Abstract
Reactions between Al containing materials and counterfeit refrigerant chloromethane (R-40) were investigated under various conditions. The addition of Mg effected a reaction indicating that trimethylaluminum may have been produced in the reaction. A different study involving Al-foil, AlCl3 and chloromethane resulted in cracks detected on the Al-foil which reduced in weight by 19% and methane was detected. Studies conducted either at room temperature or at 80°C with two other genuine refrigerants, R-142b (1-chloro-1,1-difluoroethane) and R-133a (2-chloro-1,1,1-trifluoroethane) did not form organoaluminum compounds. Several commercially available refrigerant oils were subjected to Me3Al. Only polyester oil resulted in a decrease in the quantity of methane released upon final deactivation with alcohol. Various chemicals were added to Me3Al in order to find which ones would react and not produce methane. Phthalaldehyde was determined to be the most efficient reagent by deactivating approximately 50% of the methyl groups on Me3Al.
PUBLIC INTEREST STATEMENT
R-40 (chloromethane) was detected in air conditioning systems of some military vehicles used overseas. Several accidents were reported from misuse of this counterfeit refrigerant in vehicle air conditioners. It is claimed that production of trimethylaluminum or similar could cause fire and explosion in the systems. By looking into chemistry of this issue, we found that under certain circumstances, R-40 could react with aluminum to produce explosive trimethylaluminum or similar. We also studied the reactions of trimethylaluminum with refrigerant oils on market, trying to find a cheap solution to deactivate trimethylaluminum and solve the issue.
1. Introduction
Recently there have been reports on the use of cheap substitute refrigerants and the dangers associated with these counterfeit refrigerants distributed as 1,1,1,2-tetrafluoroethane R-134a. These reports are freely available from online sources and include reports on the dangers (several units experienced fires or flashing, five explosions with three known deaths) of counterfeit refrigerants (http://www.acr-news.com/fake-refrigerants-we-should-be-very-concerned, accessed 30 July 2012; http://www.labnews.co.uk/features/a-chilling-story/, accessed 23 October 2012), magazine articles on the subject (Peter, Citation2012), reports at conferences (Sassano, Citation2012) and a hazard alert warning from at least one government agency (Ministry of Business, Citation2012).
These reports would suggest that the danger arises out of the substitution of chloromethane (R-40) for R-134a apparently due to a price spike for R-134a and a glut of HCFCs (http://www.acr-news.com/fake-refrigerants-we-should-be-very-concerned, accessed 30 July 2012). In the scientific literature, chloromethane as a refrigerant has been assessed as a poison (Loizaga & De Loizaga, Citation1954; Vilches, Citation1948), and its fire and explosion hazard effects are also detailed (Nuckolls, Citation1933). Interestingly, there is one casualty report but this was from repeated exposure of a refrigerant worker to several different refrigerants including chloromethane (Mendelhoff, Citation1952). More recently, there was a report on the explosive nature of the vapor of chloromethane (Lindborg, Citation2003).
There does appear to be some conflicting reports on exactly what can occur if R-134a is contaminated with R-40. For example, one reads of R-40 reacting with R-134a producing HF which would then cause a compressor to explode (http://www.acr-news.com/fake-refrigerants-we-should-be-very-concerned, accessed 30 July 2012). More likely is the reaction between chloromathen and the aluminum in the compressor to produce trimethylaluminum which is pyrophoric (Peter, Citation2012). In light of these concerns, we decided to investigate under what conditions monochlorotrifluoroethane, R-133a, R-40, and 1-chloro-1,1-difluoroethane, R-142b would react with Al and the nature of the reaction between Me3Al and various commercially available refrigerant oils. We also report on safe methods to deactivate Me3Al consisting of exploring which chemicals would react with Me3Al and not result in methane. These substances could then be added to refrigeration systems if warranted.
2. Experimental
2.1. General method
Infrared spectra were obtained either on a PerkinElmer Spectrum one FT-IR spectrometer or a Nicolet IR-200 spectrometer. A Shimadzu GC-MS-QP5050 was used to identify gas and liquid samples. Mass spectra of oils were obtained on a Bruker Microflex LRF MALDI-TOF. A Hitachi S-4700 FE-SEM with EDS was used to characterize the surface structure and composition of solid samples. Solvents were used as received from commercial suppliers. Chloromethane (R-40) and 1-chloro-1,1-difluoroethane (R-142b) were purchased from Aldrich, and 2-chloro-1,1,1-trifluoroethane (R-133a) was purchased from Matrix Scientific. All refrigerant oils are commercially available and used as received. Trimethylaluminum, benzaldehyde, phthalaldehyde, cyclohexanone, ethyl acetate, Al beads and foils were purchased from Aldrich. Benzophenone and sec-butanol were purchased from Alfa Aesar and EMD Chemicals, respectively. All chemicals above were used as received. Reactive aluminum dust was produced by filing chunks of Al metal in glove box and stored under nitrogen.
2.2. Refrigerant gases reactions either with Al or Al and other metal compounds
2.2.1. Chloromethane reactions with Al at high temperature and pressure
Different Al forms including aluminum beads, chunks, dust and foil were used for tests. A total of 0.3–0.5 g of Al material was placed in a 300 mL Parr bomb and evacuated. In some experiments, 5 mL of 2012 Supercool PAG 46 or PAG 100 oil was added with the Al material. CH3Cl was transferred to the Parr bomb until a pressure of 70–80 psi was attained. This was heated to 100°C resulting in a pressure of 120–400 psi (variance depended on quantity of CH3Cl transferred). This was kept for either 4 or 5 days in this state (see Table ). The valve on the Parr bomb was opened to release gas until close to atmosphere pressure. A septum was put on the valve and an air-tight syringe was used to extract a sample for GC-MS. 10 mL sec-butanol was injected into the Parr bomb through the septum and the valve was closed immediately. The mixture was stirred for hours and then characterized.
Table 1. A summary of chloromethane reactions with Al containing materials in Parr bomb
2.2.2. Chloromethane reactions with Al, Mg mixture
A similar experiment to 2.2.1 was conducted, but 0.5 g Al beads and 0.25 g Mg powder mixture were used instead of pure aluminum. Reactions between chloromethane and Al, Mg mixture were also conducted following a similar procedure detailed in 2.2.3 except that 0.3 g Al dust and 0.15 g Mg powder were examined with CH3Cl.
2.2.3. Chloromethane reactions with Al, AlCl3 (or MgCl2) mixture
A total of 0.2–0.3 g Al material and 0.15–0.2 g AlCl3 (or MgCl2) were placed in a 500 mL two-neck flask. The system was evacuated and CH3Cl gas was delivered under mercury bubbler pressure. The system was heated to 100°C and kept in this state for 1 week. The reaction was cooled and 3 mL of 2012 Supercool PAG 46 was injected and stirred for 1 h, followed by 3 mL of sec-butanol. The gas was collected by an air-tight syringe for GC-MS. The materials in the flask were also subjected to IR, MALDI-TOF and SEM characterization. The chloromethane reaction with Al, AlCl3 mixture was conducted in a similar manner, except that the 2012 Supercool PAG 46 oil was added into the mixture before CH3Cl gas transfer.
2.2.4. 1-Chloro-1,1-difluoroethane (R-142b) or 2-chloro-1,1,1-trifluoroethane (R-133a) reactions with either Al or Al and other metal compounds
Similar experiments to 2.2.3 were conducted, but under R-142b or R-133a gas instead of R-40. GC-MS was used to identify the gaseous products and EDS used to identify the elements in the solids.
2.3. Me3Al reactions with various commercially available refrigerant oils
The seven oils studied were 3GS Suniso oil, Behr Hella PAO oil 68, 2012 Supercool PAG 46 and PAG 100 oil, 2014 Supercool PAG 46 oil, Four seasons ester 100 oil, and Napa PAG 46 oil. It is important to note that the various commercially available PAG oil samples are not identical based on comparison of their FTIR and MALDI-TOF spectra (see supplementary materials). For that reason, we are labelling them based on brand and year of manufacture.
A total of 6 g of oil were placed in a two-neck flask and evacuated at 50°C for 1 h. The system was then flushed with nitrogen and 1 ml of Me3Al (2M) solution in heptane was injected at room temperature. Gas was collected and measured by any displacement of water from a 250 mL inverted graduate. When gas evolution stopped from the initial reaction, 4 mL sec-butanol was injected and the volume of gas was measured. The final solution was characterized by MALDI-TOF and FTIR. These reactions were also conducted at 80°C.
2.4. Me3Al reactions with various deactivating reagents
The deactivating reagents used were benzaldehyde, phthalaldehyde, benzophenone, cyclohexanone and ethyl acetate. The general procedure which used an inverted graduated cylinder to measure the volume of gas evolution is as follows: fresh and excess deactivating reagent (e.g. 1.5 mL of benzaldehyde) was placed in a two-neck flask. The system was evacuated and then flushed with nitrogen. 1 mL Me3Al (2 M) solution in heptane was injected into the system and stirred until gas evolution ceased. The gas volume was measured. A total of 4 ml of sec-butanol was injected and the volume of gas measured again. The liquid mixture was characterized using FTIR and GC-MS. Similar reactions were conducted at 80°C.
3. Results and discussion
3.1. Under what conditions would refrigerant gases react with Al containing materials?
3.1.1. Chloromethane reactions with Al
We find that Al did not react with chloromethane gas under high temperature and pressure (Table , entry 1 and 2). This experiment was also conducted with some other species that could exist in an air conditioning (AC) system, including PAG oil (entry 3–6), trace water (entry 7). The reactions with PAG oil and water did not result in a reaction.
3.1.2. Chloromethane reactions with Al and Mg mixture
A reaction did occur when Al and Mg were subjected to chloromethane under high temperature and pressure (Table , entry 8). Interestingly, trimethylaluminum was reported to have been produced from an Al-Mg alloy under reaction conditions of 140°C and 10 atm pressure (Iwao, Uchimiya, & Koga, Citation1997). The gas in the Parr bomb resulting from the reaction was analyzed by GC-MS and indicated that methane, ethane, propane and even higher alkane molecules were produced (Figure ). After injecting 10 mL sec-butanol to destroy any potentially explosive species, the Parr bomb was opened. The inside wall of the Parr bomb, the stirring mechanism and the thermal couple were all corroded (Figure ). There was no sign of the Al beads. The black crud sitting on the bottom was collected and reacted with water forming a white suspension which is possibly Al(OH)3. Similar reactions between chloromethane and Al, Mg mixture under mercury bubbler pressure did not result in any organoaluminum products (Table , entry 1–3).
Table 2. Chloromethane reactions with Al-containing materials in flask
3.1.3. Chloromethane reactions with Al and AlCl3 (or MgCl2) mixture
Reactions were conducted to produce trimethylaluminum under the conditions detailed in Table . Al foil was added to some reactions for two reasons: one was to characterize the surface damage using SEM, and secondly, this allows for the determination of consumption of Al foil by weight change. As shown in Table , the reactions detailed in entry 1–3 were not successful. The research was extended by adding AlCl3 and MgCl2 to the Al materials (Table , entry 4–8). Interestingly, AlCl3 triggered the reaction between chloromethane and Al only at 100°C (entry 7–8), and not at room temperature (entry 6). Grosse and Mavity studied similar reactions in the 1940s and found mono- and di-methylalumilun chlorides products when AlCl3 was used. For entries 7–8, a liquid product formed on the bottom of the flask after several hours and the reaction was continued for 1 week (Figure ). A total of 3 mL of sec-butanol was injected and gas collected for GC-MS which was identified as methane (Figure ). The final weight of Al foil was 0.17 g, 0.04 g (19%) less than the weight before reaction. As is evident in Figure , the Al foils were corroded in the reaction (Figure ). There were many cracks on the surface in a SEM image (Figure ). In contrast, MgCl2 did not facilitate a reaction either at room temperature or high temperature (entry 4–5).
Figure 5. Fresh Al foil (left), corroded Al foil (middle) in Al-AlCl3-CH3Cl reaction and foil in Al-AlCl3-CH3Cl-PAG 46 reaction (right).
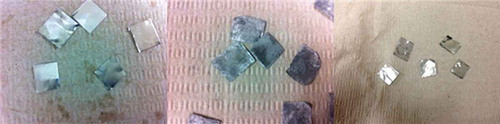
We found that the presence of 2012 Supercool PAG 46 oil reduced the quantity of Me3Al produced. The reduced reactivity is probably caused by PAG oil coating the Al surface reducing contact between the Al materials and chloromethane. This point is supported by the image of the Al foil (Figure ) and the SEM image of the surface of the Al foil (Figure ). The GC-MS of the reaction gas mixture showed that much less methane was produced indicating a reduced reaction in the flask (Figure ).
3.1.4. R-142b reactions with either Al or Al and other metal compounds
There are other Cl-containing refrigerant gases and we decided to examine if they would also react with Al. Mixtures of Al dust and foil and AlCl3 were subjected to R-142b (Table S1, entry 1–2). A further study revealed that Al dust or foil is not required for the reaction (entry 7). The GC-MS results show that 1,1-fluorochloroethene and 1,1-dichloroethene (Fig. S1) were produced, indicating eliminations or more complex reactions. The mechanism of elimination of HCl or HF from R-142b and formation of C=C moieties had been studied by Jones, Holmes, Duke, and Tipton (Citation1990). The powder (excluding the Al foil) was removed and tested by EDS, revealing the presence of fluorine which can only originate from the R-142b gas (Figure S2). This reaction did not occur at room temperature (entry 3). Unlike the reaction detailed above for R-40, R-142b did not react with Al and Mg mixtures under high temperature and pressure conditions (entries 5–6). Similar tests were conducted using R-133a, but no sign of reaction was detected under the conditions detailed in Table S2.
3.2. Does Me3Al react with various commercially available refrigerant oils?
If Me3Al was produced due to the presence of counterfeit refrigerants, that is, chloromethane, it would be useful to know if any of the various refrigerant oils could deactivate it. Seven oils were studied and can be classified into three groups according to IR evidence:
No C=O and O-H bonds: 3GS Suniso oil (Fig. S3) and Behr Hella PAO oil 68 (Fig. S4);
No or weak O-H but C=O bonds: 2012 Supercool PAG 46 (Fig. S5), PAG 100 (Fig. S6) and Four seasons ester 100 (Fig. S7);
No C=O but O-H bonds: 2014 Supercool PAG 46 (Fig. S8) and Napa PAG 46 (Fig. S9).
Interestingly, similarly labeled oil from the same commercial source had different properties. We found that Supercool PAG 46 and PAG 100 purchased in 2012 differed from the new Supercool PAG 46 purchased in 2014 based on IR and mass spectrum (Figures S5, S6, S8, S12, S13 and S15). Additionally, as the Me3Al concentration varies due to decomposition, gas release calibration measurements were conducted on a weekly basis by directly reacting 1 mL Me3Al with 4 mL of sec-butanol. Each measurement was repeated twice to estimate precision. All the gas volumes have been converted to standard values (25°C, 101.325 kPa). Table shows the gas release (i.e. methane) volumes from refrigerant oil-Me3Al-sec-butanol reactions either at room temperature or at 80°C.
Table 3. Gas measurements for refrigerant oil-Me3Al-sec-butanol reactions
Table 4. Gas measurements for deactivating reagent-Me3Al-sec-butanol reactions
From the data above, one can conclude that 3GS Suniso oil (petroleum distillates) and Behr Hella PAO (Polyalphaolefin) oil 68 did not react with Me3Al at room temperature. The IR spectra of 3GS Suniso oil and Behr Hella PAO oil 68 attained initially at room temperature and 80°C after Me3Al and sec-butanol injection are displayed in supplemental materials (Figs. S3–S4). The main peaks in the initial spectra appear unchanged. The corresponding MALDI-TOF mass spectra of these oils under the reaction condition are displayed in Figs. S10 and S11. They both contain a separation of 14 g mol−1 between major peaks corresponding to a –CH2– repeating unit. The 3GS Suniso oil has molecular weights ranging from 250 to 500 g mol−1 which is similar to petroleum crude oil with molecular weights of 250–700 g mol−1 (Rodgers & Marshall, Citation2007). A cleaner mass spectrum was obtained for Behr Hella PAO oil 68 showing molecular weights ranging from 250 to 450 g mol−1. This pattern is similar to the PAO oil with molecular weights of 200–450 g mol−1 (Hourani, Citation2012). With both oils, sec-butanol reacted with Me3Al to release methane. The small quantities of methane released initially from adding Me3Al to 3GS Suniso oil or Behr Hella PAO oil 68 of 4.4 and 9.9 mL, respectively, suggests that adventitious water was responsible for the methane produced. The fact that both oils released gas close to the theoretical values, that is, 129.1 mL for 3GS Suniso oil (cal. 128.0 mL) and 135.1 mL for Behr Hella PAO oil 68 (cal. 132.8 mL) implies that these oils do not render trimethylaluminum inactive. The initial appearance of Behr Hella PAO oil 68 is yellow due to an additive dye. It reacts with Me3Al to form a red solution which returns back to yellow after sec-butanol injection (Figs. S27–S28). This probably can be used to indicate the presence of Me3Al in an AC system.
The ester groups in Four seasons ester 100 display a very strong C=O absorption in IR spectrum, whereas a weaker C=O band was observed for 2012 Supercool PAG 46 and PAG 100 (Figs. S5–S7). 2012 Supercool PAG 46 and PAG 100 oils (Polyalkylene glycol with partial end-capping) react with Me3Al to release one and two equivalents of methane, respectively, see Table . Following that reaction, the Me3Al was completely deactivated with sec-butanol. The total volume of gas collected after sec-butanol injection for each test was close to calibrated value. All IR spectra indicate a reduction of the stretching frequencies for the C=O bond (1731, 1737 and 1738 cm−1 for 2012 Supercool PAG 46, PAG 100 and Four seasons ester 100, respectively) after reaction with Me3Al. However, it is not obvious whether the reduction is caused by a reaction or a simple dilution of the ester groups in the mixtures. As is evident in Figs. S5–S7, the IR spectra match the original after the mixture was purified by filtration to remove solids and then all filtered volatile solvents (i.e. heptane and sec-butanol) removed under vacuum. The MALDI-TOF mass spectra obtained for 2012 Supercool PAG 100 indicates a reaction occurred as evident in the appearance of a new series of peaks roughly +32 g mol−1 right shift from the unreacted oil pattern, see pattern 3 in Fig. S11. This reaction is favored by high temperature (80°C). No such evidence was observed for 2012 Supercool PAG 46. Since the total volume of methane released from reacting Me3Al with 2012 Supercool PAG 100 is similar at room temperature (cal. 132.8 mL, exp. 131.9 mL) and at 80°C (cal. 143.8 mL, exp. 149.2 mL), the reaction does not result in additional Me3Al deactivation. A separation of 58 g mol−1 between major peaks is found for 2012 Supercool PAG 46, PAG 100 and Four seasons ester 100 oils. The PAG oils are assumed to have a repeating unit of –O-CH(CH3)-CH2– or similar (William, Citation1993), while ester oils repeat by –CH2-COO– groups (Bobbo, Fedele, & Stryjek, Citation2007).
Four seasons ester 100 oil does not react with Me3Al at room temperature. A total gas release of 130.0 mL upon addition of sec-butanol matches the calibrated value (132.8 mL). However, at 80°C, there was a significant decrease in the total quantity of methane released (cal. 143.8, exp. 109.2 mL) which indicates a reaction must have occurred between Me3Al and the oil. The -CH3 groups from Me3Al can react with the carbon atoms in ester groups, thus decreasing overall methane release. The evidence for this reaction is observed in the mass spectra of the reaction at 80°C by the appearance of many new peaks (Fig. S14). The IR spectra of the reaction are not very different (Fig. S7).
The gas measurement data for 2014 Supercool PAG 46 and Napa PAG 46 oil (Polyalkylene glycol with no end-capping) suggest that Me3Al almost completely reacted with those oils, presumably with their O-H groups at room temperature or 80°C. Those –OH groups for both oils were evident in their IR spectra (Figs. S8–S9). The total gas released for those oils was close to the calibrated values, which means these oils are not useful in preventing methane from being generated. Similar to 2012 Supercool PAG oils, 2014 Supercool PAG 46 and Napa PAG 46 contain a separation of 58 g mol−1 corresponding to a –O-CH(CH3)-CH2– group in the signal for the molecular ions in the mass spectra (Figs. S15–S16). The mass spectra for both oils before and after reaction at room temperature and 80°C are invariant.
3.3. How to deactivate trimethylaluminum safely?
The chemistry of reactions between trimethylaluminum and reagents containing the C=O moiety, including aldehydes (Ichiyanagi, Kuniyama, Shimizu, & Fujisawa, Citation1998; Raluy, Diéguez, & Pàmies, Citation2009), ketones (Kabbara, Flemming, Nickisch, Neh, & Westermann, Citation1995; Van Vliet, Van Koten, De Keijser, & Vrieze, Citation1987) and esters (Bretches, Sivak, & Stainfield, Citation1988) have been previously studied. However, to our knowledge, the deactivation of trimethylaluminum without methane being released has not been addressed. Various chemicals, including benzaldehyde, phthalaldehyde, benzophenone, cyclohexanone and ethyl acetate have been examined using procedures similar to those for the aforementioned oils. The gas release data are listed in Table 4.
The data suggest that benzaldehyde reacts with Me3Al solution (cal. 132.8 mL, exp. 90.2 mL) and reduces the volume of gas released by 32.1% which suggests that one of the three methyl groups on Me3Al has reacted and has been deactivated (Allan et al., Citation2000). The volume of methane increased slightly in the reaction conducted at a temperature of 80°C (cal. 143.8 mL, exp. 112.3 mL). Benzyl alcohol, acetophenone and benzyl benzoate are found in the GC spectrum of the product mixture collected after the reaction (Fig. S18). The formation of acetophenone is expected as Me3Al is known to methylate benzaldehyde. The results indicate that the phthalaldehyde (cal. 132.8 mL, exp. 71.7 mL) is somewhat more efficient than benzaldehyde in deactivating trimethylaluminum. It is possible that the phthalaldehyde molecule may chelate Al atom and facilitate the –CH3 groups transfer. The GC spectrum indicates the formation of 2-acetylbenzaldehyde which resulted from the methylation of phthalaldehyde (Fig. S20). Benzophenone (cal. 132.8 mL, exp. 92.8 mL) was tested and found to have similar reactivity to benzaldehyde in reducing methane release. The GC spectrum shows the major products are diphenylmethane and 1,1-diphenylethylene (Fig. S22). This suggests that methylation and reduction reactions occurred on the benzophenone molecules which can react with one –CH3 group from Me3Al and reduce overall methane production (Ashby, Laemmle, & Neauman, Citation1968; Harney, Meisters, & Mole, Citation1974). Cyclohexanone (cal. 132.8 mL, exp. 108.6 mL) however is not a satisfactory deactivating agent in reaction with Me3Al due to relatively high gas production and solid formation. Cyclohexanol, 2-cyclohexylidene cyclohexanone and 2-(1-cyclohexenyl)cyclohexanone are detected by the GC-MS (Fig. S24). The Me3Al may have catalyzed reactions between cyclohexanone molecules, which would be responsible for solid formation.
Ethyl acetate reacts slightly with Me3Al at room temperature with only a 2.7% reduction in methane release (cal. 132.8 mL, exp. 129.2 mL). This reaction occurs readily at high temperature (80°C). These results are similar to those obtained for Four seasons ester 100 oil and reveal that ester groups react with Me3Al at elevated temperature. In the GC spectrum, besides the solvents (heptane and sec-butanol), ethanol, 2-methyl propanol and butyl acetate are found as products (Fig. S26). Ethanol arises from the hydrolysis of ethyl acetate while butyl acetate is a product of the esterification of acetic acid and sec-butanol. 2-methyl propanol was presumably formed by transferring two methyl groups from Me3Al to a carbon in the ester group (Bretches et al., Citation1988). This reaction can partially deactivate trimethylaluminum and reduce methane production.
4. Conclusions
The substitution of chloromethane (R-40) for other approved refrigerants is very dangerous as it sometimes results in the formation of trimethylaluminum or similar species which explode under certain conditions. We found that getting chloromethane and Al to react to yield Me3Al (or similar species) required specific conditions, that is, the presence of Mg under high chloromethane pressure and high temperature, conditions which could exist inside an air conditioning system as Mg is a known ingredient in some alloys. Finely divided metal particles can result from wear and tear of parts inside of air conditioning compressors. We found that some refrigerant oils react with Me3Al and one oil, that is, polyester oil, stabilized the methyl groups on Me3Al. This resulted in a decrease in methane production upon deactivation with alcohol. Chemicals with C=O groups can partially deactivate Me3Al by tying up methyl groups and binding to the Al atom. Among various aldehydes, ketones and esters studied, phthalaldehyde was the most effective and resulted in a decrease by approximately 50% of methane eventually produced. This suggests that adding phthalaldehyde to refrigerant oils may be one way to deactivate Me3Al. However we have not examined other consequences (e.g. viscosity) of such an addition.
Competing interests
There are no conflicts to declare.
Supplemental Material
Download PDF (14 MB)Acknowledgements
We thank Michigan Technological University and Alion Science and Technology Corporation (Proposal 1207064) for support of this research.
Supplementary material
Supplemental material for this article can be accessed here http://dx.doi.org/10.1080/23312009.2018.1487258.
Additional information
Funding
Notes on contributors
Zhichao Chen
Dr Rudy Luck is an associate professor in the field of inorganic chemistry at Michigan Technological University. Dr Zhichao Chen was a PhD student when performing this work. We have extensive research experience on synthesis and characterization of organometallic compounds. We also study catalytic applications of these compounds in the area of epoxidation of olefins. The reported work dealing with organoaluminum compounds is within our research scope. We hope this work can initiate some interest on reactive and explosive organometallic compounds such as trimethylaluminum, and solutions to safely and effectively deactivate them.
References
- Allan, J. F., Clegg, W., Elsegood, M. R. J., Henderson, K. W., McKeown, A. E., Moran, P. H., & Rakov, I. M. (2000). Journal of Organometallic Chemistry, 602(1–2), 15–23. doi:10.1016/S0022-328X(00)00111-X
- Ashby, E. C., Laemmle, J., & Neauman, H. M. (1968). Journal of the American Chemical Society, 90(19), 5179–5188. doi:10.1021/ja01021a021
- Bobbo, S., Fedele, L., Pernechele, F., & Stryjek, R. (2007). Correlation of solubility data of carbon dioxide in POE lubricants, pp b11404/11401-b11404/11408, International Institute of Refrigeration.
- Bretches, R. K., Sivak, A. J., & Stainfield, R. L. (1988). Deactivation of aluminum alkyls. WO8803539A1, Aristech Chemical Corp., USA. CAPLUS AN 1988: 611625 (Patent).
- Harney, D., Meisters, A., & Mole, T. (1974). Australian Journal of Chemistry, 27(8), 1639–1653. doi:10.1071/CH9741639
- Hourani, N. (2012). Development of a novel mass spectrometric methodology for the analysis of hydrocarbon content in the light shredder waste ( Ph. D. thesis). Jacobs University Bremen, Bremen.
- Ichiyanagi, T., Kuniyama, S., Shimizu, M., & Fujisawa, T. (1998). Chemistry Letters, 27(10), 1033–1034. doi:10.1246/cl.1998.1033
- Iwao, T., Uchimiya, R., & Koga, S. (1997). Preparation of trialkylaluminum from alkyl halide and amorphous aluminum-magnesium alloy. JP09136890A. Mitsui Toatsu Chemicals, Inc., Japan; Mitsui Chemicals Inc., CAPLUS AN 1997: 469717(Patent).
- Jones, Y., Holmes, B. E., Duke, D. W., & Tipton, D. L. (1990). The Journal of Physical Chemistry, 94(12), 4957–4963. doi:10.1021/j100375a037
- Kabbara, J., Flemming, S., Nickisch, K., Neh, H., & Westermann, J. (1995). Tetrahedron, 51(3), 743–754. doi:10.1016/0040-4020(94)00975-Z
- Lindborg, A. (2003). DKV-Tagungsber, 30, 301–308.
- Loizaga, C. A., & De Loizaga, J. R. (1954). El Dia Medico, 26(46), 1230–1231.
- Mendelhoff, J. (1952). Archives of Industrial Hygiene and Occupational Medicine, 6, 518–524.
- Ministry of Business, I. a. E. (2012). Counterfeit refrigerants in shipping container refrigeration units - Hazard alert. Wellington: Worksafe New Zealand.
- Nuckolls, A. H. (1933). Miscellaneous Hazards, 2375, 118.
- Peter, P. (2012, March 5). Air Conditioning, Heating and Refrigeration News, p. 30.
- Raluy, E., Diéguez, M., & Pàmies, O. (2009). Tetrahedron: Asymmetry, 20(13), 1575–1579. doi:10.1016/j.tetasy.2009.06.014
- Rodgers, R., & Marshall, A. (2007). Petroleomics: Advanced characterization of petroleum-derived materials by Fourier Transform Ion Cyclotron Resonance Mass Spectrometry (FT-ICR MS). In O. Mullins, E. Sheu, A. Hammami, & A. Marshall (Eds.), Asphaltenes, heavy oils, and petroleomics (pp. 63–93). New York, NY: Springer.
- Sassano, B. (2012). DuPont refrigerants it’s a chilling reality counterfeit refrigerants are on the rise…. Nuremberg: DuPont Fluorochemicals.
- Van Vliet, M. R. P., Van Koten, G., De Keijser, M. S., & Vrieze, K. (1987). Organometallics, 6(8), 1652–1664. doi:10.1021/om00151a009
- Vilches, A. M. (1948). El Dia Medico, 20(71), 2921–2927.
- William, L. B. (1993). Polyalkylene glycols. In E. Richard Booser (Ed.), CRC handbook of lubrication and tribology, volume III: Monitoring, materials, synthetic lubricants, and applications, volume III (pp. 253–267). Boca Raton, FL: CRC Press.