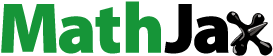
Abstract
Enrichment of carboxymethyl chitosan with zinc provides alternative means of producing aqueous soluble chitosan-based material with antimicrobial and essential trace element properties. The change in FTIR spectra of bands at 3,266 and 3,106 cm−1 of the carboxymethyl chitosan from a strong to a weak band confirmed the formation of carboxymethyl chitosan zinc supplement. From the EDX result, elemental composition of carboxymethyl chitosan is 52.89% carbon, 39.34% oxygen, 0.38% sodium, 0.54% aluminum, 2.61% calcium, and 4.19% aluminum, while carboxymethyl chitosan zinc supplement recorded 49.55% carbon, 40.40% oxygen, 1.28% sodium, 2.37% nitrogen, and 6.40% zinc. The XRD spectrum of carboxymethyl chitosan showed a lower peak intensity as compared with that of its zinc supplement. The antibacterial activities showed that carboxymethyl chitosan zinc supplement was active against all tested bacterial having recorded 6.00, 5.00, 3.00, 7.00, and 6.00 zone of inhibition (mm), respectively, against Staphylococcus aureus, Bacillus cereus, Pseudomonas syringae, Pseudomonas aeruginosa, and Escherichia coli, while carboxymetyhl chitosan was active only against S. aureus (6.00 mm). Antifungal activities revealed that carboxymethyl chitosan zinc supplement had higher zone of inhibition (mm) 42.22, 40.00, 37.78, and 48.88 mm against Collectotrichum falcritum, Rhzoctonia solani, Colletotrihum lindematianum, and Trichhoderum rubrum, while carboxymethyl chitosan recorded 26.66, 18.88, 15.88, and 22.22 respectively. The combination of aqueous solubility and antimicrobial activities of the zinc-supplemented carboxymethyl chitosan prepared should make it a good replacement for carboxymethyl chitosan in various industrial applications like food, cosmetics, biomedical, and pharmaceutical.
Public Interest Statement
Lack of certain essential mineral like zinc could cause negative developmental effect in both adult and children. This can make children prone to various infections and could affect pregnancy development in woman. Thus, this research seeks to proffer solution to zinc deficiency through the use of chitosan-based derivative-carboxymethyl chitosan zinc supplement. Metal composition of the produced products revealed that 5.40% zinc. The product showed antibacterial activities against Staphylococcus aureus, Bacillus cereus, Pseudomonas syringae, Pseudomonas aeruginosa, and Escherichia coli with 6.00, 5.00, 3.00, 7.00, and 6.00 zone of inhibition (mm), respectively. The fungicidal activities recorded 26.66, 18.88, 15.88, and 22.22 zone of inhibition (mm), respectively, against Collectotrichum falcritum, Rhzoctonia solani, Colletotrihum lindematianum, and Trichhoderum rubrum. The product could be useful in industrial applications like food, cosmetics, biomedical, and pharmaceutical.
1. Introduction
Chitosan is a natural polymer of the N-deacetylated chitin. It is the most abundant natural amino polysaccharide and the second-most abundant polysaccharide to cellulose. Chitosan has excellent properties such as biocompatibility, biodegradability, non-toxicity, and adsorption ability (Tsigos, Martinou, Kafetzopoulos, & Bouriotis, Citation2000). According to Avadi et al. (Citation2004), chitosan has a good medicinal value and chitosan-based materials also have many valuable bioactivities such as hemostatic, bacteriostatic, fungistatic, anticancer, and anticholesteremic activity. These properties make chitosan a choice material for industrial and medical application. The presence of amine and hydroxyl groups offers chitosan the opportunity for complexation with metals (Wang, Du, & Liu, Citation2004).
Of great concern to scientists these days is the adverse effect of synthetic drugs (used for human, plants, animals, environment, pests and microbes) and the deficiency of essential minerals in human nutrition. It has been established that human growth and development are directly related to the nutrition intake of some essential minerals, which have influence on various organ metabolisms (Fima, Citation2009; Mahadevan & Velavan, Citation2013).
Zinc is an essential trace element required by all living organisms. It plays critical roles as a cofactor both to stabilize proteins structurally, as well as facilitate enzymatic catalysis. It is thus not surprising that zinc is associated with various genetic traits, rare and common, that affect a multitude of physiological functions. The importance of zinc in human physiology and metabolism was identified by the consequences of zinc deficiency or metabolic disorders affecting zinc uptake (Klug, Citation2010). A total of about two billion people are suffering from zinc deficiency, leading to different types of health challenges such as diarrhea, loss of weight, the loss of appetite, long process of wound curing, abnormality of sense of taste, cognitive disorder, anorexia, retardation of growth, diminished immune response, reduced tissue regeneration, and healing after traumatic insults, as well as the occurrence of neurological disorders (Grønli, Kvamme, & Friborg, Citation2013; Hwayoung, Young, Jihye, & Jayoung, Citation2013; Osredkar & Sustar, Citation2011; Prasad, Citation2009).
The second world leading cause of child mortality is diarrhea. This could be treated effectively with zinc. Reports indicate that about 1.9 million children die annually due to diarrhea (Bryce, Boschi-Pinto, Shibuya, & Black, Citation2005). According to Bhutta et al. (Citation2000) mortality caused by diarrhea could be reduced up to 42% with the use of therapeutic zinc treatment.
Although, zinc could be found in various food sources such as nuts, seeds, legumes, and whole‐grain cereals, and is lower in tubers, refined cereals, fruits, and vegetables. However, the available zinc content in these foods is usually reduced during the processing of these agricultural products (Brown, Citation2007).
In order to reduce mortality and meet the daily zinc intake WHO‐UNICEF in 2004, recommended zinc supplements (Bryce et al., Citation2005). Zinc supplement intervention is a recent development and some trials of its effectiveness have been conducted among children suffering from diarrhea. As recorded in a Bangladesh trial, it was discovered that zinc-supplemented children had 16% faster recovery and 51% lower mortality (Baqui et al., Citation2002). Thus, research on finding low, cheap, and readily available biological material that could be embedded with zinc to reduce/carter for human zinc deficiency are currently on going.
Chitosan and zinc ions could form bond through nitrogen, oxygen, or a combination of the two, thus leaving some potential donor atoms free. These free donor atoms would make the carboxymethyl chitosan zinc supplement to exhibit high biological activity. Carboxymethyl chitosan zinc supplement would not only find useful application in food and medical industry, but would also go a long way to reduce effect of zinc deficiency in humans and animals.
Although, there are some research works on carboxymethyl chitosan zinc complex (Kong, Chen, Xing, & Park, Citation2010; Patale & Patravale, Citation2011; Song, Deng, Wu, & Liu, Citation2015; Wu et al., Citation2015), but there are no reports, to the best of our knowledge, on the elemental composition using EDX. Also, there are no previous reports on the fungicidal and antibacterial activities of this product with respect to the microbes used in this study.
The research aims to produce and investigate some instrumental properties of carboxymethyl chitosan and carboxymethyl chitosan zinc supplement, and examine the fungicidal and bacterial activities of the products.
2. Materials and methods
The brown crabs used for this research were obtained from fresh water of Odo-Osun River in Aramoko-Ekiti, Ekiti State, Nigeria, The crabs’ flesh was removed, the exoskeletons thoroughly washed, and dried in the presence of sunlight for 30 days. The dried exoskeletons were then chopped into smaller sizes and pulverized using laboratory blender. The material was sieved. A sieve size from the range of 212–249 μm was used for the experiment.
2.1. Extraction of chitosan
To extract chitosan, the method of No and Lee (Citation1995) was modified. Briefly, chitin deproteinization was done by measuring about 145 g of the chitin in a plastic container and 4% (w/v) of NaOH (solvent to solid ratio of 1:10) added. This was then placed in a thermostatically controlled water bath at 65°C for 2 h with continuous stirring. After these, the sample was filtered and washed to neutral using deionized water. The sample was oven dry at 60°C to constant weight and about 80 g sample was obtained. The deproteinated chitin (80 g) was demineralized by treating with about 10% (w/v) HCl (solvent to solid ratio of 1:10) at 65°C for 2 h with continuous stirring. Neutral demineralized sample was obtained after the treatment hours by washing with deionized water and the sample dried to constant weight at 60 °C. About 73 g sample was obtained. However, to obtain decarotenoid chitin from the demineralized sample (since there is firm bond between chitin and carotenoid pigment), the sample was treated with acetone, solid-to-liquid ratio of 1:10 (w/v) for 40 min, with continuous stirring using magnetic bar. After the reaction, the material was filtered and dried. The dried material was then bleached with 0.32% (w/v) NaOCl for 5 min at room temperature. The decolorized sample was dried and about 50 g sample was obtained. Chitosan was extracted by treating the sample (45 g) with 50% (w/v) of NaOH at 90°C for 5 h, with continuous stirring. After the reaction time, the sample was filtered, washed with hot deionized water to neutral pH, and dried in oven at 60°C, to constant weight.
2.2. Synthesis carboxymethyl chitosan (CMC) and carboxymethyl chitosan zinc supplement
In order to prepare soluble chitosan Zinc complex, the chitosan was converted to carboxymethyl chitosan by the modified method of Oluwasina, Lajide, and Owolabi (Citation2015). Chitosan 5 g was dispersed in 120 mL of isopropanol with continuous stirring for about 30 min, after which 45 mL of 15% aqueous NaOH was added, followed by an addition of 10 g of monochloroacetic acid. The reaction proceeded for about 4 h with continuous stirring. After the reaction, the solid material was obtained by filtration and the product was suspended in about 250 mL ethanol (95%) then neutralized with glacial acetic acid. The product was then washed with 70% ethanol to remove the reaction product (NaCl). The obtained solid product was suspended in acetone and stirred for about 30 min, and then oven dried at 50°C to constant weight. To produce carboxymethyl chitosan zinc supplement, the produced carboxymethyl chitosan was made to react with zinc acetate in ratio 1:1 in H2O/absolute ethanol (1:1 v/v) at room temperature for 5 h with continuous stirring. After the experiment, the product was filtered, washed with 95% warm ethanol, and dried at 40°C to constant weight.
2.3. Instrumental characterization
The structural analysis was performed to examining the change in functional groups of the samples using Fourier Transform Infrared (FTIR) (model Bruker Tensor 27) spectroscopy. The surface and elemental analysis of the samples were performed using a Scanning Electron Microscope (SEM) coupled with energy dispersive X-ray (EDX) (FEI FIB/SEM Nova 600 Nanolab). The samples were prepared for SEM by being double-coated with a palladium and gold alloy coating. The thermal stability of the materials was determined with the use of a thermogravimetric analyzer. A TGA (model Perkin Elmer STA 6000) in a nitrogen atmosphere, flow rate 20 mL min−1 at temperature between 30 and 900°C and heating rate of 10°C min−1, about 10.0 mg sample was used. The X-ray diffraction (XRD) profile of the samples was obtained using XRD (model Bruker D2 Phaser) at a voltage of 30 kV and current of 10 mA for a Cu–Kα radiation.
2.4. Antimicrobial analysis
2.4.1. Antibacterial
The antibacterial activity of the samples was determined using the agar-well diffusion method of Murray, Rosenthal, and Pfaller (Citation2006). About 20 mL of nutrient agar was dispersed into sterile Petri dish and inoculated. The plates were allowed to set and wells of 6 mm were properly bored using sterilized cork bore. The wells were filled with the extract of 0.01 mg mL−1. The plates were incubated at 37°C for 24 h. The zones of inhibition were measured with Vernier calipers in mm and recorded appropriately. The experiment was done in triplicate and mean values reported. Streptomycin sulfate and ciprofloxacin were used as positive control.
2.4.2. Antifungal
Poisoned food technique was the method of choice for this investigation. 0.01 mg mL−1 concentration of the samples was prepared and thoroughly mixed separately with 20 ml of Potatoes Dextrose Agar (PDA) in a sterile sample bottle before it was poured into a plate. For the control sets, distill water was used instead of the sample as negative control while 0.01 mg mL−1 ketoconazole was used as positive control. The poured plates were inoculated aseptically with a 6 mm fungal disk of 72-h-old pure culture of the test isolates. This was then incubated between 25 and 27°C for 72 h for 5 days (Mishra et al., Citation2012). The observations were recorded at the end of the incubation period. Percentage mycelial growth inhibition was calculated with the following formula:
where NTR is the average diameter of fungal colony in negative control set (without treatments); TR is the average diameter of fungal colony in treatment sets.
3. Result and discussion
From the FTIR spectral of the carboxymethyl chitosan (CMC) (Figure ) and the carboxymethyl chitosan zinc supplement (Figure ), the most prominent band’s characteristic peak of the CMC is 3,445 cm−1, which could be attributed to O–H and N–H (amine) stretching vibration. The peak at 3,266 and 3,106 cm−1 is absorptions for N–H (amine) stretch and 697 cm−1. This could be attributed to N–H (amine) bending vibration. The band at 2,889 cm−1 is absorption for C–H stretch and the band at 1,647 cm−1 could be attributed to C=O and 1,363 cm−1 is for C–O stretch of amide group. The band at 567.77 cm−1 could be vibration stretch of OH. After the complexation reaction for the production of carboxymethyl chitosan zinc supplement, it was noticed that the bands at 3,266 and 3,106 cm−1 of the CMC which were very strong and prominent have changed. They were very weak and not prominent as could be seen from the spectra. This could be that the complexation reaction between the zinc and carboxymethyl chitosan utilized the NH2 functional group of the carboxymethyl chitosan in forming the complex with loss of a proton from the NH2 on carboxymethyl chitosan, thus affecting the NH2 stretch, thereby reducing the intensity of the stretch. The shift in the wave number 697.66 and 567.77 cm−1 in CMC to lower wave number of 570.32 and 384.19 cm−1 in carboxymethyl chitosan zinc supplement could be attributed to N–Zn and O–Zn complexation. This indicated that both nitrogen of the amine and oxygen of the hydroxyl groups are involved in the bond formation. Some researchers have also reported shift from higher wave number to lower when bonds are formed between N–Zn and O–Zn (Khan et al., Citation2013; Wang et al., Citation2004). The shift in those bands (N–Zn and O–Zn) might have caused the shifting of C=O and C–O stretch to lower energy.
Scanning electron micrograph of the carboxymethyl chitosan and carboxymethyl chitosan zinc supplement as shown in Figure presented carboxymethyl chitosan image in a long rod-like and agglomerated form, while that of its zinc supplement was in a flat-plate-like form. Also, the image showed that the carboxymethyl chitosan has bigger particle size than the zinc supplement. The differences in the surface morphologies of these materials could be suggesting that there is a change in internal structure of carboxymethyl chitosan as carboxymethyl chitosan zinc supplement is being formed through complexation. EDX of the carboxymethyl chitosan and carboxymethyl chitosan zinc supplement gave an indication of the composition of the element present in those materials as presented in their obtained spectral in Figure . According to EDX result, elemental composition of carboxylmethyl chitosan is 52.89% carbon, 39.34% oxygen, 0.38% sodium, 0.54% aluminum, 2.61% calcium, and 4.19% aluminum. The presence of elements like calcium, aluminum, and iron could be responsible for the non-detectability of nitrogen, because those metals could make its presence to be below the detection limit. A study presented carboxymethyl chitosan with the following elemental composition, carbon 55.65%, oxygen 34.36%, Nitrogen 5.30%, Sodium 1.63%, and Chlorine 1.91% with the use of Energy Dispersive X-ray Spectroscopy (Ali, Laghari, Ansari, & Khuhawar, Citation2013). On the other hand, 49.55% carbon, 40.40% oxygen, 1.28% sodium, 2.37% nitrogen, and 6.40% zinc was recorded for carboxymethyl chitosan zinc supplement. From the FTIR, the shift in wave number at 697.66 and 567.77 cm−1 in CMC to lower wave number of 570.32 and 384.19 cm−1 in carboxymethyl chitosan zinc supplement could be attributed to N–Zn and O–Zn complexation, indicating that both nitrogen of the amine and oxygen of the hydroxyl groups are involved in the bond formation (Wang et al., Citation2004).
Figure 3. Scanning Electron micrograph of carboxymethyl chitosan (a) and (b) and carboxymethyl chitosan zinc supplement (c) and (d).
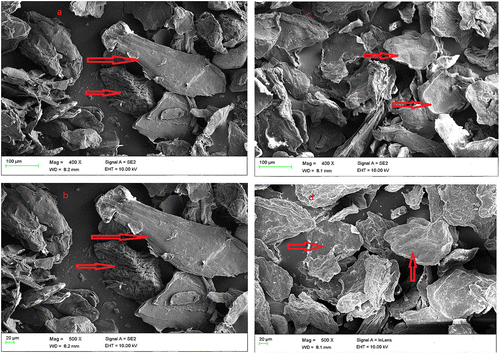
Figure 4. EDX spectral of carboxymethyl chitosan and carboxymethyl chitosan zinc supplement with carbon, nitrogen oxygen, sodium and zinc profile distributed on their surfaces.
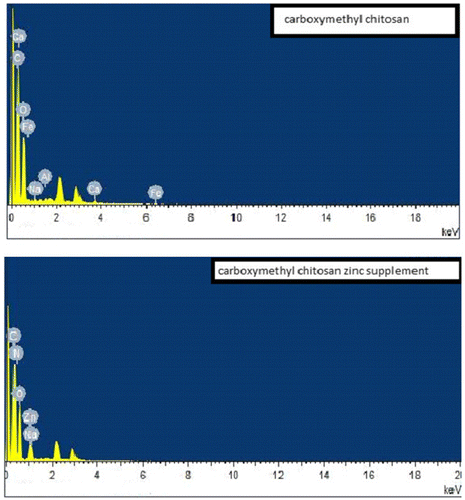
The X-ray patterns of carboxymethyl chitosan and carboxymethyl chitosan zinc supplement are shown in Figure , with clear significant differences in the spectral pattern. The spectrum of carboxymethyl chitosan showed not very strong peaks intensity as compared with that of its zinc supplement with higher intensity (as shown by the arrow). The high peak intensity could be an indication that the carboxymethyl chitosan zinc supplement is more crystalline in nature and this may be attributed to the complexation between carboxymethyl chitosan and zinc which might have led to the production of long chain rigid bulky material. Comparing the appearance of the peaks of carboxymethyl chitosan and carboxymethyl chitosan zinc supplement, it was observed that the supplement had intense peak at 2θ = 10°, whereas carboxymethyl chitosan had no peak. At 2θ equals to about 20.2°, 30° and 40° both have peaks with the zinc supplement having intense peaks. It was observed that carboxymethyl chitosan had a peak at 2θ = 37°, whereas carboxymethyl zinc supplement had none. The result is an indication that the reaction between zinc and carboxymethyl chitosan alters the crystal lattice of the caboxymethyl chitosan leading to the formation of new crystalline phase in caboxymethyl chitosan zinc supplement. It has been reported that there would be increase in the peak intensity, when chitosan or chitosan-based materials form complexes with metal ions, which was attributed to the formation of a new crystalline phase (Muzzareli, Ferrero, & Pizzoli, Citation1972; Wang et al., Citation2004). Thus, the increase in peak intensity of the carboxymethyl chitosan zinc supplement was in order as stated by other researchers (Muzzareli et al., Citation1972; Wang et al., Citation2004).
Stability of the carboxymethyl chitosan and carboxymethyl chitosan zinc supplement was examined using TGA/DTGA. It can be seen from Figure that thermal degradation process of carboxymethyl chitosan took place in five stages and that of carboxymethyl chitosan zinc was in four stages. The first stage was 30–100°C for both. This could be attributed to loss of moisture content. The second stage was from 100 to 250°C which corresponds to thermal decomposition of the main chain of polysaccharide (including saccharide rings and breaking of the C-O-C glycosidic bonds), vaporization, and elimination of volatile product such as carbondioxide (El-Nesr, Raafat, Nasef, Soliman, & Hegazy, Citation2014). The third degradation was due to detachment of NH2 group in the form of ammonia (NH3) released (Anitha, Brabu, Thiruvadigal, Gopalakrishnan, & Natarajan, Citation2012). At this stage, Carboxymethyl chitosan zinc supplement had only one decomposition stage between 250 and 400°C, but Carboxymethyl chitosan recorded two different stages 250–310°C and 310–400°C. The final degradation stage could be attributed to the degradation of inorganic component of the material. This occurs from around 400–900°C. The DTGA curve showed three degradation stages for the two materials. The DTGA curves indicated that the main thermal decay temperature was 390°C for carboxymethyl chitosan zinc supplement and 305°C for carboxymethyl chitosan. The high thermal decomposition of carboxymethyl chitosan zinc supplement could be attributed to the complexation between zinc and carboxymethyl chitosan to form along chain polymeric material which does not easily degrade under heat treatment. The implication of the result is that carboxymethyl chitosan zinc supplement would be thermally stable than the carboxymethyl chitosan
Antimicrobial activities of the carboxymethyl chitosan and zinc-supplemented carboxymethyl chitosan were examined against gram-positive bacteria; Bacillus cereus (B. cereus) and Staphylococcus aureus (S. aureus). Other bacteria are gram-negative; Pseudomonas syringae (P. syringae), Pseudomonas aeruginosa (P. aeru) and Escherichia coli (E. coli), likewises fungi such as Collectotrichum falcritum (C. falcutium), Rhzoctonia solani (R. salami), Colletotrihum lindematianum (C. linalimatianum), and Trichhoderum rubrum (T. rubruim). The results revealed that while zinc-supplemented carboxymethyl chitosan was active against all the microbes, carboxymethyl chitosan was only active against S. aureus. The standard drugs used as control have better performance than the prepared zinc-supplemented carboxymethyl chitosan and carboxymethyl chitosan. It was noticed from Table that zinc-supplemented carboxymethyl chitosan exhibited a better antibacterial activity against B. cereus 5 mm zone of inhibition, P. aeru (7 mm), E. coli and S. aureus (6 mm each), it has a low inhibition (3 mm) against P. syringae. Antibacterial activity of zinc-supplemented carboxymethyl chitosan could be attributed to the release of Zn2+, this has been reported to have the ability of penetrating the bacteria cell wall thus reacting with the cytoplastic content leading to the death of the bacteria (Shankar, Teng, Li, & Rhim, Citation2015) The low inhibitory activity of zinc-supplemented carboxymethyl chitosan against P. syringae may be due to the complex structure within the peptidoglycan layer of gram-negative bacteria that might have affected the reaction of each bacterium toward a particular drug (Anitha et al., Citation2012; Paisoonsin, Pornsunthorntawee, & Rujiravanit, Citation2013). The antibacterial activity of zinc-supplemented carboxymethyl chitosan obtained in this study compared moderately well with the reported work of Shankar et al. (Citation2015) while examining the antibacterial activity of gelatin-based zinc oxide nanoparticle (ZnO NPs) nanocomposite films prepared using zinc nitrate and zinc acetate. Antimicrobial activity of chitosan could be linked to the presence of –NH2 along its chain, but the substitution of monochloroacetic acid moieties with the proton from –NH2 in the preparation of NOCMC affects the antimicrobial activity of the produced carboxymethylchitosan. Hence, the low or non-biological activity of carboxmethyl chitosan. The final carboxymethylchitosan has a reduced number of –NH2 this would in-turn cause a reduction in the effective number of –NH3+ which would indirectly reduce the antibacterial activity of the carboxmethyl chitosan (Mourya, Inamdar, & Tiwari, Citation2010).
Table 1. Antibacterial activities of carboxymethyl chitosan, zinc-supplemented carboxymethyl chitosan and control
The antifungal potency of the carboxymethyl chitosan and that of zinc-supplemented carboxymetyl chitosan as presented in Table indicated that both had good antifungal activities against the tested microbes. Although, zinc-supplemented carboxymetyl chitosan had better potency than the carboxymetyl chitosan, they both lagged behind the control. The antifungal mechanism of chitosan has been linked to the ability of its derivatives to be involved in cell wall morphogenesis leading to interfering directly with fungal growth, because it has been observed microscopically that chitosan molecules diffuse inside hyphae interfering on the enzymes activity responsible for the fungus growth (Eweis, Elkholy, & Elsabee, Citation2006). Thus, chitosan derivatives such as carboxymethyl chitosan and zinc-supplemented carboxymethyl chitosan being much more soluble would have greater ability to diffuse into hyphae and causing higher morphogenesis thus preventing the fungi growth which would indirectly lead to the death of the microbe. The ability of zinc-supplemented carboxymethyl chitosan to release Zn2+, while diffusing and after diffusing into the cell wall of fungi could be responsible to its higher antifungal potency. The result reported in this research compared favorably with that of Mohamed, Sabaa, El-Ghandour, Abel-Aziz, and Abdel-Gawad (Citation2013) while analyzing effect of eighteen carboxymethyl chitosan (CMCh) Schiff bases and their reduced derivatives against Aspergillus fumigates, Geotricum candidum, and Candida albicans respectively.
Table 2. Antifungal effect of carboxymethyl chitosan, zinc-supplemented carboxymethyl and control
4. Conclusion
The research work through FTIR, XRD, and EDX confirmed the preparation of zinc-supplemented carboxymethyl chitosan from carboxymethyl chitosan. The TGA/DTA analysis revealed that the supplement is much more stable than the carboxymethyl chitosan and the SEM presented carboxymethyl chitosan image in rod-like form, while that of its zinc supplement was in a flat-plate-like form. Carboxymethyl chitosan and Zinc-supplemented carboxymethyl chitosan had good antifungal activity, only Zinc-supplemented carboxymethyl chitosan had good antibacterial activity against the microbes used. The aqueous solubility of zinc-supplemented carboxymethyl chitosan and its antimicrobial activities would make the prepared material a good product that could be used in pharmacy, biomedicine, food, and zinc supplement drug formulation.
Additional information
Funding
Notes on contributors
Olugbenga Oludayo Oluwasina
Olugbenga Oludayo Oluwasina is a lecturer at the Department of Chemistry Federal University of Technology, Akure, Ondo State Nigeria. He obtained his PhD in Industrial Chemistry from the same University. His research activities are focused on wood product, biomaterial, and natural products beneficiation.
References
- Ali, M. Z., Laghari, A. J., Ansari, A. K., & Khuhawar, Y. M. (2013). Synthesis and characterization of carboxymethyl chitosan and its effect on turbidity removal of river water. Journal of Applied Chemistry, 5, 72–79.
- Anitha, S., Brabu, B., Thiruvadigal, D. J., Gopalakrishnan, C., & Natarajan, T. S. (2012). Optical, bactericidal and water repellent properties of electrospun nano-composite membranes of cellulose acetate and ZnO. Carbohydrate Polymers, 87, 1065–1072.10.1016/j.carbpol.2011.08.030
- Avadi, M. R., Sadeghi, A. M. M., Tahzibi, A., Bayati, K. H., Pouladzadeh, M., Zohuriaan-Mehr, M. J., & Rafiee-Tehrani, M. (2004). Diethylmethyl chitosan as an antimicrobial agent: Synthesis, characterization and antibacterial effects. European Polymer Journal, 40, 1355–1361.10.1016/j.eurpolymj.2004.02.015
- Baqui, A. H., Black, R. E., El Arifeen, S., Yunus, M., Chakraborty, J., Ahmed, S., & Vaughan, J. P. (2002). Effect of zinc supplementation started during diarrhoea on morbidity and mortality in Bangladeshi children: Community randomised trial. BMJ, 325, 1059.10.1136/bmj.325.7372.1059
- Bhutta, Z. A., Bird, S. M., Black, R. E., Brown, K. H., Gardner, J. M., Hidayat, A., ... Shankar, A. (2000). Therapeutic effects of oral zinc in acute and persistent diarrhoea in children in developing countries: Pooled analysis of randomized controlled trials. American Journal of Clinical Nutrition, 72, 1516–1522.
- Brown, K. (2007). Information gaps for scaling‐up programs to improve zinc nutrition. A2Z Technical Brief.
- Bryce, J., Boschi-Pinto, C., Shibuya, K., & Black, R. E. (2005). WHO estimates of the causes of death in children. The Lancet, 365, 1147–1152.10.1016/S0140-6736(05)71877-8
- El-Nesr, E. M., Raafat, A. I., Nasef, M. S., Soliman, E. A., & Hegazy, A. E. (2014). Radiation synthesis and characterization of N, O-carboxymethyl chitosan/poly(vinylpyrrolidone) Copolymer Hydrogel. Arab Journal of Nuclear Science and Applications, 47, 14–27.
- Eweis, M., Elkholy, S. S., & Elsabee, M. Z. (2006). Antifungal efficacy of chitosan and its thiourea derivatives upon the growth of some sugar-beet pathogens. International Journal of Biological Macromolecules, 38, 1–8.10.1016/j.ijbiomac.2005.12.009
- Fima, L. (2009). Nutrition and growth. Journal of Clinical Research in Pediatric Endocrinology, 1, 157–163.
- Grønli, O., Kvamme, J. M., & Friborg, O. (2013). Wynn R Zinc deficiency is common in several psychiatric disorders. PLOS ONE, 8(12), 1–7.
- Hwayoung, N., Young, P. H., Jihye, K., & Jayoung, C. (2013). Salty taste acuilty is affected by the joint action of αenaca663t gene polymorphis and available zinc intake in young women. Nutrients Journal, 5, 4950–4953.
- Khan, A., Mehmood, S., Shafiq, M., Yasin, T., Akhter, Z., & Ahmad, S. (2013). Structural and antimicrobial properties of irradiated chitosan and its complexes with zinc. Radiation Physics and Chemistry, 91, 138–142.10.1016/j.radphyschem.2013.05.025
- Klug, A. (2010). The discovery of zinc fingers and their applications in gene regulation and genome manipulation. Annual Review of Biochemistry, 79, 213–231.10.1146/annurev-biochem-010909-095056
- Kong, M., Chen, X. G., Xing, K., & Park, H. J. (2010). Antimicrobial properties of chitosan and mode of action: A state of the art review. International Journal of Food Microbiology, 144, 51–63.10.1016/j.ijfoodmicro.2010.09.012
- Mahadevan, K., & Velavan, S. (2013). Analysis of salivary proteins as the biochemical indicators of nutritional status and salivary gland function. International Journal of Pharmacy and Biological Sciences, 4, 689–694.
- Mishra, P. K., Shukla, R., Singh, P., Prakash, B., Kedia, A., & Dubey, N. K. (2012). Antifungal, anti-aflatoxigenic, and antioxidant efficacy of Jamrosa essential oil for preservation of herbal raw materials. International Biodeterioration & Biodegradation, 74, 11–16.10.1016/j.ibiod.2012.06.026
- Mohamed, A. N., Sabaa, W. M., El-Ghandour, A. H. H., Abel-Aziz, M. M., & Abdel-Gawad, O. F. (2013). Preparation, Characterization and antimicrobial activity of carboxymethyl chitosan schiff bases with different benzaldehyde derivatives. Journal of American Science, 9, 247–264.
- Mourya, V. K., Inamdar, N. N., & Tiwari, A. (2010). Carboxymethyl chitosan and its applications. Advanced Materials Letters, 1, 11–33.10.5185/amlett
- Murray, P., Rosenthal, K., & Pfaller, M. (2006). Medical microbiology (5th ed.). Philadelphia, PA: Elsevier Editorial SA.
- Muzzareli, R. A. A., Ferrero, A., & Pizzoli, M. (1972). Light-scattering, X-ray diffraction, elemental analysis and infrared spectro-photometry characterization of chitosan, a chelating polymer. Talanta, 19, 1222–1226.10.1016/0039-9140(72)80068-7
- No, H. K., & Lee, M. Y. (1995). Isolation of chitin from crab shell waste. Journal of the Korean Society of the Food and Nutrition, 24, 105–113.
- Oluwasina, O. O., Lajide, L., & Owolabi, B. J. (2015). Sodium hydroxide-anthraquinone and sodium hydroxide-anthraquinone-ethanol pulping and their prepared carboxymethylcellulose. FUTA Journal of Research in Sciences, 11, 333–346.
- Osredkar, J., & Sustar, N. (2011). Copper and zinc, biological role and significance of copper/zinc imbalance. Journal of Clinical Toxicology, S3, 1–18.
- Paisoonsin, S., Pornsunthorntawee, O., & Rujiravanit, R. (2013). Preparation and characterization of ZnO− deposited DBD plasma-treated PP packaging film with antibacterial activities. Applied Surface Science, 273, 824–835.10.1016/j.apsusc.2013.03.026
- Patale, R. L., & Patravale, V. B. (2011). O, N-carboxymethyl chitosan–zinc complex: A novel chitosan complex with enhanced antimicrobial activity. Carbohydrate Polymers, 85, 105–110.
- Prasad, A. S. (2009). Impact of the discovery of human zinc deficiency on health. Journal of the American College of Nutrition, 28, 257–265.
- Shankar, S., Teng, X., Li, G., & Rhim, J. (2015). Preparation, characterization, and antimicrobial activity of gelatin/ZnO nanocomposite films. Food Hydrocolloids, 45, 264–271.10.1016/j.foodhyd.2014.12.001
- Song, J., Deng, J., Wu, Y., & Liu, W. (2015). Song F antibacterial effect of the carboxymethyl chitosan zinc peptide on several periodontal pathogens in vitro. Chinese Journal of Stomatology, 50, 413–417.
- Tsigos, I., Martinou, A., Kafetzopoulos, D., & Bouriotis, V. (2000). Chitin deacetylases: New, versatile tools in biotechnology. Trends in Biotechnology, 18, 305–312.10.1016/S0167-7799(00)01462-1
- Wang, X. H., Du, Y. M., & Liu, H. (2004). Preparation, characterization and antimicrobial activity of chitosan–Zn complex. Carbohydrate Polymers, 56, 21–26.10.1016/j.carbpol.2003.11.007
- Wu, Y. Y., Song, J., Deng, J., Pan, K. Q., Liu, X. Y., & Xu, H. P. (2015). Study of antimicrobial activity of carboxymethyl chitosan zinc complex material against cariogenic bacteria. Shanghai Journal of Stomatology, 24, 530–534.