Abstract
There are a lot of publications concerning with biosynthesis of well-separated silver nanoparticles (AgNPs) from the aqueous plant extracted lower molecular weight polyol. In this first investigation, Ph.L leaf and seed extract broths were used for “green” synthesis of assembled and well-separated AgNPs, comparatively. AgNP formation from Ag (I) ions was determined by both extract color changes, UV-Vis absorption spectra, and surface plasmon resonance (SPR). The size, size distribution, and elemental and crystalline state of the formed AgNPs were determined by electron microscopy and spectrometry. The obtained results revealed the formation of assembled poly-dispersive spherical AgNPs of size measured 52.6 nm (leaf broth) and well-separated 24.2 nm (seed broth) on average. AgNPs assembled inside diffusible nano-capsules formed from the extracted macromolecules in case of leaf extract broth. Both extracts exhibited good AgNP-protecting active ingredients according to their binding affinity to L-lysine functional groups. Further, these diffusible AgNPs exerted good and comparable antibacterial activity against Staphylococcus aureus and Escherichia coli pathogens. Our results draw attention about assembling or growth of NPs by aqueous plant extracted polyol macromolecules as simple experimental protocol.
PUBLIC INTEREST STATEMENT
For the first time, the silver nanoparticle was prepared inside capsules of polyol macromolecules of Peganum harmala leaf broth extract. The previous studies dealt with the discrete silver nanoparticles which have been prepared from lower molecular weight polyol molecules. Comparatively, discrete silver nanoparticles showed better antibacterial activity than capsulated silver nanoparticles due to its higher diffusion under normal conditions. Expectedly, capsulated silver nanoparticles will show enhanced antibacterial activity under severe conditions.
Competing Interests
The authors declare no competing interests.
1. Introduction
The anti-microbial activity of plants and their extracts are well described in traditional medicine (Al-Said, Citation1993; Bibi & Saudi, Citation2017; Moloudizargari, Mikaili, Aghajanshakeri, Mohammad, & Jalal, Citation2013; Saabi, Citation2006), and a significant proportion of the population is now reliant on folk medicine for their healthcare (Akerele, Citation1993). Peganum harmala L (Ph.L; family Nitrariaceae) is commonly used in folk medicine in Saudi Arabia (Al-Said, Citation1993; Saabi, Citation2006) and beyond (Abbott, Bettmann, & Sterling, Citation2008; Prashanth & John, Citation1999). Different parts of Ph.L, particularly the seeds, leaves, roots, and bark, powerfully inhibit different human pathogens (Bremner et al., Citation2009; Kotb, Citation1983; Moloudizargari et al., Citation2013), so its active ingredients have been intensively studied from a variety of perspectives including: (i) isolation and structural elucidation (Abdel Aziz, Abdel Kader, El-Sayed, EL-Malt, & Shaker, Citation2010; Khuroo, Citation2013); (ii) therapeutic efficacy (Khuroo, Citation2013; Moloudizargari et al., Citation2013); (iii) allelopathic properties (Mohamed, AL-Jammali, & Aki, Citation2013); and (iv) isolation of microbes from various plants parts and their action on human pathogens (Aniszewski, Citation2007).
Pharmaceutical studies on plant-derived alkaloids are growing rapidly due to their potential medical uses. Saudi Arabia’s mild climate is suited to the growth of plants containing alkaloids. Ph.L is rich in alkaloids and also flavonoids, anthraquinones, and polysaccharides. The most widely recognized alkaloids are β-carboline (e.g., quinazoline, harmaline, and harmine), which were initially isolated from the dried seeds and roots of Ph.L (Abdel Aziz et al., Citation2010; Khuroo, Citation2013; Moloudizargari et al., Citation2013). Most previous research has focused on investigating Ph.L seeds due to their high alkaloid concentrations (4.3–5.6%) and, consequently, antibacterial activity (Khuroo, Citation2013; Mohamed et al., Citation2013; Moloudizargari et al., Citation2013). The different pharmacological impacts of Ph.L parts have been reviewed in detail (Moloudizargari et al., Citation2013). Aqueous, methanolic, and chloroform Ph.L extracts display antimicrobial properties (Moloudizargari et al., Citation2013). Ph.L has also been used against parasitic diseases in Saudi Arabia (Al-Said, Citation1993), and alkaloids extracted from plants form part of the pharmaceutical industry due to their antimicrobial and antitumor activities (Aniszewski, Citation2007).
Nano-sized assemblies of noble metals have unique characteristics and applications and have therefore attracted recent attention (El-Nour, Eftaiha, Al-Warthan, & Ammar, Citation2010; Mittal, Chisti, & Banerjee, Citation2013; Nanda & Saravanan, Citation2009). Nanoparticle (NP) synthesis using a plant extract brothrepresents a simple, economical, and biologically and ecologically friendly synthesis. However, the variable morphology of the produced NPs means that further research is warranted (El-Nour et al., Citation2010; Nanda & Saravanan, Citation2009), and there are relatively few studies on traditional medicine plants as NP broths (Basu, Maji, & Ganguly, Citation2015; Das & Velusamy, Citation2013; Ghaedi, Yousefinejad, Safarpoor, ZareKhafri, & Purkait, Citation2015; Kumar, Yada, Yada, & Chem, Citation2010). Further, plant-derived ingredient-protected NPs can be considered an indirect and “green” protocol to increase their biological activity, with polyols regarded as advanced NP-protecting agents, since distinct polyols are the main phytochemicals responsible for the capping, reduction, and protection of metal NPs (El-Nour et al., Citation2010; Nanda & Saravanan, Citation2009). Nevertheless, the exact biological reduction mechanism is difficult to determine due to the complex composition and diversity of the extracted phytochemicals (El-Nour et al., Citation2010; Nanda & Saravanan, Citation2009). A limited number of studies have compared NPs synthesized from aqueous extracts of both seeds and leaves (Basu et al., Citation2015; Kora & Arunachalam, Citation2013).
In addition to their unique therapeutic and optical characteristics, silver and gold noble metals are relatively non-toxic to humans (Basu et al., Citation2015). The biosynthesis of silver nanoparticles (AgNPs) from Ph.L leaf extracts has yet to be studied, and there is only a single study on the aqueous biosynthesis of AgNPs from seed extracts (Azizia, Sedaghat, Tahvildari, Derakhshi, & Ghaemi, Citation2017) and one from a methanolic extract (Amin et al., Citation2014). Here we examine and compare the biosynthesis of AgNPs from Ph.L leaf and seed extract broths. The study also aims to confirm and characterize the synthesized AgNPs from both extracts using different spectral and optical techniques. Finally, we investigate the inhibitory activity of the synthesized AgNPs against the human bacterial pathogens Staphylococcus aureus and Escherichia coli.
2. Materials and methods
2.1. “Green” synthesis of agnps
Silver nitrate (AgNO3, 99.9% pure) and L-lysine were obtained from Sigma-Aldrich (St Louis, MO). Dry Ph.L seeds and leaves were purchased from a local herbal market in Al-Mukawh, Kingdom of Saudi Arabia.5 g of dry Ph.L leaves, thoroughly washed and finely cut, were incubated in 250 ml of water in a 250 ml elementary flask at 70–80°C for 2 h. After the solution turned a pale green, it was decanted and filtered. Immediately, 20 mg AgNO3 was added to 100 ml of Ph.L Filtrate (final concentration being 1.18 mM AgNO3) with vigorous stirring; at room temperature a color change was initially observed after 3 min, after which no further color change was detected. The reduction process was accurately tracked by UV-Vis SPR measurements. Furthermore, the change in the rate of reduction with the temperature (30–90°C) was studied. The same procedure was used with the seeds, with the color of the seed extract changing from pale yellow to light brown after 5 min at 95°C and turning dark brown after 30 min. At room temperature, no color change was observed even after 24 hrs. AgNP colloids were centrifuged at 6000 rpm and the NP deposits were collected, washed with distilled water, and dried for further analysis. For conjugation study, 1 ml of the prepared AgNPs colloid was taken and added to 90 mg of L-lysine in10 ml of distilled water. The capped AgNPs in this mixture were measured by shift UV-Visible and redistribution SEM measurements.
2.2. Characterization of synthesized AgNPs
A UV-VisShimadzu double beam spectrometer (Shimadzu Corp., Kyoto, Japan) was used to record absorption spectra of crude leaf and seed extracts before and after AgNP synthesis in the range 300–800 nm. Reduction at different temperatures (30–90°C) and incubation periods (till 24 hrs) was also examined. Aliquots were withdrawn in quartz curvatures every UV-Visible measurement period to follow the reduction process. Morphological characteristics of the synthesized AgNPs were studied using a transmission electron microscope (TEM; JEOL-1200 EX II, Jeol Ltd., Tokyo, Japan). TEM grids were prepared as previously described by placing a drop of the particle solution on a carbon grids and drying at room temperature. Fourier transform infrared spectroscopy (FTIS) spectra of Ph.L leaf and seed extracts, AgNP colloids, and deposits were recorded in the range 400–4000 cm−1. The crystalline and elemental state of AgNPswere investigated by X-ray diffraction (XRD) patternsusing the Ultima IV X-ray diffractometer (Rigaku, Tokyo, Japan) at 2 theta range 2–60°and energy-dispersive X-ray spectroscopy (EDS; X-Max, Oxford Instruments, Abingdon, UK). Seed and leaf-AgNP images were recorded using a field emission scanning electron microscope (FESEM; JEOL JDM-7600F).
2.3. Antimicrobial activity screening
Bacterial cultures and agar were purchased from the Microbiology Lab, Faculty of Science, Ain Shams University. The antimicrobial activities of the synthesized AgNPs were evaluated against Staphylococcus aureus (Gram positive) and Escherichia coli (Gram negative) by the disc diffusion method as described elsewhere (Magaldi et al., Citation2004).
3. Results and discussions
3.1. Biophysical properties
Our experiments were based on the well-established protocol of preparing plant extracts using hot (but not boiling) aqueous broths of dry plant parts. This protocol has many advantages (Kumar, Yada, & Yada, Citation2010): (i) fundamental active ingredients may be affected by boiling; (ii) many undesirable non-polar macromolecules (lipids, fats, chlorophyll, etc.) can be extracted by boiling, which interferes with the principle bio-reducing agents; and (iii) phytochemicals are dispensed homogenously in dry plant tissues. These represent the main factors contributing to NP yield.
The concentration, structure, and types of biomolecules differ in different plant parts. Consequently, AgNPs can be expected to possess different morphological properties and colors in different plant parts. The observation of a color change on addition of silver nitrate to Ph.L extracts was the primary evidence of AgNP formation. AgNPs synthesized from seed extracts had a normal brown color, whereas NPs synthesized from leaf extracts had a white color (see Supplementary Information); different NP colors indicate various NP shapes, sizes, distributions, and media (Ghosh & Pal, Citation2007; Kanchana, Devarajan, & Ayyappan, Citation2010; Kelly, Coronado, Zhao, & Schatz, Citation2003; Mody, Siwale, Singh, & Mody, Citation2010). The difference in NP characteristics can be related to the structures of the extracted phytochemicals. For instance, for both seed and leaf extracts, harmaline and harmine (Moloudizargari et al., Citation2013), and l-thioformyl-8-β-D-glucopyranoside-bis-2,3-dihydroisopyridinopyrrol (TGDPP), in addition to other similarly structured polyols, were previously confirmed as fundamental β-carboline alkaloid derivatives (Figure ), respectively (Abdel Aziz et al., Citation2010; Asgarpanah & Ramezanloo, Citation2012; Khuroo, Citation2013; Moloudizargari et al., Citation2013).
Figure 1. Fundamental active ingredients of Ph.L seeds and leaves (Abdel Aziz et al., Citation2010; Moloudizargari et al., Citation2013). General mechanism of bioreduction of Ag (I) ions to AgNPs by polyol biomolecules of plant extract (Das & Velusamy, Citation2013; Ghaedi et al., Citation2015).
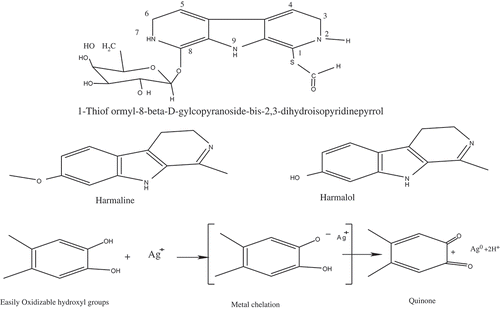
Figure compares UV-Vis spectra of leaf and seed synthesized AgNP colloids and crude extracts. A single broad continuous absorption band was observed for AgNPs synthesized from both extracts, corresponding to spherical AgNPs of wide size range (Kumar et al., Citation2010,26). Seed-AgNPs were characterized by a more intense absorption band than leaf-AgNPs, peaked at 450 nm (Figure –)). The lower absorption intensity of leaf-AgNPs indicates shielding of conducting electrons at the NP surface, preventing their local excitation by interacting electromagnetic radiation (Figure )). Generally, the different colors of the same metal NPs from the same plant extracts (e.g., AgNPs red-brown (Azizia et al., Citation2017), brown and red (Sathishkumar et al., Citation2012)) and vice versa predict quantized electron energy levels. The energetic separation between these levels depends on the NP assembly sizes, with energy decreasing as NPs increase in size (Atkins & de Paula, Citation0000).
Figure 2. UV-Vis spectra: (a) seed-AgNPs; (b) leaf- AgNPs and (c) comparison between leaf- and seed-AgNPs.
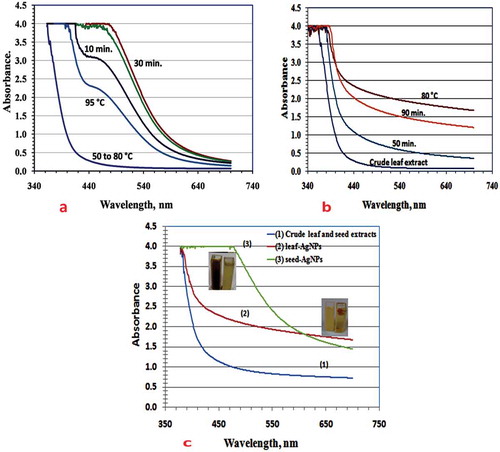
Firstly, at room temperature, seed-AgNPs did not form even after 24 hrs with vigorous stirring. Leaf-AgNPs formed after 3 min. and the most intense SPR was observed after 90 min. but it is not the optimal value. Figure ,) illustrates the reliance of Ag(I) ion reduction on temperature and incubation period (Rani & Harreddy, Citation2011; Udayasoorian, Vinothkumar, & Jayabalakrishnan, Citation2011). The start of reduction and the corresponding color change of Ag(I) ions in seed extract broth were observed at 95°C. The rapidly expanding UV-Vis absorption intensities at ~ 450 nm after 20 min provided an indication of the optimal reduction rate and SPR AgNP growth. For leaf-AgNPs, the optimal UV-Vis SPR intensity was observed at 80°C (Figure )).
Figure illustrates TEM images of seed and leaf AgNPs. Brownish-colored seed-AgNPs had different size distribution profiles to the white-colored leaf AgNPs. For leaf AgNPs, TEM measurement revealed poly-dispersive and spherical AgNPs in a colorless matrix; TEM images illustrating black and assembled AgNPs inside a linked diffusible nanoscopic template are shown (Figure ,) and Supplementary Material). Particles sizes ranged from 28.9 to 68.6 nm with an average size of 52.6 nm. For seed extracts, TEM showed poly-dispersive, spherical, and well-separated AgNPs in colloidal solution (Figure ) and Supplementary Material), with particle sizes of 11.0–30.9 nm and average size 24 nm.
Figure 3. Comparative TEM study includes images, histogram and particle diameter distribution for: (a, b) leaf-AgNPs; (c) seed-AgNPs; (d) histogram of leaf-AgNPs, mean particle diameter = 52.6 nm, standard deviation = 14.51 & standard error = 5.48 and (e) histogram of seed-AgNPs, mean particle diameter = 24.2 nm, standard deviation = 6.97 and standard error = 2.2.
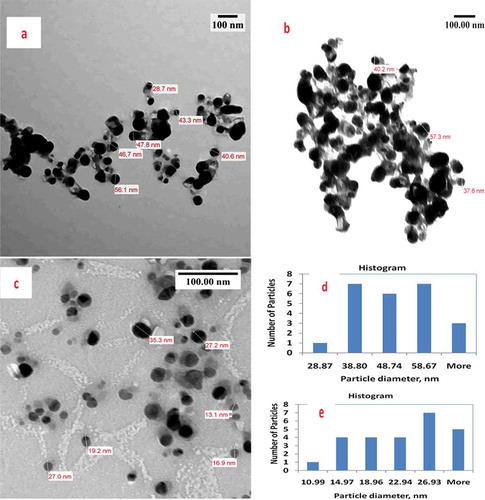
Figure illustrates EDX spectra of both seed- and leaf-derived AgNPs. The similar signal in the silver region for both extracts indicated the elemental and crystalline nature of the formed AgNPs. Carbon and oxygen peaks were ascribed to the biomolecule-protected NPs. Cl peaks were due to chloride ions present in plant extract broths. This was confirmed by XRD for both leaf and seed AgNPs (Figure ). Distinctive intense peaks at different 2θ confirmed the crystalline nature of the formed AgNPs, as reported previously (Azizia et al., Citation2017). XRD patterns confirmed the formation of face centered cubic (fcc) structure of metallic silver according to (1 1 1), (2 0 0), (2 2 0), and (3 1 1) planes (JCPDS file No. 01–071-4613). According to Scherrer Equation (Cullity, Citation1978), average size of protected silver crystals equals 11.18 nm and 9.89 nm for seed and leaf-AgNPs, respectively. The comparison between XRD patterns of seed and leaf AgNPs indicated exposed and partially hidden AgNPs, respectively. This partially hidden property indicated the hosting of some AgNPs inside cavities containing matrix, where sliver nano crystals oriented predominately in (111) plane according to the intensity of (111) plane peak. FE-SEM images confirmed the XRD results as shown in Figure ,) (Supplementary Material). AgNPs of 12.7–35.6 nm with red-brown color have previously been synthesized from Ph.L seed extracts (Azizia et al., Citation2017). In our case, roughly 4% of the particles were < 12.73 nm, with a different size distribution profile. Commonly, the plant extracted was brown colored after the formation of AgNPs and SPR peaked at 450 nm (El-Nour et al., Citation2010; Mittal et al., Citation2013). The scattering of the brown or red brown color constituents preferentially indicated the ability of NP colloid to scatter the corresponding wavelengths of visible light according to the AgNP sizes (Mittal et al., Citation2013, p, 26), whereas white light scattering indicated capsule-associated macromolecule formation of size comparable to the wavelength of the incident light (Atkins & de Paula). These capsules appeared to host and shield AgNPs (Raveendran, Fu, & Wallen, Citation2003).
Figure 4. Comparative EDX patterns and FESEM images of seed-AgNPs (a, b) and leaf-AgNPs (c, d) showing shapes, exposing and hidden of AgNPs.
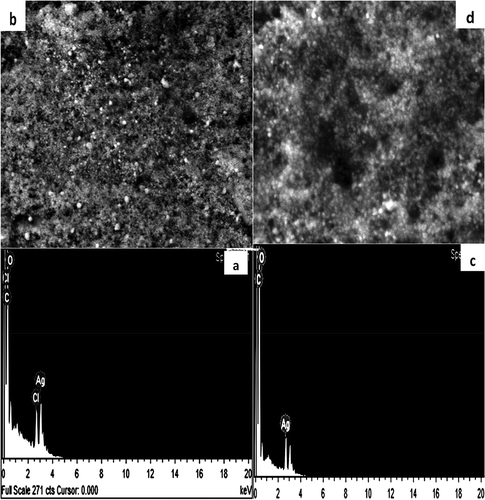
These results are consistent with the literature: (i) natural polyol polymers or macromolecules (chitosan, glucose, and starch) have been used as host polymers for NP synthesis (Aziz, Saber, Rasheed, & Ahmed, Citation2017; Raveendran et al., Citation2003); (ii) such macromolecules associate in capsule templates due to inter- and intra-hydrogen bonding (Raveendran et al., Citation2003); (iii) a limited number of AgNP white spots and chains were observed in the case of chitosan:Ag(I) extraction broth (Aziz et al., Citation2017); (vi) both large and compact molecules hosting NPs sediment easily and quicker by centrifugation than the other molecules. In our case, leaf-AgNPs sediment faster than seed-AgNPs and other reported AgNPs, after 2–5 min by 6000 rpm centrifugation (Asgarpanah & Ramezanloo, Citation2012; Kanchana et al., Citation2010; Sathishkumar et al., Citation2012).
TGDPP is a polyol macromolecule consisting of D-glucopyranoside as repeating units. Accordingly, TGDPP and other similar macromolecules act as reducing host polyol capsules for AgNPs, whereas the excessive number of hydroxyl groups facilitates Ag(I) ion reduction, formation, and protection (Rani & Harreddy, Citation2011). AgNPs were initially formed after 3 min in Ph.L leaf broth as dispersed white-colored particles. Generally, hydroxyl-based protecting agents are superior to others (e.g., thiols) due to reduced binding interactions and hence NP diffusivity.
Hosted, assembled, and loss-protected AgNPs are favored in antibacterial applications due to the high diffusivity of AgNPs towards the polar groups of bacterial cell membranes. The functionalization of AgNPs from Ph.L ingredient protection was tested using L-lysine amino acids (see Supplementary Material). The shift in UV-Vis absorption of L-lysine indicates protected AgNPs binding easily to L-lysine functional groups at room temperature. Comparatively, seed ingredient-protected AgNPsbind well less than leaf ingredient-protected AgNPs according to the relative shift in UV-Vis band absorptions. Again, this confirmed the effective hosting of leaf-AgNPsthan seed-NPs.
The functional groups of both seed and leaf crude extracts were identified by FT-IR spectra (Figure ). As expected, both extracts had similar IR spectra, because their active ingredients have the same functional groups. Generally, IR spectra were characterized by three well-defined regions. The broad band at 3307 cm−1 or 3336 cm−1 was ascribed to inter-hydrogen bonding OH group stretching (alcohols, phenols). A band at ca. 2100 cm−1 of lower intensity was ascribed to carbonyl stretch. A band at 1634 cm−1 was ascribed to aromatic C = C and free N-H stretching vibrations. A band at 403–439 cm−1 was ascribed to a poly-aromatic system. Figure illustrates IR spectra from both leaf and seed AgNP deposits. Protected biomolecules still exist, and both AgNP deposits have a similar functional group distribution due to the similar functional groups of the extracted biomolecules. Leaf AgNP deposits were characterized by more intense peaks corresponding to their extracted macromolecules. The broad band at 403–439 cm−1 represented the main difference between our investigated spectra and published spectra (Azizia et al., Citation2017).
3.2. Antibacterial activity
The antibacterial activity of AgNPs is commonly identified by the disc diffusion method (Magaldi et al., Citation2004). Generally, AgNPs show different antibacterial activities against human pathogens according to a plant type (El-Nour et al., Citation2010; Mittal et al., Citation2013). The activity of our synthesized AgNPs against Staphylococcus aureus and Escherichia coli was investigated and compared to the standard antibiotics cefoxitin and chloramphenicol. Table illustrates the inhibition by both seed and leaf-AgNPs of Staphylococcus aureus and Escherichia coli (Figure ). Our results can be summarized as follows: (i) a high level of inhibition of Staphylococcus aureus bacteria was observed according to measured zone inhibition diameters 24 and 20 mm for both seed and leaf-AgNPs, respectively (Table ); (ii) a moderate level of inhibition against of Escherichia coli bacteria was observed according to a measured zone inhibition diameters 25 and 20 mm, compared to zone inhibition diameter of + ve control 40 mm; and (iii) AgNP colloids show greater inhibition than isolated AgNPs and crude plant extracts as confirmed previously. The exact antibacterial inhibition mechanism is still unknown, but may be due to interactions between AgNPs and bacterial cytoplasm, proteins, and DNA (Asgarpanah & Ramezanloo, Citation2012). The previous studies found that AgNPs inhibited growth of Gramnegative more than Gram positivebacteria, which was rendered to difficult penetration of AgNPs for a wall thick layer of peptidoglycan of Gram positive bacteria (Das & Velusamy, Citation2013). Accordingly, inhibition of Staphylococcus aureus bacteria was a different and specific characteristic of AgNPs synthesized from both Ph.L seed and leaf extracts. It is clear that the thickness and rigidity of bacterial membranes (Das & Velusamy, Citation2013; Narang & Manu, Citation2017) are not only AgNP inhibition factors but inhibit the diffusion of the protected AgNPs. Furthermore, bacterial growth inhibition mechanisms were rendered to penetration & interaction and/or membrane functionalization by AgNPs (McDonnell & Russell, Citation1999).
Table 1. Antibacterial activity of AgNPs against S. aureus and E. coli bacteria
Figure 8. Comparison between antibacterial activity of leaf (a) and seed (b) AgNPs against Staphylococcus aureus.
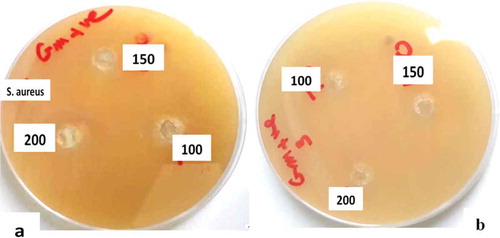
Functionalization of protected AgNPs is easier than isolated AgNPs, which may explain the higher anti-bacterial activity of colloidal AgNPs. The SEM images illustrated functionalization of protected seed-AgNPs by L-lysine functional groups (Figure ). Note the high diffusion rate of protected AgNPs to L-lysine functional groups and, therefore, the change in its distribution profile according to L-lysine functional group distribution. This NP diffusion behavior can be expected due to the basic polar groups of bacterial membranes, particularly with sulfur containing proteins (Das & Velusamy, Citation2013; McDonnell & Russell, Citation1999), resulting in the disturbance of bacterial membrane function and the death of the cell. Inhibition efficacy of both seed- and leaf-AgNPs against Gram negative is more concentration dependent than Gram positive bacteria Table . Crude root and seed extracts inhibited Gram-positive bacteria more than leaf extracts (Akbary, Fereidouni, & Akhlaghi, Citation2015), possibly due to intercalation with bacterial DNA. Both leaf and seed -AgNPs inhibited both pathogens to a similar extent. It is clear that AgNPs diminish the antibacterial activity gap between crude seed and leaf extracts in spite of a relatively higher activity of the seed extract. The AgNPs obtained with seed extract which, according to the results, are less covered by biomolecules, present better bacterial inhibitory activity than those obtained with leaf extract due to their higher diffusivity at room temperature. Expectedly, the capsulated AgNPs will show enhanced diffusion behavior under severe conditions. This study beyond the scope of our investigation.
4. Conclusions
This is the first investigation dealt with the synthesis of AgNPs utilizing the characteristic polyol macromolecules present in Ph.L leaves. Comparatively, the morphological characteristics of leaf-hosted and seed-well-separated NPs were different but their antibacterial activity was comparable. For leaf extract broth, it is found that nano-hydroxylated capsules, cavities or templates being formed on AgNPs generation (average size of 52.6 nm and 111 planes oriented) as confirmed by TEM, FE-SEM, EDX, and XRD results. Hosting polyol nano-capsules facilitates reduction, loss protection, and AgNP assembly according to 3 min. reduction time and binding affinity for the L-lysine functional group. Both leaf and seed-AgNPs inhibit the growth of Gram-positive bacteria more efficiently than Gram-negative bacteria. AgNP formation can be considered an indirect protocol to increase the antibacterial activity of the extracted active ingredients of traditional medicinal plants. Recommendation: enhancements techniques to release these losses protected and assembled AgNPs will increase their efficacy particularly in nanomedicine than the commonly separated AgNPs.
Supplymentary material
Supplymental material can be accessed for this article herehttps://doi.org/10.1080/23312009.2018.1532374
Correction
This article has been republished with minor changes. These changes do not impact the academic content of the article.
Supplemental Material
Download MS Word (10.8 MB)Acknowledgements
The authors would like to express their sincere appreciation to the Deanship of Scientific Research, Albaha University, Albaha, Kingdom of Saudi Arabian for its funding of this research through the Research Group Project number (20/1438). Titled “Synthesis of gold and AgNPs from some Saudi medical plants extracts in El-Baha region.”
Additional information
Funding
Notes on contributors
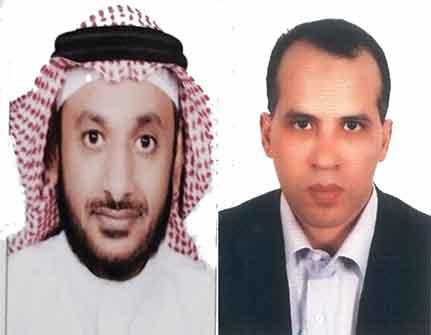
Abdulaziz Ali Alomari
My research activities are now in nanoparticles preparation for separation of tiny concentrations of heteroatom compounds from hydrocarbon matrix. Characterization and different applications of the prepared nano-particles from the goals of our studies. Silver, gold, and palladium from the target metals in our nanoparticles preparation. My current accepted manuscript dealt with the preparation of capsulated silver nanoparticles as concentrated nano forms which will be affected in nanomedicine (e.g., antibacterial) under severe conditions than the commonly separated AgNPs.
References
- Abbott, L. B., Bettmann, G. T., & Sterling, T. M. (2008). Weed Sciences, 56, 52. doi:10.1614/WS-07-094.1
- Abdel Aziz, H. G., Abdel Kader, S. M., El-Sayed, M. M., EL-Malt, E. A., & Shaker, E. S., Tenth Radiation Physics & Protection Conference, Novel β–Carboline Alkaloid from PeganumHarmala As Antibacterial Agent. 27–30 November 2010, Nasr City - Cairo, Egypt.
- Akbary, P., Fereidouni, M. S., & Akhlaghi, M. (2015). Iranian Journal of Aquatic Animal Health, 1(1), 7-16.
- Akerele, O. (1993). In: Nature’s medicinal bounty: Don’t throw it away (pp. 390). Geneva: World Health Forum, WHO.
- Al-Said, M. S. (1993). American Journal of Chinese Medicine, 21, 291. doi:10.1142/S0192415X93000340
- Amin, M., Hameed, S., Ali, A., Anwar, F., Shahid, S. A., Shakir, I., … Rahman, S. (2014). Journal of Bioinorganic Chemical Application, 2014, 1. doi:10.1155/2014/135824
- Aniszewski, T. (2007). Alkaloids – Secrets of life, alkaloid chemistry, biological significance, applications and ecological role (1st ed.). Amsterdam and Oxford: Elsevier.
- Asgarpanah, J., & Ramezanloo, F. (2012). African Journal of Pharmaceutical and Pharmacology, 6, 1573.
- Atkins, P., & de Paula, J. (2006). Atkins physical chemistry (8th ed.). Oxford University Press.
- Aziz, S. B., Saber, A. D. R., Rasheed, M. A., & Ahmed, H. M. (2017). International Journal of Electrochemical and Sciences, 12, 363. doi:10.20964/2017.01.22
- Azizia, M., Sedaghat, S., Tahvildari, K., Derakhshi, P., & Ghaemi, A. (2017). Green Chemistry Letters and Reviews, 10, 420. doi:10.1080/17518253.2017.1395081
- Basu, S., Maji, P., & Ganguly, J. (2015). Journal of Experimental Nanoscience, 11, 660. doi:10.1080/17458080.2015.1112042
- Bibi, F., & Saudi, J. (2017). Biologic Sciences, 24, 1288.
- Bremner, P., Rivera, D., Calzado, M. A., Obón, C., Inocencio, C., & Beckwith, C. (2009). Journal of Ethnopharmacol, 124, 295. doi:10.1016/j.jep.2009.05.030
- Cullity, B. D. (1978). Answers to problems: Elements of X-ray diffraction. Addison-Wesley Publishing Company.
- Das, J., & Velusamy, P. (2013). Materials Research Bulletin, 48, 4531. doi:10.1016/j.materresbull.2013.07.049
- El-Nour, K. A., Eftaiha, A., Al-Warthan, A., & Ammar, R. A. A. (2010). Arabian Journal of Chemical, 3, 135. doi:10.1016/j.arabjc.2010.04.008
- Ghaedi, M., Yousefinejad, M., Safarpoor, M., ZareKhafri, H., & Purkait, M. K. (2015). Journal of Industrial and Engineering Chemistry, 31, 167. doi:10.1016/j.jiec.2015.06.020
- Ghosh, S. K., & Pal, T. (2007). Chemical Reviews, 107, 4797. doi:10.1021/cr0680282
- Kanchana, A., Devarajan, S., & Ayyappan, S. R. (2010). Nano-Micro Letters, 2, 169. doi:10.1007/BF03353637
- Kelly, K. L., Coronado, E., Zhao, L. L., & Schatz, G. C. (2003). Journal Od Physical and Chemical, B107, 668. doi:10.1021/jp026731y
- Khuroo, M. A., Isolation, characterization and pharmacological screening of major active ingredients from medicinally important plant, Peganumharmala L, M.Sc. thesis, Department of Chemistry, University of Kashmir, India (2013)
- Kora, A. J., & Arunachalam, J. (2013). IET Nano Biotechnology, 7, 83. doi:10.1049/iet-nbt.2013.0001
- Kotb, F. T. (1983). Therapeutic medical. In J. Fabre (Ed), Medicinal plants in libya, (Arab Encyclopedia House (pp. 322). Lebanon: Beirut Press.
- Kumar, V., Yada, S., & Yada, S. K. (2010). Journal of Chemical Technological and Biotechnology, 85, 1301. doi:10.1002/jctb.2427
- Kumar, V., Yada, S. C., Yada, S. K., & Chem, J. (2010). Technological Biotechnology, 85, 1301. doi:10.1002/jctb.2427
- Magaldi, S., Mata-Essayag, S., de Capriles, C. H., Pereza, C., Colella, M. T., Olaizol, C., & Ontiveros, Y. (2004). International Journal of Infectious Diseases, 8(39), 45. doi:10.1016/j.ijid.2003.03.002
- McDonnell, G., & Russell, A. D. (1999). Clinical Microbiology Reviews, 12, 147.
- Mittal, A. K., Chisti, Y., & Banerjee, U. C. (2013). Biotechnology Advancement, 31, 346. doi:10.1016/j.biotechadv.2013.01.003
- Mody, V. V., Siwale, R., Singh, A., & Mody, H. R. (2010). Journal of Pharmaceutical and Bioallied Sciences, 2, 282. doi:10.4103/0975-7406.62708
- Mohamed, A. H. S., AL-Jammali, S. M. J., & Aki, Z. J. (2013). Journal of Sciences Innovative Researcher, 2, 585.
- Moloudizargari, M., Mikaili, P., Aghajanshakeri, S., Mohammad, H. A., & Jalal, S. (2013). Pharmacognosy Reviews, 7, 199. doi:10.4103/0973-7847.120524
- Nanda, A., & Saravanan, M. (2009). Nanomedicine: Nanotechnology, Biology and Medicine, 5, 452.
- Narang, A., & Manu, B. (2017). International Journal Emergency Researcher Management Technological, 6, 307.
- Prashanth, D., & John, S. (1999). Fitoterapia, 70(438), 438–13. doi:10.1016/S0367-326X(99)00065-9
- Rani, P. U., & Harreddy, P. R. (2011). Colloids and Surfaces A: Physicochemical and Engineering Aspects, 389, 188. doi:10.1016/j.colsurfa.2011.08.028
- Raveendran, P., Fu, J., & Wallen, S. L. (2003). Journal of American Chemical Social, 125, 13940. doi:10.1021/ja0263137
- Saabi, A. M. A. (2006). Asian Journal Plant Sciences, 5, 907. doi:10.3923/ajps.2006.907.909
- Sathishkumar, G., Gobinatha, C., Karpagam, K., Hemamalini, V., Premkumar, K., & Krishnan, S. S. (2012). Colloids and Surfaces B: Biointerfaces, 95, 235. doi:10.1016/j.colsurfb.2012.03.001
- Udayasoorian, C., Vinothkumar, K., & Jayabalakrishnan, R. M. (2011). Digest Journal of Nanomaterials and Biostructures, 6, 279.