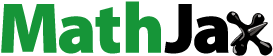
Abstract
Two complexes, a cobalt(II) complex [Co(phen)3(NO3)2]·2H2O (1) and a novel Co(III) complex with mixed ligands [diazido-bis(1,10-phenanthroline-κ2 N,N′)cobalt(III)]nitrate [Co(phen)2(N3)2]NO3 (2), have been synthesized and were characterized by physico-chemical and spectroscopic methods. The cobalt(III) complex (2) crystallizes in the orthorhombic crystal system with space group Iba2 with four formula units. The central Co(III) atom is six coordinate with four N atoms of two 1,10-phenanthroline (1,10-phen) molecules and two terminal N atoms from two azide anions giving a distorted octahedral geometry with a CoN6 chromophore. The molecular structure of the compound is consolidated by face-to-face π–π stacking between the aromatic rings of 1,10-phen ligand. The ligands, metal salt and the complexes were also evaluated for their antimicrobial activities in vitro against eight pathogens (four bacteria and four fungi species).
Public Interest Statement
The frequent, inappropriate and abusive use of drugs to fight infections caused by micro-organisms has led to resistant pathogens which are resistant to many drugs. Efforts made to develop drugs to fight against these resistant pathogens include protection of the efficacy and appropriate use of existing drugs as well as research and development of new drugs. Metal complexes have found application as novel diagnostic and antimicrobial agents. This study presents the synthesis, characterization and structure elucidation of cobalt(II) and cobalt(III) complexes with 1,10-phen, nitrate and azide co-ligands. The complexes were screened for their activities against four pathogenic bacteria and four fungi species. The results indicate that the complexes have activities higher than that of the metal salt but lower than that of 1,10-phen. The most sensitive strains are E. coli, P. aeruginosa, S. typhi, C. albicans ATCC P37037 and C. neoformans. The positive results suggest that the complexes have a broad spectrum of activity.
1. Introduction
The design and synthesis of new metal complexes based on transition metal ions and diimine ligands, such as 1,10-phenanthroline (1,10-phen) and 2,2′-bipyridine, is an interesting field for the development of new functional materials with intriguing structures and potential applications (Amani, Safari, Khavasi, & Mirzaei, Citation2007). The use of mixed functional ligands in this process can enable the modification of the physical and chemical properties of these metal complexes. Mixed ligand complexes with 1,10-phen is also interesting due to their potential role as models for biological systems such as binding of small molecules to DNA (Jennifer & Muthiah, Citation2014; Yesilel, Olmez, Yılan, Pasaoglu, & Buyukgungor, Citation2006). Since the aromatic rings have an extended π-system and can give the ligands various non-covalent π-interactions which mimic various biological processes, the study of these complexes has gained importance (Jennifer & Muthiah, Citation2014; Pook, Hentrich, & Gjikaj, Citation2015). The geometries of the complexes are greatly influenced by factors such as different coordination abilities of the ligands and the counterion. These nitrogen-containing heterocycles are metal-coordinating, electron-deficient aromatic systems which can undergo π–π stacking interactions as π-acceptors (Pook et al., Citation2015).
The chemistry of transition metal complexes has received much attention in recent years on account of their applications, amongst others, in biological systems as antimicrobial agents (McCann et al., Citation2000; Mishra, Kaushik, Verma, & Gupta, Citation2008; Shaabani, Khandar, Dusek, Pojarova, & Mahmoudi, Citation2013; Yenikaya et al., Citation2009), DNA studies (Arounaguiri, Easwaramoorthy, Ashokkumar, Dattagupta, & Maiya, Citation2000a, Citation2000b; Gopinathan, Komathi, & Arumugham, Citation2014; Kou et al., Citation2009; Kumar et al., Citation2008), larvicidal activity (Gopinathan & Arumugham, Citation2015), and cytotoxicity studies (Deegan, McCann, Devereux, Coyle, & Egan, Citation2007; Pivetta et al., Citation2012; Silva et al., Citation2011). The metal complexes of diimine ligands such as 1,10-phen and bipyridine have received much research interest because of their versatile roles as building blocks for the synthesis of metallo-dendrimers as well as their bioinorganic and biomedicinal applications (Ahmed & Khaled, Citation2015; Gopinathan & Arumugham, Citation2015; Molphy, Slator, Chatgilialoglu, & Kellett, Citation2015; Rajarajeswari, Ganeshpandian, Palaniandavar, Riyasdeen, & Akbarsha, Citation2014; Wang et al., Citation2014).
1,10-phen is a heterocyclic bidentate chelating ligand that bonds to metal atoms (ions) using the lone pairs of electrons on the nitrogen atoms resulting in a five-membered ring structure (Bencini & Lippolis, Citation2010). In this ligand, the σ-donation is complemented by the π-acceptor ability giving the complex formed greater stability (Janiak, Citation2000; Pook et al., Citation2015).
Cobalt is a component of vitamin B12 complex that is useful in the prevention of anaemia and the production of erythrocytes. Interest in cobalt complexes has also increased due to their therapeutic and biological applications (Mishra et al., Citation2008).
The azide anion is a versatile ligand that has been intensively studied because of its ability to coordinate to transition metals with different coordination modes generating a wide variety of fascinating structures (discrete molecules to 3D arrays) and their promising applications in functional materials (Chen, Jiang, Yan, Liang, & Batten, Citation2009; Lazari et al., Citation2009). The coordination modes of the azide anion range from monodentate to bridging bi-, tri- and tetra-dentate (Adhikary & Koner, Citation2010; Goher & Mautner, Citation1995; Lazari et al., Citation2009; Ye, Cheng, Li, & Hu, Citation2004). The most common bridging coordination modes of the azide ligand are μ−1,1 (end-on, EO), μ−1,3 (end-to-end, EE), μ−1,1,3 and others (Batten & Murray, Citation2003). The azide ion easily coordinates to transition metal ions and due to the π-donor properties of this ligand, its occupied π-orbitals can also overlap with the d-orbitals of the corresponding metal ion.
The nitrate group can function as a bidentate ligand, bridging group, monodentate ligand, and ionic species in various inorganic systems (Biagetti & Haendler, Citation1966). The functional characteristics probably depend on the nature and number of molecules of other ligands present.
In order to fight against antimicrobial resistance, our research team has recently focused on the synthesis and antimicrobial screening of some transition metal complexes of the ligands hexamethylenetetramine (Tabong et al., Citation2016), pyridine-2-carboxylic acid (Amah, Ondoh, Yufanyi, & Gaelle, Citation2015), 2-aminopyridine (Yuoh et al., Citation2015), mixture of ligands 1,10-phen and 2,2′-bipyridine (Ndosiri et al., Citation2013; Sado, Agwara, Yufanyi, Nenwa, & Jagan, Citation2016). The upsurge of resistant pathogens impedes the effective prevention and treatment of an ever-increasing variety of infections caused by bacteria, parasites, viruses and fungi (WHO, Citation2014). This situation poses a public health and economic burden to countries worldwide (Hawkey & Jones, Citation2009; Tanwar, Das, Fatima, & Hameed, Citation2014; WHO, Citation2014). Several efforts have been made to develop antimicrobial agents to fight against these resistant pathogens amongst which are the protection of the efficacy and appropriate use of existing drugs as well as research and development of new antimicrobial agents that are not affected by the currently known, predicted, or unknown mechanisms of resistance (Spellberg et al., Citation2008; Weinstein & Fridkin, Citation2003). Metal complexes of biologically active ligands are a target for the development of new active agents.
In view of the varied applications of cobalt mixed ligand complexes and exploring the good biological properties of cobalt and 1,10-bipy as well as the structure-directing properties of N3−, we report herein the synthesis and structure elucidation of cobalt(II) and cobalt(III) complexes of 1,10-phen, N3− and NO3−. The effects of the co-ligands on the biological activities of the complexes towards some resistant pathogens, evaluated using in vitro assays, are also presented.
2. Experimental
2.1. Materials and methods
Co(NO3)2·6H2O, sodium azide, 1,10-phen and anhydrous methanol 99.8% were obtained from Sigma–Aldrich. All chemicals (reagent grade) and solvents were used as received.
2.2. Synthesis of the complexes
2.2.1. Synthesis of [Co(phen)3(NO3)2]·2H2O (1)
A 25 mL methanol solution of 1,10-phen (0.60 g, 3.0 mmol) was added drop wise to a 25 mL methanol solution of Co(NO3)2·6H2O (0.29 g, 1.0 mmol). The mixture was refluxed at 90°C for 4 h. The pink precipitate obtained was filtered, washed with methanol and dried in vacuo. Dark brown block-shaped crystals suitable for single crystal X-ray diffraction obtained from the filtrate within two weeks were washed with acetone and dried in vacuo.
2.2.2. Synthesis of [Co(phen)2(N3)2]NO3 (2)
A 25 mL of methanol solution of 1,10-phen (0.39 g, 2.0 mmol) was added drop wise to a 25 mL methanol solution of Co(NO3)2·6H2O (0.29 g,1.0 mmol). The mixture was refluxed at 90°C for an hour. Sodium azide (0.13 g, 2 mmol) dissolved in 2 mL of water and 8 mL of methanol was added drop wise to the reaction mixture and it was further refluxed for 4 h. The yellow precipitate obtained was filtered, washed with ethanol and dried in vacuo. Dark red block-shaped crystals suitable for single crystal X-ray diffraction obtained from the filtrate within two months were washed with acetone and then dried in vacuo.
2.3. Characterization
The melting point/decomposition temperatures were recorded using a STUART SCIENTIFIC melting point apparatus. The conductivity of the complex was measured in distilled water using a HANNA multimeter type H19811–5, pH/°C/EC/TDS meter at room temperature. The infrared spectrum was recorded using a Bruker ALPHA-P spectrophotometer directly on a small sample of the complex in the range 400–4,000 cm−1, while the UV–vis spectrum of an aqueous solution of the complex was recorded using a Bruker HACH DR 3,900 UV–vis spectrophotometer at room temperature. Thermogravimetric measurements were obtained using a Pyris 6 PerkinElmer TGA 4,000 thermal analyser. TGA analysis was conducted between 35 and 930°C under nitrogen atmosphere at a flow rate of 20 mL/min and a temperature ramp of 10°C/min.
2.4. Single crystal X-ray structure determination of [Co(phen)2(N3)2]NO3 (2)
Intensity data for the compound was collected using a Bruker AXS Kappa APEX II single crystal CCD Diffractometer, equipped with graphite-monochromated MoKα radiation (λ = 0.71073 Å) at room temperature. The selected crystal for the diffraction experiment had a dimension of 0.25 × 0.25 × 0.20 mm3. Accurate unit cell parameters were determined from the reflections of 36 frames measured in three different crystallographic zones by the method of difference vectors. The data collection, data reduction and absorption correction were performed by APEX2, SAINT-plus and SADABS program (Bruker AXS, Citation2004). The structure was solved by direct methods procedure using SHELXS-97 program (Sheldrick, Citation2008) and the non-hydrogen atoms were subjected to anisotropic refinement by full-matrix least squares on F2 using SHELXL-97 program (Sheldrick, Citation2008). The positions of all the hydrogen atoms were identified from difference electron density map and were fixed accordingly. All the aromatic hydrogen atoms were constrained to ride on the corresponding non-hydrogen atoms with a distance of C-H = 0.93 Å and Uiso(H) = 1.2Ueq(C). Whereas the hydrogen atoms associated with all the N atoms were restrained to a distance of N-H = 0.88(2) Å.
2.5. Antimicrobial tests
The antimicrobial tests were carried out in the laboratory of Phytobiochemical and Medicinal Plant Study, University of Yaounde I. The tests were done on eight pathogenic micro-organisms, four bacterial strains, Staphylococcus aureus CIP 7625 (Gram-positive) and Pseudomonas aeruginosa CIP 76110, Salmonella typhi and Escherichia coli ATCC 259224 (Gram-negative) and four yeasts, Candida albicans ATCC P37039, C. albicans 194B, Candida glabrata 44B, Cryptococcus neoformans obtained from Centre Pasteur Yaoundé, Cameroon. Reference antibacterial drug gentamycin and antifungal drug nystatin were evaluated for their antibacterial and antifungal activities and their results were compared to those of the free ligands and the complex.
The disc diffusion method, using Muller Hinton Agar, from the protocol described by the National Committee for Clinical Laboratory Standard was used for preliminary screening.
Mueller-Hinton agar was prepared from a commercially available dehydrated base according to the manufacturer’s instructions. Several colonies of each micro-organism was collected and suspended in saline (0.9% NaCl). Then, the turbidity of the test suspension was standardized to match that of a 0.5 McFarland standard (corresponds to approximately 1.5 × 108 CFU/mL for bacteria or 1 × 106 to 5 × 106 cells/mL for yeast). Each compound or reference was accurately weighed and dissolved in the appropriate diluents (DMSO at 10%, Methanol at 10% or distilled water) to yield the required concentration (2 mg/mL for compound or 1 mg/mL for reference drug), using sterile glassware.
Whatman filter paper No. 1 was used to prepare discs approximately 6 mm in diameter, which were packed up with aluminium paper and sterilized by autoclaving. Then, 25 μL of stock solutions of compound or positive control were delivered to each disc, leading to 50 μg of compound or 25 μg of reference drug.
The dried surface of a Müeller-Hinton agar plate was inoculated by flooding over the entire sterile agar surface with 500 μL of inoculum suspensions. The lid was left ajar for 3–5 min to allow for any excess surface moisture to be absorbed before applying the drug impregnated discs. Discs containing the compounds or antimicrobial agents were applied within 15 min of inoculating the MHA plate. Six discs per petri dish were plated. The plates were inverted and placed in an incubator set to 35°C. After 18 h (for bacteria) and 24 h (for yeasts) of incubation, each plate was examined. The disc diameter and the diameter of the zones of complete inhibition (as judged by the unaided eye) were measured. Zones were measured to the nearest whole millimetre, using sliding callipers, which was held on the back of the inverted petri plate. All experiments were carried out in duplicate. The compound was considered active against a microbe if the inhibition zone was 6 mm and above.
2.6. Minimum inhibitory concentration (MIC) of the complexes
The microbroth dilution method was used to determine the MIC of the compounds and the reference antibiotic on a given micro-organism. A polystyrene tray containing 80 wells is filled with small volumes of serial twofold dilutions of the complex and reference antibiotics. The inoculum suspension and standardization is done according to McFarland standard. The bacterial inoculum is then inoculated into the wells and incubated at 37°C overnight. The lowest concentration of antibiotic that completely inhibits visual growth of bacteria (no turbidity) is recorded as MIC.
3. Results and discussion
3.1. Synthesis of the complex
The reaction of Co(NO3)2·6H2O and 1,10-phen with and without azide in methanol yielded two complexes whose physicochemical properties are summarized in Table . The complexes which are coloured and air stable were obtained in good yields (>75%). Complex 1, a Co(II) complex, had a sharp melting point (314°C) indicating its purity, while the melting point of complex 2, a Co(III) complex, could not be determined due to the explosive nature of the azide. The molar conductivity values of 68.4 and 120 Ωcm−2 mol−1 in water, for complexes 1 and 2, respectively, indicate that complex 1 is a non-electrolyte, while complex 2 is a 1:1 electrolyte type.
Table 1. Physical data of the complexes
The chemical reaction leading to the formation of complex 2 is a redox process where Co(II) becomes oxidized to Co(III). The oxidation of Co(II) to Co(III) generates a diamagnetic complex and indicates an octahedral structure for complex 2. Most Co3+ complexes are diamagnetic; the mixed ligand under the investigation behaves as a strong field.
3.2. Crystal structure of complex 2
The crystals obtained for complex 1 were not suitable for single crystal X-ray structure determination. Thus, only the crystal structure of complex 2 was determined.
The ORTEP representation of the crystal structure of [Diazido-bis(1,10-phen-κ2 N,N′)cobalt(III)]nitrate, [Co(C12H8N2)2(N3)2]NO3 with the atom numbering scheme used in the corresponding tables is shown in Figure . The crystal packing diagram for [Co(C12H8N2)2(N3)2]NO3 seen along the crystallographic c-axes is shown in Figure . The crystal data and structure refinement are presented in Table , while the selected bond lengths and bond angles are shown in Table .
Table 2. Crystal data and structure refinement for [Co(C12H8N2)2(N3)2]NO3 (2)
Table 3. Selected bond lengths (Å) and angles (o) for complex (2)
Complex 2 crystallizes in the orthorhombic crystal system with space group Iba2 with four formula units. The asymmetric unit consists of one molecule of 1,10-phen, one azide anion, one nitrate anion and one Co(III) ion. The molecular structure of complex 2 contains one monomeric cation, [Co(phen)2(N3)2]+ and one nitrate ion, as revealed in Figure . Chemically, each Co atom is six coordinate; it is bonded to four N atoms of two phen molecules [Co1—N1 1.955 (2) Å, Co1—N1i 1.955 (2) Å, Co1—N2 1.9356 (17) Å, Co1—N2i 1.9356 (17) Å] and two terminal N atoms from two azide anions [Co1—N3 1.933 (3) Å, Co1—N3i 1.933 (3) Å] giving a slightly distorted octahedral geometry around the Co atom with CoN6 chromophore. The axial positions are occupied by the N2 and N2i atoms of two different phen molecules [N2—Co1—N2i 179.98 (16)°] while the equatorial plane is formed by the coordinating atoms N1, N1i [N1—Co1—N1i 89.01 (13)°] from phen and N3, N3i [N3—Co1—N3i 93.86 (17)°] from the two azide anions. The Co-N(phen) bond lengths are shorter than Co-N(phen) bonds reported in the literature (Guo, Shi, Si, Duan, & Shi, Citation2013; Pook et al., Citation2015). The structure of (2) contains one crystallographically independent Co(III) atom with the angles around Co1 slightly distorted from the ideal 90° and 180° of a perfect octahedron (Richers, Bertke, & Rauchfuss, Citation2015). The bond angles N3—Co1—N2 89.55 (11)°, N3i—Co1—N2 90.44 (10)°, N3—Co1—N2i 90.44 (10)° and N3i—Co1—N2i 89.55 (11)° indicate that the Co1—N2 and N3—Co1 bonds are in two different molecular planes perpendicular to each other. The two phen ligands which form five-membered chelate rings with Co are oriented in two different molecular planes (Liang et al., Citation2003; Meundaeng, Prior, & Rujiwatra, Citation2013). These bond angles slightly deviate from 90° indicating a distorted square planar arrangement in the equatorial plane. The N5—N4—N3 bond angle of 175.3° (3) indicates that the azide anion is non-linear. The presence of nitrate O and azide N atoms in the molecular structure act as acceptor centres for the formation of C-H … O and C-H … N intermolecular interactions. These weak, C5-H5 … O2, C11-H11 … O2, C8-H8 … N5, intermolecular interactions, result in the formation of two dimensional supramolecular sheets in the ab plane. The adjacent two-dimensional supramolecular sheets exchange π–π interactions experienced between the 1,10-phen moiety forming an extended three-dimensional network in the solid. The cobalt atoms between the stacked sheets running along the c-direction have an interlayer Co–Co distance of 7.369 Å.
3.3. Infrared spectroscopy
The characteristic absorption bands in the IR spectra of the ligands and the complexes (1 and 2) are summarized in Table .
Table 4. Characteristic IR bands of the ligands and the complexes
In the spectrum of the phen ligand, the absorption bands at 1,586 and 1,492 cm−1 assigned to ν(C = N) and ν(C = C) stretching vibrations, respectively, are shifted in the complexes to 1,518 and 1,480 cm−1 (1) and 1,578 and 1,512 cm−1 (2), respectively, indicating the participation of the C = N of phen in bonding (Liang et al., Citation2003; Lu, Zhu, & Yang, Citation2003). The strong absorption band at 2,103 cm−1 in the spectrum of the azide ligand, assigned to the asymmetric stretching vibration vas(N3) of the azide is shifted to 2,073 cm−1 in complex 2 indicating terminal coordination of the azide to the metal ion (Goher et al., Citation2004; Liang et al., Citation2003). The new bands at 497 and 419 cm−1 which were not found in the spectra of the ligands, indicates the presence of Co-N bonding between the metal and the nitrogen atoms of both phen and the azide.
3.4. UV–vis spectroscopy
The UV–vis spectra of the complexes were measured in aqueous solution at ambient temperature.
The ground term symbol for d6 Co(III) is 5D and the octahedral high spin spectroscopic ground state is 5T2g. Thus, a single spin-allowed transition 5Eg ← 5T2g is predicted but this will generally be split because of Jahn-Teller distortion. Most of the six coordinate complexes of cobalt(III) are low spin and have the spectroscopic ground term symbol 1A1g. Two transitions: 1T1g ← 1A1g and 1T2g ← 1A1g are expected for six coordinate low spin Co(III) complexes (Lever, Citation1984). The ground term symbol of d7 Co(II) is 4F. In octahedral Co(II) complexes, three transitions 4T2g ← 4T1g(F), 4A2g ← 4T1g(F) and 4T1g(P) ← 4T1g(F) are expected. The transition to 4A2g is usually very weak and often appears as a shoulder.
The electronic absorption spectrum of Co(III) (complex 2) shows a single broad band centred at 18,518.5 cm−1 (530 nm). This d–d absorption band in has been assigned to 5Eg ← 5T2g transition (Abdelhak et al., Citation2014). The electronic spectrum of Co(II) (complex 1) shows a split absorption band centred at 34,482 cm−1 (290 nm) and 31,746 cm−1 (315 nm) probably due to intraligand transitions. A shoulder is observed at 28,571 cm−1 (350 nm) assigned to ligand-to-metal charge transfer transition.
3.5. Thermogravimetric analysis of [Co(phen)2(N3)2]NO3
To examine the thermal stability of the complex 2, thermal gravimetric (TG) analysis was carried out. This analysis (Figure ) shows that the complex is thermally stable up to 250°C and upon further heating, it decomposes in a single step from 300 to 420°C. The major weight loss (~83%) takes place rapidly at 300°C, attributed to the complete decomposition of the sample (calc. 89.6%). A stable mass is reached at 460°C.
Caution! Azide complexes of metals with organic ligands are potentially explosive and should be handled with care.
3.6. Antimicrobial tests
The potency of the metal salt, 1,10-phen, N3− and the complexes together with the reference antibacterial drug (Gentamycin) and antifungal drug (Nystatin) were evaluated against four bacteria and four fungi strains. The diameter of the zone of inhibition (mm) was used to compare the antimicrobial activity of the test compound with that of the reference drug. Results of the antimicrobial activity are presented in Table and histograms shown in Figures –.
Table 5. Diameter of zone of inhibition of the complex, ligands and the metal salt
The metal salt, 1,10-phen and azide showed considerable antimicrobial activity against the micro-organisms. The ligand 1,10-phen was very active against all the fungi and bacteria species tested. The metal salt and azide were more active against bacteria species while 1,10-phen showed higher activity against the fungi. Coordination of the ligands to the metal ion impacted the antimicrobial activity against all fungi and bacteria species tested with inhibition zone diameters in the range 13–38 mm. Complex 1 showed higher activity against the bacteria species while complex 2 exhibited higher activities against the fungi species. The complexes were generally more potent towards the fungi species than the reference antifungal (nystatin). The complexes exhibited highest activity against the fungus C. albicans ATCC P37039, which can lead to the development of vagina candidiasis. Compared to the reference antibiotic gentamicin, the complexes showed comparable antibacterial activity against the bacteria species E. coli and P. aeruginosa. These results are comparable to those of the complex [azido-bis(1,10-phen-κ2 N,N′)copper(II)]nitrate hydrate, [Cu(C12H8N2)2N3]NO3·H2O (Sado et al., Citation2016).
The complexes are also more active than the reference drug nystatin towards the fungi species, with complex 2 being most active. This indicates that reaction of metal ions with the ligands plays an important role in enhancing its antimicrobial activity. This increase in activity could be due to the reduction of the polarity of the metal ion by partial sharing of the positive charge with the ligand’s donor atoms so that there is electron delocalization within the metal complex. This may increase the hydrophobic and lipophilic character of the metal complex, enabling it to permeate the lipid layer of the organism killing them more effectively (Amah et al., Citation2015; Chohan, Munawar, & Supuran, Citation2001).
The antimicrobial activities of the complexes were quantified by the determination of their MICs. The MIC indicates the lowest drug concentration at which a visible inhibition growth of the micro-organisms is noticed. The values for the different pathogens were determined by using the microbroth dilution test. The MICs values for the complexes are summarized in Table and the histograms on Figure .
Table 6. MIC (mg/mL) of the complexes
The MIC values indicate that the ligands (1,10-phen and azide) have higher activity against the bacteria species than the fungi, with 1,10-phen being more active than azide. The complexes have activities higher than that of the metal salt but lower than that of 1,10-phen, towards the micro-organisms. The most sensitive strains are the bacteria species E. coli, P. aeruginosa, S. typhi and the fungi C. albicans ATCC P37037 and C. neoformans. The complexes have a broad spectrum of activity, since they showed high activity towards all the tested strains. The MIC values obtained are lower than those of a copper(II) complex against the same micro-organisms, indicating that the cobalt complexes synthesized are more potent (Yenikaya et al., Citation2009). Comparison of the MIC values of the complexes with that of the reference drugs indicates that the complexes are potentially more active than the reference drugs. The disparity in the effectiveness of the complexes against the tested pathogens depends either on the impermeability of the cells of the microbes or the difference in the ribosomes of the microbial cells (Yenikaya et al., Citation2009).
4. Conclusion
A novel mixed ligand Co(III) complex with 1,10-phen and azide co-ligands has been reported. The equatorial and terminal azide ligands coordinate in a non-linear manner to the central metal ion while two 1,10-phen ligands coordinate to the Co(III) ion through four N-atoms, two axial and two equatorial. The Co(III) ion in the complex adopts a slightly distorted octahedral environment comprising four phenanthroline N-atoms and two nitrile N-atoms from the azides. The molecular structure of the compound is consolidated by weak C-H … O and C-H … N intermolecular interactions and face-to face π–π stacking between the aromatic rings of 1,10-phen ligand. The results of the preliminary antimicrobial screening against four pathogenic bacteria and four fungi species indicates that the complexes are very active and could be further screened in vitro against a wide range of pathogens. The MIC values indicate that the ligands (1,10-phen and azide) have higher activity against the bacteria species than the fungi, with 1,10-phen being more active than azide. The complexes have activities higher than that of the metal salt but lower than that of 1,10-phen, towards the micro-organisms. The most sensitive strains are the bacteria species E. coli, P. aeruginosa, S. typhi and the fungi C. albicans ATCC P37037 and C. neoformans. The generally positive results of the antimicrobial screening against four bacteria and four fungi species suggest that complex 1 is more active towards the bacteria, while complex 2 is more active towards the fungi. Both complexes (1 and 2) may represent good candidates as an antibacterial and antifungal agent, respectively. However, additional and profound in vitro antimicrobial studies mainly in relation to elucidation of the mechanism of growth inhibition and toxicity of the complexes is ongoing.
Funding
AMO and DMY acknowledge the Government of Cameroon for financial support through the Fonds d’Appuis à la Recherche.
Acknowledgement
The authors thank the Laboratory of Phytobiochemical and Medicinal Plant Study, University of Yaounde I for the antimicrobial tests.
Additional information
Notes on contributors
Moise Ondoh Agwara
Moise Ondoh Agwara, PhD, is Associate Professor of Chemistry at the Department of Inorganic Chemistry, University of Yaounde I in Cameroon. He obtained a PhD in chemistry from the University of Ibadan, Nigeria in 1986. Research activities within his research group are focused on the development of the chemistry of transition metal complexes with heterocyclic N-, O- and N,O-donor ligands and some co-ligands. Such interest derives from the fascinating structural chemistry of the complexes obtained, their interesting physico-chemical properties and their diverse applications such as antimicrobials, in photoluminescence and as precursors for the development of nanostructured functional materials.
References
- Abdelhak, J., Namouchi Cherni, S., Amami, M., El Kébir, H., Zid, M. F., & Driss, A. (2014). Iron(III) and cobalt(III) complexes with oxalate and phenanthroline: synthesis, crystal structure, spectroscopy properties and magnetic properties. Journal of Superconductivity and Novel Magnetism, 27, 1693–1700. doi:10.1007/s10948-014-2479-2
- Adhikary, C., & Koner, S. (2010). Structural and magnetic studies on copper(II) azido complexes. Coordination Chemistry Reviews, 254, 2933–2958. doi:10.1016/j.ccr.2010.06.001
- Ahmed, S. K., & Khaled, S. (2015). Syntheses, spectral characterization, thermal properties and DNA cleavage studies of a series of Co(II), Ni(II) and Cu(II) polypyridine complexes with some new imidazole derivatives of 1,10-phenanthroline. Arabian Journal of Chemistry. doi:10.1016/j.arabjc.2015.04.025
- Amah, C., Ondoh, A. M., Yufanyi, D. M., & Gaelle, D. S. Y. (2015). Synthesis, crystal structure and antimicrobial properties of an anhydrous copper(ii) complex of pyridine-2-carboxylic acid. International Journal of Chemistry, 7, 10–20. doi:10.5539/ijc.v7n1p10
- Amani, V., Safari, N., Khavasi, H. R., & Mirzaei, P. (2007). Iron(III) mixed-ligand complexes: Synthesis, characterization and crystal structure determination of iron(III) hetero-ligand complexes containing 1,10-phenanthroline, 2,2′-bipyridine, chloride and dimethyl sulfoxide, [Fe(phen)Cl3(DMSO)] and [Fe(bipy)Cl3(DMSO)]. Polyhedron, 26, 4908–4914. doi:10.1016/j.poly.2007.06.038
- Arounaguiri, S., Easwaramoorthy, D., Ashokkumar, A., Dattagupta, A., & Maiya, B. G. (2000a). Cobalt(III), nickel(II) and ruthenium(II) complexes of 1,10-phenanthroline family of ligands: DNA binding and photocleavage studies. Journal of Chemical Sciences, 112(1), 1–17. doi:10.1007/bf02704295
- Arounaguiri, S., Easwaramoorthy, D., Ashokkumar, A., Dattagupta, A., & Maiya, B. G. (2000b). Cobalt(III), nickel(II) and ruthenium(II) complexes of 1,10-phenanthroline family of ligands: DNA binding and photocleavage studies. Proceedings of the Indian Academy of Sciences (Chemical Sciences), 112(1), 1–17. 10.1007/BF02704295
- Batten, S. R., & Murray, K. S. (2003). Structure and magnetism of coordination polymers containing dicyanamide and tricyanomethanide. Coordination Chemistry Reviews, 246, 103–130.10.1016/S0010-8545(03)00119-X
- Bencini, A., & Lippolis, V. (2010). 1,10-Phenanthroline: A versatile building block for the construction of ligands for various purposes. Coordination Chemistry Reviews, 254, 2096–2180. doi:10.1016/j.ccr.2010.04.008
- Biagetti, R. V., & Haendler, H. M. (1966). Pyridine complexes of cobalt(II) and nickel(II) nitrates. Inorganic Chemistry, 5, 383–386.10.1021/ic50037a012
- Bruker AXS. (2004). APEX2, SAINT-Plus and XPREP. Madison, WI: Author.
- Chen, Z.-L., Jiang, C.-F., Yan, W.-H., Liang, F.-P., & Batten, S. R. (2009). Three-dimensional metal azide coordination polymers with amino carboxylate coligands: Synthesis, structure, and magnetic properties. Inorganic Chemistry, 48, 4674–4684. doi:10.1021/ic802026n
- Chohan, Z. H., Munawar, A., & Supuran, C. T. (2001). Transition metal ion complexes of schiff-bases. Synthesis, characterization and antibacterial properties. Metal Based Drugs, 8, 137–143. doi:10.1155/MBD.2001.137
- Deegan, C., McCann, M., Devereux, M., Coyle, B., & Egan, D. A. (2007). In vitro cancer chemotherapeutic activity of 1,10-phenanthroline (phen), [Ag2(phen)3(mal)]·2H2O, [Cu(phen)2(mal)]·2H2O and [Mn(phen)2(mal)]·2H2O (malH2=malonic acid) using human cancer cells. Cancer Letters, 247, 224–233. doi:10.1016/j.canlet.2006.04.006
- Goher, M. A. S., Hafez, A. K., Abu-Youssef, M. A. M., Badr, A. M. A., Gspan, C., & Mautner, F. A. (2004). New metal(II) complexes containing monodentate and bridging 3-aminopyridine and azido ligands. Polyhedron, 23, 2349–2356. doi:10.1016/j.poly.2004.06.011
- Goher, M. A. S., & Mautner, F. A. (1995). New unexpected coordination modes of azide and picolinato anions acting as bridging ligands between copper(II) and sodium or potassium ions. Synthesis, crystal structures and spectral characterizations of [MCu(picolinato)(N3)2]n (M = Na or K) complexes. Polyhedron, 14, 1439–1446.10.1016/0277-5387(94)00415-B
- Gopinathan, H., & Arumugham, M. N. (2015). Larvicidal activity of synthesized copper(II) complexes against Culex quinquefasciatus and Anopheles subpictus. Journal of Taibah University for Science, 9, 27–33. doi:10.1016/j.jtusci.2014.04.008
- Gopinathan, H., Komathi, N., & Arumugham, M. N. (2014). Synthesis, structure, DNA binding, cleavage and biological activity of cobalt (III) complexes derived from triethylenetetramine and 1,10 phenanthroline ligands. Inorganica Chimica Acta, 416, 93–101. doi:10.1016/j.ica.2014.03.015
- Guo, Y., Shi, T., Si, Z., Duan, Q., & Shi, L. (2013). Novel magnetic CoII complexes: Synthesis and characterization. Inorganic Chemistry Communications, 34, 15–18. doi:10.1016/j.inoche.2013.04.039
- Hawkey, P. M., & Jones, A. M. (2009). The changing epidemiology of resistance. Journal of Antimicrobial Chemotherapy, 64(Supplement 1), i3–i10. doi:10.1093/jac/dkp256
- Janiak, C. (2000). A critical account on π–π stacking in metal complexes with aromatic nitrogen-containing ligands †. Journal of the Chemical Society, Dalton Transactions, 3885–3896.10.1039/b003010o
- Jennifer, S. J., & Muthiah, P. T. (2014). Synthesis, crystal structures and supramolecular architectures of square pyramidal Cu(II) complexes containing aromatic chelating N,N′-donor ligands. Chemistry Central Journal, 8, 42. Retrieved from http://journal.chemistrycentral.com/content/8/1/4210.1186/1752-153X-8-42
- Kou, Y.-Y., Tian, J.-L., Li, D.-D., Liu, H., Gu, W., & Yan, S.-P. (2009). Oxidative DNA cleavage by Cu(II) complexes of 1,10-phenanthroline-5,6-dione. Journal of Coordination Chemistry, 62, 2182–2192. doi:10.1080/00958970902763271
- Kumar, R. S., Arunachalam, S., Periasamy, V. S., Preethy, C. P., Riyasdeen, A., & Akbarsha, M. A. (2008). Synthesis, DNA binding and antitumor activities of some novel polymer-cobalt(III) complexes containing 1,10-phenanthroline ligand. Polyhedron, 27, 1111–1120. doi:10.1016/j.poly.2007.12.008
- Lazari, G., Stamatatos, T. C., Raptopoulou, C. P., Psycharis, V., Pissas, M., Perlepes, S. P., & Boudalis, A. K. (2009). A metamagnetic 2D copper(II)-azide complex with 1D ferromagnetism and a hysteretic spin-flop transition. Dalton Transactions, 3215–3221, doi:10.1039/b823423j
- Lever, A. B. P. (1984). Inorganic electronic spectroscopy (2nd ed.). Netherlands: Elsevier Science.
- Liang, M., Wang, W.-Z., Liu, Z.-Q., Liao, D.-Z., Jiang, Z.-H., Yan, S.-P., & Cheng, P. (2003). A new mixed-ligand copper(II) complex containing azide and 1,10-phenanthroline: crystal structure and properties. Journal of Coordination Chemistry, 56, 1473–1480. doi:10.1080/00958970310001617058
- Lu, L.-P., Zhu, M.-L., & Yang, P. (2003). Crystal structure and nuclease activity of mono (1,10-phenanthroline) copper complex. Journal of Inorganic Biochemistry, 95, 31–36. doi:http://dx.doi.org/10.1016/S0162-0134(03)00049-710.1016/S0162-0134(03)00049-7
- McCann, M., Geraghty, M., Devereux, M., O’Shea, D., Mason, J., & O’Sullivan, L. (2000). Insights into the mode of action of the anti-candida activity of 1,10-phenanthroline and its metal chelates. Metal Based Drugs, 7, 185–193.10.1155/MBD.2000.185
- Meundaeng, N., Prior, T. J., & Rujiwatra, A. (2013). Bis(1,10-phenanthroline-κ 2 N, N′)(sulfato-κ O )copper(II) ethanol monosolvate. Acta Crystallographica Section E: Structure Reports Online, 69, m568–m569. doi:10.1107/s1600536813026093
- Mishra, A., Kaushik, N. K., Verma, A. K., & Gupta, R. (2008). Synthesis, characterization and antibacterial activity of cobalt(III) complexes with pyridine-amide ligands. European Journal of Medicinal Chemistry, 43, 2189–2196. doi:10.1016/j.ejmech.2007.08.015
- Molphy, Z., Slator, C., Chatgilialoglu, C., & Kellett, A. (2015). DNA oxidation profiles of copper phenanthrene chemical nucleases. Frontiers in Chemistry, 3, 28. doi:10.3389/fchem.2015.00028
- Ndosiri, N., Agwara, M., Paboudam, A., Ndifon, P., Yufanyi, D., & Amah, C. (2013). Synthesis, charaterization and antifungal activities of Mn(II), Co(II), Cu(II) and Zn(II) mixed-ligand complexes containing 1,10-phenanthroline and 2,2-bipyridine. Research Journal of Pharmaceutical, Biological and Chemical Sciences, 4, 386–397.
- Pivetta, T., Isaia, F., Verani, G., Cannas, C., Serra, L., Castellano, C., & Demartin, F. (2012). Mixed-1,10-phenanthroline Cu(II) complexes: Synthesis, cytotoxic activity versus hematological and solid tumor cells and complex formation equilibria with glutathione. Journal of Inorganic Biochemistry, 114, 28–37. doi:10.1016/j.jinorgbio.2012.04.017
- Pook, N.-P., Hentrich, P., & Gjikaj, M. (2015). Crystal structure of bis[tris(1,10-phenanthroline k2 N,N’)cobalt(II)] tetranitrate N,N’-(1,4-phenylenedicarbonyl)diglycine solvate octahydrate. Acta Cryst, E71, 910–914. doi:10.1107/S2056989015013006
- Rajarajeswari, C., Ganeshpandian, M., Palaniandavar, M., Riyasdeen, A., & Akbarsha, M. A. (2014). Mixed ligand copper(II) complexes of 1,10-phenanthroline with tridentate phenolate/pyridyl/(benz)imidazolyl Schiff base ligands: Covalent vs non-covalent DNA binding, DNA cleavage and cytotoxicity. Journal of Inorganic Biochemistry, 140, 255–268. doi:10.1016/j.jinorgbio.2014.07.016
- Richers, C. P., Bertke, J. A., & Rauchfuss, T. B. (2015). Crystal structure of di-m-hydroxido-k4O:O-bis-[bis(acetylacetonato-k2O,O’)cobalt(III)]. Acta Crystallographica, E71, 983–985. doi:10.1107/S2056989015013663
- Sado, D. Y. G., Agwara, M. O., Yufanyi, M. D., Nenwa, J., & Jagan, R. (2016). Crystal structure and antimicrobial properties of a copper(II) complex with 1,10-phenanthroline and azide co-ligand. Synthesis and Reactivity in Inorganic, Metal-Organic, and Nano-Metal Chemistry, 00–00, doi:10.1080/15533174.2016.1212220
- Shaabani, B., Khandar, A. A., Dusek, M., Pojarova, M., & Mahmoudi, F. (2013). Synthesis, crystal structure, antimicrobial activity and electrochemistry study of chromium(III) and copper(II) complexes based on semicarbazone Schiff base and azide ligands. Inorganica Chimica Acta, 394, 563–568. doi:10.1016/j.ica.2012.08.027
- Sheldrick, G. M. (2008). A short history of SHELX. Acta Crystallographica Section A, 64, 112–122.
- Silva, P. P., Guerra, W., Silveira, J. N., Ferreira, A. M. D. C., Bortolotto, T., Fischer, F. L., & Terenzi, H. (2011). Two New Ternary Complexes of Copper(II) with Tetracycline or Doxycycline and 1,10-Phenanthroline and Their Potential as Antitumoral: Cytotoxicity and DNA Cleavage. Inorganic Chemistry, 50, 6414–6424. doi:10.1021/ic101791r
- Spellberg, B., Guidos, R., Gilbert, D., Bradley, J., Boucher, H. W., Scheld, W. M., & Infectious Diseases Society of America (2008). The epidemic of antibiotic-resistant infections: A call to action for the medical community from the infectious diseases society of America. Clinical Infectious Diseases, 46, 155–164. doi:10.1086/524891
- Tabong, C. D., Yufanyi, D. M., Paboudam, A. G., Nono, K. N., Eni, D. B., & Agwara, M. O. (2016). Synthesis, Crystal structure, and antimicrobial properties of [diaquabis(hexamethylenetetramine)diisothiocyanato-kn]nickel(ii) complex. Advances in Chemistry, 2016, 8. Article ID 5049718. doi:http://dx.doi.org/10.1155/2016/5049718
- Tanwar, J., Das, S., Fatima, Z., & Hameed, S. (2014). Multidrug resistance: An emerging crisis. Interdisciplinary Perspectives on Infectious Diseases, 2014, 7. Article ID 541340. doi:http://dx.doi.org/10.1155/2014/541340
- Wang, Q., Huang, M., Huang, Y., Zhang, J.-S., Zhou, G.-F., Zeng, R.-Q., & Yang, X.-B. (2014). Synthesis, characterization, DNA interaction, and antitumor activities of mixed-ligand metal complexes of kaempferol and 1,10-phenanthroline/2,2′-bipyridine. Medicinal Chemistry Research, 23, 2659–2666. doi:10.1007/s00044-013-0863-2
- Weinstein, R. A., & Fridkin, S. K. (2003). Routine cycling of antimicrobial agents as an infection-control measure. Clinical Infectious Diseases, 36, 1438–1444. doi:10.1086/375082
- WHO. (2014). Antimicrobial resistance: Global report on surveillance. Geneva: Author.
- Ye, M.-D., Cheng, Y.-Q., Li, X.-H., & Hu, M.-L. (2004). Crystal structure of bis[di(1,10-phenanthroline)azidocopper(II)] biphenyl-4,4’-dicarboxylate pentahydrate, [Cu(C12H8N2)2N3]2(C14H8O4)·5H2O. Zeitschrift für Kristallographie, NCS219, 165–167.
- Yenikaya, C., Poyraz, M., Sarı, M., Demirci, F., İlkimen, H., & Büyükgüngör, O. (2009). Synthesis, characterization and biological evaluation of a novel Cu(II) complex with the mixed ligands 2,6-pyridinedicarboxylic acid and 2-aminopyridine. Polyhedron, 28, 3526–3532. doi:10.1016/j.poly.2009.05.079
- Yesilel, O. Z., Olmez, H., Yılan, O. O., Pasaoglu, H., & Buyukgungor, O. (2006). Syntheses, spectral and thermal studies, and crystal structure of 1,10-phenanthroline and picolinamide complexes of cobalt(II) squarate. Z. Naturforsch, 61b, 1094–1100. Retrieved from http://znaturforsch.com
- Yuoh, A. C. B., Agwara, M. O., Yufanyi, D. M., Conde, M. A., Jagan, R., & Eyong, K. O. (2015). Synthesis, crystal structure, and antimicrobial properties of a novel 1-d cobalt coordination polymer with dicyanamide and 2-aminopyridine. International Journal of Inorganic Chemistry, 2015, 8. Article ID 106838. doi:10.1155/2015/106838