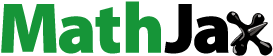
Abstract
Chitosan (CTS) film was prepared by solution casting technique and characterized by means of Fourier Transform Infrared Spectroscopy (FT-IR), Thermogravimetric Analysis (TGA) and Scanning Electron Microscopy (SEM). Besides swelling index, the CTS film was investigated as a slow-release matrix for 1- and 2-naphthol (pesticide precursors) under different pH conditions. Swelling index of the CTS film was determined in different pH conditions i.e. pH 2.59, 6.84 (distilled water) and 11.02. The swelling order was found; pH 2.59 > 6.84 > 11.02. Similarly, the slow-release study was carried out at pH 2.59, 6.84 and 11.02. Over all, the release amount was found higher in acidic medium followed by neutral and basic medium. This behaviour might be due to higher swelling and dissolution of CTS film in acidic medium whereas in neutral and basic medium CTS film swells moderately.
Public Interest Statement
This work demonstrates the effect of pH on slow-release behaviour of chitosan film using 1- and 2-naphthol as model pesticides. Although, chitosan was found a good matrix for slow-release application however, burst release was observed under acidic, basic and neutral pH. Therefore, it was strongly recommended to encapsulate or disperse chitosan within a hydrophobic polymer to prolong the release behaviour.
1. Introduction
A pesticide is any substance intended for preventing, destroying, or mitigating any pest such as insect (insecticide), weeds/herbs (herbicide) and/or fungi (fungicide) (Zhang, Jiang, & Ou, Citation2011). However, pesticide leaching and runoff are the major causes of environmental pollution particularly surface and groundwater contamination (Pérez-Martínez, Morillo, Maqueda, & Ginés, Citation2001). Unfortunately, more than 90% of the conventional pesticides are lost to environment (Xu, Cao, Li, Wang, & Huang, Citation2014), causing severe surface and groundwater contamination (Lewan, Kreuger, & Jarvis, Citation2009). According to the WHO, about three million pesticide poisoning cases with 220,000 deaths occur, each year worldwide whereas, 500,000 people were died from self-harm in 2000 (Eddleston et al., Citation2002). According to another survey, on average 300,000 deaths have been recorded from pesticide poisoning every year (Eddleston et al., Citation2005). Veterinary Diagnostic Laboratory, United States, reported that 0.04% of all animal deaths occur due to pesticide toxicosis (Pimentel, Citation2005). In aquatic environment, irrigation and agricultural runoff have increased pesticide levels where the aquatic life has been affected badly (Capkin, Altinok, & Karahan, Citation2006; Stansley, Citation1993). Therefore, controlled release technologies have been developed to improve the utilization of pesticides and reduce pesticide loss for the last few decades (Teodorescu, Lungu, & Stanescu, Citation2009). In conclusion, controlled-release formulations (CRFs) are the most suitable alternative which can maintain the effective amount over a prolonged period of time and reduce environmental pollution, as well (Xu et al., Citation2014).
The desired controlled release characteristics can be achieved using natural, biodegradable, eco-friendly and cost-effective matrices. Generally, biocomposite formulations are based on the cross-linking of matrix in the presence of active agents (Rudzinski et al., Citation2002). As such, several naturally occurring polymers have been used as controlled release matrices such as alginate (Zhang, Wang, & Wang, Citation2010), cellulose ether (Salsa, Veiga, & Pina, Citation1997), starch (Doane, Shasha, & Russell, Citation1977), amylose starch derivatives (Mulhbacher, Ispas-Szabo, Lenaerts, & Mateescu, Citation2001), polyethylene oxides (Maggi, Segale, Torre, Ochoa Machiste, & Conte, Citation2002), hydroxypropyl methylcellulose (Samani, Montaseri, & Kazemi, Citation2003), cellulose, chitin (McCormick & Lichatowich, Citation1979) and CTS (Cota-Arriola, Onofre Cortez-Rocha, Burgos-Hernández, Marina Ezquerra-Brauer, & Plascencia-Jatomea, Citation2013). However, CTS is suggested because of its natural abundance, biodegradability and eco-friendliness (Rahim & Mas Haris, Citation2015).
CTS, a deacetylated chitin, is a naturally occurring copolymer of unique characteristics such as good biodegradability, environment friendliness, cost-effectiveness and non-toxicity (Rahim & Mas Haris, Citation2015). CTS is a high molecular weight polymer with a degree of deacetylation more than 60%, it can be derived from chitin by alkaline N-deacetylation (Varma, Deshpande, & Kennedy, Citation2004). Whereas, chitin is a polymer of N-acetylglucosamine, the second most abundant polysaccharide in nature after cellulose, a major constituent of the exoskeleton of sea animals such as crabs, shrimps, molluscs and lobsters (Da Silva et al., Citation2009), and also a main constituent of cell walls of fungi and yeast (Abramczyk & Szaniszlo, Citation2009).
In the present study, CTS film was prepared by solution casting technique and its slow-release applications were studied under different pH conditions using 1- and 2-naphthol as model pesticides.
2. Materials and methods
2.1. Materials
CTS with degree of N-deacetylation 95% and relative average molecular weight (Mw~1.05 × 105) was supplied by Advanced Materials Research Centre, Kedah, Malaysia. 1-naphthol (CAS: 90-15-3) and 2-naphthol (product No. 29291) were purchased from ACROS Organics, New Jersey, USA and BDH Chemicals, England, respectively. Glacial acetic acid (CAS: 64-19-7) was brought from QRëC, Malaysia.
2.2. Preparation of CTS film
CTS powder of 2.000 ± 0.0004 g was added to 100 mL of 2% acetic acid aqueous solution (98 mL water + 2 mL acetic acid) and stirred for 30 min using mechanical stirrer with stirring speed of 500 rpm. A glass Petri-dish was covered with polyethylene plastic to overcome the adhesion of CTS film with the Petri-dish, CTS gel was poured into the Petri-dish and dried in oven at 60°C for 48 h.
2.2.1. Loading of naphthols into CTS film
Saturated solutions of 1- or 2-naphthol were prepared in 2% acetic acid aqueous medium. 1- and 2-naphthol, respectively, of 163.2 and 140.4 mg were added to 100 mL volumetric flask separately. Next, 2 mL of acetic acid was added, and diluted up to 100 mL with distilled water. The solutions were stirred using magnetic stirrer for 6 h, at ambient temperature of 27 ± 1°C. Furthermore, 90 or 50% solutions were prepared by dilution of 90 or 50 mL of saturated solution up to 100 mL with 2% acetic acid aqueous solution, respectively. CTS powder of 2.000 ± 0.0004 g was added to 100 mL of either 90 or 50% of 1- or 2-naphthol and stirred for 30 min using mechanical stirrer and the resulting CTS gel was poured into a polyethylene layered Petri-dish and dried in oven at 60°C for 48 h.
2.3. Characterizations
Thermal stability of the CTS powder and film was studied using Perkin-Elmer TGA–7 Thermogravimetric Analyser. TGA was performed in the temperature range of 30 to 900°C with heating rate of 10°C min−1 under nitrogen atmosphere.
FT-IR spectra of CTS and 1-naphthol loaded CTS film were obtained using PerkinElmer Spotlight-200 instrument with Attenuated Total Reflection (ATR) technique. The spectrum background was collected before each sample and the scanning range was adjusted from 650 to 4,000 cm−1. Scanning resolution and number of scans were adjusted 4 cm−1 and 16, respectively.
Morphological investigations of the CTS film were carried out using Leo Supra 50Vp Field Emission Scanning Electron Microscope (FESEM). The samples were dried in oven at 60°C for 24 h, coated onto gold film and observed in the microscope at different magnifications under reduce pressure using pre-pump; Edwards XDS-10 Oil-Free Dry Scroll Vacuum Pump.
2.4. Swelling experiment
The CTS film of 0.2000 ± 0.0008 g was immersed in a screw caped glass bottles containing 50 mL of the media and bottles were incubated at ambient condition. After a predetermined time intervals, the samples were removed, rubbed gently with soft tissue paper and weighed immediately. The study was carried out in triplicate and swelling indices were determined using the following equation (Zaidi, Citation2005):where Wi is the weight of dry CTS film while Wf is the weight of CTS film at time t.
2.5. Preparation of calibration curves
Stock solution of 500 μg mL−1 of 1- or 2-naphthol was prepared by dissolving 500 mg of 1- or 2-naphthol in distilled water using a 1,000 mL volumetric flask. A series of working solutions of 1, 10, 20, 30, 40, 50, 60, 70, 80, 90 and 100 μg mL−1 were prepared from stock solution at different pH (2.59, 6.84 and 11.02) by dilution technique. As such, the pH was adjusted using either 0.1 M solution of CH3COOH or NaOH while pH was measured by Eutech Meter (pH 700, USA). The working solutions were analysed by UV–vis Spectrophotometry and the possible calibrations were obtained by plotting absorbance vs. wavelength.
2.6. Release study of 1- and 2-naphthol
The naphthol loaded CTS film of 0.2000 ± 0.0009 g was kept in 50 mL of distilled water (pH 6.84), solution of pH 2.59 or pH 11.02. The released amount was determined after predetermined time intervals using UV–vis Spectroscopy. Distilled water, a solution of pH 2.59 and pH 11.02 were used as blanks during the sample analysis. The concentration was determined until the equilibrium was achieved.
3. Results and discussion
3.1. FT-IR analysis of CTS film
The FT-IR spectra of CTS film, 1-naphthol and 2-naphthol loaded CTS film are shown in Figure . The FT-IR spectra of CTS and naphthol loaded CTS film indicated a strong and broad absorption band centred at about 3,423 cm−1 corresponds to a combination of N–H and O–H stretchings. The band at 2,880 cm−1 corresponds to the skeletal C–H stretching. The bands at 1,625, 1,423, 1,375 and 1,153 cm−1 correspond to N–H (bending), C–H (CH2, bending), C–H (CH3, symmetrical deformations) and C–O–C (bridge anti-symmetric stretching), respectively. The bands at 1,066 and 1,028 cm−1 correspond to the C–O stretching vibrations while the band at 896 cm−1 corresponds to the C–H out of plane bending vibration (monosaccharide rings) (Fernandes Queiroz, Melo, Sabry, Sassaki, & Rocha, Citation2014). The spectra indicated that there is neither any alteration of peaks nor the appearance of new peaks which shows that there is no change in the chemical structural of CTS.
3.2. TGA analysis
The TGA thermograms of CTS and naphthol loaded CTS film indicate two-step degradation, as shown in Figure . The first step slow degradation of a weight loss of 18% in the temperature region of 40–212°C might be due to volatile compounds and bound water. The second step, rapid decomposition of 58.1% in the temperature region of 238–379°C indicates thermal cleavage and chain scission of C–C and C–O bonds (Peniche-Covas, Argüelles-Monal, & San Román, Citation1993). The DTG thermogram shows two main decomposition peaks in the range of 89.10–124.15 and 146.60–308.29°C. The main decomposition temperatures were observed 115 and 305°C for first and second peak, respectively. The char residue of 33% was observed at 900°C. As such, CTS film follows thermal decomposition behaviour of CTS, indicates that there is no chemical change in the structural backbone of CTS after making its acidified film.
3.3. Morphology
The CTS film before and after the release tests are shown in Figure . CTS film was collected after the release experiment and dried in oven at 60°C for 24 h. Morphology of the CTS film was observed under SEM at different magnifications. The CTS film before the release experiments shows smooth surface having no pores and roughness. As such at lower magnification (50x), figures (a), (b), (c) and (d) indicate no clear effect of pH on the morphology of CTS film however, at higher magnification (50,000×), figures (a′), (b′), (c′) and (d′) indicate that the CTS film collected from lower pH (pH 2.59) indicates homogeneous distribution of micro-holes, might be created by acid dissolution. As such, CTS films collected from pH 6.84 to 11.02 having continuously smooth surface without any perforation which shows that CTS film is quite stable in neutral and basic medium.
3.4. Water uptake study
The swelling of polymer involves the absorption of a liquid resulting in an increase in weight and volume. The hydration of polymers and saturation of capillaries within the polymeric materials play an important role in the uptake of liquids. The liquid enters into the capillaries braking the hydrogen bonding resulting in swelling of the polymers. The swelling of a polymer can be determined using weight gained by the polymer after putting in a liquid medium.
The results of water uptake by CTS film at different pH conditions (pH 2.59, 6.84 and 11.02) are shown in Figure . The results indicate that the swelling of CTS film is much faster and on average the swelling index of CTS film in acidic medium is 3.69 and 1.18 times higher than basic and neutral medium at 2nd hour. Whereas, at levelling off point (equilibrium state), the swelling index of CTS film is 1.26 and 1.25 times basic and neutral medium. The results clearly show that the swelling of CTS film is higher in acidic medium followed by neutral and basic medium. This behaviour of the CTS film might be due to ability of CTS to protonate in acidic medium and tends to dissolve. As, we can see after 72nd hour the swelling of CTS film in acidic medium decreases, this might be its dissolution, as supported by the SEM images.
3.5. Preparation of calibration curves
1-naphthol indicated local maxima (λL.max) at 321.5, 321.5 and 332.5 nm in distilled water, acidic and basic medium, respectively. Similarly, 2-naphthol exhibits λL.max at 274, 274 and 281.5 nm, respectively, in distilled water, acidic and basic medium. The UV–Vis spectra of 1- and 2-naphthol in distilled water, acidic and basic media are shown in Figure . The calibration curves of 1- and 2-naphthol are displayed in Figure (a) and (b), respectively. It can be seen from the calibration curves that 1- and 2-naphthol can be measured with high accuracy from 1 to 40 μg mL−1 in distilled water while 1-naphthol can be measured from 1 to 60 μg mL−1 in both acidic and basic media. As such, 2-naphthol can be measured from 1 to 100 μg mL−1 and 1 to 70 μg mL−1, respectively, in acidic and basic medium.
Figure 5. The UV-Spectra of 1-naphthol in: (a) distilled water, (b) pH 2.59, (c) pH 11.02, and 2-naphthol in: (d) distilled water, (e) pH 2.59 and (f) pH 11.02.
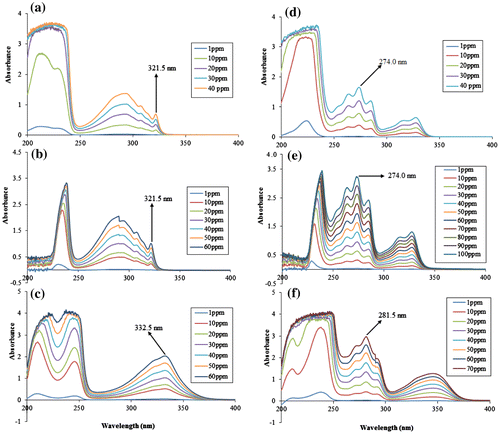
The absorbance of 1- and 2-naphthol is greatly influenced by pH of the solvent, as there are various interactions between solute and solvent. Although, both 1- and 2-naphthol can form hydrogen bonding with water and among yourself as well. However, in acidic medium the hydrogen bonding is affected destructively due to protonation of hydroxyl group of the naphthol and water molecules. As such, in case of basic medium using NaOH, naphthols have acidic properties and react with alkalis to form sodium salt (Practical-Lesson, Citation2013), as shown in Figure .
3.6. Slow-release study
CTS film was used as slow-release matrix for 1- and 2-naphthol under different pH conditions. The release behaviour of 1-naphthol from initial loading of 50 and 90% is shown in Figure (a) and (b), respectively. The results clearly show, the absolute release amount increases with initial loading and also with time up to 72 and 48 h, respectively, with initial loading of 50 and 90%. The absolute release amount in acidic medium was found higher than neutral followed by basic medium. The absolute amount released at equilibrium in distilled water, acidic and basic media from 50% initial loading was found 87.27, 90.00 and 54.95 mg L−1, respectively. The initial loading of 90% shows 61.94, 114.77 and 119.97 mg L−1, respectively, in distilled water, acidic and basic medium.
Figure 8. Release behaviour of 1-naphthol from CTS film for the initial loading of: (a) 50% and (b) 90%.
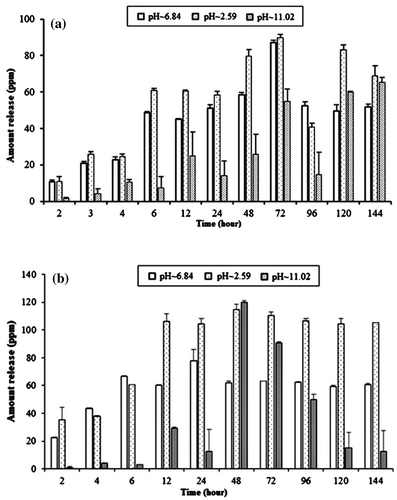
The absolute release amount of 2-naphthol with initial loading of 50 and 90% is shown in Figure (a) and (b), respectively. The results indicate that the release amount increases with time and maximum amount was released after 48 h. On average, the release of both 1- and 2-naphthol is higher in low pH; pH of 2.59 followed by 6.84 and 11.02. The absolute amount released at equilibrium in water, acidic and basic media from 50% initial loading was found 40.01, 45.00 and 33.96 mg L−1, respectively. The initial loading of 90% shows 49.32, 76.00 and 62.32 mg L−1, respectively, in distilled water, acidic and basic medium.
3.7. Release mechanism
CTS film swells and hydration take place. Once the film hydrated, diffusion of water molecules helps to break down hydrogen bonding between naphthol and the active sites of CTS (–NH2). In the next step, the impregnated naphthols diffusing out with water molecules without any barrier force. In case of this study, SEM images of CTS film indicate that at lower pH, CTS dissolves leading to a clear perforation, and thus the release amount is higher at pH 2.59. As such, at high pH the CTS film shrinks and holds the naphthols tightly resulting in lower amount to be released which was confirmed by the SEM (a smooth surface without any perforation). A theoretical model is presented to show the possible diffusion way of naphthols from CTS film (Figure ).
4. Conclusion
CTS film was prepared and characterized by means of various techniques. The swelling index of the CTS film was carried out in different pH conditions. The results indicated that the swelling is higher in lower pH, this might be due to protonation of the amino group of the CTS film resulting in dissolution of the CTS monomers. The order of swelling indices was found; acidic medium > neutral medium > basic medium. Similarly, slow-release studies of the CTS film were performed under different pH conditions to know the effect of pH on the release behaviour of CTS film. On average, the release of naphthols was found higher in acidic medium followed by neutral and basic media. Overall, the release behaviour is quite controlled, consistent and prolonged over a period of 12 h. In conclusion, CTS film is a good biodegradable and eco-friendly polymer therefore, CTS encapsulated with other hydrophobic natural polymers is strongly recommended for slow-release applications. In this context, our research group is working to encapsulate and/or disperse CTS within a hydrophobic polymer in order to prolong the release of active chemicals.
Funding
The authors are thankful to Universiti Sains Malaysia for providing financial assistance [grant number 1001/PKIMIA/814124]. Also author Muhammad Rahim is thankful to The World Academy of Sciences and Universiti Sains Malaysia for providing, 2012-USM-TWAS-Postgraduate-Fellowship.
Additional information
Notes on contributors
Muhammad Rahim
Muhammad Rahim passed his MSc degree in Inorganic Chemistry (2012) from the University of Peshawar, Pakistan. Currently, he is a PhD student in the School of Chemical Sciences, Universiti Sains Malaysia, Malaysia. His research interests are: polymeric advance materials and its applications. He published six articles. Norhidayah Abu, passed his MSc degree in Inorganic Chemistry (2016) from Universiti Sains Malaysia, Malaysia. Mas Rosemal Hakim Mas Haris, Associate Professor of Chemistry at School of Chemical Sciences, Universiti Sains Malaysia. He obtained his PhD from Virginia Polytechnic Institute & State University (1987–1989). His research interests cover: (1) Synthesis and characterization new phosphazene-based compounds (monomers, oligomers and polymers for specific applications). (2) Tailored modification of rubber and its derivatives: preparation of (a) water-soluble and (b) flame retardant polymeric materials. (3) Structural characterization and reactivity study of natural products.
References
- Abramczyk, D., & Szaniszlo, P. J. (2009). Immunoaffinity purification of the class V chitin synthase of wangiella (exophiala) dermatitidis. Preparative Biochemistry and Biotechnology, 39, 277–288.10.1080/10826060902953244
- Capkin, E., Altinok, I., & Karahan, S. (2006). Water quality and fish size affect toxicity of endosulfan, an organochlorine pesticide, to rainbow trout. Chemosphere, 64, 1793–1800.10.1016/j.chemosphere.2005.12.050
- Cota-Arriola, O., Onofre Cortez-Rocha, M., Burgos-Hernández, A., Marina Ezquerra-Brauer, J., & Plascencia-Jatomea, M. (2013). Controlled release matrices and micro/nanoparticles of chitosan with antimicrobial potential: Development of new strategies for microbial control in agriculture. Journal of the Science of Food and Agriculture, 93, 1525–1536.10.1002/jsfa.2013.93.issue-7
- Da Silva, C. A., Chalouni, C., Williams, A., Hartl, D., Lee, C. G., & Elias, J. A. (2009). Chitin is a size-dependent regulator of macrophage TNF and IL-10 production. The Journal of Immunology, 182, 3573–3582.10.4049/jimmunol.0802113
- Doane, W., Shasha, B., & Russell, C. (1977). Encapsulation of pesticides within a starch matrix. Controlled Release Pesticides, 53, 74-83.10.1021/symposium
- Eddleston, M., Gunnell, D., Karunaratne, A., De Silva, D., Sheriff, M. R., & Buckley, N. A. (2005). Epidemiology of intentional self-poisoning in rural Sri Lanka. The British Journal of Psychiatry, 187, 583–584.10.1192/bjp.187.6.583
- Eddleston, M., Karalliedde, L., Buckley, N., Fernando, R., Hutchinson, G., Isbister, G., ... Smit, L. (2002). Pesticide poisoning in the developing world—A minimum pesticides list. The Lancet, 360, 1163–1167.10.1016/S0140-6736(02)11204-9
- Fernandes Queiroz, M., Melo, K. R. T., Sabry, D. A., Sassaki, G. L., & Rocha, H. A. O. (2014). Does the use of chitosan contribute to oxalate kidney stone formation? Marine Drugs, 13, 141–158.10.3390/md13010141
- Lewan, E., Kreuger, J., & Jarvis, N. (2009). Implications of precipitation patterns and antecedent soil water content for leaching of pesticides from arable land. Agricultural Water Management, 96, 1633–1640.10.1016/j.agwat.2009.06.006
- Maggi, L., Segale, L., Torre, M., Ochoa Machiste, E., & Conte, U. (2002). Dissolution behaviour of hydrophilic matrix tablets containing two different polyethylene oxides (PEOs) for the controlled release of a water-soluble drug. Dimensionality study. Biomaterials, 23, 1113–1119.10.1016/S0142-9612(01)00223-X
- McCormick, C., & Lichatowich, D. (1979). Homogeneous solution reactions of cellulose, chitin, and other polysaccharides to produce controlled-activity pesticide systems. Journal of Polymer Science: Polymer Letters Edition, 17, 479–484.
- Mulhbacher, J., Ispas-Szabo, P., Lenaerts, V., & Mateescu, M. A. (2001). Cross-linked high amylose starch derivatives as matrices for controlled release of high drug loadings. Journal of Controlled Release, 76, 51–58.10.1016/S0168-3659(01)00425-4
- Peniche-Covas, C., Argüelles-Monal, W., & San Román, J. (1993). A kinetic study of the thermal degradation of chitosan and a mercaptan derivative of chitosan. Polymer Degradation and Stability, 39, 21–28.10.1016/0141-3910(93)90120-8
- Pérez-Martínez, J. I., Morillo, E., Maqueda, C., & Ginés, J. M. (2001). Ethyl cellulose polymer microspheres for controlled release of norfluazon. Pest Management Science, 57, 688–694.10.1002/ps.339
- Pimentel, D. (2005). Environmental and economic costs of the application of pesticides primarily in the United States. Environment, Development and Sustainability, 7, 229–252.10.1007/s10668-005-7314-2
- Practical-Lesson. (2013). Methodical instruction for students of the 2 course pharmaceutical faculty, lesson no. 11 (practical–6 h). Retrieved January 16, 2016, from http://intranet.tdmu.edu.ua/data/kafedra/internal/zag_him/metod_rozrobky/en/pharm/prov_pharm/ptn/organic%20chemistry/2%20course/11.%20Phenols.%20Ethers.%20Small%20practicum.htm
- Rahim, M., & Mas Haris, M. R. H. (2015). Application of biopolymer composites in arsenic removal from aqueous medium: A review. Journal of Radiation Research and Applied Sciences, 8, 255–263.10.1016/j.jrras.2015.03.001
- Rudzinski, W. E., Dave, A. M., Vaishnav, U. H., Kumbar, S. G., Kulkarni, A. R., & Aminabhavi, T. M. (2002). Hydrogels as controlled release devices in agriculture. Designed Monomers and Polymers, 5, 39–65.10.1163/156855502760151580
- Salsa, T., Veiga, F., & Pina, M. (1997). Oral controlled-release dosage forms. I. Cellulose ether polymers in hydrophilic matrices. Drug Development and Industrial Pharmacy, 23, 929–938.10.3109/03639049709148697
- Samani, S. M., Montaseri, H., & Kazemi, A. (2003). The effect of polymer blends on release profiles of diclofenac sodium from matrices. European Journal of Pharmaceutics and Biopharmaceutics, 55, 351–355.10.1016/S0939-6411(03)00030-4
- Stansley, W. (1993). Field results using cholinesterase reactivation techniques to diagnose acute anticholinesterase poisoning in birds and fish. Archives of Environmental Contamination and Toxicology, 25, 315–321.10.1007/BF00210723
- Teodorescu, M., Lungu, A., & Stanescu, P. O. (2009). Preparation and Properties of Novel Slow-Release NPK agrochemical formulations based on poly(acrylic acid) hydrogels and liquid fertilizers. Industrial & Engineering Chemistry Research, 48, 6527–6534.10.1021/ie900254b
- Varma, A. J., Deshpande, S. V., & Kennedy, J. F. (2004). Metal complexation by chitosan and its derivatives: A review. Carbohydrate Polymers, 55, 77–93.10.1016/j.carbpol.2003.08.005
- Xu, L., Cao, L.-D., Li, F.-M., Wang, X.-J., & Huang, Q.-L. (2014). Utilization of chitosan-lactide copolymer nanoparticles as controlled release pesticide carrier for pyraclostrobin against colletotrichum gossypii southw. Journal of Dispersion Science and Technology, 35, 544–550.10.1080/01932691.2013.800455
- Zaidi, S. J. (2005). Preparation and characterization of composite membranes using blends of SPEEK/PBI with boron phosphate. Electrochimica Acta, 50, 4771–4777.10.1016/j.electacta.2005.02.027
- Zhang, J., Wang, Q., & Wang, A. (2010). In situ generation of sodium alginate/hydroxyapatite nanocomposite beads as drug-controlled release matrices. Acta Biomaterialia, 6, 445–454.10.1016/j.actbio.2009.07.001
- Zhang, W., Jiang, F., & Ou, J. (2011). Global pesticide consumption and pollution: With China as a focus. Proceedings of the International Academy of Ecology and Environmental Sciences, 1, 125–144.