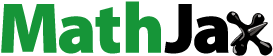
Abstract
An investigation of oxidative–reductive mechanisms of pharmaceutically important molecules gives us information about the metabolic fact of targeted drug. As compared to recent ongoing, time-consuming and costly techniques, there is an urgent needing for development of a sensitive technique, which can help easy understanding of these pathways. Therefore, in the present work, an effective, low-cost and time-saving technique to investigate the reaction mechanism of furosemide in aqueous acid medium is attempted. Furosemide undergoes two-proton and two-electron transfer reaction. The product obtained was analysed by UV spectra. It was found that the chemical oxidation and electrochemical oxidation of furosemide follows two different pathways. In addition, an effective technique has been developed to determine furosemide in its trace level. Good recoveries and low detection limit accomplished the magnitude of the proposed method. The proposed method was adopted for furosemide determination in human urine and pharmaceutical samples.
Public Interest Statement
Furosemide, an anthranillic acid derivative is widely used as a strong diuretic, for both adults and children. Several serious side affects are associated with overdosage of furosemide. Hence, determination of furosemide in its trace level is an important aspect of present day. In the present work, a highly sensitive and selective method was developed for trace determination of furosemide. Physiochemical parameters such as electrode process, number of electrons transferred, heterogeneous rate constant, effect of some excipients, etc were calculated. A probable reaction mechanism was proposed. Differential pulse voltammetric method developed was used for pharmaceutical and urine sample analysis.
1. Introduction
Furosemide, chemically known as 4-chloro-N-furfuryl-5-sulfamoylanthranilic acid, is an anthranillic acid derivative, which is been used as a strong diuretic (Rankin, Williams, & Lemke, Citation2002). Both adults and childrens are treated with furosemide available under brand name Lasix either orally or by intravenous. It has been used in the treatment of edema associated with renal impairment (Laura & Ronald, Citation1990), nephrotic syndrome, hypertension, heart failure (Alon, Scagliotti, & Garola, Citation1994) hepatic cirrhosis . Furosemide has poor aqueous solubility and its efficacy is due to the ability to inhibit NaCl/KCl/2Cl transporter in the ascending limb of the loop of Henle (Shetti, Sampangi, Hegde, & Nandibewoor, Citation2009). Furosemide is always associated with numerous side effects such as urination, thirst, muscle cramps, diaurehea and constipation. Serious side effect include dehydration, dark urine, clay coloured stools, nausea, vomiting, fever, jaundices, electrolyte abnormalities and rapid weight loss. In view of frequent and widespread usage of furosemide in neonatal clinics and the adverse effects associated with its usage, determination of furosemide and explaining the reaction mechanism is the need of the hour.
A number of methods have been reported which include liquid chromatography with spectrophotometric (Carda-Broch, Esteve-Romero, & Garcı́a-Alvarez-Coque, Citation2000; Ferraro, Castellano, & Kaufman, Citation2001; Nava-Ocampo, Velázquez-Armenta, Reyes-Pérez, Ramirez-Lopez, & Ponce-Monter, Citation1999), spectrofluorimetric detection (Ioannou, Andrikopoulou, Glynou, Tzompanaki, & Rusakova, Citation1998; Luis et al., Citation2004; Vree, van den Biggelaar-Martea, & Verwey-van Wissen, Citation1994), chemiluminescent (Rao, Zhang, Luo, & Baeyens, Citation1999) and micellar electrokinetic chromatographic methods (Luis et al., Citation2002), electrochemical methods (Baranowska, Markowski, Gerie, & Baranowski, Citation2008; Barroso, Alonso, & Jiménez, Citation1995). Electrochemical methods have proved to be sensitive for the determination of organic molecules, including drugs and related molecules in pharmaceutical dosages forms and biological fluids and their oxidizable property (Hegde, Shetti, & Nandibewoor, Citation2009; Kalanur, Jaldappagari, & Balakrishnan, Citation2011). Carbon electrodes, especially paste electrodes, are widely used in the electrochemical investigations because of their low background current, wide potential windows, chemical inertness, low cost, and suitability for detection of various organic and biological compounds (Genxi & Peng, Citation2013; Shetti, Malode, & Nandibewoor, Citation2012). In addition, easy fabrication of the electrode has been achieved by incorporating different substances during paste preparation. This results in so-called modified electrode with desired composition and predetermined properties (Gholivand & Mohammadi-Behzad, Citation2014; Khoobi, Ghoreishi, Masoum, & Behpour, Citation2013; Mokhtari, Karimi-Maleh, Ensafi, & Beitollahi, Citation2012).
As compared with literature, carbon paste electrode (CPE) has been applied for the first time to elucidate reaction mechanism of furosemide. In the present work, the centre of attraction is to know the reaction mechanism of furosemide and optimized electrochemical method for furosemide. Proposed is an electrochemical reaction mechanism and applicability for determination in pharmaceutical sample and human urine sample. Further, comparison with other reported methods revealed the superiority of the proposed method.
2. Experimental
2.1. Apparatus and chemicals
Electrochemical analyzer (CHI Company, D630, USA) was used to study the electrochemical deeds of the drug under investigation at an ambient temperature of 25 ± 0.1°C. A three-electrode system consisting of CPE as working electrode, platinum wire as counter electrode and Ag/AgCl (3 M KCl) as reference electrode in a 10 ml single compartment was used for the investigations. The phosphate buffer solutions ranging 3.0–11.2 pH (I = 0.2) were prepared according to literature (Christian & Purdy, Citation1962) and pH of the solutions were measured by pH meter (Elico Ltd., LI120, India). Furosemide (Sigma-Aldrich, USA) was used to prepare 1.0 mM stock solution in ethanol. Double-distilled water, analytical-grade chemicals and reagents without further purification were used throughout the experiments.
2.2. Preparation of electrode
One gm of graphite powder and 0.5 ml paraffin oil was hand-mixed and homogenized in a small agate mortar. Cavity of PTFE (polytetrafluoro ethylene) tube was firmly packed with the paste. On the weighing paper, the electrode surface was smoothened and rinsed with distilled water. Carefully removing the older surface and then pressing out the inner paste out, the new portion of the electrode surface was exposed. Prior to use the CPE was activated in phosphate buffer solution of pH 5 by cyclic voltammetric sweeps between 0.4– 1.4 V with a scan rate 50 mVS−1 (Malode & Nandibewoor, Citation2013). Randles–Sevcik formula can be used to calculate the electro-active area of the electrode using cyclic voltammetric technique and K3Fe (CN)6 1.0 mM as a probe at different scan rates in 0.1 M KCl as supporting electrolyte. At T = 298 K and for a reversible process the equation is as follows: (Malode, Shetti, & Nandibewoor, Citation2012a).(1)
(1)
In Equation (1) for 1.0 mM K3Fe (CN)6 and 0.1 M KCl as supporting electrolyte: Ip refers to the anodic peak current, n is the number of electron transferred during the electrode reaction = 1. A0 is the surface area of the electrode, DR is the diffusion coefficient = 7.6 × 10−6 cm2 s−1, υ is the scan rate and C0 is the concentration of K3Fe (CN)6. From the slope of the plot of Ip vs. υ1/2, the area of the electrode surface was calculated to be 0.036 ± 0.0014 cm2.
3. Results and discussion
3.1. Cyclic voltammetric behaviour of furosemide
Electrochemical behaviour studies of 0.1 mM furosemide was studied using cyclic voltammetric method. Since the pH of the solution affects the electrode reaction, optimization of solution pH was carried out using phosphate buffer solution ranging between pH 3.0 and 11.2 (I = 0.2 M). In the potential range, 0.6–1.6 V (Figure ) two-oxidation peak, Pa1 and Pa2 were observed for pH 3–6. On increasing the pH above 6, Pa1 diminished and finally disappeared. On reversing the scan rate, there was no peak corresponding to Pa1 and Pa2 indicating the irreversibility of the electrode reaction. Further, with increase in solutions pH, the peak potential (Figure (A)) shifted to values that are more negative. In addition, the plot of peak current as a function of pH (Figure (B)) shows that the maximum peak current was been obtained at pH 5. Hence, pH 5 was been considered as optimum pH for further studies.
Figure 1. Voltammetric behaviour of 0.1 mM FUR at carbon paste electrode at scan rate 50 mV s−1: (a) pH 3, (b) pH 4, (c) pH 5, (d) pH 6, (e) pH 7, (f) pH 8, (g) pH 9, (h) pH 10, (i) pH 11.2.
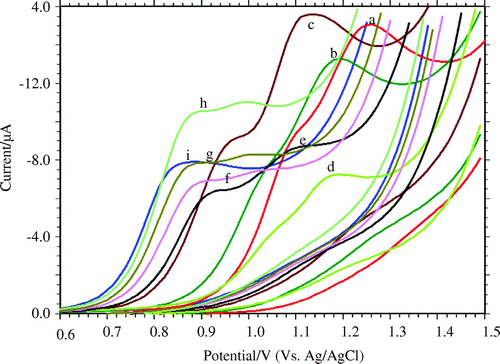
Voltammogram comparison of blank and 0.1 mM furosemide in phosphate buffer (pH 5; I = 0.2 M) shows two well-defined peaks at 0.95 and 1.15 V, respectively (Figure ).
Figure 3. Voltammetric behaviour at carbon paste electrode a: blank solution b: 1 mM FUR at scan rate 50 mV s−1 pH 5.
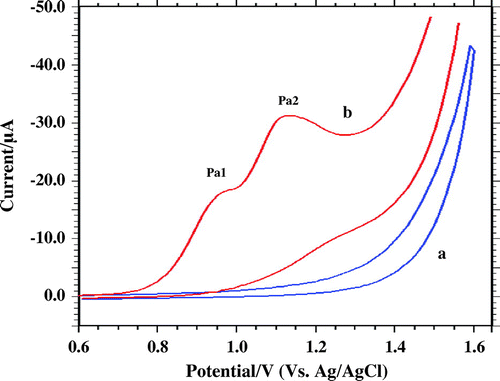
To evaluate the electrode process of furosemide cyclic voltammetric technique, varying scan rates in the range of 10.0–700.0 m V s−1 were used (Figure ). Increase in scan rate increased the peak current with peak potential shifting towards value that is more positive. There is a good linear relationship between peak current and scan rate represented by equation: Ip 1 (μA) = 41.168υ (V s−1) + 4.822; R2 = 0.9932 and Ip 2 (μA) = 23.728υ (V s−1) + 2.2883; R2 = 0.991 (Figure ).
Figure 4. Effect of scan rate on Ip (Peak current). Voltammogram recorded at Scan rate (a) 0.01, (b) 0.05, (c) 0.08, (d) 0.1, (e) 0.12, (f) 0.15, (g) 0.2, (h) 0.25, (i) 0.30, (j) 0.35, (k) 0.40, (l) 0.5, (m) 0.7 V s−1.
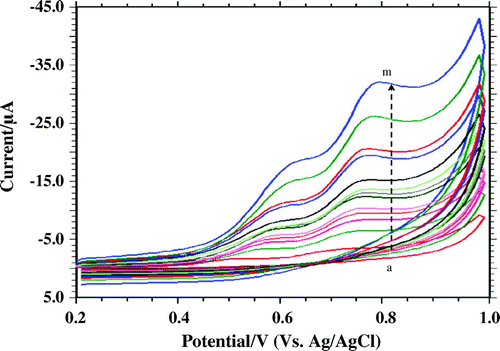
A close theoretical value of 0.5 for diffusion-controlled process was been obtained from the slope of the plot, log Ip vs. log υ (Figure ). The expression was expressed as follows log Ip1 = 0.5791 log υ + 1.2919 R2 = 0.9844 and log Ip2 = 0.5319 log υ + 1.5297 R2 = 0.9825. The irreversibility of the electrode reaction was further affirmed from the plot of peak potential and logarithm of scan rate where Ep value shifted to more positive value with increase in scan rate. The relationship between peak potential and logarithm of scan rate is as follows: Ep 1 (V) = 0.0633 log υ (V s−1) + 1.0449; R2 = 0.9965 and Ep 2 (V) = 0.057 log υ (V s−1) + 1.2264; R2 = 0.9923 (Figure ). Considering the strong adsorption of the reactant and irreversibility of the electrode process, Laviron equation is used (Laviron, Citation1979):
Figure 8. Differential pulse voltammetry for increasing concentration of FUR: (a) 0.1, (b) 0.2, (c) 0.3, (d) 0.4, (e) 0.5, (f) 0.6, (g) 0.7, (h) 0.9, (i) 1.0, (j) 2.0 mM.
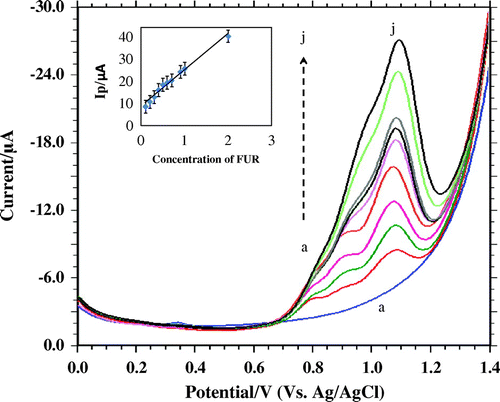
Number of electrons transferred (n) is calculated easily from the above equation, considering the slope of plot Ep1 vs. log υ and Ep2 vs. log υ., αn was calculated as 0.934 and 1.03 for Ep1 vs. log υ and Ep2 vs. log υ with a slope of 0.0633 and 0.057, respectively. According to Bard and Faulkner (Citation2004),
where Ep/2 is the potential where the current is at half the peak value. Therefore, from this we get the value of α to be 0.75. Hence, the number of electrons (n) transferred in the electro oxidation of furosemide was calculated to be 1.2 ≈ 1 for first peak and 1.3 ≈ 1 for second peak. Therefore, the total number of electrons is 2 for the electrode reaction of furosemide. From the intercept of Ep vs. log υ plot and E0 value obtained from intercept of Ep vs. υ curve by extrapolating to vertical axis at υ = 0, k0 was obtained to be 3.5 × 103 for peak 1 and 3.23 × 103 for second peak, respectively.
3.2. Reaction mechanism
Comparison of the proposed mechanism with other reported methods showed different pathway for oxidation of furosemide. The mechanism of oxidation of furosemide by diperiodatoargentate (III) osmium (VIII) catalyzed in alkaline medium involved Mannic-like reaction. The product formed was identified as 2-(4-carboxy-2-oxo-but-3-enylamino)-4-chloro-5-sulfamoyl-benzoic acid by its IR spectrum (Malode et al., Citation2012b). The enzymatic and chemical oxidation of furosemide as studied and reported (Chen & Burka, Citation2007) also followed the Mannic-like reaction forming a stable pyridinium salt. Whereas the photosensitized oxidation of furosemide showed strongly solvent-dependent mode of reaction involving charge transfer from amino group to singlet oxygen (Zanocco, Günther, LEMP, de la Fuente, & Pizarro, Citation1998). The electrochemical reaction mechanism included transfer of two protons and two electrons. The probable reaction mechanism is elucidated in Scheme .
4. Analytical application
4.1. Calibration curve
Differential pulse voltammetric method was used to construct a calibration plot. The peak current (Ip1 and Ip2) followed the linearity in the range of 5.0 × 10−7 to 10.0 × 10−6 M (Figure ). Limit of detection (LOD) and limit of quantification (LOQ) were been calculated based on the peak current using the following equation shown below (Bukkitgar, Shetti, Kulkarni, & Nandibewoor, Citation2015).
The LOD and LOQ values were calculated to be 4.47 × 10−9 M and 1.4 × 10−8 M, respectively. The LOD and LOQ values calculated by the present method are better compared to the early reported methods (Table ).
Table 1. Comparison of LOD with reported methods
Further, the calibration plot obtained was used for quantitative analysis of human urine samples and pharmaceutical samples. For the quantitative analysis of pharmaceutical samples, commercially available tablets (Lasix) were ground to powder and dissolved in ethanol. The concentration was adjusted to fall in the range of calibration plot. The recoveries in different samples were been found to lie in the range 96.2–100.5 with R.S.D. of 2.03%. The results obtained were in good agreement with label marked (Table ).
Table 2. Analysis of FUR in tablets by DPV and recovery studies
Since the pharmaceutical samples contain additional exepients, effect of some exepients were studied using differential pulse voltammetric method. One-hundred folds of excipients like citric acid, dextrose, glucose, gum acacia, lactic acid, oxalic acid, starch and sucrose did not interfere with the voltammetric signal of FUR. Thus, the procedures were able to assay furosemide in the presence of excipients, and hence it can be considered specific (Table ).
Table 3. Application of DPV for the determination of FUR in spiked human urine
As for the urine sample, the drug-free urine was spiked with known amount of drug. Standard addition method was used to prepare the sample solution (Bukkitgar, Shetti, Kulkarni, & Doddamani, Citation2016) . Good recovery was obtained from the results indicating the applicability of the proposed method for real sample analysis. The recovery was in the range 97.1–100.6% and the R.S.D. was 1.3% (Table ).
Table 4. Influence of potential interferents on the voltammetric response of 1.0 × 10−5 M FUR
5. Conclusion
Electrochemical investigation of furosemide on CPE was successfully demonstrated in the present work. The proposed reaction mechanism involved transfer of two protons and electrons, respectively. Further, using cyclic voltammetric method, the effect of pH of electrolyte solution and scan rate was investigated. Plots obtained from these studies were used to calculate heterogeneous rate constant, diffusion coefficient, number of electrons transferred. Further, it was also possible to understand that the electrode reaction is diffusion controller and irreversible. In addition to this, a differential pulse voltammetric method has been developed for analytical application of the proposed method. The LOD and LOQ calculated had superiority compared to early reported methods.
Additional information
Funding
Notes on contributors
Shikandar D. Bukkitgar
Shikandar D. Bukkitgar is a research student in Department of chemistry, K.L.E. Society’s K.L.E. Institute of Technology, Hubli, India. He did his B Sc degree in Chemistry from Karnatak University, Dharwad, 2006; M Sc degree in biochemistry from Kuvempu University, Shimoga. His research interests are electroanalysis, nanomaterials applications.
Nagaraj P. Shetti
Nagaraj P. Shetti is an associate professor and head in Department of chemistry, K.L.E. Society’s K.L.E. Institute of Technology, Hubli, India. He did his B Sc degree in Chemistry from Karnatak University, Dharwad, 2003; M Sc degree in Chemistry from Karnatak University, Dharwad, Ph D from Karnatak University, Dharwad, 2010, and postdoctoral research from Korea University, Republic of Korea, 2011–2012. His research interests are liquid-phase oxidation reactions, kinetics and catalysis, nanomaterial applications, electroanalytical chemistry, and biosensors. He has published more than 40 articles in reputed journals.
References
- Alon, U. S., Scagliotti, D., & Garola, R. E. (1994). Nephrocalcinosis and nephrolithiasis in infants with congestive heart failure treated with furosemide. The Journal of Pediatrics, 125, 149–151.10.1016/S0022-3476(94)70143-1
- Baranowska, I., Markowski, P., Gerie, A., & Baranowski, J. (2008). Determination of selected drugs in human urine by differential pulse voltammetry technique. Bioelectrochemistry, 73, 5–10.
- Bard, A. J., & Faulkner, L. R. (2004). Elecrochemical methods fundamentals and applications (2nd ed.). New York, NY: Wiley.
- Barroso, M. B., Alonso, R. M., & Jiménez, R. M. (1995). Electrochemical determination of the loop diuretics piretanide and furosemide in pharmaceutical formulations and urine. Analytica Chimica Acta, 305, 332–339.10.1016/0003-2670(94)00373-T
- Bukkitgar, S. D., Shetti, N. P., Kulkarni, R. M., & Doddamani, M. R. (2016). Electro oxidation of nimesulide at 5% barium-doped zinc oxide nanoparticle modified glassy carbon electrode. Journal of electroanalytical chemistry, 762, 37–42.
- Bukkitgar, S. D., Shetti, N. P., Kulkarni, R. M., & Nandibewoor, S. T. (2015). Electro-sensing base for mefenamic acid on a 5% barium-doped zinc oxide nanoparticle modified electrode and its analytical application. RSC Advances, 5, 104891–104899.10.1039/C5RA22581G
- Carda-Broch, S., Esteve-Romero, J., & Garcı́a-Alvarez-Coque, M. C. (2000). Furosemide assay in pharmaceuticals by Micellar liquid chromatography: Study of the stability of the drug. Journal of Pharmaceutical and Biomedical Analysis, 23, 803–817.10.1016/S0731-7085(00)00378-2
- Chen, L. J., & Burka, L. T. (2007). Chemical and enzymatic oxidation of furosemide: Formation of pyridinium salts. Chemical Research in Toxicology, 20, 1741–1744.10.1021/tx700262z
- Christian, G. D., & Purdy, W. C. (1962). The residual current in orthophosphate medium. Journal of Electroanalytical Chemistry, 3, 363–367.
- Ferraro, M. C. F., Castellano, P. M., & Kaufman, T. S. (2001). A spectrophotometric-partial least squares (PLS-1) method for the simultaneous determination of furosemide and amiloride hydrochloride in pharmaceutical formulations. Journal of Pharmaceutical and Biomedical Analysis, 26, 443–451.10.1016/S0731-7085(01)00432-0
- Genxi, L., & Peng, M. (2013). Electrochemical analysis of proteins and cells. Berlin Heidelberg: Springer.
- Gholivand, M. B., & Mohammadi-Behzad, L. (2014). Fabrication of a highly sensitive sumatriptan sensor based on ultrasonic-electrodeposition of Pt nanoparticles on the ZrO2 nanoparticles modified carbon paste electrode. Journal of Electroanalytical Chemistry, 712, 33–39.10.1016/j.jelechem.2013.10.024
- Hegde, R. N., Shetti, N. P., & Nandibewoor, S. T. (2009). Electro-oxidation and determination of trazodone at multi-walled carbon nanotube-modified glassy carbon electrode. Talanta, 79, 361–368.10.1016/j.talanta.2009.03.064
- Heidarimoghadam, R., & Farmany, A. (2016). Rapid determination of furosemide in drug and blood plasma of wrestlers by a carboxyl-MWCNT sensor. Materials Science and Engineering: C, 58, 1242–1245.10.1016/j.msec.2015.09.062
- Ioannou, P. C., Andrikopoulou, D. A., Glynou, K. M., Tzompanaki, G. M., & Rusakova, N. V. (1998). Spectrofluorimetric determination of anthranilic acid derivatives based on terbium sensitized fluorescence. The Analyst, 123, 2839–2843.10.1039/a806093b
- Kalanur, S. S., Jaldappagari, Seetharamappa, & Balakrishnan, S. (2011). Enhanced electrochemical response of carbamazepine at a nano-structured sensing film of fullerene-C60 and its analytical applications. Electrochimica Acta, 56, 5295–5301.10.1016/j.electacta.2010.08.071
- Khoobi, A., Ghoreishi, S. M., Masoum, S., & Behpour, M. (2013). Multivariate curve resolution-alternating least squares assisted by voltammetry for simultaneous determination of betaxolol and atenolol using carbon nanotube paste electrode. Bioelectrochemistry, 94, 100–107.10.1016/j.bioelechem.2013.04.002
- Laviron, E. (1979). General expression of the linear potential sweep voltammogram in the case of diffusionless electrochemical systems. Journal of Electroanalytical Chemistry and Interfacial Electrochemistry, 101, 19–28.10.1016/S0022-0728(79)80075-3
- Laura, L. B. P., & Ronald, D. S. (1990). Furosemide (frusemide) a pharmacokinetic/pharmacodynamic review (part I). Clinical Pharmacokinetics, 18, 381–408.
- Luis, M. L., Corujedo, S., Blanco, D., Fraga, J. M. G., Jimenez, A. I., Jimenez, F., & Arias, J. J. (2002). Micellar electrokinetic capillary chromatography analysis of diuretics in pharmaceutical formulations. Talanta, 57, 223–231.10.1016/S0039-9140(02)00018-8
- Luis, M. L., Fraga, J. M. G., Jimenez, A. I., Jimenez, F., Hernandez, O., & Arias, J. J. (2004). Application of PLS regression to fluorimetric data for the determination of furosemide and triamterene in pharmaceutical preparations and triamterene in urine. Talanta, 62, 307–316.10.1016/j.talanta.2003.07.010
- Malode, S. J., & Nandibewoor, S. T. (2013). Electrochemical oxidation and determination of nimesulide using a carbon paste electrode. Zeitschrift für Physikalische Chemie, 227, 73–88.10.1524/zpch.2012.0232
- Malode, S. J., Shetti, N. P., & Nandibewoor, S. T. (2012a) Voltammetric behaviour of theophylline and its determination at multi-wall carbon nanotube paste electrode. Colloids and Surfaces B: Biointerfaces, 97, 1–6
- Malode, S. J., Shetti, N. P., & Nandibewoor, S. T. (2012b). Mechanistic aspects of Os(VIII) catalysed oxidation of loop diuretic drug furosemide by Ag(III) periodate complex in aqueous alkaline medium. Journal of Chemical Sciences, 124, 421–430.10.1007/s12039-011-0182-4
- Mokhtari, A., Karimi-Maleh, H., Ensafi, A. A., & Beitollahi, H. (2012). Application of modified multiwall carbon nanotubes paste electrode for simultaneous voltammetric determination of morphine and diclofenac in biological and pharmaceutical samples. Sensors and Actuators B: Chemical, 169, 96–105.10.1016/j.snb.2012.03.059
- Nava-Ocampo, A. A., Velázquez-Armenta, E. Y., Reyes-Pérez, H., Ramirez-Lopez, E., & Ponce-Monter, H. (1999). Simplified method to quantify furosemide in urine by high-performance liquid chromatography and ultraviolet detection. Journal of Chromatography B: Biomedical Sciences and Applications, 730, 49–54.10.1016/S0378-4347(99)00176-0
- Rankin, G. O., Williams, D. A., & Lemke, T. L. (2002). Foye’s principles of medicinal chemistry (5th ed., p. 518). Baltimore, MD: Lippicott Williams.
- Rao, Y., Zhang, X. R., Luo, G., & Baeyens, W. R. G. (1999). Chemiluminescence flow-injection determination of furosemide based on a rhodamine 6G sensitized cerium(IV) method. Analytica Chimica Acta, 396, 273–277.10.1016/S0003-2670(99)00425-0
- Semaan, F. S., Pinto, E. M., Cavalheiro, Éder T. G., Brett C. M. A. (2008). A graphite-polyurethane composite electrode for the analysis of furosemide. Electroanalysis, 20, 2287–2293.10.1002/elan.v20:21
- Shetti, N. P., Malode, S. J., & Nandibewoor, S. T. (2012). Electrochemical behavior of an antiviral drug acyclovir at fullerene-C60-modified glassy carbon electrode. Bioelectrochemistry, 88, 76–83.10.1016/j.bioelechem.2012.06.004
- Shetti, N. P., Sampangi, L. V., Hegde, R. N., & Nandibewoor, S. T. (2009). Electrochemical oxidation of loop diuretic furosemide at gold electrode and its analytical applications. International Journal of Electrochemical Science, 4, 104–121.
- Vree, T. B., van den Biggelaar-Martea, M. V., & Verwey-van Wissen, C. P. W. G. M. (1994). Determination of furosemide with its acyl glucuronide in human plasma and urine by means of direct gradient high-performance liquid chromatographic analysis with fluorescence detection preliminary pharmacokinetics and effect of probenecid. Journal of Chromatography B: Biomedical Sciences and Applications, 655, 53–62.10.1016/0378-4347(94)00093-X
- Zanocco, A. L., Günther, G. S., LEMP, E. M., de la Fuente, J. R., & Pizarro, N. U. (1998). Kinetics and mechanism of the photosensitized oxidation of furosemide. Photochemistry and Photobiology, 68, 487–493.10.1111/php.1998.68.issue-4