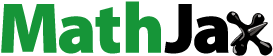
Abstract
Garlic is the most widely used bulb crop next to onion in Ethiopia. It has been used in many local peoples of Ethiopia as a flavoring agent in food and medicinal value for different diseases. The concentrations of eight heavy metals (Fe, Zn, Mn, Cu, Co, Ni, Cd, and Pb) in soil samples used for cultivation of garlic in East Gojjam Zone (Debre Markos, Dejen, Bichena, and Debre Werk) in Ethiopia were determined. The results obtained revealed that the mean heavy metal concentrations for Fe, Zn, Mn, Cu, Co, Ni, Cd, and Pb were 23,866–32,262 mg/kg, 137.1–213.4 mg/kg, 401.8–583.7 mg/kg, 52.1–77.3 mg/kg, 106.6–177.6 mg/kg, 87.5–123.5 mg/kg, 2.3–2.5 mg/kg, and 13.8–23.2 mg/kg, respectively. In general, the levels of metals in soil collected from all the sampling sites was found to decreased in the order of: Fe > Mn > Zn > Co > Ni > Cu > Pb > Cd. In general, the results reveal that the level of contamination of the soils by the heavy metals is not high at present and the soil is not polluted by toxic heavy metals (Cd and Pb). Therefore, the soils studied were not harmful for cultivation of garlic and other agricultural purposes.
Keywords:
Public Interest Statement
Garlic has been used throughout recorded history for both culinary and medicinal purposes. The soil condition is of great importance, because it is a universal medium for plant growth, which supplies essential nutrients to the plants. There is also a risk of contamination of soils with excess heavy metals because of atmospheric deposition, the use of pesticides and fertilizers that contain considerable amounts of metals. Therefore, it is the aim of this work to prove that the heavy metal content in the garlic growing soil has significant difference based on the soil type in East Gojjam Zone, Amhara Regional State, Ethiopia where garlic is growing abundantly. The results of this study reveal that the level of contamination of the soils by the heavy metals is not high at present and the soil is not polluted by toxic heavy metals. It is essential to asses the levels of heavy metals in soil used for agricultural activities to minimize the risk of contamination of food by heavy metals. Therefore, the soils studied were not harmful for cultivation of garlic and other agricultural purposes.
Competing Interests
The authors declare no competing interest.
1. Introduction
Soil is unconsolidated minerals and organic material found on the immediate earth surface that serves as a natural medium for plants growth and other developmental activities (Citation1). Soil is composed of mineral constituents, organic matter (humus), living organisms, air, and water, and it regulates the natural cycles of these components (Citation2, 3). Heavy metals occur naturally in soils, which are formed by geological processes, such as alteration and erosion of the geological underground materials (Citation4, 5). Besides the parent material, the sources of contamination in soils are multifarious, and include agricultural and industrial pollution (Citation4). High rate of run-off and soil losses are the main driving forces to transport the pollutants (Citation6, 7) thus soil erosion processes based on nature-based solutions have to be found (Citation3).
Environmental contamination by heavy metals has become a world-wide problem during recent years due to the fact that heavy metals unlike some other pollutants are not biodegradable (Citation8). Consequently, they are not detoxified but are bioaccumulated in the environment. Heavy metal refers to any metallic element whose density is relatively high and is toxic or poisonous even at low concentration (Citation9). Heavy metals are metals and metalloids having densities greater than 5 g/cm3 (Citation10, 11). Heavy metals are released into the environment through man’s industrial, domestic and commercial activities, industrial effluents, pesticides and fungicides as well as manure from poultry farms (Citation10). Soil pollution by heavy metals has serious health implication especially with regards to crops/vegetables grown on such soils (Citation12, 13). Most of these heavy metals are necessary for growth and normal functions of both plants and animals at trace amount such as Fe, Zn, Mn, Cu, Co and Ni but large amount of any of them may cause acute or chronic toxicity. Long-term effect of heavy metal exposure to human and higher animals includes mental lapse, kidney failure, and central nervous system disorder (Citation13). For example, chronic exposure of Cd can have harmful effects such as lung cancer, bone fractures, kidney dysfunction, hypertension. Exposure to lead (Pb) may cause plumbism, anemia, nephropathy, gastrointestinal colic, and central nervous system symptoms (Citation14).
Heavy metals occupy a special position in soil chemistry because they play very important physiological roles in nature (Citation15, 16). Generally, topsoil layer contain largest amount of pollutants. The contaminant concentration in soil mainly depends on the adsorption properties of soil matter. The solubility of heavy metal ions in soil was mainly influence by many factors such as pH, conductivity, moisture content. (Citation17). As a result of increasing anthropogenic activities, heavy metals pollution of soil, water, and atmosphere represents a growing environmental problem affecting food quality and human health. Heavy metals may enter the food chain as a result of their uptake by edible plants (Citation18).
Garlic (Allium sativum L.) has been used throughout recorded history for both culinary and medicinal purposes (Citation19). It is the most widely used bulb crop next to onion in Ethiopia. It contributes significant nutritional value to the human diet as a seasoning in many foods (Citation20). In addition to its flavoring agent in food, it has also been used in many local peoples of Ethiopia as a medicinal value for different diseases (Citation21). The yield of crop depends on fertility, presence of micronutrients and heavy metals in the soil. The soil condition is of great importance, because it is a universal medium for plant growth, which supplies essential nutrients to the plants (Citation22, 23). There is also a risk of contamination of soils with excess heavy metals because of atmospheric deposition, the use of pesticides and fertilizers that contain considerable amounts of metals (Citation22). East Gojjam Zone of the Amhara Regional State of Ethiopia is one of the areas in which garlic is growing abundantly in the country. However, information about micronutrient and heavy metal contents in soils in the study areas are scarce. Therefore, the aim of this study is to know the heavy metal content of the soil used for garlic growing in East Gojjam Zone of Amhara Regional State, Ethiopia.
2. Materials and methods
2.1. Description of the study area
This research was carried out in selected sites of four different agricultural locations of East Gojjam Zone of the Amhara Regional State, Ethiopia namely: Debre Markos, Dejen, Bichena, and Debre Werk areas. The climate condition of the study sites is shown in Table and the location of the study area is shown in Figure .
Table 1. Description of the climate conditions of the study sites.
2.2. Equipements
Digestive furnace (Model: KDN-20C, China) was used to digest the samples. Flame atomic absorption spectrophotometer (Model: AA-320N, Shanghai, China) equipped with deuterium lamp background correction and hollow cathode lamps was used for the analysis of the heavy metals (Fe, Zn, Mn, Cu, Co, Ni, Cd, and Pb). Electronic analytical balance (AA-200DS, Deriver Instrument Company, Germany) was used for weighing the samples.
2.3. Chemicals and reagents
Aqua regia (prepared from 3:1 ratio of 35–38% HCl and 65–68% HNO3) and 30% H2O2 (Uni-Chem® Chemical reagent, India) were used for sample digestion. Li2CO3 (99%, KIRAN LIGHI, Laboratories, India) were used as a matrix for the method blank. 1000 ppm stock standard solutions of the heavy metals Fe, Zn, Mn, Cu, Co, Ni, Cd, and Pb (India) were used to prepare calibration standard and spiking standard solutions. Double distilled water was used throughout the study. The glassware and polyethylene containers used for analysis were washed with tap water, then soaked overnight in 10% (v/v) HNO3 solution and rinsed several times with double distilled water to eliminate absorbance due to detergent.
2.4. Soil sample collection and pretreatment
A total of 100 soil samples were collected in February 2014 from the four different agricultural areas that are used for cultivation of garlic using soil sampler. The soil samples were collected from topsoil at the depths of 0–20 cm using soil auger. From each of the four agricultural sites, five sub-sites were taken for the purpose of random sampling and five soil samples were randomly collected from each of the five sub-sites in each four agricultural areas and pooled together to obtain a composite sample. Finally, four soil bulk samples one from each stated areas were transferred in to polyethylene bags and transported to the laboratory. The samples were air dried for one week, ground with porcelain mortar and pestle, passed through 0.5 mm sieve, and then kept in clean polythene bags for further analysis.
2.5. Sample digestion
Wet digestion method was used for digestion of the soil samples. 0.5 g of each of the air-dried, ground, and sieved soil samples was accurately weighted into a digestion tube. 6 ml aqua regia and 1.5 ml H2O2 were measured and added into the digestive tube and swirled gently to mix the sample properly. The digestion tubes were then placed on digestive furnace (Model: KDN-20C, China) and heated at a temperature of 180°C for 3 h. All the digests were cooled and filtered through Whatman No.42 filter paper and diluted to 50 ml by double distilled water. Each sample was digested in replicates of five and transferred to acid-washed stoppered glass bottle, labeled and kept for metal analysis.
2.6. Method validation and quality control
In order to validate the analytical method, the following method validation parameters such as instrumental detection limit, limit of detection, limit of quantification, precision and accuracy studies were carried out (Citation24, 25).
2.6.1. Instrumental detection limit
Instrumental detection limit (IDL) is the smallest signal above background noise that an instrument can detect reliably. The IDL is calculated to be the concentration equal to three times the standard deviation of the blank signal (Citation24). In this study, IDL for each metal was determined from analysis of seven replicates of calibration blank and the concentration was calculated as:
where sbl is standard deviation of the calibration blank.
2.6.2. Limit of detection
Limit of detection (LOD) is the minimum concentration of analyte that can be detected but not necessarily quantified with an acceptable uncertainty. LOD for each metal was determined from analysis of seven replicates of method blanks which were digested in the same digestion procedure as the actual samples. LOD was calculated as (Citation24):
where sbl is the standard deviation of the method blank.
2.6.3. Limit of quantification
The limit of quantification (LOQ) is the lowest concentration of an analyte in a sample which can be quantitatively determined with acceptable uncertainty. LOQ was obtained from triplicate analysis of seven method blanks which were digested in the same digestion procedure as the actual samples. The LOQ was calculated as (Citation24):
where sbl is the standard deviation of the method blank.
2.6.4. Precision and accuracy
Precision and accuracy of the results were assessed by determining recovery and repeatability of the analysis of matrix spike, matrix spike duplicate and laboratory control samples. For doing so, each sample was spiked in replicates of five at near mid-range calibration concentration. The spiked sample were digested and analyzed following the same analytical procedure as the soil samples. Precision was expressed as relative standard deviation (RSD) of replicate results. The relative standard deviations of the sample were obtained as:
The percentage recoveries of the analyte were calculated to evaluate the accuracy of the analytical procedure. Recovery was then calculated as (Citation26):
2.6.5. Analysis of matrix spike and matrix spike duplicate sample
Both matrix spikes (MS) and matrix spikes (MSD) were prepared by spiking 0.5 g of each soil sample with 2 ml of a mixture of spiking standards so that the spike level were 4 mg/L of Fe, 2 mg/L of Cu, Zn, Mn, and Ni and 1 mg/L of Co, Cd, and Pb. They were all carried through the same digestion and analysis steps as the unspiked sample. The relative percent differences (RPD) between the MS and MSD results were calculated as (Citation27):
2.6.6. Analysis of laboratory control sample
Five replicates of 0.5 g lithium carbonate spiked with 2 ml of a mixture of spiking standards so that the spike level were 4 mg/L of Fe, 2 mg/L of Cu, Zn, Mn, and Ni, and 1 mg/L of Co, Cd, and Pb. These were undergoing the same digestion procedure described for the soil sample but with no added sample. The % LCS recoveries for each metal were calculated as (Citation28):
where %R = percent recovery, LCS = Laboratory Control Sample Results, S = amount of spike added.
2.6.7. Calibration blank and method blank
A calibration blank (2% HNO3) was prepared to determine the background signal and establish the baseline of an instrument. For the method blank, 0.5 g Li2CO3 was added as a matrix and prepared using the same digestion procedure described for the soil sample but with no added sample.
2.7. Determination of heavy metals
The instrument was calibrated using calibration blank and five series of working standard solutions of each metal to be analyzed. The digested samples were determined for the concentrations of heavy metals (Fe, Zn Mn, Cu, Co, Ni, Cd, and Pb) using flame atomic absorption spectrophotometer (FAAS, Model: AA-320N, Shanghai, China). Final concentrations of the metals in the soil samples were calculated using the following formula (Citation29):
where V = Final volume (50 ml) of solution, and M = Initial weight (0.5 g) of sample measured.
2.8. Instrument working conditions
The flame atomic absorption spectrophotometer working conditions are as shown in Table .
Table 2. Instrument operating conditions for the determination of heavy metals in soil samples by FAAS
2.9. Statistical analysis
Statistical analysis of the data were carried out using one-way analysis of variance (ANOVA) to assess significant variation in the mean concentrations of heavy metals in soils. A Probability level of p < 0.05 was considered statistically significant. Pearson’s correlation coefficient was used to determine the association between the heavy metals. All statistical analyses were done by SPSS version 16.0 (IBM Corp., USA) software for windows.
3. Results and discussion
3.1. Regression analysis and detection limits
As can be seen from Table , calibration curves for all the metals showed good linearity with coefficients of determination (r2) ranged between 0.9997 and 0.9999, which were greater than the acceptable limit (0.998) for the linearity of the regression line (Citation30). This showed that there is a good correlation between concentration and absorbance indicating good calibration of the instrument.
Table 3. Linear regression equations, coefficient of determination, instrumental detection limit (IDL), limit of detection (LOD), and limit of quantification (LOQ)
The instrumental detection limits (IDL) ranged from 0.003 to 0.008 mg/L which were below the method detection limits (MDL), indicating good sensitivity of the measuring instrument for analysis. The MDL value lied in the range of 0.078–0.286 mg kg−1. The method quantification limit (MQL) value lied in between 0.287 and 1.070 mg kg−1. The result shows both the MDL and MQL values were greater than the IDL; hence, the results of the analysis could be reliable.
3.2. Accuracy and precision
As it can be seen from Table , the mean percent recoveries for the studied metals in the matrix spike sample ranged between 90.2 and 101.70%. All the recovery values were within the acceptable range of 80–120% for metal analysis (Citation31). The precision of the method was expressed as RSD of the five replicate readings. The RSD values obtained for garlic matrix spike samples ranged from 0.17 to 10.32% (Table ), which was under the required control limits ≤15% (Citation32). These results indicate that the proposed method was precise and accurate.
Table 4. Recovery and precision test results of metals for soil matrix spike sample
3.3. Precision of matrix spike and matrix spike duplicate sample
The relative percent difference (RPD) values were calculated from the matrix spike and matrix spike duplicate result to measure the precision. As it can be seen from Table , the relative percent difference between matrix spike and matrix spike duplicate ranged from 1.92 to 8.37 which were in the acceptable limits ≤15% (Citation33). This showed that the overall analytical process was less affected by matrix specific variability and the data generated was in the acceptable quality range. These results indicate that the proposed method was precise.
Table 5. Relative percent difference (RPD) results of matrix spike and matrix spike duplicate analysis
3.4. Laboratory control sample results
The laboratory control sample (LCS) recoveries and relative standard deviations of each analyte are summarized in Table . The percent recovery values of the LCS lied in the range of 90.16–97.11% and the RSD ranged from 2.90 to 10.36%. All the values were found under the recommended control limits 80–120% for LCS recovery and ≤15% RSD. These results showed that the analytical method possesses the required precision and accuracy.
Table 6. Recovery and precision test results for the laboratory control samples
3.5. Concentration of heavy metals in soil samples
The mean concentrations of heavy metals in the soil samples are shown in Table and Figure . The data revealed that all the analyzed metals accumulated by the soil at different concentrations. Results are expressed as mean ± standard deviation of five replicate analyses.
Table 7. Mean concentrations of metals (mean ± SD, n = 5, mg/kg dry weight) in soil samples
The results in Table reveal that the concentration of iron (Fe) was highest among the heavy metals analyzed from all the sample sites and the levels obtained were found to be 24,563 mg/kg in Debre Markos soil, 29,266 mg/kg in Dejen soil, 32,262 mg/kg in Bichena soil, and 23,866 mg/kg in Debre Werk soil. These results found were lower than the standard set by Pendias and Pendias, (Citation33) and FAO/WHO, 2001 (Citation34). The high level of iron might be due its abundance because iron is the fourth most abundant element in the earth’s crust. The concentration of zinc (Zn) was in the range of 137.1–213.4 mg/kg. The lowest zinc content was obtained in soil collected from Debre Markos area and the highest in soil collected from Dejen areas. The concentration of zinc in this study is much lower than the standard limit set by Ewers, 1991 (Citation35). The high level of Zn in these agricultural sites could probably be due to the widely used vegetable farming activities at these areas and high usage of various types of pesticides and fertilizers. The results of manganese (Mn) concentration were 583.7, 499.8, 552.5, and 401.8 mg/kg kg in sampling sites of Debre Werk, Dejen, Bichena, and Debre Markos, respectively. The concentrations of manganese found in this study were lower than the FAO/WHO, 2001 (Citation34) and Pendias and pendias, 1992 (Citation33) recommended limit. The high manganese values in the study area indicated the high availability of Mn for plant uptake in the garlic farming area.
The concentration of copper (Cu) ranged between 52.1 and 77.3 mg/kg. The lowest concentration of copper was found in soil sample collected from Debre Markos areas while the highest concentration in Debre Werk soil. The content of Cu reported in this study was generally lower than the permissible levels set by Ewers, 1991 (Citation35) in soil. The results of cobalt (Co) concentrations were 106.6, 167.5, 177.6, and 140.7 mg/kg in sample sites of Debre Markos, Dejen, Bichena, and Debre Werk, respectively. The levels of Co obtained in this study in all the sampling sites were higher than Ewers, 1991 (Citation35) recommended limit which is 50 mg/kg. Wide application of various types of pesticides and fertilizer may be contributed to the increased availability of Co in the soil. The average concentrations of nickel in soil grown in Debre Markos, Dejen, Bichena and Debre Werk were 87.5, 104.8, 100.2, and 123.5 mg/kg, respectively. The contents of nickel found in this study were higher than the Ewers (1991) recommended limit. Wide application of various types of pesticides and fertilizer may be contributed to the increased availability of Ni in the soil.
Cadmium (Cd) was the least abundant element in the soil samples studied. The cadmium content of soil in all the sampling sites were almost similar that is 2.50 mg/kg in Debre Markos soil, 2.3 mg/kg in Dejen and Debre Werk soil, and 2.4 mg/kg in Bichena soil. The high level of cadmium might be due to the use of cadmium-containing phosphate fertilizers and contamination from cadmium-containing dusts. The concentrations of cadmium obtained in this study were lower than Ewers, 1991(Citation35) recommended maximum limit for soil that is 3 mg/kg. From this study also it was found that various agricultural activities carried out in the areas were not able to increase the Cd content in the soils. In the studied soil samples, the results of lead (Pb) concentrations were 15.6, 13.8, 23.2, and 13.9 mg/kg in sample sites of Debre Markos, Dejen, Bichena, and Debre Werk, respectively. The relatively high levels of lead might have resulted from accumulation of lead through air pollution such as automobile exhaust fumes and from some pesticides, such as lead arsenates applied during cultivation. The values of Pb obtained in this study were lower than Ewers, 1991(Citation35) recommended maximum limit that is 100 mg/kg.
In general, the results of the heavy metals analyzed in the study areas showed that their concentration level is below the standard guide lines for maximum limit proposed for agricultural soil except in the case of cobalt and nickel. Even though these heavy metal concentrations fell below the critical permissible concentration level in most heavy metals, it seems that their persistence in the soils of the study site may lead to increased uptake of these heavy metals by plants.
One-way analysis of variance (ANOVA) was made at 95% confidence level. The results showed that there was significant differences (p < 0.05) in the concentrations of the heavy metals Fe, Zn, Cu, Mn, Co, and Pb among the analyzed soil samples while there was no significant difference (p > 0.05) in the concentrations of Ni and Cd. In general, the mean concentration of heavy metals in soils collected from all sampling site decreased in the order of: Fe > Mn > Zn > Co > Ni > Cu > Pb > Cd.
3.6. Correlation analysis
Pearson’s correlation coefficient was used to examine the relationship between the various heavy metals in the soil samples from all the sample sites. Table shows the correlation matrix of the relationship between heavy metals concentration of soil samples.
Table 8. Correlation coefficient (r) matrix for heavy metals concentration in soil samples
Rakesh and Raju (2013) (Citation17) reported that high correlation coefficient (near +1 or −1) means a good relation between two variables, and its concentration around zero means no relationship between them at a significant level of 0.05% level, it can be strongly correlated, if r > 0.7, whereas r values between 0.5 and 0.7 shows moderate correlation between two different parameters.
As can be seen from Table , the results of the correlation coefficients showed strong positive correlation between Co with Fe (r = 0.853), Ni with Zn (r = 0.794) Ni with Cu (r = 0.981), Cd with Mn (r = 0.837), and Pb with Fe (r = 0.736). This strong positive correlation shows that the elements are closely associated, thus suggesting their common origin. There were also moderate positive correlations Cu with Zn (r = 0.662) and Co with Zn (r = 0.675). Strong negative correlations were found between Zn with Mn (r = −0.757), Zn with Cd (r = −0.991), Mn with Cu (r = −0.960), Mn with Ni (r = −0.980), and Cd with Ni (r = −0.859). Moderate negative correlations were found between Cd with Cu (r = −0.44) and Cd with Co(r = −0.587). The other elements have weak negative or positive correlation indicating that the presence or absence of one element affect in lesser extent to the other.
4. Conclusion
The concentrations of heavy metals in soil samples used for cultivation of Garlic in East Gojjam Zone of the Amhara Regional State Ethiopia have been determined. The study indicates that the soils serve as the potential source of the heavy metals in the environment and the concentration of the heavy metals: Fe, Zn, Mn, Cu, Cd, and Pb are all far below the maximum tolerable levels set by Ewers and FAO/WHO but the values of Co and Ni were higher than maximum tolerable levels set by Ewers. The significance difference and increase in the elemental concentrations may be due to application of various types of pesticides and fertilizer in the vegetable farming areas. In general, the results also show that the level of contamination of the soils by the heavy metals is not high at present and the soil is not polluted by toxic heavy metals (Cd and Pb). Therefore, the soils studied were not harm for the cultivation of garlic and other agricultural purposes, so there is no serious implication for health hazard. In conclusion, the present study will give brief information about the heavy metal contents of the soil, and these results may serve as a base line data for determination of mineral contents and physicochemical properties of the soil in the study area.
Funding
This work was supported by the Ambo University [grant number AU00014/2008].
Additional information
Notes on contributors
Wodaje Addis
Wodaje Addis, Alemayehu Abebaw have spent together as a member of a research group for couple of years mainly focusing on the determination of essential and non-essential metal concentration in garlic and soil sample using different analytical techniques. Our research group has equipped with the state-of-the-art analytical instruments which are capable of determining heavy metals in food and soil samples. Besides, the quality control, method development and statistical data analysis are also main components of our research. In addition, the authors have the experience with the analysis of environmental samples. In wider context, the research group has been working in the analysis of food, plant, soil, and water samples using analytical instruments available in their laboratory.
References
- Haliru, H.A.; Ling, L.P.; Suhaiza, S.O. Heavy Metal Concentration Levels in Soil at Lake Geriyo Irrigation Site, Yola, Adamawa State, North Eastern Nigeria. Int. J. Environ. Monit. Anal. 2014, 2, 106–111. doi:10.11648/j.ijema.20140202.17.10.11648/j.ijema.20140202.17
- Keesstra, S.D.; Geissen, V.; Mosse, K.; Piiranen, S.; Scudiero, E.; Leistra, M., Schaik, L.V. Soil as a Filter for Groundwater Quality. Curr. Opin. Environ. Sustain. 2012, 4 (5), 507–516. doi:10.1016/j.cosust.2012.10.00710.1016/j.cosust.2012.10.007
- Keesstra, S.D.; Bouma, J.; Wallinga, J.; Tittonell, P.; Smith, P.; Cerdà, A.; Montanarella, L.; Quinton, J.N.; Pachepsky, Y.; Putten, W.H.V.; Bardgett, R.D.; Moolenaar, S.; Mol, G.; Jansen, B.; Fresco, L.O. The Significance of Soils and Soil Science Towards Realization of the United Nations Sustainable Development Goals. Soil. 2016, 2, 111–128. doi:10.5194/soil-2-111-2016.
- Moor, C.; Lymberopoulou, T.; Dietrich, V.K. Determination of Heavy Metals in Soils, Sediments and Geological Materials by ICP- AES and ICP-MS. Mikrochim. Acta. 2001, 136, 123–128.10.1007/s006040170041
- Kabir, E.; Ray, S.; Kim, K.H.; Yoon, H.O.; Jeon, E.H.; Kim, Y.S.; Cho, Y.S.; Yun, S.T.; Brown, R.J.C. Current Status of Trace Metal Pollution in Soils Affected by Industrial Activities. Sci. World J. 2012, 1–18. doi:10.1100/2012/91670510.1100/2012/916705
- Rodrigo-Comino, J.; Brings, C.; Iserloh, T. Temporal Changes in Soil Water Erosion on Sloping Vineyards in the Ruwer-Mosel Valley. The Impact of Age and Plantation Works in Young and Old Vines. J. Hydrol. Hydromech. 2017, 65 (4), 402–409. doi:10.1515/johh-2017-0022
- Rodrigo-Comino, J.; Cerdà, A. Improving Stock Unearthing Method to Measure Soil Erosion Rates in Vineyards. Ecol. Indic. 2018, 85, 509–517. doi:10.1016/j.ecolind.2017.10.042.
- Bazrafshan, E.; Mohammadi, L.; Ansari-Moghaddam, A.; Mahvi, A.H. Heavy Metals Removal from Aqueous Environments by Electrocoagulation Process– A Systematic Review. J. Environ. Health Sci. Eng. 2015, 13, 74, 1–16. doi:10.1186/s40201-015-0233-8
- Inobeme, A.; Ajai, A.I.; Iyaka, Y.A.; Ndamitso, M.; Uwem, B. 2014. Determination of Physicochemical and Heavy Metal Content of Soil Around Paint Industries in Kaduna. Int. J. Sci. Technol. Res. 2014, 3 (8), 221–225.
- Ukpong, E.C.; Antigha, R.E.; Moses, E.O. Assessment of Heavy Metals Content in Soils and Plants Around Waste Dumpsites in Uyo Metropolis, Akwa Ibom State. The IJES. 2013, 2 (7), 75–86.
- Tutic, A.; Novakovic, S.; Lutovac, M.; Biocanin, R.; Ketin, S.; Omerovic, N. The Heavy Metals in Agrosystems and Impact on Health and Quality of Life. Maced. J. Med. Sci. 2015, 3 (2), 345–355. doi:10.3889/oamjms.2015.048.
- Steffana, J.J.; Brevika, E.C.; Burgess, L.C.; Cerda, A. The Effect of Soil on Human Health: an Overview. Eur. J. Soil Sci. 2017, 1–13. doi:10.1111/ejss.12451.
- Nwaogu, L.A.; Ujowundu, C.O.; Iheme, C.I.; Ezejiofor, T.N.I.; Belonwu, D.C. Effect of Sublethal Concentration of Heavy Metal Contamination on Soil Physicochemical Properties, Catalase and Dehydrogenase Activities. Int. J. Biochem Res. Rev. 2014, 4 (2), 141–149. doi:10.9734/IJBCRR/2014/6341.
- Hu, B., Jia, X.; Hu, J.; Xu, D.; Xia, F.; Li, Y. Assessment of Heavy Metal Pollution and Health Risks in the Soil-Plant-Human System in the Yangtze River Delta, China. Int. J. Environ. Res. Public Health. 2017, 14 (9), 1042: 1–18. doi:10.3390/ijerph14091042
- Akpoveta, O.V.; Osakwe, S.A.; Okoh, B.E.; Otuya, B.O. Physicochemical Characteristics and Levels of Some Heavy Metals in Soils Around Metal Scrap Dumps in Some Parts of Delta State, Nigeria. J. Appl. Sci. Environ. Manage. 2010, 14 (4), 576–660.
- Oves, M.; Saghir, K.M.; Huda, Q.A.; Nadeen, F.M.; Almeelbi, T. Heavy Metals: Biological Importance and Detoxification Strategies. J. Bioremediat. Biodegrad. 2016, 7 (2), 1–15. doi:10.4172/2155-6199.1000334.
- Rakesh, S.M.; Raju, N.S. Correlation of Heavy Metal Contamination with Soil Properties of Industrial Areas of Mysore, Karnataka, India by Cluster Analysis. Int. Res. J. Environment Sci. 2013, 2 (10), 22–27.
- Shaapera, U.; Nnamonu, L.A.; Eneji, I.S. Assessment of Heavy Metals in Rana esculenta Organs from River Guma, Benue State Nigeria. Am. J. Anal. Chem. 2013, 4 (09), 496–500. doi:10.4236/ajac.2013.49063.
- Karuppiah, P.; Rajaram, S. Antibacterial Effect of Allium sativum Cloves and Zingiber officinale Rhizomes Against Multiple-drug Resistant Clinical Pathogens. Asian Pac. J. Trop. Biomed. 2012, 2 (8), 597–601. doi:10.1016/S2221-1691(12)60104-X.
- Diriba, S.G.; Kebede, W.; Nigussie, D.R.; Getachew, T.; Sharma, J.J. Postharvest Quality and Shelf Life of Garlic Bulb as Influenced by Storage Season, Soil Type and Different Compound Fertilizers. J. Postharvest. Technol. 2013, 1 (1), 69–83.
- Wodaje, A.; Alemayehu, A. Levels of Essential and Non-essential Metals in Garlic (Allium sativum L.) Samples Collected from Different Locations of East Gojjam Zone, Ethiopia. JoMCCT, 2014, 5 (3), 10–19.
- Pujar, K.G.; Hiremath, S.C.; Pujar, A.S.; Pujeri, U.S.; Yadawe, M.S. Analysis of Physico-Chemical and Heavy Metal Concentration in Soil of Bijapur Taluka, Karnataka. Sci. Revs. Chem. Commu. 2012, 2 (1), 76–79.
- Tripathi, I.P.; Dwivedi, A.P. Heav Metal Analysis of Soil Samples Collected from in and Around Satna. IJIRR. 2015, 2 (3), 516–520.
- Prichard, E.; Barwick, V. Quality Assurance in Analytical Chemistry. John Wiley and Sons: New York, ISBN 978-0131291928, 2007, pp 86–88
- Mitra, S. Sample Preparation Techniques in Analytical Chemistry. John Wiley and sons, Inc.: Hoboken, 2003, pp 162, 6–244.
- Javed, I.; William, A.C.; Shelly, L.C.; Theegala, C.S. Metals Determination in Biodiesel (B100) by ICP-OES with Microwave Assisted Acid Digestion. Open Anal. Chem. J. 2010, 4, 18–26. doi:10.2174/1874065001004010018.
- USEPA. Method 200.9: Trace Elements in Water, Solids, and Biosolids by Stabilized Temperature Graphite Furnace Atomic Absorption Spectrometry, Revision 3.0, EPA-821-R-01-011. Washington, D.C., 2001.
- USEPA. National Functional Guidelines for Inorganic Superfund: Data Review. USEPA- 540-R10-011, Washington, D.C., 2010.
- Uwah, E.I.; Gimba, M.S.; Gwaski, P.A. Determination of Zn, Mn, Fe and Cu in Spinach and Lettuce Cultivated in Potiskum, Yobe State, Nigeria. J. Agri. Econo. Develo. 2012, 1 (4), 69–74.
- Christian, G.D. Analytical Chemistry, 6th ed.; John Wiley and Sons: New York, ISBN 0-471451622, 2003; p 128.
- Harvey, D. Modern Analytical Chemistry, 1st ed.; McGraw-Hill, Depauw University: USA, ISBN 0-072375477, 2000, pp 709.
- Ararso, N.; Alemayeh, A. Determination of Selected Essential and Non-essential Metals in the Stems and Leaves of Rhamnus prinoides (gesho). Sci. Technol. Arts Res. J. 2013, 2 (4), 20–36. doi:10.4314/star.v214.5.
- Pendias, A.K.; Pendias, H. Elements of Group Viii. In Trace Elements in Soils and Plants. CRC Press: Boca Raton, 1992, pp 271–276.
- FAO/WHO (Codex Alimentarius Commission). Food Additives and Contaminants. Joint FAO/WHO Food Standards Program, ALINORM 01/12A, 2001; pp 1–289.
- Ewers, U. Standards, Guidelines and Legislative Regulations Concerning Metals and Their Compounds. In Metals and Their Compounds in the Environment: Occurrence: Merian, E., Ed.; Analysis and Biological Relevance, VCH: Weinheim, 1991; pp 458–468.