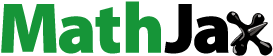
Abstract
Gamma radiation has been used for the fabrication of polyethylene (PE) wax from low density polyethylene pellets composited with 30wt% magnetic oxide Fe3O4 nanoparticles. The surface of (PE/Fe3O4) pellets was grafted by acrylic acid AAc using gamma irradiation techniques. A polarity of COOH groups support (PE/Fe3O4) uses, where functionalization give it facilities to be ready in hydrophilic and/or hydrophobic environments and increasing the capability in adsorbing wide variety of dyes molecules. Wax increases the stability and this will help to improve the recyclability of (PE-g-PAAc)/Fe3O4; recyclability is also helped by Fe3O4, compared to traditional dye adsorbents. The grafted polyethylene wax can serve as a good adsorbent support for a large variety of dyes. The chemical structure of the modified PE surface was achieved by a grafting process using attenuated total reflectance–Fourier transform infrared spectroscopy (ATR-FTIR). Samples of (PE-g-PAAc)/Fe3O4 have been characterized for water uptake and surface morphology using a scanning electron microscope (SEM); the crystal structure has been determined using X-ray diffraction; and dye removal efficiency was assessed using toluidine blue (TB) as a model.
Public Interest Statement
• | Uses of radiation technology for preparing wax-magnetic nanocomposite (Fe3O4/PE) pellets grafted by PAAc. | ||||
• | Functionalized give wax to be ready in hydrophilic and/or hydrophobic environments. | ||||
• | The (PE-g-PAAc)/Fe3O4 pellets were expanded due to the swelling process. | ||||
• | Capability of adsorbing a TB dye was examined. |
1. Introduction
There are many advantages of radiation grafting over other conventional methods due to its simplicity, and because catalysts and additives are not needed to initiate the grafting reaction (Citation1). Grafting is a process that the polymer acquisition a new feature given them a prioritie to use in a requisite application. Grafting occurs by monomers covalently bonding onto the polymer chains. This method produces functional groups that adhere to polymer substrates by the strongest chemical bond. Grafted copolymers are promising because they have very different properties to the original polymer before grafting. This article is aimed at the synthesis of magnetic wax (hydrophobic) polymers and coating with a (hydrophilic) layer obtained by grafting of poly acrylic acid (PAAc), using gamma radiation without significant damage to the polymer substrate. Use of this new material will be encouraged for diverse applications in biomedical, catalysis, sensors and dye adsorbent fields. The degree of grafting is adjusted by irradiation dose and monomer concentration with other parameters, e.g. temperature, type of solvent and pH). Gamma irradiation is a promising technique to fabricate a wide range of polymeric materials (Citation2–6) especially to develop selective copolymers for certain applications. The main purpose of the modification is to improve biocompatibility, wettability, mechanical and thermal properties.
Poly ethylene (PE) wax is a synthetic hydrocarbon wax consisting of ethylene monomer chains, and has a comparatively high melting point. PE waxes are compatible with other waxes that are highly hydrophobic. Exposed wax to gamma rays may cause a weight change as a result of irradiation-induced oxidation due to oxygen absorption from the surrounding atmospheric. Furthermore, gamma irradiation, especially at high doses above 50 kGy, causes the generation of radiolytic gases such as methane, carbon oxides, and hydrogen, and leads to the formation of cavity and increases the roughness of a surface (Citation7, 8). To retain most wax characteristics and also acquire additional properties of the grafted moiety PAAc which hydrophilic in nature. Introducing magnetic oxide (Fe3O4) as a composite gives a new material with three phases collected in one.
The PE grafting matrix is a popular subject of research and is used in numerous applications. But grafting of PE wax using acrylic acid that has been grafted onto different polymeric materials has not been done to date. Acrylic acid is pH sensitive and can undergo many chemical reactions to produce new chemical compounds. Due to AAc molecules is good adherent onto almost polymer substrate surface by grafting process induced by gamma radiation.
The main feature of polymer–metal nanocomposite is the stabilization of metal nanoparticles. Different techniques are used to fabricate them, depending on their final application. Fe3O4/PE-g-PAAc is an eco-environmental material exhibiting magnetic properties, and is easily recovered using magnets. This feature will be very useful if Fe3O4/PE-g-PAAc is used to remove dye from the environment.
In this article, gamma radiation is used for fabrication and modification of PE/Fe3O4 magnetic composite wax to enhance dye removal. The surface of PE/Fe3O4 was grafted with PAAC so the hydrophilic part comes into contact with the hydrophobic matrixes; thus the new material Fe3O4/PE-g-PAAc was used as a dye adsorbent. The modification of the wax surface is of considerable importance for enhanced dye adsorption. The Fe3O4/PE-g-PAAc material will be characterized.
2. Material and methods
2.1. Material
LDPE (MFI 7 g/10 min, density 0.925 g cm−3, melting temperature (120 °C), was supplied by Exxon Mobil Co., Al-Jubail Saudi Arabia. Black iron oxide/magnetite (Fe3O4) powder was from READE Co., Manhattan, USA. Acrylic acid monomer 99% was supplied by Sigma-Aldrich Co, St. Louis, USA
2.2. Preparation of magnetic polyethylene wax (PE/Fe3O4) nanocomposite and PAAc grafting process
Preparation procedures of PE wax from LDPE through radiation-thermolysis processes were reported in a previous work (Citation9). Homogenized black pellets of the iron oxide 30% (Fe3O4) nanoparticle composite with PE wax were grafted by PAAc using gamma radiation techniques using the following procedures. Radiation-induced grafting is a method to functionalize the surfaces of existing PE/Fe3O4 pellets. A weighed amount of PE/Fe3O4 pellets and 20% acrylic acid monomer were placed in a test tube. One ml of 0.1 M FeCl3 was added to the mixture to prevent homo polymer formation of PAAc, and the mixture was kept at room temperature for 2 h. After that the test tube was exposed to Co-60 gamma cell at dose of 20 kGy with dose rate 2.08 kGy h−1. The product (PE-g-PAAc)/Fe3O4 was washed with 0.1 ethanol/water several times, and then dried and again washed with water. The sample was then finally dried in an oven for 3 h at 50○C and further analysis was carried out. The grafting percentage was calculated using the following equation:(1)
(1)
where Wi is the initial weight of the PE/Fe3O4 sample used and Wg is the weight of the grafted (PE-g-PAAc)/Fe3O4 sample after washing and drying.(2)
(2)
Wt is the weight of swelled (PE-g-PAAc)/Fe3O4 sample and Wd is the weight of the initial dry sample.
2.3. Gamma irradiation
A cobalt-60 Canadian gamma cell 220 was constructed in the National Centre For Radiation Research and Technology, Nasr City, Cairo with a dose rate of 2.08 kGys h−1 was used.
2.4. Characterization of magnetize (PE-g-PAAc)/Fe3O4 wax nanocomposite property
2.4.1. FTIR spectroscopy
Attenuated total reflectance-Fourier transform infrared (ATR-FTIR) spectroscopy was conducted on a Vertex 70 FTIR spectrometer equipped with HYPERION™ series microscope (Bruker Optik GmbH, Ettlingen, Germany), over the 4000–400 cm−1 range, at a resolution of 4 cm−1. Software OPUS 6.0 (Bruker) was used for data processing, which was baseline corrected by the rubber band method with CO2 bands excluded.
2.4.2. X-ray diffraction
X-ray diffraction (XRD) patterns were obtained with the XRD-6000 series from Shimadzu Scientific Instruments (SSI), Kyoto, Japan.
2.4.3. Transmission and scanning electron microscope
The surface morphology of PE/Fe3O4 was evaluated, as well as the degree of dispersion of the Fe3O4 nanoparticles in the prepared composites. The dispersion of Fe3O4 nanoparticles on the PE matrix was confirmed by the transition electron microscope (TEM) (JEM-100CXII) obtained from JEOL Ltd (Tokyo, Japan). The samples were prepared by placing a melted drop of PE/Fe3O4 on a carbon coated copper grid and exposing the grid (200 mesh) to hot air to form a very thin layer of PE/Fe3O4 on the grid and examined with resolution: 0.3 nm, magnification:×330–250,000. The effect of gamma radiation on the surface morphology of PE/Fe3O4 pellets was examined by SEM model (JSM-5400) from JEOL Ltd.
3. Results and discussion
3.1. Surface morphology of magnetized (PE-g-PAAc)/Fe3O4 wax nano composite
The TEM revealed the size distribution of the Fe3O4 nanoparticles composite embedded PE wax varied from 10 to 12 nm, as shown in Figure (a). Scanning electron microscopy (SEM) was performed to investigate the surface morphology of unirradiated and irradiated PE/30%Fe3O4 wax as shown in Figure (b) for the unirradiated samples and Figure 1(c) for the irradiated samples, which become rougher. Figure (d) shown the surface of grafting sample (PE-g-PAAc)/Fe3O4.
3.2. Crystallinity of magnetic (PE/Fe3O4) wax nano composite
From Figure it can be seen that the sites and the intensity of the diffraction peaks are consistent with the standard pattern for {JCPDS-019–0629} with high intensity Bragg peaks at 2θ = 29.82○, 35.7○, 42.2○, 52.6○, and 64.1○, corresponding to the (220), (311), (400), (422) and (440) crystal planes which were the characteristic peaks of standard Fe3O4 crystal. The pattern indicates that polyethylene wax with magnetic oxide exhibited or conserved the crystalline structures of both of them. The Bragg peaks in the X-ray diffractogram (Figure ) for 0 (2θ = 21.1°) and (2θ = 23.29°) is indicative of orthorhombic crystallinity in these samples which correspond to the (110) and (200) planes of PE, and cubic-phase Fe3O4.
According to the Debye–Scherrer formula, the crystallite size Dhkl for the sample is given by (Citation10):(3)
(3)
A crystallite size (D) of Fe3O4 particle can be estimated. Where β is the full width at half-maximum (FWHM) value of XRD lines, Cu Kα radiation is 1.54 Å and K is the shape factor, which can be assigned a value of 0.90, cos θ is the cosine of the Bragg angle and β is the half-height of angle diffraction in radians. Convert β from degree to radians by:(4)
(4)
The crystal size is determined by taking the reflecting peak at 35.7°, which is the characteristic peak of (311) Fe3O4. The calculated average crystallite sizes of Fe3O4 particles are about 9 nm.
3.3. Chemical modification of PE/Fe3O4 by PAAc grafting was performed by FTIR
Radiation grafting of PAAc onto PE/Fe3O4 wax nanocomposite is undoubtedly a fascinating field for research as well as a practical way to improve PE wax value. The grafting occurs on the surface of PE wax and not in the bulk. The mechanism of grafting is free radical mechanime. FTIR established that chemical changes were induced onto PE wax. Figure shows the two characteristic peaks for PE wax, located at: 2,914 and 2,847 cm−1, attributed to asymmetric and symmetric CH2, respectively. Two very low intensity peaks located at 3,034 and 1,373 cm−1 are attributed to the symmetrical stretching and bending vibration of CH3, which may be for polypropylene (branched chain) (Citation11). This indicated that PE wax contained some branched CH3 molecules, i.e. that polyethylene wax has a degree of branching. Two peaks of PE located at 1,463 and 723 cm−1 are attributed to the spectral vibration of (CH2) scissoring and rocking, respectively. The peak at 723 cm−1 is chosen to be the internal reference of the polyethylene (and remains unchanged during the grafting reactions). Figure shows the FTIR peaks of the carbonyl group (C = O) located at 1,709 cm−1, indicating that surface grafting of PAAc occurred. Figures and did not differentiate expected in the peak of the carbonyl group (C = O). FTIR spectroscopy was performed in order to prove the formation of Fe3O4 as composite with polymer matrix. The FTIR spectrum in Figures and exhibits two peaks at 562 and 620 cm−1; these peaks were assigned to the Fe–O stretching mode of the tetrahedral and octahedral sites (Citation12).
3.4. Swelling as a function of time
Figure shows the swelling behaviour of PE-g-PAAc/Fe3O4 as a function of time. It is observed that the grafted sample show maximum swelling (52%) in 570 min in water at room temperature, indicating that PE wax as completely hydrophobic becomes partially hydrophilic through the grafting of PAAc.
Figure (a) and 6(b) shows the images of the irradiated pellet samples of PE/Fe3O4 before and after grafting of PAAc, respectively. In Figure 6(c), the pellets were expanded due to swelling process. Swelled PE pellets containing iron oxide were introduced after PAAc grafting. The pellets swelled by 20 and 52%. Images of the pellets of (PE/Fe3O4) wax in Figure 6 have shown that the swelling is due to the formation of a hydrophilic layer on the surface of the wax.
Figure 6. Wax pellet nanocomposites of (a) irradiated (PE/Fe3O4); (b) unswelled (PE-g-PAAc)/Fe3O4; (c) swelled and expanded (PE-g-PAAc)/Fe3O4.
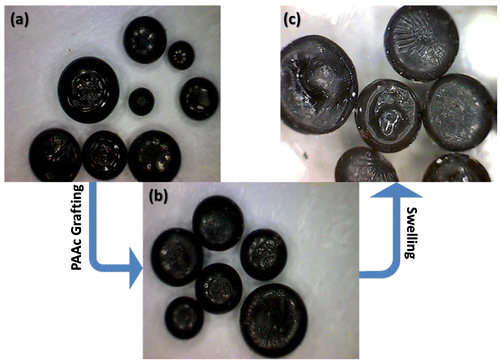
Figure 7. The sequence of dye removal efficiency using (b) (PE-g-PAAc)/Fe3O4 compared with (a) (PE/Fe3O4).
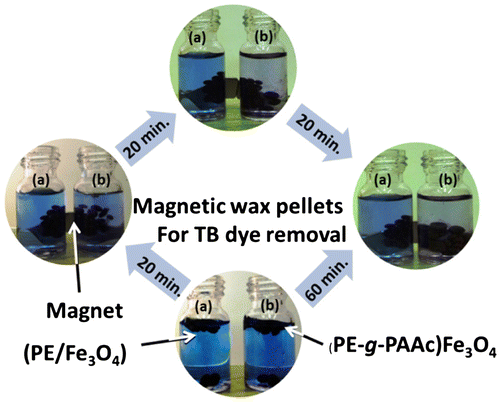
Scheme 1. The grafting procedure using gamma irradiation techniques in the design of safe polymer–metal nanocomposites (PE-g-PAAc)/Fe3O4 with magnetic property for safe dye removal.
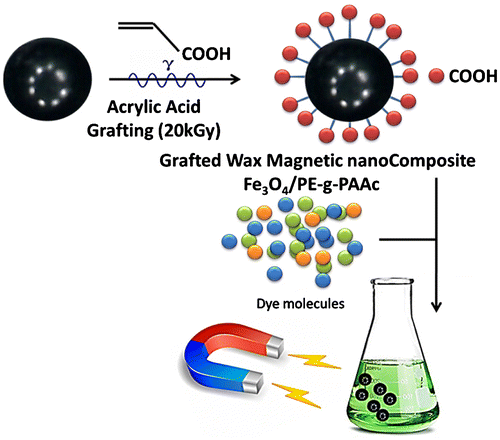
Figure (a) shows the rough and cavity for irradiated PE wax pellets + 30% Fe3O4 samples. This cavity of PE surface is beneficial and increasing the grafting percentage for PAAc which reach to 52%. The grafting samples of (PE-g-PAAc)/Fe3O4 led to a significant increase in the hydrophilic characterization of PE wax.
3.5. Testing the dye removal efficiency of PE-g-PAAc
To determine the removal efficiency of 0.05 g PE-g-PAAc as dye adsorbents, 3 ml of test dye solution (5 × 10−5 M) TB was taken (scheme 1). At room temperature the change of colour was observed after 20 min and the difference between (a) PE/Fe3O4 and (b) (PE-g-PAAc)/Fe3O4 in Figure clearly indicated. The adsorbent efficiency of PE/Fe3O4 was enhanced after PAAc grafting and the decolourization reached 78% after 1 h.
4. Conclusion
Radiation-induced grafting of AAc is a successful method for preparing (PE-g-PAAc)/Fe3O4. The grafted PAAc layers introduce a hydrophilic property beside the hydrophilic property of PE wax and Fe3O4 feed magnetic property. This indicates that (PE-g-PAAc)/Fe3O4 can be used as a safe dye adsorbent. Functionalization of PE wax surfaces (PE-g-PAAc)/Fe3O4 shown a removal percentage about 78%.
Additional information
Notes on contributors
Mohamed Mohamady Ghobashy
The author is a specialist in: (1) nanotechnology applications; (2) the author’s specific interests are: Ionic Polymer Metal Composite (IPMC), as electroactive polymer (EAP) and magnetic polymer; (3) application of radiation chemistry for synthesis of polymer and hydrogel with a wide range biomedical application; other kind of polymeric materials likes (Organogel, fabric polymer and grafted films for different applications like self-cleaning and self-healing hydrogel
References
- Ghobashy, M.M.; Khafaga, M.R. Radiation Synthesis and Magnetic Property Investigations of the Graft Copolymer Poly (Ethylene-g-Acrylic Acid)/Fe3O4 Film. J. Supercond. Novel Magn. 2016, 30, 401–406.
- Ghobashy, M.M. Effect of sulfonated groups on the proton and methanol transport behavior of irradiated PS/PEVA membrane. Int. J. Plast. Technol. 2017. doi:10.1007/s12588-017-9176-5.
- Ghobashy, M.M.; Reheem, A.M.; Mazied, N.A. Ion Etching Induced Surface Patterns of Blend Polymer (Poly Ethylene Glycol – Poly Methyl Methacrylate) Irradiated with Gamma Rays. Int. Polym. Process. 2017, 32, 174–182.10.3139/217.3258
- Ghobashy, M.M.; Elhady, M.A. pH-Sensitive Wax Emulsion Copolymerization with Acrylamide Hydrogel Using Gamma Irradiation for Dye Removal. Radia. Phys. Chem. 2017, 134, 47–55.10.1016/j.radphyschem.2017.01.021
- Ghobashy, M.M.; Khafaga, M.R. Chemical Modification of Nano Polyacrylonitrile Prepared by Emulsion Polymerization Induced by Gamma Radiation and Their Use for Removal of Some Metal Ions. J. Polym. Environ. 2017, 25, 343. doi:10.1007/s10924-016-0805-4.
- Younis, S.A.; Ghobashy, M.M.; Samy, M. Development of Aminated Poly(Glycidyl Methacrylate) Nanosorbent by Green Gamma Radiation for Phenol and Malathion Contaminated Wastewater Treatment. J. Environ. Chem. Eng. 2017, 5(3), 2325–2336. ISSN 2213-3437. doi:10.1016/j.jece.2017.04.024.
- Elbarbary, A.M.; Ghobashy, M.M. Controlled Release Fertilizers Using Superabsorbent Hydrogel Prepared by Gamma Radiation. Radiochim. Acta 2017, 105(10), 865–876. doi:10.1515/ract-2016-2679.
- Elbarbary, A.M.; Ghobashy, M.M. Phosphorylation of Chitosan/HEMA Interpenetrating Polymer Network Prepared by γ-Radiation for Metal Ions Removal from Aqueous Solutions. Carbohyd. Polym. 2017, 162, 16–27.10.1016/j.carbpol.2017.01.013
- Ghobashy, M.M.; Elhady, M.A. Radiation Crosslinked Magnetized Wax (PE/Fe3O4) Nano Composite for Selective Oil Adsorption. Compo. Commun. 2017, 3, 18–22.10.1016/j.coco.2016.12.001
- Ghobashy, M.M. Combined Ultrasonic and Gamma-Irradiation to Prepare TiO2 @PET-g-PAAc Fabric Composite for Self-Cleaning Application. Ultrason. Sonochem. 2017, 37, 529–535.10.1016/j.ultsonch.2017.02.014
- Arkatkar, A.; Arutchelvi, J.; Bhaduri, S.; Uppara, P.V.; Doble, M. Degradation of Unpretreated and Thermally Pretreated Polypropylene by Soil Consortia. Int. Biodeter. Biodegrad. 2009, 63, 106–111.10.1016/j.ibiod.2008.06.005
- Ishii, M.; Nakahira, M. Infrared Absorption Spectra and Cation Distributions in (Mn, Fe)3O4. Solid State Commun. 1972, 11, 209–212.10.1016/0038-1098(72)91162-3