Abstract
The eco-friendly synthesis of imines was reported in this work using water as solvent under microwave irradiation. This protocol achieved excellent yields in short time. Water plays an important role in this reaction, in contrast with some previous studies.
Keywords:
Public Interest Statement
Nowadays, cheap, nontoxic, and safe source is a fundamental point in the production of materials, this results in the reduction of environmental impacts. In the context, our research has been developing new compounds from these points, and good results have already been achieved.
1. Introduction
The imine group is present in several compounds that have demonstrated great relevance due to their biological properties, such as antibacterial, antifungal properties, and their inhibition of the enzyme DNA-topoisomerase I, an important target against cancer (Asadi, Asadi, Torabi, & Lotfi, Citation2012; Ganguly, Chakraborty, Banerjee, & Choudhuri, Citation2014; Jhaumeer-Laulloo, Gupta Bhowon, Mungur, Fawzi Mahomoodally, & Hussein Subratty, Citation2012; Lee et al., Citation2014; Qin, Long, Panunzio, & Biondi, Citation2013). In the field of new materials, imines, and their metal clusters, are present in the formation of thermostable polymers, as synthetic intermediates, corrosion inhibitors, and high-performance catalysts (Chen, Xiang, & Feng, Citation2012; Coulthard, Unsworth, & Taylor, Citation2015; Dogan & Kaya, Citation2013; Doi, Kawai, Murayama, Kashida, & Asauna, Citation2016; Kielland, Escudero-Adán, Martínez Belmonte, & Kleij, Citation2013; Seifzdeh, Basharnavaz, & Bezaatpour, Citation2014; Zarei, Citation2014). The synthesis of imines is performed with aldehydes or ketones and a primary amine and an apparatus to remove water, such as a Dean Stark trap, or a dehydration agent to increase the yield. Recently, the literature registered the use of catalysts, such as montmorillonite, ZnCl2, and TiCl4 (Qin et al., Citation2013). Unfortunately, the synthesis of Schiff bases is not facile and in some cases extremely slow, such as when there are withdrawing groups linked to benzylamines. Previously, 2-N-amine-3-N-(phenylmethylene)-pyridine was prepared from benzaldehyde and 2,3-diaminepyridine in THF reflux for 4 h, more 18 h of stirring at room temperature, using molecular sieves (Khanna, Weier, Lentz, Swenton, & Lankin, Citation1995). Recently, the same compound was obtained by stirring in ethanol at room temperature for 48 h (Jhaumeer-Laulloo et al., Citation2012). The long reaction time and the use of THF as a solvent are not part of 12 green rules. Our research group believes in the words written by Deligeorgiev et al. (Citation2010), “Green Chemistry is not a new branch of science. It is a new philosophical approach that, through the introduction and expansion of its principles could lead to a substantial development in chemistry, the chemical industry and environmental protection.” In this context, water is an eco-friendly solvent because it has the following properties: (a) cheap; (b) nontoxic; (c) non-flammable; (d) safe; and (e) the products are usually isolated by filtration (Esteves-Sousa et al., Citation2012; Panda & Jain, Citation2012; Rodrigues-Santos & Echevarria, Citation2007, Citation2011). The involvement of water in the formation of imine has caused controversy among authors. Saggiomo and Lüning (Citation2009) stated important considerations about this matter. The research group indicated that water did not act as a catalyst or solvent, and the imines formed during or after workup. However, Cordes and Jencks (Citation1962) and Kommi, Kumar, Chakraborti, Chebolu, and Chadraborti (Citation2013) published an article suggesting that the water molecules participated in the formation of the imine. Our work describes an efficient synthesis of imines, employing water as solvent under microwave irradiation, corroborating with the Codes and Kommi et al.
2. Results and discussion
To find the best reaction conditions, the reaction of compound 1a and 2a was performed under several conditions, entry 3–8 (Table ).
Table 1. Reaction times, yields, temperature and method for the synthesis of 2-N-amine-3-N-(2-hydroxy-phenylmethylene)-5-bromo-pyridine 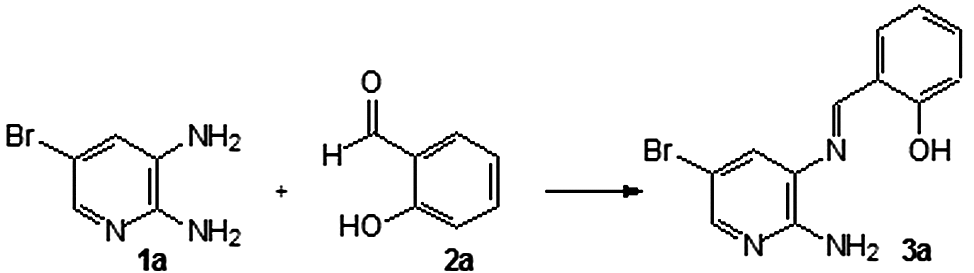
As it can been observed in Table , it was not possible to obtain good yields from toluene when using alternative methods, such as ultrasound or microwave irradiation. However, the use of water was successful for this reaction. After 1-h reflux, a yellow solid was obtained in 98% yield. Ultrasound irradiation in water also achieved good yield (96%), but the best method was obtained by microwave irradiation in water (98%), it decreased the reaction time by sixfold compared to traditional reflux conditions. It is important to report that no oil pump vacuum was used to help in the formation of the product, as indicated by Saggiomo and Lüning (Citation2009). The solid was washed with water and dried. Thus, microwave irradiation in water was selected to obtain the 3a–n derivatives. Good results (54–95%) were obtained, as observed in Table , even when an electron withdrawing group was present. In addition, the reaction time was significantly reduced to 7–10 min, with the exception of compound 3n (30 min).
Table 2. Reaction times and yields for compounds 3a–n obtained using water and MW irradiation ![]()
The attack of the amine group (5-bromo-2,3-diaminepyridine) could produce the isomer A (3-amine, E-iosmer) and/or isomer B (2-amine, E-isomer) (Figure ).
After analysis of the 1H NMR chemical shifts, NOESY spectroscopy and crystal structures obtained by X-ray diffraction, only the E-isomer A was observed; therefore, this reaction was regioselective, with exception of compound 3m, which presented both isomers (A and B) due to the electron strength-withdrawing effect of the nitro group (σp = 0.78) para to the aldehyde. The isomers were observed by TLC and were separated by fractioned recrystallization. Due to the electronic effects, 1H NMR spectra showed the differences of the NH2 and H-imine groups for each isomer. Isomer A had an NH2 (H-7) group downfield (δ = 6.41) and an H-imine (H-9) group upfield (δ = 8.91). The opposite was observed in the NH2 and H-imine groups of isomer B (NH2, δ = 6.03 and H-imine, δ = 9.33) (see ESI). The 1H NOESY spectra in DMSO-d6 revealed the correlation between the H-imine and H-4 for isomer A, characterizing conformer A. The absence of a correlation between the NH2 (H-7) and H-imine (H-9) indicated the possibility of conformer A for isomer B (Figure ). In the 1H NOESY spectra of the isomers, there were no correlations between the hydrogen aromatic rings, which indicated that there was not formation of the Z-isomer. These data were corroborated by the crystal structures (see Supplementary data).
Figure 2. NOESY spectra of 3m isomer A and 3m isomer B and their possible conformations (for the best visualization of the content, see electronic supplementary information, page S20 and S22).
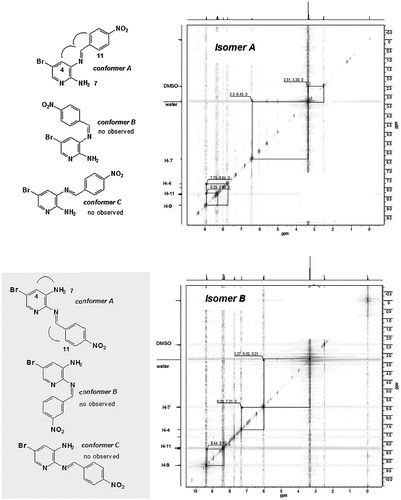
Eight crystal structures were obtained in this study (3b, 3b′, 3c, 3e, 3f, 3g, 3h, and 3l); (see Supplementary data) the structures 3b and 3b′ represent the same compound but with different conformations (Figure ). Compound 3b contained fewer π–π conjugation effects between the A and B rings; this observation is based on C6–C7, C5–N3, and the C6=N3 bond lengths and the torsion angles among C5–N3–C6–C7 (see Supplementary Information).
3. Conclusions
The easy, quick, and eco-friendly synthesis of imines under microwave irradiation and water was more efficient when compared to other methods described in the literature (Jhaumeer-Laulloo et al., Citation2012; Khanna et al., Citation1995). The synthesis was regioselective, with the exception of that of compound 3m, due to the reactivity of the 4-nitrobenzaldehyde.
4. Experimental
4.1. Microwave irradiation
A mixture of 2,3-diaminepyridine, 1a–c (2.66 mmol), and aldehyde, 2a–o, (2.66 mmol), and then water (4 mL) was irradiated (CEM-discover-power 0–300 W, magnetron: 2,450 MHz-open vessel system) at 100°C for indicated in Table . The reaction progress was monitored by TLC (EtOAc/hexane, 7:3). After irradiation, the mixture was washed with water (5 × 10 mL) and dried at room temperature. The compounds (3a–n) were recrystallized by ethanol.
4.2. 2-N-amine-3-N-(2-hydroxy-phenylmethylene)-5-bromo-pyridine (3a)
mp 171–172°C; IR (ν/cm−1); 3,449, 3,266, 3,133, 2,906, 1,617, 1,588, 1,482, 1,439, 1,383, 1,240, 1,383, 1,240, 1,203, 1,098, 1,027, 803, 549; 1H NMR (400 MHz, DMSO-d6) δ 6.18 (s, 2H, NH2), 6.97 (m, 2H, H-9/12), 7.4 (t, 1H, J = 8.0 Hz, H-11), 7.61 (s, 1H, H-4), 7.73 (d, 1H, J = 8.0 Hz, H-13), 7.93 (s, 1H, H-6), 8.87 (s, 1H, H-7), 11.81 (s, 1H, OH); DEPTQ (100 MHz, DMSO-d6) δ 105.5, 116.5, 119.3, 120.1, 127.0, 131.5, 132.0, 133.5, 145.8, 153.4, 159.6, 163.4.
4.3. 2-N-amine-3-N-(4-hydroxy-3-methoxy-phenylmethylene)-5-bromo-pyridine (3b)
mp 207–209°C; IR (ν/cm−1) 3,491, 3,384, 2,924, 2,853, 1,593, 1,490, 1,453, 1,426, 1,241, 1,206, 1,028, 815, 734; 1H NMR (400 MHz, DMSO-d6) δ 3.86 (s, 3H), 6.20 (s, 2H, NH2), 6.87 (d, 1H, J = 8.0 Hz, H-9), 7.35 (d, 1H, J = 8.0 Hz, H-13), 7.54 (s, 1H, H-9), 7.66 (s, 1H, H-4), 7.85 (s, 1H, H-6), 8.55 (s, 1H, H-7), 9.80 (s, 1H, OH); DEPTQ (100 MHz, DMSO-d6) δ 55.6, 105.3, 110.8, 115.1, 124.8, 125.0, 127.8, 132.5, 144.8, 148.0, 150.5, 154.3, 160.4.
4.4. 2-N-amine-3-N-(4-hydroxy-phenylmethylene)-5-bromo-pyridine (3c)
mp 220–222°C; IR (ν/cm−1) 3,054, 3,009, 1,607, 1,444, 1,404, 1,352, 1,237, 1,171, 837; 1H NMR (500 MHz, DMSO-d6) δ 6.11 (s, 2H, NH2), 6.85 (d, 2H, J = 5.0 Hz, H-10), 7.54 (s, 1H, H-4), 7.85 (m, 3H, H-9/H9′/H6), 8.57 (s, 1H, H-7), 10.17 (s, 1H, OH); DEPTQ (125 MHz, DMSO-d6) δ 105.4, 115.6, 125.0, 127.4, 131.2, 132.6, 144.8, 154.2, 160.3, 160.9.
4.5. 2-N-amine-3-N-(3,4-dihydroxy-phenylmethylene)-5-bromo-pyridine (3d)
mp 223–225°C; IR (ν/cm−1) 3,461, 3,400, 3,348, 3,033, 1,607, 1,593, 1,447, 1,393, 1,263, 1,147, 756; 1H NMR (500 MHz, DMSO-d6) δ 6.05 (s, 2H, NH2),6.54 (dd, J = 5.0 Hz, 1H, H-5),6.83 (d, 2H, J = 8.0, H-12), 7.24 (d, 1H, J = 8.0 Hz, H-13), 7.28 (d, J = 8.0 Hz, 1H, H-6), 7.43 (s, 1H, H-9), 7.78 (d, J = 4.0 Hz,1H, H-4), 8.43 (s, 1H, H-7), 9.48 (sl, 2H, 2xOH); DEPTQ (125 MHz, DMSO-d6) δ 112.6, 114.7, 115.4, 112.5, 123.0, 128.0, 131.6, 145.1, 145.6, 149.3, 155.1, 159.2.
4.6. 2-N-amine-3-N-(2,4-dimethoxy-pyridinylmehylene)-5-bromo-pyridine (3e)
mp 152–153°C; IR (ν/cm−1) 3,452, 3,263, 3,128, 2,944, 1,593, 1,574, 1,466, 1,322, 1,088, 1,009, 806, 564; 1H NMR (500 MHz, DMSO-d6) δ 3.94 (s, 3H, OCH3), 4.00 (s, 3H, OCH3), 6.18 (s, 2H, NH2), 6.51 (d, 1H, J = 8.0 Hz, H-12), 7.51 (s, 1H, H-4), 7.85 (s, 1H, H-6), 8.49 (d, 1H, J = 8.0 Hz, H-13), 8.70 (s, 1H, H-7); DEPTQ (100 MHz, DMSO-d6) δ 53.6, 53.8, 102.8, 105.3, 110.3, 125.0, 132.6, 140.0, 145.1, 154.2, 153.9, 162.1, 164.9.
4.7. 2-N-amine-3-N-(3,4-methylenedioxy-phenylmethylene)-5-bromo-pyridine (3f)
mp 175–177°C; IR (ν/cm−1) 3,450, 3,269, 3,141, 2,901, 1,617, 1,590, 1,441, 1,242, 1,099, 908, 805, 551; 1H NMR (400 MHz, DMSO-d6) δ 6.12 (s, 2H, H-14), 6.25 (s, 2H, NH2), 7.04 (d, 1H, J = 8.0 Hz, H-12), 7.41 (d, 1H, J = 8.0 Hz, H-13), 7.59 (s, 1H, H-9), 7.73 (s, 1H, H-4), 7.87 (s, 1H, H-7), 8.62 (s, 1H, H-7); DEPTQ (125 MHz, DMSO-d6) δ 101.7, 105.2, 106.6, 108.2, 125.1, 126.6, 130.9, 131.8, 145.7, 148.0, 150.4, 154.4, 159.7.
4.8. 2-N-amine-3-N-(4-methy-phenylmethylene)-5-bromo-pyridine (3g)
mp 156–157°C; IR (ν/cm−1) 3,420, 3,115, 3,018, 2,913, 2,854, 1,600, 1,560, 1,462, 1,373, 1,241, 1,165, 808; 1H NMR (500 MHz, DMSO-d6) δ 2.37 (s, 3H), 6.19 (s, 2H, NH2), 7.31 (d, 2H, J = 8.0 Hz, H-10/10′), 7.60 (d, 1H, J = 2.0 Hz, H-4), 7.88 (d, 1H, J = 2.0 Hz, H-6), 7.90 (d, 2H, J = 8.0 Hz, H-9/9′), 8.68 (s, 1H, H-7); DEPTQ (125 MHz, DMSO-d6) δ 21.2, 105.3, 125.3, 129.1, 129.3, 132.0, 133.4, 141.8, 145.5, 154.3, 160.6.
4.9. 2-N-amine-3-N-(4-methoxy-cinnamylmethylene)-5-bromo-pyridine (3h)
mp 173–174°C; IR (ν/cm−1) 3,457, 3,200, 3,127, 2,919, 2,835, 1,610, 1,592, 1,467, 1,240, 1,020, 760, 571; 1H NMR (500 MHz, DMSO-d6) δ 3.87 (s, 3H, OCH3), 6.05 (s, 2H, NH2), 7.09 (d, 1H, J = 8.0 Hz, H-12), 7.15 (dd, 1H, J = 9.00 and 16.0 Hz, H-8), 7.38 (t, 1H, J = 7.00 Hz, H-14), 7.53 (d, 1H, J = 2.0 Hz, H-4), 7.58 (d, 1H, J = 16.0 Hz, H-9), 7.67 (dd, J = 2.0 and 7.0 Hz, H-15), 7.86 (d, 1H, J = 2.0 Hz, H-6), 8.51 (d, 1H, J = 9.0 Hz, H-7); DEPTQ (125 MHz, DMSO-d6) δ 55.6, 105.5, 111.7, 120.8, 123.6, 125.2, 127.8, 128.8, 131.3, 132.5, 139.5, 145.3, 154.1, 157.3, 163.2.
4.10. 2-N-amine-3-N-(4-trifluoromethyl-phenylmethylene)-5-bromo-pyridine (3i)
mp 108–112°C; IR (ν/cm−1) 3,466, 3,268, 3,134, 1,616, 1,559, 1,468, 1,382, 1,318, 1,160, 1,108, 1,061, 837; 1H NMR (500 MHz, DMSO-d6) δ 6.35 (s, 2H, NH2), 7.70 (d, 1H, H-4), 7.86 (d, 2H, J = 8.0 Hz, H-10), 7.94 (d, 1H, H-6), 8.23 (d, 2H, J = 8.0 Hz, H-10), 8.85 (s, 1H, H-7), DEPTQ (125 MHz, DMSO-d6) δ 105.3, 125.7, 125.9, 129.8, 129.9, 131.2, 139.7, 146.7, 154.6, 159.4.
4.11. 2-N-amine-3-N-(3,4-methylenedioxy-phenylmethylene)-pyridine (3j)
mp 140–141°C; IR (ν/cm−1) 3,471, 3,122, 1,624, 1,603, 1,274, 1,171, 1,150, 745, 728; 1H NMR (500 MHz, DMSO-d6) δ 6.00 (s, 2H, H-14), 6.10 (s, 2H, NH2), 6.53 (dd, 1H, J = 5.0 and 7.5 Hz, H-5), 7.02 (d,1H, J = 7.5 Hz, H-13), 7.34 (dd, 1H, J = 8.0, H-12), 7.40 (dd, 1H, J = 1.0 and 8.0 Hz, H-4), 7.70 (dd, 1H, J = 1.0 Hz, H-9), 7.80 (dd, 1H, J = 1.0 and 5.0 Hz, H-6), 8.56 (s, 1H, H-7); DEPTQ (125 MHz, DMSO-d6) δ 101.6, 106.5, 108.2, 112.4, 122.9, 126.0, 130.6, 131.2, 145.7, 148.0, 150.1, 155.4, 158.1.
4.12. 2-N-amine-3-N-(3,4-dihydroxy-phenylmethylene)-5-bromo-pyridine (3k)
mp 200–201°C; IR (ν/cm−1) 3,430, 3,347, 3,074, 1,582, 1,533, 1,465, 1,445, 1,359, 1,290, 1,271, 1,171, 1,148, 914, 886, 805, 555; 1H NMR (400 MHz, DMSO-d6) δ 6.05 (s, 1H, J = 8.0 Hz, H-13), 6.83 (s, 1H, J = 8.0 Hz, H-12), 7.27 (d, 1H, J = 8.0 Hz, H-13), 7.42 (s, 1H, H-9), 7.51 (s, 1H, H-4), 7.84 (s, 1H, H-6), 8.48 (s, 1H, H-7), DEPTQ (100 MHz, DMSO-d6) δ 105.5, 115.0, 115.4, 122.8, 125.1, 127.8, 132.8, 144.7, 145.5, 149.6, 154.1, 160.7.
4.13. 2-N-amine-3-N-(phenylmethylene)-5-bromo-pyridine (3l)
mp 120–122°C; IR (ν/cm−1) 3,461, 3,263, 3,140, 2,995, 1,607, 1,570, 1,464, 1,401, 1,373, 1,244, 1,161, 690; 1H NMR (500 MHz, DMSO-d6) δ 6.21 (s, 2H, NH2), 7.48–7.52 (m, 3H, H-10/10′/11), 7.63 (d, 1H, J = 4.0 Hz, H-4), 7.90 (d, 1H, J = 2.0 Hz, H-6), 8.01 (d, 2H, J = 2.0 and 8.0 Hz, H-9/H9′), 8.74 (s, 1H, H-7), DEPQ (100 MHz, DMSO-d6), 106.4, 119.0, 128.6, 129.3, 130.8, 131.9, 132.8, 134.0, 147.4, 167.3.
4.14. 2-N-amine-3-N-(4-nitro-phenylmethylene)-5-bromo-pyridine (3m-isomer A)
mp 212–214°C; IR (ν/cm−1) 3,491, 3,385, 1,591, 1,503, 1,456, 1,332, 1,244, 1,101, 913, 845, 740, 683, 567; 1H NMR (400 MHz, DMSO-d6) δ 6.41 (s, 2H, NH2), 7.75 (d, 1H, J = 4.0 Hz, H-4), 7.95 (d, 1H, J = 4.0 Hz, H-6), 8.28 (d, 2H, J = 8.0, H-9/9′), 8.33 (d, 2H, J = 10.0 Hz, H-10/10′), 8.91 (s, 1H, H-7), DEPTQ (100 MHz, DMSO-d6) δ 114.1, 124.1, 124.6, 128.3, 130.0, 131.1, 135.1, 145.6, 148.8, 152.2.
4.15. 2-N-(4-nitro-phenylmethylene)-3-N-amine-5-bromo-pyridine (3m-isomer B)
mp 195–197°C; IR (ν/cm−1) 3,389, 3,053, 2,968, 1,674, 1,611, 15,602, 1,449, 1,279, 1,259, 1,150, 1,150, 1,029, 751, 563, 505; 1H NMR (500 MHz, DMSO-d6) δ 6.01 (s, 2H, NH2), 7.29 (d, 1H, J = 2.0 Hz, H-4), 7.70 (d, 1H, J = 2.0 Hz, H-6), 8.30 (d, 2H, J = 8.0 Hz, H-9/9′), 8.37 (d, 2H, J = 8.0, H-10/10′), 9.33 (s, 1H, H-7); DEPTQ (125 MHz, DMSO-d6) δ 119.9, 123.3, 123.8, 130.3, 134.9, 141.6, 142.4, 142.5, 148.8, 155.9.
4.16. 4-N-amine-3-N-(2-hydroxy-phenylmethilene)-benzoic acid (3n)
mp 194–195°C; IR (ν/cm−1) 3,498, 3,391, 3,098, 1,590, 1,508, 1,334, 1,241, 1,100, 913, 845, 682; 1H NMR (500 MHz, DMSO-d6) δ 5.88 (s, 2H, NH2), 6.76 (d, 1H, J = 8.0 Hz, H-5), 6.94 (d, 1H, J = 5.0 Hz), 6.96 (d, 1H, J = 5.0 Hz), 7.38 (t, 1H, J = 8.0 Hz, H-11), 7.60 (s, 1H), 7.75 (d, 1H, J = 7.0 Hz), 8.90 (s, 1H); 12.36 (s, 1H, OH); DEPTQ (125 MHz, DMSO-d6) δ 113.9, 116.4, 117.9, 119.8, 120.1, 129.5, 132.0, 133.0, 133.7, 147.3, 159.7, 161.4.
Supplementary data
Details and selected data of crystallographic analyses can be found in the submitted electronic supplementary material. Crystallographic data (including structure factors) for the structures in this paper have been deposited with the Cambridge Crystallographic Data Centre as supplementary publication nos. CCDC 1048091-1048096 and CCDC 1054188. Copies of the data can be obtained, free of charge, on application to CCDC, 12 Union Road, Cambridge CB2 1EZ, UK, (Fax: +44 0 1223 336033 or e-mail: [email protected]), more 1H NMR, 13C NMR, and IR spectrums for all compounds.
Supplementary material
Supplementary for this article can be accessed here http://dx.doi.org/10.1080/23312009.2016.1207863.
Additional information
Funding
Notes on contributors
Cláudio E. Rodrigues-Santos
Our group has developed projects in organic synthesis focusing on two points, Green chemistry: utilizing new catalytic, solvent-free, microwave and ultrasound, and in the synthesis of bioactive compounds. This paper is related to the first point.
References
- Asadi, M., Asadi, Z., Torabi, S., & Lotfi, N. (2012). Synthesis, characterization and thermodynamics of complex formation of some new Schiff base ligands with some transition metal ions and the adduct formation of zinc Schiff base complexes with some organotin chlorides. Spectrochimica Acta Part A: Molecular and Biomolecular Spectroscopy, 94, 372–377.10.1016/j.saa.2012.03.061
- Chen, L., Xiang, Y., & Feng, T. (2012). Hybrid compounds of Schiff base Cu, Fe, Co complexes with molybdovanadophoric heteropolyacids: Synthesis, characterization and their catalytic performance to hydroxylation of benzene with H2O2. Applied Organometallic Chemistry, 26, 108–113.10.1002/aoc.v26.3
- Cordes, E. H., & Jencks, W. P. J. (1962). On the mechanism of Schiff base formation and hydrolysis. Journal of the American Chemical Society, 84, 832–837.10.1021/ja00864a031
- Coulthard, G., Unsworth, W, P., & Taylor, R. J. K. (2015). Propylphosphonic anhydride (T3P) mediated synthesis of β-lactams from imines and aryl-substituted acetic acids. Tetrahedron Letters, 56, 3113–3116.
- Deligeorgiev, T., Gadjev, N., Vasilev, A., Kaloyanova, St., Vaquero, J. J., Alvarez-Builla, J. (2010). Green chemistry in organic synthesis. Mini-Reviews in Organic Chemistry, 7, 44–53.
- Dogan, F., & Kaya, I. J. (2013). Thermal decomposition studies of Schiff-base-substitute polyphenol–metal complexes. Journal of Applied Polymer Science, 128, 3354–3362.
- Doi, T., Kawai, H., Murayama, K., Kashida, H., & Asauna, H. (2016). Synthesis and pharmacologicalporperties of 2-azabicyclo [2.2.2] octane derivatives representing conformational restricted isopethidine analogues. European Journal of Medicinal Chemistry, 22, 1–7.
- Esteves-Sousa, A., Rodrigues-Santos, C. E., Cistia, C. N. D., Silva, D. R., Sant’Anna, C. M. R., & Echevarria, A. (2012). Solvent-free synthesis, DNA-topoisomerase II activity and molecular docking study of new asymmetrically N,N′-substituted ureas. Molecules, 17, 12882–12894.
- Ganguly, A., Chakraborty, P., Banerjee, K., & Choudhuri, S. K. (2014). The role of a Schiff base scaffold, N-(2-hydroxy acetophenone) glycinate-in overcoming multidrug resistance in cancer. European Journal of Pharmaceutical Sciences, 51, 96–104.
- Jhaumeer-Laulloo, S., Gupta Bhowon, M. G., Mungur, S., Fawzi Mahomoodally, M. F., & Hussein Subratty, A. H. (2012). In vitro anti-glycation and anti-oxidant properties of synthesized schiff bases. Medicinal Chemistry, 8, 409–414.10.2174/1573406411208030409
- Khanna, I. K., Weier, R. M., Lentz, K. T., Swenton, L., & Lankin, D. C. (1995). Facile, regioselective syntheses of N-alkylated 2, 3-diaminopyridines and imidazo[4, 5-b]pyridines. The Journal of Organic Chemistry, 60, 960–965.10.1021/jo00109a029
- Kielland, N., Escudero-Adán, E. C., Martínez Belmonte, M. M., & Kleij, A. W. (2013). Unsymmetrical octanuclear Schiff base clusters: Synthesis, characterization and catalysis. Dalton Transactions, 42, 1427–1436.10.1039/C2DT31723K
- Kommi, D. N., Kumar, D., Bansal, R., Chebolu, R., Chadraborti, A. K. (2013). “All water chemistry” for a concise total synthesis of the novel class anti-anginal drug (RS), (R), and (S)-ranolazine. Green Chemistry, 15, 756–767.10.1039/c3gc36997h
- Lee, S. K., Tan, K. W., Ng, S. W., Ooi, K. K., Ang, K. P., Abdah, M. A. (2014). Zinc (II) complex with a cationic Schiff base ligand: Synthesis, characterization, and biological studies. Spectrochimica Acta Part A: Molecular and Biomolecular Spectroscopy, 121, 101–108.10.1016/j.saa.2013.10.084
- Panda, S. S., & Jain, S. C. (2012). “On water” synthesis of spiro-indoles via Schiff bases. Monatshefte für Chemie—Chemical Monthly, 143, 1187–1194.10.1007/s00706-011-0697-x
- Qin, W., Long, S., Panunzio, M., & Biondi, S. (2013). Schiff bases: A short survey on an evergreen chemistry tool. Molecules, 18, 12264–12289.10.3390/molecules181012264
- Rodrigues-Santos, C. E., & Echevarria, A. (2007). An efficient and fast synthesis of 4-aryl-3,4-dihydrocoumarins by (CF3SO3)3Y catalysis under microwave irradiation. Tetrahedron Letters, 48, 4505–4508.10.1016/j.tetlet.2007.04.144
- Rodrigues-Santos, C. E., & Echevarria, A. (2011). Convenient syntheses of pyrazolo[3,4-b]pyridin-6-ones using either microwave or ultrasound irradiation. Tetrahedron Letters, 52, 336–340.10.1016/j.tetlet.2010.11.054
- Saggiomo, V., & Lüning, U. (2009). On the formation of imines in water—A comparison. Tetrahedron Letters, 50, 4663–4665.10.1016/j.tetlet.2009.05.117
- Seifzdeh, D., Basharnavaz, H., & Bezaatpour, A. (2014). A Schiff base compound as effective corrosion inhibitor for magnesium in acidic media. Materials Chemistry and Physics, 138, 794–802.
- Zarei, M. (2014). An efficient and green method for the synthesis of 2-azetidinones mediated by propylphosphonic anhydride (T3P®). Monatshefte für Chemie—Chemical Monthly, 145, 1495–1499.