Abstract
Camptothecin (CPT), a modified monoterpene indole alkaloid, is a potential anticancer drug, and due to high demand, search for its new plant-based sources is a priority. Genus Ophiorrhiza is a candidate group in the search for new resources of CPT. Here, CPT contents in 38 Ophiorrhiza accessions, belonging to 11 species and 3 varieties, collected from the southern Western Ghats region in India were quantified by HPTLC-densitometry. Ophiorrhiza mungos (396.54 μg/g, dr. wt.) and O. mungos var. angustifolia (373.19 μg/g, dr. wt.) were the two best CPT sources among the screened species/varieties. O. rugosa var. decumbens (18.55 μg/g, dr. wt.) and O. hirsutula (17.14 μg/g, dr. wt.) showed moderate contents of CPT. This is the first systematic CPT screening of O. hirsutula, O. barnesii, O. incarnata, O. radicans and O. villosa. This study shows the significance of choosing high CPT-yielding ecotypes/chemotypes of Ophiorrhiza species or varieties for commercial purposes.
Public Interest Statement
Camptothecin (CPT), a modified monoterpene indole alkaloid, is a potential anticancer drug. High demand of CPT as a drug necessitates its supply demand equilibrium, and prompts to search for its new natural sources. Genus Ophiorrhiza is a candidate group in the search for new resources of CPT. A total of 82 accessions (phase I 44, phase II 38) of 14 species and 3 varieties in genus Ophiorrhiza collected from the southern Western Ghats region in India were screened for CPT using HPTLC-densitometry. We found O. mungos and O. mungos var. angustifolia as the two best sources of CPT in genus Ophiorrhiza. Some of the screened Ophiorrhiza species/varieties showed zero or non-detectable levels of CPT. This study shows the significance of choosing high CPT-yielding ecotypes/chemotypes of Ophiorrhiza species or varieties for commercial purposes.
1. Introduction
Camptothecin (CPT) (Figure ), a topoisomerase inhibitor, was first isolated from Camptotheca acuminata in 1958 (Wall et al., Citation1966), and it became a potential anticancer drug through a series of clinical trials (Hsiang, Hertzberg, Hecht, & Liu, Citation1985; Li, Zu, Shi, & Yao, Citation2006; Liu et al., Citation2000; Pommier, Citation2006). However, low aqueous solubility and toxicity of CPT led to its temporary withdrawal from the market, and semisynthetic analogs replaced it (Khazir, Mir, Pilcher, & Riley, Citation2014; van Hattum et al., Citation2002). Newer semisynthetic CPT analogs such as Karenitecin, Diflomotecan, and Gimatecan are in various stages of clinical trials against advanced stages of solid tumors (Garcia-Carbonero & Supko, Citation2002; Zunino & Pratesi, Citation2004). Recent clinical studies revealed two other CPT analogs Elomotecan and DRF-1042 as potent drugs against various tumors (Khazir et al., Citation2014). Natural CPT derivatives 9-methoxy CPT and 10-hydroxy-CPT were also isolated (Lorence & Nessler, Citation2004), and both them are water soluble with antitumor potentials.
Recent advances in therapeutic strategies using target oriented drug delivery systems made CPT a major candidate in the treatment of multi-drug resistant cancers (Porta et al., Citation2013; Tang, Cao, & Cheng, Citation2014). Newer drug delivery with liposomal and copolymer vehicle mediated systems drastically improved the safety and efficacy of CPT (Bissett et al., Citation2004; Khazir et al., Citation2014; Wachters et al., Citation2004). CPT release mediated by pH-sensitive block copolymers was also reported recently (Luo, Yang, Xu, Chen, & Zhao, Citation2014). Stem cell-based research in apoptogenic signaling of CPT is also in progress (García et al., Citation2014). This could stimulate CPT-based applications in regenerative medicine. In addition to its basic anticancer properties, CPT is also used for other pharmacological activities (López-Meyer, Nessler, & McKnight, Citation1994).
CPT has been isolated from various plants such as C. acuminata, C. lowreyana, C. yunnanensis, Nothapodytes nimmoniana, Pyrenacantha klaineana, Merrilliodendron megacarpum, Ervatamia heyneana, Mostuea brunonis, O. mungos, O. pumila, and O. filistipula (Lorence & Nessler, Citation2004). Due to its demand, the search for alternative, viable plant-based sources of CPT is a priority. Cultivable and tissue culture multipliable plants are the priority sources of CPT. Ophiorrhiza is a candidate group in this resource search for CPT (Renjith et al., Citation2013). Genus Ophiorrhiza belongs to the family Rubiaceae and is represented by 47 species and 9 varieties in the Indian subcontinent (Deb & Mondal, Citation2001). Sixteen species and 3 varieties are reported from the southern Western Ghats region in India (Joseph & Joseph, Citation2009; Sasidharan, Citation2004). In the first phase of this work, we reported CPT contents in 44 accessions belonging to 9 Ophiorrhiza species, and 3 varieties (Renjith et al., Citation2013). HPTLC–densitometry is one of the techniques useful in chemical profiling of plant extracts and quantification of the bioactive molecules in them (Haridas, Rajani, Mathew, & Sabulal, Citation2015; Renjith et al., Citation2013; Thomas et al., Citation2010). CPT contents in plant sources (C. acuminata) and in their tissue cultured systems were also analyzed by HPLC-based techniques (López-Meyer et al., Citation1994; Sankar-Thomas & Lieberei, Citation2011). Here, in the second phase of this screening program, we report the quantification of CPT contents in 38 accessions of Ophiorrhiza, collected from southern Western Ghats, belonging to 11 species and 3 varieties by HPTLC-densitometry.
2. Results and discussion
CPT peaks (Rf 0.42 ± 0.01, n = 20) in Ophiorrhiza extracts were well resolved from other signals (Figure ). CPT contents (Table ) in Ophiorrhiza extracts were determined by means of the calibration plot, y = (2 × 106 + 06) × + 67.12, R2 = 0.999, and linearity of the calibration curve in the range 0.0001–0.004 μg was ensured. On repeated measurements, mean percentage recovery observed for CPT was 100.10 ± 0.72 (Table ). % Residual Standard Deviations (RSD) (for 20 accessions, n = 6) were determined as 1.02. Limit of detection (LOD, 25 accessions, n = 6) and limit of quantification (LOQ, 25 accessions, n = 6) were determined for CPT as 0.000085 and 0.000258 μg, respectively.
Figure 2. HPTLC profile of the (a) MeOH extract of O. mungos (52748) collected from Brymore, Thiruvananthapuram in Kerala, (b) CPT standard (0.003 μg/spot).
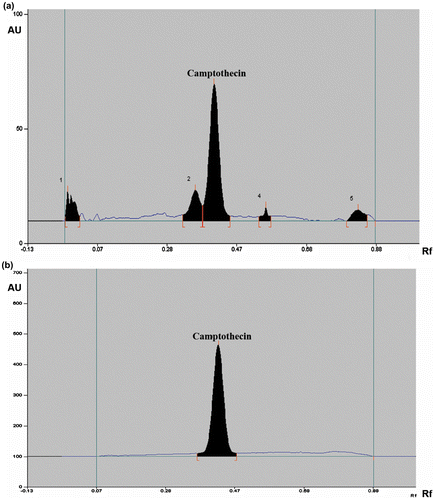
Table 1. Estimation of CPT in Ophiorrhiza species/varieties by HPTLC-densitometry
Table 2. Recovery data
CPT contents (μg/g, dr. wt.) in 38 accessions of 11 species and 3 varieties of Ophiorrhiza collected from various locations in Western Ghats are given in Table . O. mungos (396.54 μg/g, dr. wt.) and O. mungos var. angustifolia (373.19 μg/g, dr. wt.) were the two best sources of CPT among the species/varieties screened. O. mungos (O. mungos var. mungos) is a herb of 8–100 cm height. O. mungos var. angustifolia is a smaller herb of 20–35 cm height, and it is a better candidate for cultivation and tissue culture based multiplication (Renjith et al., Citation2013). O. mungos and O. mungos var. angustifolia showed distinctly different chemical profiles on HPTLC under identical conditions (Renjith et al., Citation2013; Figure ), justifying their taxonomic status (Deb & Mondal, Citation2001). O. rugosa var. decumbens (18.55 μg/g, dr. wt.) and O. hirsutula (17.14 μg/g, dr. wt.) showed moderate CPT contents. Various Ophiorrhiza species (or varieties) showed significant intra and inter species variations in their CPT contents. The present data clearly support our previous report of CPT contents in species/varieties such as O. mungos, O. mungos var. angustifolia, O. eriantha, O. grandiflora, O. pectinata, O. trichocarpon, and O. caudata (Renjith et al., Citation2013). This study is the first systematic CPT screening in O. barnesii, O. hirsutula, O. incarnata, O. radicans, and O. villosa. The four first-time screened Ophiorrhiza species (O. barnesii, O. incarnata, O. radicans, O. villosa) and two other species (O. caudata, O. grandiflora) showed zero or non-detectable levels of CPT (Table ). Ophiorrhiza species with zero or near-zero levels of CPT can be ruled out as its primary sources.
The integrity of the analyte (CPT) (Figure ) was confirmed by its isolation from the MeOH extract of O. mungos var. angustifolia and spectral characterization. A pale yellow powder, IR (λmax, cm−1): 3430, 1737, 1650, 1600, 1579, 1437, 1156, 1040; 1H NMR (δ): 5.28 (H-5), 8.66 (H-7), 8.10 (d, H-9, J = 8.0), 7.70 (t, H-10, J = 7.2), 7.85 (t, H-11, J = 7.6), 8.16 (d, H-12, J = 8.4), 7.37 (s, H-14), 5.41 (dd, H-17 J = 16.4, 23.4), 0.90 (t, H-18, J = 7.2), 1.89 (H-19), 6.30 (H-OH); 13C NMR (δ): 152.6 (C2), 145.5 (C3), 50.1 (C5), 129.7 (C6), 131.5 (C7), 128.0 (C8), 128.4 (C9), 127.5 (C10), 130.26 (C11), 129.0 (C12), 148.1 (C13), 96.7 (C14), 150.0 (C15), 119.1 (C16), 156.8 (C16a), 65.3 (C17), 7.7 (C18), 30.7 (C19), 72.4 (C20), 172.2 (C21); LC-EI-MS, m/z: 349.52 (M + H+). These spectral data are in good agreement with literature reports (Krishnan, Dileepkumar, Nair, & Oommen, Citation2014; Rehman et al., Citation2009). CPT standard and isolated CPT showed exactly similar Rf values on HPTLC. On relative distribution analysis, root (403.64 μg/g, dr. wt.) of O. mungos var. angustifolia showed highest CPT content compared to its stem (216.35 μg/g, dr. wt.) and leaves (192.47 μg/g, dr. wt.) (Table ). But in C. acuminata highest CPT content was found in its leaves (0.4–0.5%, dr. wt.) compared to its seeds and bark (López-Meyer et al., Citation1994).
Table 3. Distribution of camptothecin in different parts of O. mungos var. angustifolia
3. Experimental
3.1. Plant materials
Thirty-eight accessions of 11 Ophiorrhiza species viz., Ophiorrhiza barnesii C.E.C. Fisch. (1 accession), O. caudata C.E.C. Fisch (1), O. eriantha Wight (3), O. grandiflora Wight (1), O. hirsutula Wight ex Hook.f. (1), O. incarnata C.E.C. Fisch. (1), O. mungos L. (8), O. pectinata Arn. (6), O. radicans Gardn. (1), O. trichocarpon Blume (3), O. villosa Roxb. (1), and three varieties viz., O. mungos L. var. angustifolia (Thwaites) Hook. f. (4), O. rugosa Wall. var. decumbens Gardner ex Thwaites (1), O. rugosa Wall. var. prostrata (D. Don) Deb & Mondal (6) were collected (January–April 2013) from the southern Western Ghats region in India. Ophiorrhiza specimens were identified by Dr K. Satheesh Kumar, one of the authors, and voucher specimens were deposited at the Institute Herbarium (TBGT) (Table ).
3.2. Extraction
Shade dried, powdered plant materials (whole plants, 38 accessions, 10 g each) were Soxhlet extracted (separately) using 200 ml MeOH for 6 h. In order to find the relative CPT distribution, O. mungos var. angustifolia root, stem and leaves (52734; 10 g each) were Soxhlet extracted (separately) with MeOH (200 ml, 6 h). After extraction, solvents were fully removed using a rotary evaporator (Buchi, Switzerland) and yields of extracts (w/w) were recorded.
3.3. HPTLC analysis
Quantification of CPT in Ophiorrhiza (whole plant) extracts was carried out using an HPTLC (CAMAG, Switzerland) made up of Linomat V sample applicator, twin trough plate development chamber, TLC Scanner 3, and WinCATS Software 4.03 (Renjith et al., Citation2013). Briefly, Ophiorrhiza extracts dissolved in MeOH were filtered through nylon 0.45 μ membrane filters (PALL Gelman Laboratory, India). Filtered extracts of O. mungos, O. mungos var. angustifolia, O. mungos var. angustifolia (52734, root, stem and leaf extracts) (5 μl or 0.5 μg each), O. trichocarpon (10 μl or 10 μg, 20 μl or 200 μg) and other Ophiorrhiza species (20 μl or 200 μg each) were applied onto silica gel HPTLC plates (60F-254, E. Merck, Germany, 20 × 10 cm, 0.2 mm thickness) as 6 mm wide bands with the automatic Linomat V sample applicator fitted with a micro syringe in N2 flow (application rate—150 nl/s, space between two bands—11 mm, slit dimension—6 × 0.45 mm, scanning speed—20 mm/s). CPT standard (Sigma Aldrich, India) was also applied along with the Ophiorrhiza extracts. Plates were developed up to 80 mm in 20 ml of EtOAc:CHCl3:MeOH (5:4.5:0.5, v/v) mobile phase in the twin trough glass chamber, under saturated conditions (30 min). These plates were scanned densitometrically at 366 nm (tungsten lamp) using TLC Scanner 3 and the data were analyzed using WinCATS Software 4.03 (Table ).
3.4. Data analysis, validation
HPTLC method was validated in terms of accuracy, precision, repeatability, and linearity (Fischedick, Glas, Hazekamp, & Verpoorte, Citation2009; Reich & Schibli, Citation2007; Renjith et al., Citation2013; Thomas et al., Citation2010). CPT contents (Table ) in Ophiorrhiza extracts were determined by means of the calibration plot, y = (2 × 106 + 06) x + 67.12, R2 = 0.999, made of standard CPT (at 0.001 μg/μl in MeOH). Linearity of CPT calibration curve in the range 0.0001–0.004 μg was ensured. Specificity was tested by repeated application of standard CPT. Rf values of CPT were reproducible and found to be same as the values observed for the CPT peak in Ophiorrhiza extracts (0.42 ± 0.01, n = 20) (Figure ). Integrity and purity of CPT peak were also tested by spectral analysis. Recovery studies were carried out by the addition of three varying concentrations of CPT to pre-analyzed Ophiorrhiza extracts (O. mungos 52753, 52755) and they were analyzed under similar conditions. Repeatability of sample application (instrumental precision) was assessed by applying an Ophiorrhiza extract on the HPTLC plate, developed under similar conditions, the same spot was scanned six times, and the % coefficient of variation was acceptable (Table ). Robustness of the method was checked by slightly altering the mobile phase composition and plate developing distance. Two different mobile phases viz., 20 ml each of EtOAc:CHCl3:MeOH (5.0:4.5:0.5, v/v) and EtOAc:CHCl3:MeOH (4.5:5.0:0.5, v/v), were used and developing distances were varied to 75, 85 and 90 cm. No considerable effect on the data (Rf values of CPT) was found. % RSD (SD of CPT area/average CPT area) × 100 (for 20 accessions, n = 6) were determined. Limit of detection (LOD, average of 3.3 × SD of CPT area/slope of calibration curve for 25 accessions, n = 6) and limit of quantification (LOQ, average of 10 × SD of CPT area/slope of calibration curve for 25 accessions, n = 6) were determined for CPT (Haridas et al., Citation2015; Renjith et al., Citation2013; Thomas et al., Citation2010). HPTLC analyses were carried out at laboratory conditions, 21 ± 0.5°C and 40% relative humidity (Barigo Humidity Meter, Germany).
Table 4. Repeatability (precision) data
3.5. Isolation, characterization of CPT
CPT (Figure ) was isolated from the MeOH extract of O. mungos var. angustifolia (whole plant) by repeated column chromatography using hexane:ethyl acetate and characterized using FT-IR (PerkinElmer, USA), 1H-, 13C-, 2D-NMR (Bruker, Germany), and LC-MS (Thermo Finnigan, USA).
4. Conclusions
High demand of CPT as a drug necessitates a supply demand equilibrium and prompts to search for its new natural sources. A total of 82 accessions (phase I 44, phase II 38) of 14 species and 3 varieties in genus Ophiorrhiza collected from the southern Western Ghats region in India were screened for CPT (Renjith et al., Citation2013). O. mungos and O. mungos var. angustifolia are the two best sources of CPT in genus Ophiorrhiza. In this study, four (O. barnesii, O. incarnata, O. radicans, O. villosa) of the five first-time screened species showed zero or non-detectable levels of CPT. These screening data can be utilized to choose elite eco-/chemotypes of Ophiorrhiza species/varieties for multiplication, and drug development.
Funding
This work was supported by Board of Research in Nuclear Sciences, Department of Atomic Energy, Government of India [grant number 2010/35/4/BRNS/1278] dated 11/08/2010.
Acknowledgments
We acknowledge Kerala Forest Department for permitting us to collect Ophiorrhiza specimens from protected forest areas. R.R. and S.C.V. are DAE-BRNS/MS University research fellows.
Additional information
Notes on contributors
Sabulal Baby
Dr Sabulal Baby is Principal Scientist and Head at the Phytochemistry and Phytopharmacology Division of Jawaharlal Nehru Tropical Botanic Garden and Research Institute at Thiruvananthapuram in India. Dr Sabulal received his doctorate in Chemistry from the Indian Institute of Technology Bombay. He did postdoctoral research at the University of Pennsylvania, USA and University of Toronto, Canada. His research areas are phytochemistry and biochemistry. He published over fifty peer reviewed papers in these research areas.
References
- Bissett, D., Cassidy, J., de Bono, J. S., Muirhead, F., Main, M., Robson, L., … Twelves, C. (2004). Phase I and pharmacokinetic (PK) study of MAG-CPT (PNU 166148): A polymeric derivative of camptothecin (CPT). British Journal of Cancer, 91, 50–55.10.1038/sj.bjc.6601922
- Deb, D. B., & Mondal, D. C. (2001). Taxonomic revision of the genus Ophiorrhiza L. (Rubiaceae) in Indian subcontinent. Bulletin of the Botanical Survey of India, 39, 1–148.
- Fischedick, J. T., Glas, R., Hazekamp, A., & Verpoorte, R. (2009). A qualitative and quantitative HPTLC densitometry method for the analysis of cannabinoids in Cannabis sativa L. Phytochemical Analysis, 20, 421–426.10.1002/pca.v20:5
- García, C. P., Videla Richardson, G. A., Romorini, L., Miriuka, S. G., Sevlever, G. E., & Scassa, M. E. (2014). Topoisomerase I inhibitor, camptothecin, induces apoptogenic signaling in human embryonic stem cells. Stem Cell Research, 12, 400–414.10.1016/j.scr.2013.12.002
- Garcia-Carbonero, R., & Supko, J. G. (2002). Current perspectives on the clinical experience, pharmacology, and continued development of the camptothecins. Clinical Cancer Research, 8, 641–661.
- Haridas, P., Rajani, K., Mathew, P. J., & Sabulal, B. (2015). Levodopa in Mucuna pruriens and its degradation. Scientific reports, 5, 11078.
- Hsiang, Y. H., Hertzberg, R., Hecht, S., & Liu, L. F. (1985). Camptothecin induces protein-linked DNA breaks via mammalian DNA topoisomerase I. Journal of Biological Chemistry, 260, 14873–14878.
- Joseph, G., & Joseph, J. P. (2009). Rediscovery of Ophiorrhiza caudata (Rubiaceae) from the Western Ghats of Kerala. Rheedea, 19, 45–46.
- Khazir, J., Mir, B. A., Pilcher, L., & Riley, D. L. (2014). Role of plants in anticancer drug discovery. Phytochemistry Letters, 7, 173–181.10.1016/j.phytol.2013.11.010
- Krishnan, S. A., Dileepkumar, R., Nair, A. S., & Oommen, O. V. (2014). Studies on neutralizing effect of Ophiorrhiza mungos root extract against Daboia russelii venom. Journal of Ethnopharmacology, 151, 543–547.10.1016/j.jep.2013.11.010
- Li, Q. Y., Zu, Y. G., Shi, R. Z., & Yao, L. P. (2006). Review camptothecin: Current perspectives. Current Medicinal Chemistry, 13, 2021–2039.10.2174/092986706777585004
- Liu, L. F., Desai, S. D., Li, T. K., Mao, Y., Sun, M., & Sim, S. P. (2000). Mechanism of action of camptothecin. Annals of the New York Academy of Sciences, 922, 1–10.
- López-Meyer, M., Nessler, C. L., & McKnight, T. D. (1994). Sites of accumulation of the antitumor alkaloid camptothecin in Camptotheca acuminata. Planta Medica, 60, 558–560.10.1055/s-2006-959571
- Lorence, A., & Nessler, C. L. (2004). Camptothecin, over four decades of surprising findings. Phytochemistry, 65, 2735–2749.10.1016/j.phytochem.2004.09.001
- Luo, Y. L., Yang, X.-L., Xu, F., Chen, Y.-S., & Zhao, X. (2014). pH-triggered PMAA-b-HTPB-b-PMAA copolymer micelles: Physicochemical characterization and camptothecin release. Colloid and Polymer Science, 292, 1061–1072.10.1007/s00396-013-3149-9
- Pommier, Y. (2006). Topoisomerase I inhibitors: Camptothecins and beyond. Nature Reviews Cancer, 6, 789–802.10.1038/nrc1977
- Porta, F., Lamers, G. E. M., Morrhayim, J., Chatzopoulou, A., Schaaf, M., den Dulk, H., … Kros, A. (2013). Folic acid-modified mesoporous silica nanoparticles for cellular and nuclear targeted drug delivery. Advanced Healthcare Materials, 2, 281–286.10.1002/adhm.v2.2
- Rehman, S., Shawl, A. S., Kour, A., Sultan, P., Ahmad, K., Khajuria, R., & Qazi, G. N. (2009). Comparative studies and identification of camptothecin produced by an endophyte at shake flask and bioreactor. Natural Product Research, 23, 1050–1057.10.1080/14786410902750944
- Reich, E., & Schibli, A. (2007). High-performance thin-layer chromatography for the analysis of medicinal plants. New York, NY: Thieme Medical Publishers Inc.10.1055/b-002-66241
- Renjith, R., Sibi, C. V., Rajani, K., Roja, G., Venkataraman, R., Satheeshkumar, K., & Sabulal, B. (2013). Search for camptothecin-yielding Ophiorrhiza species from southern Western Ghats in India: A HPTLC-densitometry study. Industrial Crops and Products, 43, 472–476.
- Sankar-Thomas, Y. D., & Lieberei, R. (2011). Camptothecin accumulation in various organ cultures of Camptotheca acuminata Decne grown in different culture systems. Plant Cell, Tissue and Organ Culture (PCTOC), 106, 445–454.10.1007/s11240-011-9942-6
- Sasidharan, N. (2004). Biodiversity documentation for Kerala. Part 6. Flowering plants. Peechi: Kerala Forest Research Institute.
- Tang, Q., Cao, B., & Cheng, G. (2014). Co-delivery of small interfering RNA using a camptothecin prodrug as the carrier. Chemical Communications, 50, 1323–1325.10.1039/C3CC47970F
- Thomas, M. T., Rajani, K., Anil, J. J., Sreeja, P. C., Mathew, P. J., Dan, M., & Sabulal, B. (2010). Elite genotypes/chemotypes, with high contents of madecassoside and asiaticoside, from sixty accessions of Centella asiatica of south India and the Andaman Islands: For cultivation and utility in cosmetic and herbal drug applications. Industrial Crops and Products, 32, 545–550.10.1016/j.indcrop.2010.07.003
- van Hattum, A. H., Hoogsteen, I. J., Schlüper, H. M. M., Maliepaard, M., Scheffer, G. L., Scheper, R. J., … Boven, E. (2002). Induction of breast cancer resistance protein by the camptothecin derivative DX-8951f is associated with minor reduction of antitumour activity. British Journal of Cancer, 87, 665–672.10.1038/sj.bjc.6600508
- Wachters, F. M., Groen, H. J., Maring, J. G., Gietema, J. A., Porro, M., Dumez, H., … van Oosterom, A. T. (2004). A phase I study with MAG-camptothecin intravenously administered weekly for 3 weeks in a 4-week cycle in adult patients with solid tumours. British journal of cancer, 90, 2261–2267.
- Wall, M. E., Wani, M. C., Cook, C. E., Palmer, K. H., McPhail, A. T., & Sim, G. A. (1966). Plant antitumor agents. I. The isolation and structure of camptothecin, a novel alkaloidal leukemia and tumor inhibitor from Camptotheca acuminata. Journal of the American Chemical Society, 88, 3888–3890.10.1021/ja00968a057
- Zunino, F., & Pratesi, G. (2004). Camptothecins in clinical development. Expert Opinion on Investigational Drugs, 13, 269–284.10.1517/13543784.13.3.269