Abstract
In this study, we designed and synthesized a series of 1,4-benzothiazine and evaluated them for anticancer activity toward HT-29 human colon cancer cells using SRB assay. Before the synthesis, docking studies were performed using various molecular targets of colon cancer including IL-2, IL-6, COX-2, caspase-3, and caspase-8. The molecular dynamic (MD) simulation was also executed to examine the stability of ligand-receptor complex of more stable dock conformation. Further computational study was carried out in order to predict the pharmacokinetic profile of titled compounds. Among 34 tested compounds, compounds AR13 and AR15 were found to be active against HT-29 cells (GI50 < 10 μM). Moreover, Compounds AR5, AR22, and AR34 showed the moderate activity with GI50 < 70 μM. The binding energy was found to be > −5 kcal/mol for AR13 and AR15 with all the molecular targets and the ligand-protein complex was found stable after its formation. Again, computational analysis revealed that both molecules AR13 and AR15 had good ADMET profiling. These encouraging outcomes allowed us to conclude that both AR13 and AR15 may emerge as lead compounds against colon cancer.
Public Interest Statement
On a global scale, colon cancer is one of the fourth most common cancer in men and the third most common cancer in women. Most of the drugs available for colon cancer treatment are cost effective and with major side effects. In this project, we wanted to synthesize and explore 1,4-benzothiazine derivatives. In vitro (ADMET profiling, MD Simulation and docking) and in vitro (HT-29 cells) studies were performed to get the acceptance of these derivates for colon cancer treatment. This study may be helpful for the development of potent 1,4-benzothizine scaffold toward the colon cancer treatment.
1. Introduction
On a global scale, colon cancer is the fourth most common cancer in men and the third most common cancer in women (Janakiram et al., Citation2008).Colorectal cancer is a malignant tumor with a higher rate of mortality and morbidity, approximately six lakh population die worldwide per year; it is the fourth supreme common form of cancer and the third imperative cause of cancer-related death worldwide (Thun, DeLancey, Center, Jemal, & Ward, Citation2010).Most of the patients die with a year after diagnosis. Chemotherapy and surgery are the primary treatment for colorectal cancer; however, synthetic drugs are costly and have several side effects. Searching for newer anticancer agents with improved efficacy and lower side effects are the hot trends in research nowadays (Al-Rashood et al., Citation2006; Chen et al., Citation2007; Cheng, Oritani, Horiguchi, Yamada, & Mong, Citation2000; Khan, Choudhary, Khan, & Rani, Citation2005).In this regard, 1,4-benzothiazine is the most effective agent as it has diverse biological responses particularly antifungal, immunomulation, (Fringuelli, Schiaffella, & Vecchiarelli, Citation2001) antioxidant, antimicrobial, (Goyal, Gautam, Khandelwal, & Gautam, Citation2013) properties. Many drugs from benzothiazine derivatives are available in the market like as meloxicam and piroxicam. On the other hand, it is noted that 1,4-benzothiazine are important pharmacophore (Figure ) which exhibits antitumor activity against the various tumor cell lines (Ajani, Citation2012; Bollu et al., Citation2015; Fringuelli et al., Citation2003; Zięba, Latocha, & Sochanik, Citation2013).
Figure 1. Reported and proposed pharmacophore for anticancer effect of 1,4-Benzothiazine containing derivatives.
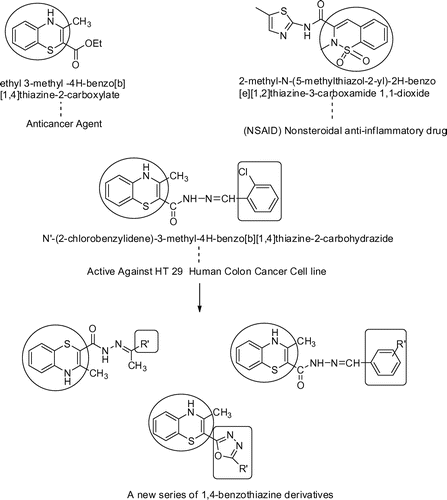
It has been previously noted that 1,4-benzothiazine are important pharmacophore to exhibit antitumor activity against the various tumor cell lines. All the selected derivatives have been designed on the basis of computation drug design and the selected structures were found to have good binding affinities with the colon cancer assigned targets. Thus, we hypothesized to synthesize some potent 1,4-benzothiazine derivatives for the anticancer activity particularly against colorectal cancer. Hence, we synthesized a new series of 1,4-benzothiazine derivatives (Figure ) and evaluated them for in vitro antitumor properties particularly toward colorectal cancer. Before performing synthesis of compounds, molecular docking was performed with various colorectal cancer targets like interleukin-2 (IL-2), interleukin-6 (IL-6), cyclooxygenase-2 (COX-2), caspase-3 and caspase-8. We performed the virtual screening of around 300 compounds, among which 34 compounds exhibited good affinity to all these targets with interaction energy −5 kcal/mol. All these significant information allowed us to synthesize these compounds and later they were tested against HT-29 human colon cancer cell line for their antitumor effect. The further computational analysis was also performed to predict the ADME parameters. Molecular modeling and Molecular dynamic simulation were executed to explore the better understanding of the molecular mechanism and stability of ligand-protein complex in the active site domain. Subsequently, the molecular mechanism might be helpful for colorectal cancer treatment in future.
2. Experiment
2.1. Materials and methods
The melting points of synthesized compounds were calculated using open capillary melting point apparatus. FT-IR spectra (KBr) were recorded on a Thermo Scientific (Nicole 6700) spectrophotometer. The 1H and 13C NMR spectra were reported on Joel and Bruker High-Resolution NMR spectrometer at 400 and 800 MHz, respectivily, using TMS as an internal standard. Chemical shifts were reported in ppm (δ) and signals were described as singlet (s), doublet (d), triplet (t) and multiple (m). The mass spectra were recorded via direct-infusion on a Waters Micro-Mass ZQ 2000 mass spectrophotometer and data were acquired with electrospray ionization (ESI) source.
2.2. Chemistry
All the chemicals and solvents, purchased from Sigma-Aldrich (India), Himedia (India) and S. D. Fine, were used without further purification. Thin layer chromatography analyses of compounds were performed on silica gel G coated Aluminum foils. The solutions of compounds were applied as a spot on the aluminum foils about 2 cm above from the lower edge. The mobile phases were preferred according to the polarity of compounds (Ethyl acetate: hexane).
The synthetic routes of compounds (AR1–AR34) are shown in the Figure . The chemical structure of the synthesized derivatives was analyzed and confirmed on the basis of spectral data. The physical data of entitled compounds are given in Table . The spectral characterizations of synthesized compounds are given in Supplementary (Table S1). The formation of 1,4-benzothiazine derivatives was supported by the presence of str. of Ar–C=C stretching (1,600–1,700 cm−1), Ar–C–C (1,000–1,350 cm−1), Ar–N–H (3,300–3,500 cm−1), C=O (1,665–1,760 cm−1), NH–N (1,690–1,760 cm−1), C–S (650–775 cm−1), and appeared N=CH around δ = 9.0 in 1H NMR spectra. Further mass spectra were used to confirm the assigned structure of compounds.
Table 1. Physical data of substituted 1,4-Benzothiazine derivatives (AR1–AR34)
2.2.1. General procedure for preparation of 2-carboethoxy-3-methyl-7-substituted 1,4 benzothiazine (2a-e)
In a solution of 2-amino-5-substituted thiophenol (1a-e) (0.1 mol) in dry THF, ethyl-α-chloroacetate (0.1 mol) was added with constant stirring in the presence of 0.5 mol% of pipyridine. The reaction mixture was refluxed for 2 h, and then it was cooled; the precipitate was filtered and purified by recrystallization from ethyl alcohol to yield. The dry products (2a-e) were prepared (Khairnar, Girase, & Chaudhari, Citation2013).
2.2.2. Method of preparation of 3-methyl-7-substituted-4H-1,4-Benzothiazine-2-carbohydrazide (3a-e)
In a solution of 0.1 mol of (2a-e) in 20 mL of ethanol, hydrazine hydrate (0.1 mol, 99%) was added, followed by the addition of a catalytic amount of conc. H2SO4 (0.5 mol%). The reaction mixture was refluxed for 2 h. The reaction mixture was poured in crushed ice. The solid mass that separated out and excess solvent was removed. The precipitate was recrystallized from ethanol and the product dried.(3a-e).
2.2.3. Method of preparation of 3,7-Dimethyl-4H-benzo[1,4]thiazine-2-carboxlicacid(1-phenylethylidene)-hydrazide (4a-e) (AR1–AR9)
Compounds (3a-e) and substituted ketone was taken in 100 ml RBF with methanol and refluxed it for 45 min. in the presence of 10 ml glacial acetic acid in order to get the precipitate with the help of cold water. The precipitate was collected by filtration under vacuum, recrystallized by ethanol (4a-e).
2.2.4. Method of preparation of 3,7-Dimethyl-4H-benzo[1,4]thiazine-2-carboxylicacid (6a-e) (AR10–AR26)
An equimolar amount of (3a-e) (0.01 mol) and various substituted aromatic aldehyde (0.01 mol) in the presence of glacial acetic acid was refluxed for 3–4 h. The reaction mixture was poured into crushed ice. The separated solid mass was filtered, washed with water and purified by recrystallization from ethyl alcohol to give the final products (6a-e).
2.2.5. Method of preparation of 3,7-Dimethyl-2-(5-methyl-[1,3,4]oxadiazole-2-yl)-4H-benzo [1,4] thiazine (5a-e) (AR27–AR34)
An equimolar amount of compounds (3a-e) (0.0054 mol) and various substituted benzoic acid (0.0054 mol) was refluxed with phosphorus oxychloride (5 ml) for 2–3 h till the completion of reaction. The reaction mixture was cooled at room temperature and poured into crushed ice. The precipitate was filtered, washed with water and further purified by recrystallization with ethanol to get the final products (5a-e).
2.3. Spectral data
2.3.1. 3-Methyl-4H-benzo[1,4]thiazine-2-carboxylicacid[1-(2,4-dichloro-phenyl)-ethylidene]-hydrazide (AR1)
Yield: 82%; mp: 162–165°C; IR (KBr, cm−1) 1,662.9 (Ar–C=C), 1,242.1 (Ar–C–C), 3,437.9 (Ar–N–H), 741.8 (C–S), 1,783.2 (C=O), 3,311.1 (NH–N), 776.6 (C–Cl); 1H NMR (400 MHz, DMSO-d6) δ ppm: 10.25 (s, 1H, NH), 8.29 (s, 1H, NH), 7.97 (s, 1H, Ar–H), 7.88–7.86 (d, 2H, Ar–H, J = 8 Hz), 7.56–7.53 (m, 2H, Ar–H), 7.00–6.98 (d, 2H, Ar–H, J = 8 Hz), 3.29 (s, 3H, CH3), 1.89 (s, 3H, CH3); 13C NMR (800 MHz, DMSO-d6) δ ppm: 19.29, 29.32, 93.40, 107.91, 114.09, 117.62, 119.47, 123.33, 127.38, 127.77, 131.39, 133.02, 137.88, 156.13, 165.70, 176.12; MS (ESI) m/z (%): 392.3 (68.5%) [M+H]+.
2.3.2. 3-Methyl-4H-benzo[1,4]thiazine-2-carboxylicacid[1-(4-bromo-phenyl)-ethylidene]-hydrazide (AR2)
Yield: 76%; mp: 169–171 °C; IR (KBr, cm−1) 1,663.0 (Ar–C=C), 1,242.0 (Ar–C–C), 3,457.5 (Ar–N–H), 741.9 (C–S), 1,783.7 (C=O), 3,310.4 (NH–N), 657.4 (C–Br); 1H NMR (400 MHz, DMSO-d6) δ ppm: 10.13 (s, 1H, NH), 8.73 (s, 1H, NH), 8.27–8.25 (d, 2H, Ar–H, J = 8 Hz), 8.08–8.06 (d, 2H, Ar–H, J = 8 Hz),7.97–7.95 (d, 2H, Ar–H, J = 8 Hz), 7.42–7.38 (m, 1H, Ar–H) 7.01–6.96 (m, 1H, Ar–H), 2.35 (s, 3H, CH3), 1.86 (s, 3H, CH3); 13C NMR (800 MHz, DMSO-d6) δ ppm: 27.20, 29.31, 101.78, 117.61, 119.47, 123.33, 124.60, 127.77, 130.68, 132.22, 134.79, 137.88, 145.86, 148.10, 165.70, 172.40; MS (ESI) m/z (%): 402.2 (44.0%) [M+H]+.
2.3.3. 3-Methyl-4H-benzo[1,4]thiazine-2-carboxylicacid[1-(4-hydroxy-phenyl)-ethylidene]-hydrazide (AR3)
Yield: 69%; mp: 164–166°C; IR (KBr, cm−1) 1,663.1 (Ar–C=C), 1,242.5 (Ar–C–C), 3,443.0 (Ar–NH), 742.8 (C–S), 1,783.2 (C=O), 3,310.5 (NH–N), 3,198.5 (OH), 1H NMR (400 MHz, CDCl3-d) δ ppm: 10.25 (s, 1H, NH), 8.46 (s, 1H, NH), 7.43–7.41 (d, 2H, Ar–H, J = 8 Hz), 7.29–7.26 (m, 1H, Ar–H), 7.13–7.10 (m, 1H, Ar–H) 6.96–6.94 (d, 4H, Ar–H, J = 8 Hz), 5.17 (s, 1H, OH), 3.54 (s, 3H, CH3), 1.68 (s, 3H, CH3), 13C NMR (800 MHz, DMSO-d6) δ ppm: 23.18, 29.31, 97.88, 115.62, 116.32, 117.62, 119.47, 123.33, 127.38, 127.77, 131.17, 137.88, 137.88, 140.59, 153.19, 165.70, 177.96; MS (ESI) m/z (%): 339.8 (68.0%) [M+H]+.
2.3.4. 3-Methyl-4H-benzo[1,4]thiazine-2-carboxylicacid[1-(4-methoxy-phenyl)-ethylidene]-hydrazide (AR4)
Yield: 74%; mp: 179–181°C; IR (KBr, cm−1) 1,681.4 (Ar–C=C), 1,244.4 (Ar–C–C), 3,445.9 (Ar–NH), 741.5 (C–S), 1,781.4 (C=O), 3,305.2 (NH–H), 2,740.3 (O–CH3); 1H NMR (400 MHz, CDCl3-d) δ ppm: 10.14 (s, 1H, NH), 8.79 (s, 1H, NH), 7.42–7.40 (d, 2H, Ar–H, J = 8 Hz), 7.29–7.25 (m, 1H, Ar–H), 7.13–7.09 (m, 1H, Ar–H), 6.98–6.96 (d, 4H, Ar–H, J = 8 Hz), 3.53 (s, 3H, CH3), 1.70 (s, 3H, CH3), 1.34 (s, 3H, OCH3); 13C NMR (800 MHz, DMSO-d6) δ ppm: 2.20, 29.32, 44.07, 64.84, 98.20, 105.79, 113.33, 117.62, 119.47, 123.33, 127.38, 129.60, 132.95, 137.88, 152.78, 157.29, 165.70; MS(ESI) m/z (%): 353.8 (58.5%) [M+H]+.
2.3.5. 3-Methyl-4H-benzo[1,4]thiazine-2-carboxylicacid[1-(3,4-dichloro-phenyl)-ethylidene]-hydrazide (AR5)
Yield: 85%; mp: 171–172°C; IR (KBr, cm−1) 1,682.4 (Ar–C=C), 1,240.5 (Ar–C–C), 3,446.7 (Ar–NH), 742.3 (C–S), 1,781.6 (C=O), 3,305.4 (NH–N), 797.5 (C–Cl); 1H NMR (400 MHz, DMSO-d6) δ ppm: 10.48 (s, 1H, NH), 10.14 (s, 1H, NH), 7.94–7.93 (d, 2H, Ar–H, J = 4 Hz), 7.89–7.87 (d, 1H, Ar–H, J = 8 Hz), 7.80 (s, 1H, Ar–H), 7.72–7.68 (m, 2H, Ar–H), 6.81–6.79 (d, 1H, Ar–H, J = 8 Hz), 2.26 (s, 3H, CH3), 1.89 (s, 3H, CH3); 13C NMR (800 MHz, DMSO-d6) δ ppm: 27.31, 29.32, 63.97, 104.41, 117.61, 119.47, 123.32, 127.37, 127.76, 128.65, 130.58, 131.51, 132.23, 136.48, 137.88, 160.19, 165.69; MS (ESI) m/z (%): 392.8 (76.5%) [M+H]+.
2.3.6. 3-Methyl-4H-benzo[1,4]thiazine-2-carboxylic acid (1-p-tolyl-ethylidene)-hydrazide (AR6)
Yield: 89%; mp: 181–183°C; IR (KBr, cm−1) 1,663.3 (Ar–C=C), 1,241.0 (Ar–C–C), 3,438.3(Ar–N–H), 741.6 (C–S), 1,783.7 (C=O), 3,311.1 (NH–N), 1,479.3 (–CH3); 1H NMR (400 MHz, DMSO-d6) δ ppm: 10.24 (s, 1H, NH), 8.29 (s, 1H, NH), 7.99–7.97 (d, 2H, Ar–H, J = 8 Hz), 7.87–7.85 (d, 2H, Ar–H, J = 8 Hz), 7.55–7.53 (d, 2H, Ar–H, J = 8 Hz), 6.99–6.96 (m, 2H, Ar–H), 2.28 (s, 3H, CH3), 1.19 (s, 3H, CH3); 13C NMR (800 MHz, DMSO-d6) δ ppm: 29.32, 32.72, 37.28, 60.59, 108.50, 117.62, 119.47, 123.32, 127.38, 127.77, 129.78, 131.51, 137.88, 142.47, 165.70, 166.62; MS (ESI) m/z (%): 337.8 (40.0%) [M+H]+.
2.3.7. 3-Methyl-4H-benzo[1,4]thiazine-2-carboxylicacid[1-(4-chloro-phenyl)-ethylidene]-hydrazide (AR7)
Yield: 63%; mp: 159–161°C; IR (KBr, cm−1) 1,662.3 (Ar–C=C), 1,240.1 (Ar–C–C), 3,435.3 (Ar–N–H), 741.4 (C–S), 1,783.2 (C=O), 3,311.1 (NH–N), 777.7 (–C–Cl); 1H NMR (400 MHz, DMSO-d6) δ ppm: 10.14 (s, 1H, NH), 8.78 (s, 1H, NH), 7.98–7.96 (d, 2H, Ar–H, J = 8 Hz), 7.42–7.38 (m, 2H, Ar–H), 7.34–7.32 (d, 2H, Ar–H, J = 8 Hz), 7.00–6.98 (d, 2H, Ar–H, J = 8 Hz), 2.29 (s, 3H, CH3), 1.19 (s, 3H, CH3); 13C NMR (800 MHz, DMSO-d6) δ ppm: 27.69, 29.33, 63.40, 95.59, 107.16, 117.62, 119.47, 123.33, 127.38, 127.77, 128.79, 135.87, 137.88, 159.06, 165.70; MS (ESI) m/z (%): 357.2 (60.0%) [M+H]+.
2.3.8. 3-Methyl-4H-benzo[1,4]thiazine-2-carboxylic acid (1-phenyl-ethylidene)-hydrazide (AR8)
Yield: 78%; mp: 150–152°C; IR (KBr, cm−1) 1,681.8 (Ar–C=C), 1,242.0 (Ar–C–C), 3,443.9 (Ar–N–H), 741.9 (C–S), 1,781.4 (C=O), 3,306.6 (NH–N); 1H NMR (400 MHz, CDCl3-d) δ ppm: 10.2 (s, 1H, NH), 8.69 (s, 1H, NH), 7.42–7.40 (d, 2H, Ar–H, J = 8 Hz), 7.36–7.35 (d, 1H, Ar–H, J = 4 Hz), 7.29–7.25 (m, 2H, Ar–H), 7.13–7.09 (m, 3H, Ar–H), 6.97–6.95 (d, 2H, Ar–H, J = 8 Hz), 1.51 (s, 3H, CH3), 1.35 (s, 3H, CH3); 13C NMR (800 MHz, DMSO-d6) δ ppm: 29.32, 29.99, 62.75, 75.58, 117.61, 119.47, 123.33, 127.38, 127.77, 131.34, 137.88, 158.03, 165.70; MS (ESI) m/z (%): 323.4 (78.0%) [M+H]+.
2.3.9. 3-Methyl-4H-benzo[1,4]thiazine-2-carboxylicacid(1-thiophen-2-yl-ethylidene)- hydrazide (AR9)
Yield: 72%; mp: 163–165°C; IR (KBr, cm−1) 1,663.3 (Ar–C=C), 1,242.6 (Ar–C–C), 3,460.8 (Ar–N–H), 1,782.9 (C=O), 3,310.6 (NH–N), 742.8, 713.5 (C–S); 1H NMR (400 MHz, CDCl3-d) δ ppm: 10.02 (s, H, NH), 9.01 (s, 1H, NH), 7.96–7.94 (d, 1H, Ar–H, J = 8 Hz), 7.62–7.60 (d, 1H, Ar–H, J = 8 Hz), 7.16–7.14 (d, 2H, Ar–H, J = 8), 7.09–7.05 (m, 3H, Ar–H), 2.51 (s, 3H, CH3), 1.35 (s, 3H, CH3); 13C NMR (800 MHz, DMSO-d6) δ ppm: 24.22, 29.33, 83.37, 100.91, 117.61, 119.47, 123.32, 127.38, 127.77, 127.92, 137.88, 148.23, 165.70, 170.43; MS (ESI) m/z (%): 329.6 (44.5%) [M+H]+.
2.3.10. 3-Methyl-4H-benzo[1,4]thiazine-2-carboxylic acid benzylidene-hydrazide (AR10)
Yield: 86%; mp: 185–187°C; IR (KBr, cm−1) 1,663.1 (Ar–C=C), 1,240.7 (Ar–C–C), 3,450.4 (Ar–N–H), 741.7 (C–S), 1,783.1 (C=O), 3,311.1 (NH–N); 1H NMR (400 MHz, CDCl3-d) δ ppm: 10.10 (s, 2H, NH), 9.19 (s, 1H, N=CH), 7.42–7.40 (d, 2H, Ar–H, J = 8 Hz), 7.29–7.25 (m, 2H, Ar–H), 7.13–7.09 (m, 3H, Ar–H), 7.00–6.98 (d, 2H, Ar–H, J = 8 Hz), 3.53 (s, 3H, CH3), 1.75 (s, 1H, H); 13C NMR (800 MHz, DMSO-d6) δ ppm: 29.32, 117.61, 119.47, 123.33, 126.04, 127.38, 127.77, 128.84, 129.40, 131.91, 137.88, 162.00, 165.70; MS (ESI) m/z (%): 309.0 (80.0%) [M+H]+.
2.3.11. 3-Methyl-4H-benzo[1,4]thiazine-2-carboxylicacid(3-ethoxy-4-hydroxybenzylidene)-hydrazide (AR11)
Yield: 78%; mp: 179–181°C; IR (KBr, cm−1) 1,663.5 (Ar–C=C), 1,244.0 (Ar–C–C), 3,438.3 (Ar–N–H), 741.9 (C–S), 1,782.9 (C=O), 3,309.2 (NH–N), 775.1 (C–Cl); 1H NMR (400 MHz, CDCl3-d) δ ppm: 9.80 (s, 2H, NH), 8.69 (s, 1H, N=CH), 8.01–7.99 (d, 2H, Ar–H, J = 8 Hz), 7.87–7.85 (d, 2H, Ar–H, J = 8 Hz), 7.18–7.14 (m, 1H, Ar–H), 7.02–6.99 (m, 1H, Ar–H), 6.87–6.85 (d, 2H, Ar–H, J = 8 Hz), 6.01 (s, 1H, OH), 4.27–4.23 (m, 2H, CH2), 2.49(s, 3H, CH3), 1.47–1.37 (s, 3H, CH3); 13C NMR (800 MHz, DMSO-d6) δ ppm: 15.19, 29.32, 64.26, 111.79, 116.02, 117.61, 119.47, 123.32, 123.79, 125.96, 127.38, 127.77, 137.88, 147.58, 150.54, 161.11, 165.70; MS (ESI) m/z (%): 369.8 (60.0%) [M+H]+.
2.3.12. 3-Methyl-4H-benzo[1,4]thiazine-2-carboxylicacid(3,4,5-trimethoxy-benzylidene)-hydrazide (AR12)
Yield: 65%; mp: 167–169°C; IR (KBr, cm−1) 1,663.2 (Ar–C=C), 1,232.7 (Ar–C–C), 3,446.2 (Ar–N–H), 740.6 (C–S), 1,782.1 (C=O), 3,311.3 (NH–N), 2,839.1 (–OCH3); 1H NMR (400 MHz, DMSO-d6) δ ppm: 10.51 (s, 1H, NH), 8.62 (s, 1H, N=CH), 8.10–8.08 (d, 1H, Ar–H, J = 8 Hz), 8.03–8.02 (d, 1H, Ar–H, J = 4 Hz), 7.31 (s, 1H, NH), 7.27–7.25 (d, 2H, Ar–H, J = 8 Hz), 7.18 (s, 1H, Ar–H), 7.15–7.10 (m, 2H, Ar–H), 6.94–6.91 (m, 1H, ArOH), 3.88–3.69 (m, 1H, Ar–H), 3.29 (s, 1H, OCH3), 2.04 (s, 3H, CH3); 13C NMR (800 MHz, DMSO-d6) δ ppm: 19.29, 29.31, 36.65, 56.40, 60.64, 104.90, 106.04, 117.61, 119.47, 123.33, 127.39, 127.78, 137.87, 140.67, 153.64, 165.70; MS (ESI) m/z (%): 399.8 (78.0%) [M+H]+.
2.3.13. 3-Methyl-4H-benzo[1,4]thiazine-2-carboxylic acid (2-chloro-benzylidene)-hydrazide (AR13)
Yield: 89%; mp: 172–174°C; IR (KBr, cm−1) 1,663.3 (Ar–C=C), 1,242.0 (Ar–C–C), 3,438.3 (Ar–N–H), 744.0 (C–S), 1,783.4 (C=O), 3,310.8 (NH–N), 778.0 (C–Cl); 1H NMR (400 MHz, DMSO-d6) δ ppm: 10.51 (s, 2H, NH), 8.94 (s, 1H, N=CH), 8.12–8.11 (d, 2H, Ar–H, J = 4 Hz), 7.55–7.50 (m, 2H, Ar–H), 7.46–7.42 (m, 1H, Ar–H), 7.27–7.25 (d, 2H, Ar–H, J = 8 Hz), 7.14–7.10 (m, 1H, Ar–H), 6.93–6.90 (m, 1H, Ar–H), 3.30 (s, 3H, CH3); 13C NMR (800 MHz, DMSO-d6) δ ppm: 29.32, 78.59, 105.08, 117.62, 119.47, 123.33, 127.38, 127.77, 128.68, 130.67, 133.67, 135.17, 137.87, 158.78, 165.70; MS (ESI) m/z (%): 343.0 (48.5%) [M+H]+.
2.3.14. 3-Methyl-4H-benzo[1,4]thiazine-2-carboxylic acid (3-hydroxy-benzylidene)-hydrazide (AR14)
Yield: 68%; mp: 186–188°C; IR (KBr, cm−1) 1,662.8 (Ar–C=C), 1,239.7 (Ar–C–C), 3,420.1 (Ar–N–H), 742.4 (C–S), 1,781.9 (C=O), 3,311.3 (NH–N), 3,420.1 (C–OH); 1H NMR (400 MHz, DMSO-d6) δ ppm: 10.50 (s, 1H, NH), 9.87 (s, 1H, NH), 9.85 (s, 1H, N=CH), 8.10–8.09 (d, 2H, Ar–H, J = 4 Hz), 7.46 (s, 1H, Ar–H), 7.27–7.25 (d, 2H, Ar–H, J = 4 Hz), 7.15–7.10 (m, 1H, Ar–H), 6.93–6.92 (m, 2H, Ar–H), 5.46 (s, 1H, OH), 3.31 (s, 3H, CH3); 13C NMR (800 MHz, DMSO-d6) δ ppm: 29.32, 114.52, 117.62, 119.47, 120.40, 123.34, 127.39, 127.78, 130.42, 135.56, 137.87, 153.98, 158.14, 161.97, 165.70, 167.83; MS (ESI) m/z (%): 325.8 (78.0%) [M+H]+.
2.3.15. 3-Methyl-4H-benzo[1,4]thiazine-2-carboxylicacid(3,4-dichloro-benzylidene)-hydrazide (AR15)
Yield: 87%; mp: 171–173°C; IR (KBr, cm−1) 1,662.6 (Ar–C=C), 1,242.2 (Ar–C–C), 3,438.3 (Ar–N–H), 742.4 (C–S), 1,782.2 (C=O), 3,310.6 (NH–N), 777.2 (C–Cl); 1H NMR (400 MHz, DMSO-d6) δ ppm: 10.51 (s, 1H, NH), 9.94 (s, 1H, NH), 8.69–8.67 (d, 1H, Ar–H), 8.13 (s, 1H, N=CH), 7.84 (s, 1H, Ar–H), 7.27–7.25 (d, 2H, Ar–H, J = 8 Hz), 7.14–7.10 (m, 2H, Ar–H), 6.94–6.92 (d, 1H, Ar–H, J = 8 Hz), 2.75 (s, 3H, CH3); 13C NMR (800 MHz, DMSO-d6) δ ppm: 29.32, 51.41, 117.61, 119.47, 123.33, 127.38, 127.77, 128.50, 131.77, 137.87, 148.05, 153.91, 159.13, 160.46, 165.70; MS (ESI) m/z (%): 378.0 (78.0%) [M+H]+.
2.3.16. 3-Methyl-4H-benzo[1,4]thiazine-2-carboxylic acid (4-methyl-benzylidene)-hydrazide (AR16)
Yield: 62%; mp: 187–189°C; IR (KBr, cm−1) 1,663.2 (Ar–C=C), 1,242.1 (Ar–C–C), 3,446.2 (Ar–N–H), 742.2 (C–S), 1,783.1 (C=O), 3,310.4 (NH–N), 1,479.3 (–CH3); 1H NMR (400 MHz, DMSO-d6) δ ppm: 10.51 (s, 1H, NH), 9.87 (s, 1H, NH), 8.62 (s, 1H, N=CH), 7.73–7.71 (d, 2H, Ar–H, J = 8 Hz), 7.28–7.26 (d, 2H, Ar–H, J = 8 Hz), 7.12–7.10 (d, 2H, Ar–H, J = 8 Hz), 6.93–6.92 (m, 2H, Ar–H), 2.46 (s, 3H, CH3), 2.33 (s, 3H, CH3); 13C NMR (800 MHz, DMSO-d6) δ ppm: 21.64, 29.33, 117.62, 119.47, 123.33, 127.38, 127.77, 128.80, 130.42, 131.67, 137.88, 141.82, 154.06, 161.74, 165.70; MS (ESI) m/z (%): 323.2 (38.0%) [M+H]+.
2.3.17. 3-Methyl-4H-benzo[1,4]thiazine-2-carboxylicacid(4-hydroxy-benzylidene)-hydrazide (AR17)
Yield: 69%; mp: 178–180°C; IR (KBr, cm−1) 1,663.4 (Ar–C=C), 1,261.7 (Ar–C–C), 3,438.3 (Ar–N–H), 742.3 (C–S), 1,781.7 (C=O), 3,307 (NH–N), 3,480.3 (C–OH); 1H NMR (400 MHz, DMSO-d6) δ ppm: 10.51 (s, 1H, NH), 9.73 (s, 1H, NH), 8.53 (s, 1H, N=CH), 8.05–8.03 (d, 1H, Ar–H, J = 8 Hz), 7.27–7.25 (d, 2H, Ar–H, J = 8 Hz), 7.14–7.10 (m, 2H, Ar–H), 6.94–6.92 (d, 2H, Ar–H, J = 8 Hz), 6.84–6.82 (d, 1H, Ar–H, J = 8 Hz), 5.59 (s, 1H, OH), 2.28 (s, 3H, CH3); 13C NMR (800 MHz, DMSO-d6) δ ppm: 29.33, 116.22, 117.62, 119.47, 123.33, 125.56, 127.38, 127.77, 129.52, 130.59, 137.88, 154.19, 160.88, 165.70; MS (ESI) m/z (%): 325.2 (78.5%) [M+H]+.
2.3.18. 3-Methyl-4H-benzo[1,4]thiazine-2-carboxylicacid(4-hydroxy-3-methoxy-benzylidene)- hydrazide (AR18)
Yield: 72%; mp: 172–174°C; IR (KBr, cm−1) 1,663.0 (Ar–C=C), 1,262.5 (Ar–C–C), 3,446.2 (Ar–N–H), 743.5 (C–S), 1,783.8 (C=O), 3,310.6 (NH–N), 2,742.3 (O–CH3), 3,480.3 (C–OH); 1H NMR (400 MHz, CDCl3-d) δ ppm: 10.51 (s, 1H, NH), 9.01 (s, 1H, NH), 8.5 (s, 1H, N=CH), 7.96–7.94 (d, 1H, Ar–H, J = 8 Hz), 7.59 (s, 1H, Ar–H), 7.27–7.25 (d, 1H, Ar–H, J = 8 Hz), 7.14–7.10 (m, 2H, Ar–H), 6.94–6.92 (d, 1H, Ar–H, J = 8 Hz), 6.90–6.84 (d, 1H, Ar–H, J = 24 Hz), 5.59 (s, 1H, OH), 3.79 (s, 3H, OCH3), 2.63 (s, 3H, CH3); 13C NMR (800 MHz, DMSO-d6) δ ppm: 29.32, 55.96, 110.43, 115.94, 117.62, 119.47, 124.06, 125.90, 127.38, 127.77, 137.88, 148.44, 150.41, 161.13, 165.70; MS (ESI) m/z (%): 355.8 (54.5%) [M+H]+.
2.3.19. 3-Methyl-4H-benzo[1,4]thiazine-2-carboxylicacid(2,6-dichloro-benzylidene)-hydrazide (AR19)
Yield: 83%; mp: 152–154°C; IR (KBr, cm−1) 1,663.0 (Ar–C=C), 1,262.3 (Ar–C–C), 3,441.9 (Ar–N–H), 742.5 (C–S), 1,784.6 (C=O), 3,311.1 (NH–N), 706.1, 776.6 (C–Cl); 1H NMR (400 MHz, DMSO-d6) δ ppm: 10.51 (s, 1H, NH), 8.83 (s, 1H, NH), 8.67 (s, 1H, N=CH), 7.60–7.57 (d, 2H, Ar–H, J = 12 Hz), 7.50–7.48 (d, 1H, Ar–H, J = 8 Hz), 7.27–7.25 (d, 1H, Ar–H, J = 8 Hz), 7.15–7.11 (m, 2H, Ar–H), 6.93–6.90 (m, 1H, Ar–H), 2.63 (s, 3H, CH3); 13C NMR (800 MHz, DMSO-d6) δ ppm: 29.32, 117.61, 119.47, 123.32, 127.38, 129.17, 129.70, 132.69, 134.66, 137.88, 152.97, 157.16, 165.70; MS (ESI) m/z (%): 378.2 (80.0%) [M+H]+.
2.3.20. 3-Methyl-4H-benzo[1,4]thiazine-2-carboxylicacid(4-bromo-benzylidene)-hydrazide (AR20)
Yield: 76%; mp: 163–165°C; IR (KBr, cm−1) 1,663.2 (Ar–C=C), 1,242.4 (Ar–C–C), 3,445.5 (Ar–N–H), 741.3 (C–S), 1,783.7 (C=O), 3,311.6 (NH–N), 697.8 (C–Br); 1H NMR (400 MHz, DMSO-d6) δ ppm: 10.51 (s, 1H, NH), 9.87 (s, 1H, NH), 8.66 (s, 1H, N=CH), 7.96–7.94 (d, 2H, Ar–H, J = 8 Hz), 7.80–7.78 (d, 2H, Ar–H, J = 8 Hz), 7.28–7.26 (d, 1H, Ar–H, J = 8 Hz), 7.14–7.10 (m, 2H, Ar–H), 6.93–6.92 (d, 1H, Ar–H, J = 4 Hz), 2.26 (s, 3H, CH3); 13C NMR (800 MHz, DMSO-d6) δ ppm: 29.32, 117.61, 119.47, 122.95, 123.33, 127.39, 127.78, 129.53, 130.69, 132.50, 132.89, 137.88, 153.95, 165.70; MS (ESI) m/z (%): 388.2 (48.5%) [M+H]+.
2.3.21. 3-Methyl-4H-benzo[1,4]thiazine-2-carboxylicacid(4-methoxy-benzylidene)-hydrazide (AR21)
Yield: 88%; mp: 179–181°C; IR (KBr, cm−1) 1,663.0 (Ar–C=C), 1,251.6 (Ar–C–C), (Ar–N–H), 740.9 (C–S), 1783.7 (C=O), 3,311.0 (NH–N), 2,838.8 (–OCH3); 1H NMR (400 MHz, DMSO-d6) δ ppm: 10.51 (s, 1H, NH), 9.83 (s, 1H, NH), 8.59 (s, 1H, N=CH), 7.78–7.76 (d, 2H, Ar–H, J = 8 Hz), 7.27–7.26 (d, 1H, Ar–H, J = 4 Hz), 7.15–7.11 (m,2H, Ar–H), 7.02–7.00 (d, 2H, Ar–H, J = 8 Hz), 6.94–6.90 (d, 1H, Ar–H, J = 16 Hz), 3.79 (s, 3H, OCH3), 2.63 (s, 3H, CH3); 13C NMR (800 MHz, DMSO-d6) δ ppm: 29.33, 55.85, 114.86, 117.62, 119.47, 123.33, 127.38, 127.77, 130.46, 134.71, 137.88, 154.15, 161.00, 162.14, 165.70; MS (ESI) m/z (%): 339.0 (78.0%) [M+H]+.
2.3.22. 3-Methyl-4H-benzo[1,4]thiazine-2-carboxylicacid(3-methoxy-benzylidene)-hydrazide (AR22)
Yield: 81%; mp: 185–187°C; IR (KBr, cm−1) 1,663.0 (Ar–C=C), 1,263.0 (Ar–C–C), 3,442.6 (Ar–N–H), 741.8 (C–S), 1782.2 (C=O), 3,311.4 (NH–N), 2,838.8 (–OCH3); 1H NMR (400 MHz, DMSO-d6) δ ppm: 10.51 (s, 1H, NH), 9.94 (s, 1H, NH), 8.65 (s, 1H, N=CH), 8.12–8.10 (d, 1H, Ar–H, J = 8 Hz), 8.05–8.03 (d, 1H, Ar–H, J = 8 Hz), 7.41–7.40 (d, 1H, Ar–H, J = 4 Hz), 7.36 (s, 1H, Ar–H), 7.27–7.25 (d, 1H, Ar–H, J = 8 Hz), 7.15–7.11 (m, 1H, Ar–H), 6.94–6.90 (m, 2H, Ar–H), 3.77 (s, 3H, OCH3), 2.63 (s, 3H, CH3); 13C NMR (800 MHz, DMSO-d6) δ ppm: 29.32, 55.67, 112.05, 112.92, 117.61, 119.47, 121.75, 123.32, 127.38, 127.77, 130.51, 135.65, 137.88, 159.99, 161.98, 165.70; MS (ESI) m/z (%): 339.0 (56.5%) [M+H]+.
2.3.23. 3-Methyl-4H-benzo[1,4]thiazine-2-carboxylicacid(4-nitro-benzylidene)-hydrazide (AR23)
Yield: 62%; mp: 173–175°C; IR (KBr, cm−1) 1,663.1 (Ar–C=C), 1,242.5 (Ar–C–C), 3441.9 (Ar–N–H), 743.1 (C–S), 1,784.1 (C=O), 3,311.1 (NH–N), 1,344.6 (–NO2); 1H NMR (400 MHz, DMSO-d6) δ ppm: 10.51 (s, 1H, NH), 9.74 (s, 1H, NH), 8.34 (s, 1H, N=CH), 8.21–8.19 (d, 2H, Ar–H, J = 8 Hz), 8.13–8.11 (d, 2H, Ar–H, J = 8 Hz), 7.27–7.25 (d, 1H, Ar–H, J = 8 Hz), 7.14–7.11 (m, 2H, Ar–H), 6.93–6.92 (d, 1H, Ar–H, J = 4 Hz), 2.63 (s, 3H, CH3); 13C NMR (800 MHz, DMSO-d6) δ ppm: 29.31, 117.61, 119.47, 123.32, 124.75, 127.38, 127.77, 128.86, 131.11, 135.57, 137.88, 138.78, 165.70; MS (ESI) m/z (%): 354.0 (52.0%) [M+H]+.
2.3.24. 3-Methyl-4H-benzo[1,4]thiazine-2-carboxylicacid(3-chloro-benzylidene)-hydrazide (AR24)
Yield: 74%; mp: 177–179°C; IR (KBr, cm−1) 1,663.1 (Ar–C=C), 1,242.2 (Ar–C–C), 3459.6 (Ar–N–H), 742.0 (C–S), 1,783.3 (C=O), 3,310.8 (NH–N), 779.3 (C–Cl); 1H NMR (400 MHz, DMSO-d6) δ ppm: 10.51 (s, 1H, NH), 9.96 (s, 1H, NH), 8.68 (s, 1H, N=CH), 8.08–8.06 (d, 1H, Ar–H, J = 8 Hz), 7.89 (s, 1H, Ar–H), 7.82–7.80 (d, 1H, Ar–H, J = 8 Hz), 7.52–7.50 (d, 1H, Ar–H, J = 8 Hz), 7.27–7.25 (d, 1H, Ar–H, J = 8 Hz), 7.14–7.10 (m, 2H, Ar–H), 6.93–6.90 (d, 1H, Ar–H, J = 12 Hz), 2.63 (s, 3H, CH3); 13C NMR (800 MHz, DMSO-d6) δ ppm: 29.32, 117.61, 119.47, 122.97, 123.32, 126.86, 127.77, 128.35, 131.34, 134.20, 136.25, 137.88, 153.82, 161.13, 165.70; MS (ESI) m/z (%): 343.2 (78.5%) [M+H]+.
2.3.25. 3-Methyl-4H-benzo[1,4]thiazine-2-carboxylicacid(2-fluoro-benzylidene)-hydrazide (AR25)
Yield: 79%; mp: 162–164°C; IR (KBr, cm−1) 1,662.6 (Ar–C=C), 1,233.7 (Ar–C–C), (Ar–N–H), 743.9 (C–S), 1,784.0 (C=O), 3,311.1 (NH–N), 652.5 (C–F); 1H NMR (400 MHz, DMSO-d6) δ ppm: 10.51 (s, 1H, NH), 9.87 (s, 1H, NH), 8.79 (s, 1H, N=CH), 8.10–8.08 (d, 1H, Ar–H, J = 8 Hz), 8.01–7.99 (d, 1H, Ar–H, J = 8 Hz), 7.58–7.56 (d, 1H, Ar–H, J = 8 Hz), 7.27–7.25 (d, 1H, Ar–H, J = 8 Hz), 7.14–7.10 (m, 2H, Ar–H), 6.93–6.90 (m, 2H, Ar–H), 2.63 (s, 3H, CH3); 13C NMR (800 MHz, DMSO-d6) δ ppm: 29.32, 116.72, 117.61, 119.47, 121.54, 123.32, 125.51, 127.38, 127.77, 128.34, 134.28, 137.89, 155.60, 161.39, 162.65, 165.70; MS (ESI) m/z (%): 327.0 (62.5%) [M+H]+.
2.3.26. 3-Methyl-4H-benzo[1,4]thiazine-2-carboxylicacid(2-bromo-benzylidene)-hydrazide (AR26)
Yield: 67%; mp: 157–159°C; IR (KBr, cm−1) 1,687.8 (Ar–C=C), 1,243.3 (Ar–C–C), 3,461.4 (Ar–N–H), 743.6 (C–S), 1,780.4 (C=O), 3,311.1 (NH–N), 659.5 (C–Br); 1H NMR (400 MHz, DMSO-d6): δ ppm: 10.51 (s, 1H, NH), 8.89 (s, 1H, N=CH), 8.12–8.10 (d, 1H, Ar–H, J = 8 Hz), 7.75–7.73 (d, 1H, Ar–H, J = 8 Hz), 7.49–7.44 (m, 2H, Ar–H), 7.28–7.26 (d, 1H, Ar–H, J = 8 Hz), 7.14–7.11 (m, 2H, Ar–H), 6.93–6.91 (d, 1H, Ar–H, J = 8 Hz), 3.12 (s, 1H, NH), 2.63 (s, 3H, CH3); 13C NMR (800 MHz, DMSO-d6) δ ppm: 29.32, 117.61, 119.47, 121.69, 122.68, 123.33, 126.32, 127.38, 127.77, 132.63, 133.92, 134.54, 137.88, 155.17, 165.70; MS (ESI) m/z (%): 388.0 (78.0%) [M+H]+.
2.3.27. 4-[5-(3-Methyl-4H-benzo[1,4]thiazin-2-yl)-[1,3,4]oxadiazol-2-yl]-phenol (AR27)
Yield: 61%; mp: 162–164°C; IR (KBr, cm−1) 1,739.6 (Ar–C=C), 1,268.5 (Ar–C–C), 3,436.5 (Ar–N–H), 757.0 (C–S), 1,782.1 (C=O), 1,600.9 (C=N–N), 1,507.4 (–CH=N), 1,268.5 (–C–O), (OH), 1,558.4 (C=C); 1H NMR (400 MHz, DMSO-d6): δ ppm: 10.51 (s, 1H, NH), 8.25–8.23 (d, 1H, Ar–H, J = 8 Hz), 8.03–8.01 (d, 2H, Ar–H, J = 8 Hz), 7.35–7.34 (d, 1H, Ar–H, J = 4 Hz), 7.28–7.23 (m, 2H, Ar–H), 6.93–6.90 (d, 2H, Ar–H, J = 12 Hz), 4.28 (s, 1H, OH), 2.63 (s, 3H, CH3); 13C NMR (800 MHz, DMSO-d6) δ ppm: 29.31, 73.25, 102.73, 108.94, 115.59, 116.33, 117.62, 122.71, 123.25, 127.77, 131.97, 142.84, 149.54, 159.43; MS (ESI) m/z (%): 323.6 (38.0%) [M+H]+.
2.3.28. 2-[5-(4-Chloro-phenyl)-[1,3,4]oxadiazol-2-yl]-3-methyl-4H-benzo[1,4]thiazine (AR28)
Yield: 73%; mp: 174–176°C; IR (KBr, cm−1) 1,683.3 (Ar–C=C), 1,282.7 (Ar–C–C), 3,418.7 (Ar–N–H), 761.1 (C–S), 1,799.3 (C=N–N), 1,320.6 (–CH=N), 1,311.5 (–C–O), 1,592.0 (C=C), 815.7 (C–Cl); 1H NMR (400 MHz, CDCl3-d): δ ppm: 10.17 (s, 1H, NH), 8.02–8.00 (d, 1H, Ar–H, J = 8 Hz), 7.91–7.89 (d, 2H, Ar–H, J = 8 Hz), 7.45–7.42 (m, 2H, Ar–H), 7.03–7.01 (d, 2H, Ar–H, J = 8 Hz), 6.85–6.82 (d, 1H, Ar–H, J = 8 Hz), 2.16 (s, 3H, CH3); 13C NMR (800 MHz, DMSO-d6) δ ppm: 34.20, 55.90, 114.27, 115.29, 116.26, 122.91, 123.43, 127.76, 128.86, 129.32, 131.81, 134.67, 163.29, 167.48; MS (ESI) m/z (%): 341.8 (64.0%) [M+H]+.
2.3.29. 2-[5-(4-Bromo-phenyl)-[1,3,4]oxadiazol-2-yl]-3-methyl-4H-benzo[1,4]thiazine (AR29)
Yield: 69%; mp: 146–148°C; IR (KBr, cm−1) 1,681.1 (Ar–C=C), 1,295.7 (Ar–C–C), 3,404.2 (Ar–N–H), 757.1 (C–S), 1,800.3 (C=N–N), 1,396.1 (–CH=N), 1,229.1 (–C–O), 1,586.0 (C=C), 680.3 (C–Cl); 1H NMR (400 MHz, CDCl3-d): δ ppm, 9.52 (s, 1H, NH), 8.11–8.10 (d, 1H, Ar–H, J = 4 Hz), 7.99–7.97 (d, 2H, Ar–H, J = 8 Hz), 7.94–7.92 (d, 2H, Ar–H, J = 8 Hz), 7.62–7.58 (m, 2H, Ar–H), 7.46–7.43 (d, 1H, Ar–H, J = 12 Hz), 2.16 (s, 3H, CH3); 13C NMR (800 MHz, DMSO-d6) δ ppm: 29.33, 55.90, 95.31, 114.27, 117.64, 123.43, 127.36, 129.49, 130.46, 131.76, 132.17, 132.85, 167.07; MS (ESI) m/z (%): 386.2 (42.5%) [M+H]+.
2.3.30. 3-Methyl-2-(5-p-tolyl-[1,3,4]oxadiazol-2-yl)-4H-benzo[1,4]thiazine (AR30)
Yield: 56%; mp: 151–153°C; IR (KBr, cm−1) 1,608.8 (Ar–C=C), 1,244.4 (Ar–C–C), 3,441.9 (Ar–N–H), 756.4 (C–S), 1,608.8 (C=N–N), 1,504.8 (–CH=N), 1,309.7 (–C–O), 1,562.8 (C=C), 1475.1 (-CH3); 1H NMR (400 MHz, CDCl3-d): δ ppm: 9.03 (s, 1H, NH), 8.06–8.04 (d, 2H, Ar–H, J = 8 Hz), 7.99–7.95 (m, 2H, Ar–H), 7.89–7.87 (d, 1H, Ar–H, J = 8 Hz), 7.47–7.45 (d, 2H, Ar–H, J = 8 Hz), 7.30–7.28 (d, 1H, Ar–H, J = 8 Hz), 2.41 (s, 3H, CH3), 2.16 (s, 3H, CH3); 13C NMR (800 MHz, DMSO-d6) δ ppm: 21.52, 21.60, 122.74, 123.16, 125.83, 127.58, 129.59, 129.80, 130.39, 134.76, 141.96, 143.49, 154.03, 167.77; MS (ESI) m/z (%): 321.8 (78.0%) [M+H]+.
2.3.31. 2-[5-(4-Methoxy-phenyl)-[1,3,4]oxadiazol-2-yl]-3-methyl-4H-benzo[1,4]thiazine (AR31)
Yield: 67%; mp: 170–172°C; IR (KBr, cm−1) 1,604.2 (Ar–C=C), 1,255.0 (Ar–C–C), 3,459.6 (Ar–N–H), 756.0 (C–S), 1,681.7 (C=N–N), 1,504.1 (–CH=N), 1,303.9 (–C–O), 1,472.9 (C=C), 2,836.6 (–OCH3); 1H NMR (400 MHz, CDCl3-d): δ ppm: 9.32 (s, 1H, NH), 8.05–8.02 (d, 2H, Ar–H, J = 8 Hz), 7.87–7.86 (d, 1H, Ar–H, J = 8 Hz), 7.47–7.45 (d, 2H, Ar–H, J = 8 Hz), 7.02–6.99 (m, 2H, Ar–H), 6.93–6.91 (d, 2H, Ar–H, J = 8 Hz), 3.86 (s, 3H, OCH3), 2.16 (s, 3H, CH3); 13C NMR (800 MHz, DMSO-d6) δ ppm: 24.17, 55.90, 95.16, 114.27, 115.27, 122.62, 123.43, 126.96, 128.85, 129.32, 131.81, 162.41, 163.28, 167.48; MS (ESI) m/z (%): 337.6 (44.0%) [M+H]+.
2.3.32. 2-[5-(3-Methyl-4H-benzo[1,4]thiazin-2-yl)-[1,3,4]oxadiazol-2-yl]-phenol (AR32)
Yield: 62%; mp: 142–144°C; IR (KBr, cm−1) 1,608.7 (Ar–C=C), 1,246.2 (Ar–C–C), 3,437.7 (Ar–N–H), 753.9 (C–S), 1,743.8 (C=N–N), 1,560.8 (–CH=N), 1,288.1 (–C–O), 1,477.6 (C=C), (–OH); 1H NMR (400 MHz, DMSO-d6): δ ppm: 9.36 (s, 1H, NH), 8.16–8.14 (d, 1H, Ar–H, J = 8 Hz), 7.74–7.70 (m, 2H, Ar–H), 7.60–6.58 (d, 1H, Ar–H, J = 8 Hz), 7.48–7.46 (d, 1H, Ar–H, J = 8 Hz), 7.36–7.34(d, 1H, Ar–H, J = 8 Hz), 6.92–6.89 (m, 2H, Ar–H), 4.39 (s, 1H, OH), 2.46 (s, 3H, CH3); 13C NMR (800 MHz, DMSO-d6) δ ppm: 31.36, 113.35, 117.57, 119.68, 122.44, 124.99, 127.31, 130.74, 131.37, 132.66, 133.97, 136.16, 146.70, 150.72, 167.77, 172.40; MS (ESI) m/z (%): 323.8 (78.5%) [M+H]+.
2.3.33. Acetic acid 2-[5-(3-methyl-4H-benzo[1,4]thiazin-2-yl)-[1,3,4]oxadiazol-2-yl]-phenyl ester (AR33)
Yield: 59%; mp: 175–177°C; IR (KBr, cm−1) 1,605.0 (Ar–C=C), 1,246.6 (Ar–C–C), 3,433.1 (Ar–N–H), 753.7 (C–S), 1,742.7 (C=N–N), 1,478.4 (–CH=N), 1,288.2 (–C–O), 1,450.4 (C=C); 1H NMR (400 MHz, CDCl3-d): δ ppm: 9.01 (s, 1H, NH), 8.27–8.25 (d, 1H, Ar–H, J = 8 Hz), 7.87–7.85 (d, 1H, Ar–H, J = 8 Hz), 7.59–7.56 (d, 1H, Ar–H, J = 8 Hz), 7.38–7.34 (m, 2H, Ar–H), 7.18–7.16 (d, 1H, Ar–H, J = 8 Hz), 6.89–6.85 (m, 2H, Ar–H), 2.84 (s, 3H, COCH3), 2.16 (s, 3H, CH3); 13C NMR (800 MHz, DMSO-d6) δ ppm: 21.49, 51.39, 113.36, 117.57, 119.68, 122.44, 127.31, 129.10, 130.74, 132.66, 135.75, 136.16, 143.65, 147.22, 150.73, 161.59, 162.79, 172.40; MS (ESI) m/z (%): 365.2 (58.5%) [M+H]+.
2.3.34. 2-[5-(3,5-Dinitro-phenyl)-[1,3,4]oxadiazol-2-yl]-3-methyl-4H-benzo[1,4] thiazine (AR34)
Yield: 64%; mp: 159–161°C; IR (KBr, cm−1) 1,624.2 (Ar–C=C), 1,282.7 (Ar–C–C), 3,440.6 (Ar–N–H), 725.2 (C–S), 1,704.2 (C=N–N), 1,473.2 (–CH=N), 1,282.7 (–C–O), 1,541.7 (–NO2); 1H NMR (400 MHz, DMSO-d6): δ ppm: 10.50 (s, 1H, NH), 8.34 (s, 2H, Ar–H), 8.20–8.18 (d, 1H, Ar–H, J = 8 Hz), 8.15–8.13 (d, 1H, Ar–H, J = 8 Hz), 7.59–7.56 (m, 2H, Ar–H), 7.51 (s, 1H, Ar–H), 2.63 (s, 3H, CH3); 13C NMR (800 MHz, DMSO-d6) δ ppm: 29.30, 97.46, 119.28, 120.53, 122.59, 123.24, 127.07, 129.37, 134.45, 135.76, 148.79, 163.28, 164.41; MS (ESI) m/z (%): 397.2 (66.5%) [M+H]+.
2.4. Docking study
The leading structures of compounds were drawn with ChemDraw Ultra 12.0 and their geometry was upgraded six times with Gauss view 5.0. On the other hand, National Centre for Biotechnology Information (NCBI) and Protein Data Bank (PDB) were used as chemical sources to get the recognized five homological cancer protein target namely IL-2 (1Z92), IL-6 (1IL6), Caspase-3 (1QX3), caspase-8 (1IBC) and COX-2 (4COX), respectively (De Boer et al., Citation1992; Du et al., Citation2010; Romanowski, Scheer, O’Brien, & McDowell, Citation2004).Operating site was perceived with the support of CASTp database. Further, in silico molecular docking studies of entitled by-product were executed using Autodock 4.1 along with its LGA algorithm for automated flexible ligand docking and binding energy evaluated in the form of negative kcal/mol probable hydrogen bonds and Pi bonds were evaluated.
2.5. Prediction of physiochemical properties
The Med Chem designer and QikProp were recycled to anticipate the ADME properties of the compounds for evaluating the drug-likeness of the 34 molecules. Chemical structure was improved via LigPrep. Further, we calculated the ADME profile of all these structures. In this study, we have appraised% ABS, QPPCaco, QPlog khsa and Lipinski’s violation (Lagorce, Sperandio, Galons, Miteva, & Villoutreix, Citation2008).
2.6. Molecular dynamic simulation
The molecular dynamic (MD) simulation proposed the good inhibitor activity of the compound. A dynamic simulation was accomplished and tracks the behavior of used inhibitor (AR13) into active site domain of IL-6. Perfect molecular docking position of ligand–protein was selected for MD simulation using Elmar Krieger MD simulation tools (Krieger, Darden, Nabuurs, Finkelstein, & Vriend, Citation2004). AMBER03 force field was allowed to perform real-time MD simulation (Best, Buchete, & Hummer, Citation2008). The compsite was solvated with HOH model (density = 0.997 g/l) into the defined 10 A0 larger simulation cell edge than protein and the delinquency physiological pH = 7.4 were accustom. Further, we used 0.9% NaCl (physiological solution) which contain Na+ and Cl− ions concentration as a mass fraction to maintain and neutralize the simulation cell boundary. Temperature and pressure were allocated on 298 K and 1 bar, individually. Then, the system was submitted for 3,000 ps time for running the MD simulation to get the portrait (sim) path. Finally, sims were evaluated and conforming data operated using Sigma Plot 11.0 tools.
2.7. Cytotoxicity assay using HT-29 cell line
HT-29 human colon cell line was grown in Roswell Park Memorial Institute media (RPMI 1640) containing 10% fetal bovine serum and 2 mM L-glutamine in a T-75 flask in 5% CO2/95% air at 37°C. After growing, 100 μL cells containing media was inoculated into 96 well plates at a concentration of 5 × 103 cells/well. After that, media were detached and 100 μL compounds containing media was added to each well (0.1, 1.0, 10.0 and 100.0 μM) and incubated for another 48 h. Next day, 50 μL of the cold 30% trichloroacetic acid (TCA) was added and incubated at 4°C for 1 h to terminate the reaction. The supernatant was evacuated and the plates were washed five times with tap water and air dried. Further, 50 μL (0.4% w/v, in 1% acetic acid) sulforhodamine B (SRB) was added into each of the wells and kept for 20 min at room temperature. Later, the residual dye was removed by washing five times with 1% acetic acid and again air dried. Finally, 10 mM trizma base was added to elude the bound stain and the absorbance was read on an Elisa plate reader at a wavelength of 540 nm with 690 nm reference wavelength (Boyd, Citation1997; Vichai & Kirtikara, Citation2006).
Further, 50% growth inhibition (GI50) was calculated using formula [(Ti−Tz)/(C−Tz)] × 100%. The abbreviations used in the formula were considered as; Time zero (Tz), control growth (C), and test growth in the presence of drug at the four concentration levels (Ti).
3. Result and discussion
3.1. Design
The conformational rational approach was followed to design the anticancer compounds using previously reported active pharmacophore and structure-activity relationship of potent anticancer compounds demonstrated some essential pharmacophoric features for the activity. (Ramachandran, Nambikkairaj, Kumaran, De, & Kumar, Citation2013). These essential pharmacophoric features are nitrogen atom which generates an electron-donating effect on the aromatic ring in order to produce the potent antitumor activity against HT-29 human rectal cancer cell line.
All the synthesized compounds are fulfilled these essential pharmacophoric features for the antitumor activity. Subsequently, it was decided to synthesize the entitled compounds to fulfill the criteria and screen them toward HT-29 human colon cancer cell lines.
3.2. Chemistry
The pattern of synthesis of the entitled compounds (AR1–AR34) was showed in the Figure . The equimolar amount of 2-amino-5-substituted thiophenol (1a-e) in dry THF was reacted with ethyl-α-chloroacetate with constant stirring by the addition of catalytic amounts of 0.5 mol% of pipyridine. The reaction mixture was refluxed for 2 h, and then it was cooled; the precipitate was filtered and further purified by recrystallization from ethyl alcohol to afford the compounds (2a-e). In addition, in the ethanolic solution of (2a-e), hydrazine hydrate (99%) was added followed by the addition of a catalytic amount of conc. H2SO4. The mixture was refluxed for 2 h and excess solvent was removed. The reaction mixture was allowed to cool down to obtain the solid crystals of products. The solid was recrystallized from ethanol to furnish 1,4-benzothiazine derivatives (AR1–AR34). Thin layer chromatography (TLC) was used for completion of the reaction as followed mobile phase ethyl acetate and methanol (7:3). The spectral analysis (FTIR, 1H 13C-NMR and MS) were applied to characterize the structure of synthesized compounds.
3.3. Docking study
In-silico molecular docking was performed using five established colon cancer targets namely IL-2, IL6, caspase-3, caspase-8 and COX-2 by Autodoc 4.1 along with LGA algorithm parameter for automated flexible ligand docking. The binding affinity (kcal/mol), hydrogen, pi bonds and contracting amino acids were evaluated (Table ). Best docking poses along with bonds and its distance of compounds AR5, AR13, AR15, AR22, AR31, and AR34, respectively, are shown in Figure .
Table 2. Docking affinity of titled compounds with assigned anticancer proteins
Figure 3. Show the best docking poses active compounds with contracted amino acid along with bonds and distance.
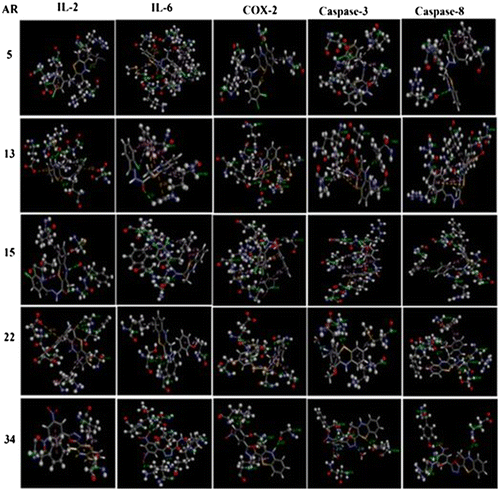
The molecular docking studies of all 34 compounds exhibited good binding affinity with the selected targets. Among them, compounds AR5, AR13, AR15, AR22, AR34, and AR31 exhibited affinities with all the selected targets. However, Compound AR13 exhibited good binding affinity with the COX-2 (−8.9 kcal/mol with 2H and 7π bonds), IL-2 (−6.2 kcal/mol with 3H and 5π bonds), IL-6 (−6.9 kcal/mol with 1H and 8π bonds), Caspase-3 (−6.0 kcal/mol with 1H and 8π bonds), and caspase-8 (−6.0 kcal/mol with 1H and 12π bonds) along with better complementarity with the amino acids. Compound AR15 also exhibited good binding affinity with caspase-3 (−6.4 kcal/mol with 1H and 12π bonds), IL-2 (−6.2 kcal/mol with 2H and 4π bonds), IL-6 (−6.7 kcal/mol with 1H and 9π bonds), COX-2 (−8.2 kcal/mol with 1H and 14π bonds) and Caspase-8 (−6.4 kcal/mol with 3H and 5π bonds). Virtual docking results related to synthesized compounds and targeted receptors are shown in Supplementary (Table S1) data which revealed that AR13 and AR15 had potent affinities to all the molecular targets. On the other hand, AR5, AR22, AR31, and AR34 also expressed higher binding affinities to all the assigned targets.
3.4. Prediction of ADME properties
A computational study was performed via QikProp tools to predict the physiochemical properties of the compounds (AR1–AR34). The ranges of the calculated property of the molecules with average value were shown in Table . Herein, we predicted% ABS, QPPCaco, QPlog khsa and Lipinski’s violation. From all these parameters, synthesized compounds displayed the great% ABS ranging from 69.23 to 100%. Moreover, all of the synthesized compounds followed the violated Lipinski’s parameters. Further predicted parameter like QPlog Khsa is a parameter of binding affinity of compounds with human serum albumin, exhibited between accepted ranges from −1.5 to 1.5.
Table 3. Pharmacokinetic parameters important for good oral bioavailability and protein binding parameters of compounds (AR1–AR34)
3.5. Molecular dynamic (MD) Simulation
MD simulation was executed on highly stable docking conformation of AR13 with IL-6. This compound exposed good binding affinity, H bond, and contraction with essential structure of IL-6. And the complex was further subjected to MD simulation to find out root-mean-square deviation (RMSD), potential energy and binding energy of the IL-6 complexed with AR13. The RMSD, potential energy, and binding energy are shown in Figure . Through the graphic profile, we observed the structural backbone stability of the complex during the MD simulation. There was no more fluctuation observed into the RMSD after the 300 ps time. This observation indicated the stability of backbone structure with ligand and we found better stability between AR13 and IL-6 (near the 3,000 ps time in MD simulation). Potential and binding energies of ligand-protein complex were calculated with time which determined that the potential energy (kJ/mol) did not show more fluctuation after 100 ps time, whereas average complex binding energy was observed near −24.85 kJ/mol. The fluctuation into the residue of the fundamental structure was shown in Figure . Finally, we performed the calculations from the data where we found the better structural stability of AR13 along with IL-6 into active site domain.
Figure 4. Shown the stability profile of complex A. Complex binding energy vs. time which indicated that complex stability under the MD simulation, B. Potential energy of complex with respective time and C. Average RMSD (Root Mean Square Deviation) graph which indicated convergence of the simulated structure toward an equilibrium state with respect to a reference structure (starting structure).
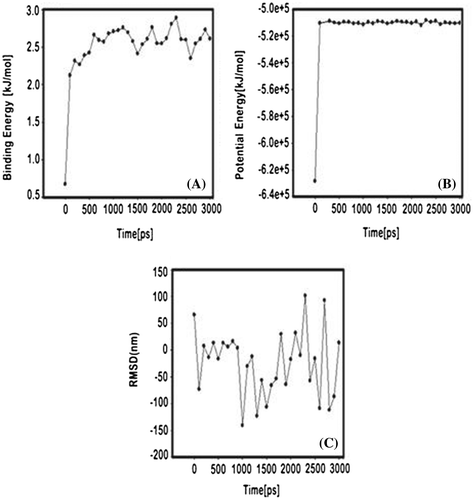
3.6. In vitro anticancer activity
HT-29 human colorectal cancer cell line was treated with AR1–AR34 at varying concentrations (10−7, 10−6, 10−5, and 10−4 M in DMSO) for 48 h. Cell viability of colon cells was dose dependent for both AR13 and AR15 where an increase in dose was associated with declined in cell viability. When both compounds were incubated with HT-29 cells, toxicity was observed at 0.1 μM concentration in both cases. The calculated 50% growth inhibition (GI50) was 0.1 and 6.3 μM, for AR13, and AR15, respectively. Further, compounds AR5, AR22, AR31, and AR34 demonstrated the moderate activity against the HT-29 human rectal cancer cell line with GI50 of 40, 37.6, 71.5 and 60.4 μM, respectively. Figure represents the microscopic images of HT-29 cells during various compounds treatment and calculated GI50 is tabulated in Table .
Figure 6. Microscopically images of HT-29 human colon cancer cell line on 80X with respect treatment of drugs (μM) was used, which are shown the GI50 inhibition such as AR5, AR13, AR15, AR16, AR22, AR23, AR31, AR34, and Control HT-29 cancer cell, positive control cell (Adriamycin), respectively.
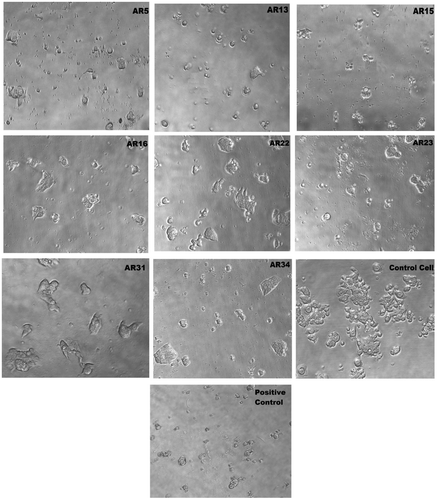
Table 4. Substitution of synthesized compound with their anticancer activity
3.7. Structure-activity relationship
In this study, 1,4-benzothiazine derivatives (AR1–AR34) were synthesized in order to predict the pharmacophoric substituent’s, responsible for the activity. Benzothiazine ring exhibited various pharmacological activities, which might be due to the presence of dinitro substitution at 1,4-position in the basic scaffold. The heterocyclic ring of 1,4-benzothiazine become smaller, however, the –NH acidity increases, and therefore the nitrogen center become less responsive with respect to electrophiles (Fringuelli et al., Citation2003). 1,4-benzothiazine analogs induce thymocyte apoptosis; exploratory SAR analysis recommended the benzothiazine skeleton, side chain nature, position and length modulate apoptosis activity. Replacing the imidazole in the side chain with different piperazines significantly decrease the activity, while replacing imidazole with benzimidazole causes only slight changes in apoptotic activity (TH Khan, Citation2012).It is noteworthy that 1,4-benzothiazine ring is also exerted significant effect against cancer. However, it may be enumerated that compounds (AR1–AR34) fulfill the essential pharmacophoric requirements for anticancer activities.
The current work contains the correlation and prediction of structure-activity relationship on the basis of their anticancer activity and significant exchange at the essential structure. Compounds AR5, AR22, AR31, and AR34 demonstrated minor to the moderate protection and also supported the pharmacophoric characteristics for the antitumor activity. The most active compounds AR13 and AR15 of this series having 2-chloro and 3,4-dichloro substitution at the position of R, produced significant effect against HT-29 human cancer cell line with GI50 < 10 μM. Moreover, the replacement of p-methoxy group with more electrons withdrawing 2-chloro group at the position R produced more stability and enhanced efficacy. Consequently, p-methoxy showed the mild protection against HT-29 Human colon cancer cell line with GI50 71.5 μM. In addition, substitutions at the position -R with 3-methoxy, 3,4-dichlorophenyl, and 3,5-dinitrophenyl led to AR22, AR5, and AR34, respectively with decreased anticancer activity. However, the substitution with lipophilic and electron withdrawing group at R position displayed the increasing anticancer effect. Moreover, oxadiazole and acetophenone formation on the side chain did not exert effectiveness toward the HT-29 human colon cancer cell line. Therefore, the results indicated that nitrogen into the heterocyclic ring along with hydrophobic substitution and electrophilic substitutions were essential for the anticancer activity.
4. Conclusion
A novel series of 3-methyl-7-substituted-4H-1,4-benzothiazine-2-carbohydrazide derivatives was designed, synthesized and their anticancer activity was evaluated by in vitro SRB assay on HT-29 human colorectal cancer cell line. Before the synthesis, molecular docking studies were accomplished to determine the probable molecular mechanism toward assigned targets. Moreover, a computational study was executed for forecast of ADME properties of entitled compounds. The findings from the computational study demonstrated good oral absorption, human albumin protein binding and also followed the Lipinski rules (Rule of five). Hence, the titled compounds might be stable in the form of pharmaceutical doses. Among them, compounds AR13 and AR15 were exhibited significant effects against the colon cancer cell line as well as best binding scaffold toward the cancer targets. The compounds AR22, AR5, AR34 and AR31 showed the moderate anticancer activity toward the HT-29 human colon cancer cell line. The GI50 values exhibited by the entitled compounds also supported the computational approach. In addition, the molecular dynamic simulation was also shown the stability of the fundamental structure of best pose ligand-protein complex along with MD run. However, these titled compounds might be emerged as lead compounds for the future. The scope of the present study is to perform in vivo experiments of AR13 and AR15 to evaluate their potency and efficacy for colon carcinogenesis in rat model. It is also necessary to find out the molecular mechanism of action (IL-2, IL-6, Cox-2, Caspase-3, and Caspase-8) of both these compounds in albino Wistar rat.
Funding
This work was supported by the University Grants Commission (UGC), New Delhi, India for providing UGC-MRP grant [project number 42-680/2013(SR)] and Department of Science and Technology (DST), India [reference number DST/SB/EMEQ-320/2014].
Suplementary_material.docx
Download MS Word (5.3 MB)Acknowledgments
Dr Sudipta Saha would like to express his gratitude to Advanced Centre for Treatment, Research and Education in Cancer (ACTREC), Navi Mumbai, India for undertaking anticancer screening. The authors express their sincere thanks to Delhi University, IIT Kanpur, and IIT Delhi and USIC BBAU, Lucknow for providing the spectral data.
Additional information
Notes on contributors
Sudipta Saha
Dr Sudipta Saha obtained his PhD in Pharmaceutical Sciences from National University of Singapore, Singapore and did his Post doctorate from experimental Therapeutic Centre, Astar, Singapore. Presently he is working as assistant professor in Department of Pharmaceutical Sciences, Babasaheb Bhimrao Ambedkar University (A Central University), Lucknow, Uttar Pradesh. His research interest is mainly into organic synthesis and cancer pharmacology. He has 35 Journal articles, two book chapters and three projects up to February, 2017.
References
- Ajani, O. O. (2012). Functionalized 1,4-Benzothiazine: A versatile scaffold with diverse biological properties. Archiv der Pharmazie, 345, 841–851. doi:10.1002/ardp.201200140
- Al-Rashood, S. T., Aboldahab, I. A., Nagi, M. N., Abouzeid, L. A., Abdel-Aziz, A. A., Abdel-hamide, S. G., ... El-Subbagh, H. I. (2006). Synthesis, dihydrofolate reductase inhibition, antitumor testing, and molecular modeling study of some new 4(3H)-quinazolinone analogs. Bioorganic & Medicinal Chemistry, 14, 8608–8621. doi:10.1016/j.bmc.2006.08.030
- Best, R. B., Buchete, N. V., & Hummer, G. (2008). Are current molecular dynamics force fields too helical? Biophysical Journal, 95, L07–L09. doi:10.1529/biophysj.108.132696
- Bollu, R., Palem, J. D., Bantu, R., Guguloth, V., Nagarapu, L., Polepalli, S., & Jain, N. (2015). Rational design, synthesis and anti-proliferative evaluation of novel 1,4-benzoxazine-[1,2,3]triazole hybrids. European Journal of Medicinal Chemistry, 89, 138–146. doi:10.1016/j.ejmech.2014.10.051
- Boyd, M. R. (1997). 2 The NCI in-vitro. Anticancer drug development guide: preclinical screening, clinical trials, and approval, 2, 23–42.10.1007/978-1-4615-8152-9
- Chen, C. J., Song, B. A., Yang, S., Xu, G. F., Bhadury, P. S., Jin, L. H., ... Lu, P. (2007). Synthesis and antifungal activities of 5-(3,4,5-trimethoxyphenyl)-2-sulfonyl-1,3,4-thiadiazole and 5-(3,4,5-trimethoxyphenyl)-2-sulfonyl-1,3,4-oxadiazole derivatives. Bioorganic & Medicinal Chemistry, 15, 3981–3989. doi:10.1016/j.bmc.2007.04.014
- Cheng, Q., Oritani, T., Horiguchi, T., Yamada, T., & Mong, Y. (2000). Synthesis and biological evaluation of novel 9-functional heterocyclic coupled 7-deoxy-9-Dihydropaclitaxel analogue. Bioorganic & Medicinal Chemistry Letters, 10, 517–521. doi:10.1016/S0960-894X(00)00031-7
- De Boer, E. C., De Jong, W. H., Steerenberg, P. A., Aarden, L. A., Tetteroo, E., De Groot, E. R., ... Ruitenberg, E. J. (1992). Induction of urinary interleukin-1 (IL-1), IL-2, IL-6, and tumour necrosis factor during intravesical immunotherapy with bacillus Calmette-Guérin in superficial bladder cancer. Cancer Immunology, Immunotherapy, 34, 306–312. doi:10.1007/BF01741551
- Du, J., Yang, H., Zhang, D., Wang, J., Guo, H., Peng, B., Guo, Y., & Ding, J. (2010). Structural basis for the blockage of IL-2 signaling by therapeutic antibody basiliximab. The Journal of Immunology, 184, 1361–1368. doi:10.4049/jimmunol.0903178
- Fringuelli, R., Schiaffella, F., Utrilla Navarro, M. P., Milanese, L., Santini, C., Rapucci, M., ... Riccardi, C. (2003). 1,4-Benzothiazine analogues and apoptosis: Structure–activity relationship. Bioorganic & Medicinal Chemistry, 11, 3245–3254. doi:10.1016/S0968-0896(03)00296-7
- Fringuelli, R., Schiaffella, F., & Vecchiarelli, A. (2001). Antifungal and immunomodulating activities of 1,4-benzothiazine azole derivatives: Review. Journal of chemotherapy, 13, 9–14. doi:10.1179/joc.2001.13.1.9
- Goyal, K., Gautam, N., Khandelwal, N., & Gautam, D. C. (2013). Synthesis and biological activity of substituted 4 H -1,4-benzothiazines, their sulfones, and ribofuranosides. Nucleosides, Nucleotides and Nucleic Acids, 32, 81–97. doi:10.1080/15257770.2013.765012
- Janakiram, N. B., Indranie, C., Malisetty, S. V., Jagan, P., Steele, V. E., & Rao, C. V. (2008). Chemoprevention of colon carcinogenesis by oleanolic acid and its analog in male F344 rats and modulation of COX-2 and apoptosis in human colon HT-29 cancer cells. Pharmaceutical Research, 25, 2151–2157. doi:10.1007/s11095-008-9582-7
- Khairnar, B. J., Girase, P. S., & Chaudhari, B. (2013). A facile and green synthesis of “5-(3-methyl-7-substituted-4H-1, 4-benzothiazin-2-yl)-4-aryl-4H-1, 2, 4-triazole-3-thiols” under ultrasound irradiation. Oriental Journal Of Chemistry, 29, 285–289.10.13005/ojc/290145
- Khan, M. T. H., Choudhary, M. I., Khan, K. M., & Rani, M. (2005). Structure–activity relationships of tyrosinase inhibitory combinatorial library of 2,5-disubstituted-1,3,4-oxadiazole analogues. Bioorganic & Medicinal Chemistry, 13, 3385–3395. doi:10.1016/j.bmc.2005.03.012
- Krieger, E., Darden, T., Nabuurs, S. B., Finkelstein, A., & Vriend, G. (2004). Making optimal use of empirical energy functions: Force-field parameterization in crystal space. Function, and Bioinformatics, 57, 678–683. doi:10.1002/prot.20251
- Lagorce, D., Sperandio, O., Galons, H., Miteva, M. A., & Villoutreix, B. O. (2008). FAF-drugs2: Free ADME/tox filtering tool to assist drug discovery and chemical biology projects. BMC Bioinformatics, 9, 396. doi:10.1186/1471-2105-9-396
- Ramachandran, M., Nambikkairaj, B., Kumaran, K., De, A. K., & Kumar, G. R. (2013). In silico analysis of 3D-QSAR and molecular docking for Bcl-2 inhibitors to potential anticancer drug development. Journal of Applied Pharmaceutical Science, 3, 23–29. doi:10.7324/JAPS.2013.31005
- Romanowski, M. J., Scheer, J. M., O’Brien, T., & McDowell, R. S. (2004). Crystal structures of a ligand-free and malonate-bound human caspase-1: Implications for the mechanism of substrate binding. Structure, 12, 1361–1371. doi:10.1016/j.str.2004.05.010
- TH Khan, M. (2012). Novel tyrosinase inhibitors from natural resources–their computational studies. Current medicinal chemistry, 19, 2262–2272. doi:10.2174/092986712800229041
- Thun, M. J., DeLancey, J. O., Center, M. M., Jemal, A., & Ward, E. M. (2010). The global burden of cancer: Priorities for prevention. Carcinogenesis, 31, 100–110. doi:10.1093/carcin/bgp263
- Vichai, V. & Kirtikara, K. (2006). Sulforhodamine B colorimetric assay for cytotoxicity screening. Nature Protocols, 1, 1112–1116. doi:10.1038/nprot.2006.179
- Zięba, A., Latocha, M., & Sochanik, A. (2013). Synthesis and in vitro antiproliferative activity of novel 12(H)-quino[3,4-b][1,4]benzothiazine derivatives. Medicinal Chemistry Research, 22, 4158–4163. doi:10.1007/s00044-012-0384-4