali, S., Shahzadi, S., Shahid, M., & Munawar, K. S. (2015). Synthesis and spectroscopic and thermogravimetric characterization of heterobimetallic complexes with Sn(IV) and Pd(II); DNA binding, alkaline phosphatase inhibition and biological activity studies. Journal of Coordination Chemistry, 68, 662–677.10.1080/00958972.2014.994515
- Islami-Moghaddam, M., Mansouri-Torshizi, H., Divsalar, A., & Saboury, A. A. (2009). Synthesis, characterization, cytotoxic and DNA binding studies of diimine Platinum(II) and Palladium(II) complexes of short hydrocarbon chain ethyldithiocarbamate ligand. Journal of the Iranian Chemical Society, 6, 552–569.10.1007/BF03246535
- Kalia, S. B., Kaushal, G., Lumba, K., & Priyanka. (2008). Thermoanalytical investigations of 4-methylpiperazine-1-carbodithioic acid ligand and its iron(III), cobalt(II), copper(II) and zinc(II) complexes. Journal of Thermal Analysis and Calorimetry, 91, 609–613.10.1007/s10973-007-8331-1
- Liu, T. Z., Chou, L. Y., & Humphreys, M. H. (1979). Inhibition of intestinal alkaline phosphatase by palladium. Toxicology Letters, 4, 433–438.10.1016/0378-4274(79)90108-5
- Lockhart, T. P., Manders, W. F., & Holt, E. M. (1986). Solution and solid-state molecular structures of Me2Sn(OAc)2 and its hydrolyzate, ([Me2Sn(OAc)]2O)2, by solution and solid-state carbon-13 NMR. X-ray diffraction study of the hydrolyzate. Journal of the American Chemical Society, 108, 6611–6616.10.1021/ja00281a026
- Magnus, K. A., Ton-That, H., & Carpenter, J. E. (1994). Recent structural work on the oxygen transport protein hemocyanin. Chemical Reviews, 94, 727–735.10.1021/cr00027a009
- Malik, M. R., Vasylyeva, V., Merz, K., Metzler-Nolte, N., Saleem, M., Ali, S., … Ahmad, S. (2011). Synthesis, crystal structures, antimicrobial properties and enzyme inhibition studies of zinc(II) complexes of thiones. Inorganica Chimica Acta, 376, 207–211.10.1016/j.ica.2011.06.017
- Mansouri-Torshizi, H., Moghaddam, M. I., Divsalar, A., & Saboury, A. A. (2009). Diimine Platinum(II) and Palladium(II) complexes of dithiocarbamate derivative as potential antitumor agents: Synthesis, characterization, cytotoxicity, and detail DNA-binding studies. Journal of Biomolecular Structure and Dynamics, 26, 575–586.10.1080/07391102.2009.10507273
- Mansouri-Torshizi, H., Saeidifar, M., Ghasemi, Z. Y., Khastan, M., Divsalar, A., & Saboury, A. A. (2011). 디티오카르바메이트 유도체의 새로운 1,10-페난트롤린 팔라디움(II) 착물의 DNA 결합 성질 및 세포독성에 관한 연구 [DNA binding studies and cytotoxicity of the novel 1,10-phenanthroline Palladium(II) complexes of dithiocarbamate derivatives]. Journal of the Korean Chemical Society, 55, 70–80.10.5012/jkcs.2011.55.1.070
- Pellerito, C., Nagy, L., Pellerito, L., & Szorcsik, A. (2006). Biological activity studies on organotin(IV)n+ complexes and parent compounds. Journal of Organometallic Chemistry, 691, 1733–1747.10.1016/j.jorganchem.2005.12.025
- Rafiq, M., Ali, S., Shahzadi, S., Shahid, M., Sharma, S. K., & Qanungo, K. (2014). Synthesis, characterization, and biological activities of homo- and heterobimetallic complexes of Sn(IV) and Pd(II) with 2-mercapto-5-methyl benzimidazole. Journal of the Iranian Chemical Society, 11, 169–178.10.1007/s13738-013-0287-4
- Robertson, N., & Cronin, L. (2002). Metal bis-1,2-dithiolene complexes in conducting or magnetic crystalline assemblies. Coordination Chemistry Reviews, 227, 93–127.10.1016/S0010-8545(01)00457-X
- Sarker, S. D., Nahar, L., & Kumarasamy, Y. (2007). Microtitre plate-based antibacterial assay incorporating resazurin as an indicator of cell growth, and its application in the in vitro antibacterial screening of phytochemicals. Methods, 42, 321–324.10.1016/j.ymeth.2007.01.006
- Sastri, C. V., Eswaramoorthy, D., Giribabu, L., & Maiya, B. G. (2003). DNA interactions of new mixed-ligand complexes of cobalt(III) and nickel(II) that incorporate modified phenanthroline ligands. Journal of Inorganic Biochemistry, 94, 138–145.10.1016/S0162-0134(02)00622-0
- Shahzadi, S., & Ali, S. (2008). Structural chemistry of organotin(IV) complexes. Journal of the Iranian Chemical Society, 5, 16–28.10.1007/BF03245811
- Sharma, N., Kaistha, A., Bhatt, S. S., & Chaudhry, S. C. (2003). Synthesis and characterization of Organotin(IV) 2,4‐Dinitrophenoxides. Synthesis and Reactivity in Inorganic and Metal-Organic Chemistry, 33, 497–507.10.1081/SIM-120020001
- Sharma, P., & Sharma, J. D. (2001). In vitro hemolysis of human erythrocytes—By plant extracts with antiplasmodial activity. Journal of Ethnopharmacology, 74, 239–243.10.1016/S0378-8741(00)00370-6
- Singh, R. V., Fahmi, N., & Biyala, M. K. (2005). Coordination behavior and biopotency of N and S/O donor ligands with their palladium(II) and platinum(II) complexes. Journal of the Iranian Chemical Society, 2, 40–46.10.1007/BF03245778
- Tabassum, S., & Pettinari, C. (2006). Chemical and biotechnological developments in organotin cancer chemotherapy. Journal of Organometallic Chemistry, 691, 1761–1766.10.1016/j.jorganchem.2005.12.033
- Valla, V., Bakola-Christianopoulou, M., Akrivos, P., Kojic, V., & Bogdanovic, G. (2006). Synthesis, structure and in vitro biological activity of new hydroxy-naphthoquinonato triorganotin compounds. Synthesis and Reactivity in Inorganic, Metal-Organic, and Nano-Metal Chemistry, 36, 765–775.10.1080/15533170601028298
- Wark, T. A., & Stephan, D. W. (1990). Early/late heterobimetallic complexes: Syntheses and spectral and structural studies of thiolato-bridged titanium/copper and vanadium/copper complexes. Inorganic Chemistry, 29, 1731–1736.10.1021/ic00334a028
- Zhang, X., Song, H., Li, Q., Liu, X. L., & Tang, L. (2007). Synthesis, structure and biological activity of organotin derivatives with pyridylmethylthiobenzoic acid. Polyhedron, 26, 3743–3749.10.1016/j.poly.2007.04.029
- Zhang, Y., Wang, X., & Ding, L. (2011). Synthesis and DNA binding studies of Mg(II) complex of Schiff base derived from vanillin and l-tryptophan. Nucleosides, Nucleotides and Nucleic Acids, 30, 49–62.10.1080/15257770.2010.543117