Abstract
Epigallocatechin gallate (EGCG) is the principle antioxidant of the green tea extract. The effect of EGCG was studied against the hepatotoxicity induced by N-nitrosodiethylamine (NDEA) in rats. The male rats were exposed to NDEA (0.1 mg/ml) dissolved in drinking water separately and along with 5, 10 and 15 mg/ml of EGCG for 21 days. The activity of serum aspartate amino transferase (AST), serum alanine transaminase (ALT), alkaline phosphatase (ALP), and lactate dehydrogenase (LDH) was measured in blood serum. Lipid peroxidation, protein carbonyl content and micronucleus frequency were estimated in the rat hepatocytes. To study the effect on DNA damage, comet assay was performed on rat hepatocytes and blood lymphocytes. The results showed a dose-dependent significant decrease in the levels of blood serum enzymes (AST, ALT, ALP and LDH) in rats exposed to NDEA along with EGCG. Histological sections of the liver also revealed a protective effect of EGCG. A significant dose-dependent reduction in the lipid peroxidation, protein carbonyl content and micronucleus frequency was observed in rats exposed to NDEA along with EGCG. The results obtained from the comet assay in rat hepatocytes as well as blood lymphocytes also showed a dose-dependent significant decrease in the mean tail length. It is suggested that the EGCG is both antigenotoxic and hepatoprotective agent.
Public Interest Statement
Nitroso compounds are well-known carcinogen present in a number of food stuffs such as milk products, meat products and preserved fruit juices. Their presence has been reported to cause acute hepatocellular damage. Natural antioxidants can play a major role in combating the toxic effects of the nitroso compounds. In this context, one of the antioxidants of the green tea extracts i.e. epigallocatechin gallate (EGCG) has been studied against the toxic effects induced by N-nitrosodiethylamine (NDEA) in rats. The results suggest that the EGCG is effective in reducing the toxic effects induced by NDEA and could play a role of therapeutic agent for various kinds of liver ailments.
Competing interests
The authors declare no competing interest.
1. Introduction
N-Nitroso compounds (NOCs) are well-known carcinogens present in a vast variety of food stuffs such as milk products, meat products and preserved fruit juices (Prasad & Krishnaswamy, Citation1994). Among various NOCs, N-nitrosodiethylamine (NDEA) is a well-known hepatocarcinogen present in tobacco smoke, water containing high concentration of nitrates, fried meals, cosmetics, agricultural chemicals and pharmaceutical agents (Ajiboye et al., Citation2013). NOCs are also responsible for the induction of hepatic fibrosis and also alter various biochemical parameters (Ahmad & Ahmad, Citation2012). The changes in chromosomal numbers, non random chromosome aberrations and DNA damage are frequently associated with chemical toxicity and carcinogenesis (Roy, Chakrabarty, Sinha, Bhattacharya, & Siddiqi, Citation2003). Flavonoids are naturally occurring polyphenolic compounds found in fruits, vegetables and some drinks like red wine, beer and green tea (Cabrera, Artacho, & Giménez, Citation2006). Various in vivo and in vitro studies have shown that plant polyphenols enhances intracellular antioxidants and anti-inflammatory capacity, therefore can be used as chemopreventive agents (Ali, Rahul, Naz, & Jyoti, Citation2015; Amin, Kucuk, Khuri, & Shin, Citation2009; Hong et al., Citation2012; Shin, Wang, Zheng, Xiang, & Liang, Citation2014). Epigallocatechin-3-gallate (EGCG), an important component of tea, has shown a number of protective effects, such as against ultraviolet A induced photo damage in human skin fibroblasts, (Shin et al., Citation2014), oesophageal cancers (Yang & Wang, Citation1993), stomach cancers (Oguni, Chen, Lin, & Hara, Citation1992) and hepatic cancers (Zhang, Wu, & Weng, Citation2014). Since thousands of years, the leaves of Camellia sinensis are being used as a traditional medicine in several Asian countries (Isbrucker, Bausch, Edwards, & Wolz, Citation2006). Several in vitro and in vivo studies have been carried out in order to understand the biochemical and cellular properties of various compounds present in the tea leaf extract (Hou, Lambert, Chin, & Yang, Citation2004). Among various poly-phenolic substances, EGCG is generally considered as a principal antioxidant in the green tea extract (Isbrucker et al., Citation2006). Besides having antigenotoxic/antioxidant potential the researchers have reported it as a pro-oxidant and genotoxic agent at high concentrations (Furukawa, Oikawa, Murata, Hiraku, & Kawanishi, Citation2003; Roy et al., Citation2003). At high concentration EGCG catalyzes the formation of hydrogen peroxide responsible for its genotoxic potential (Long, Clement, & Halliwell, Citation2000). A low incidence of various types of cancer has also been reported with the frequent consumption of fruits and vegetables (Yang, Lee, Chen, & Yang, Citation1997). Many epidemiological, in vitro cell culture and in vivo animal studies have been shown cancer chemopreventive activity of EGCG (Ahmad, Katiyar, & Mukhtar, Citation1998; Katiyar & Mukhtar, Citation1996). During the manufacturing of black tea, most of the tea polyphenols such as EGCG, epigallo catechin (EGC), and epicatechin are oxidized and polymerized to form flavins (Yang et al., Citation1997). Green tea infusion has been reported to reduce the incidence of lung tumour by NDEA and 4-methylnitrosoamino-1-(3-pyridyl)-1-butanone (NNK) (Wang, Agarwal, Khan, & Mukhtar, Citation1992). Not only the lung carcinomas but also the oesophageal (Wang et al., Citation1995) and gastric (Katiyar, Agarwal, Wood, & Mukhtar, Citation1992; Wang et al., Citation1995) carcinomas induced by NDEA and other nitrosoamines have been reported to be reduced by the consumption of tea extract.
In the present study, the effect of EGCG was studied against NDEA induced toxicity in rats and the results indicate that EGCG is effective in reducing the toxic effects of NDEA.
2. Materials and methods
2.1. Chemicals
N-nitrosodiethylamine and EGCG were purchased from Sigma chemicals Co. (USA). Agarose (normal and low melting), Triton X, ethidium bromide, dimethylsulphoxide, Tris, EDTA and all other chemicals were purchased from SISCO Research Laboratories, India. May Grunwald’s stain and Giemsa stain were procured from Merck Ltd. (India).
2.2. Animals and treatment
Male rats (Wistar strain), weighing 100–120 g, were used in the study. The animals were divided into nine groups (5 rats/group). The first group was allowed to feed on N-nitrosodiethylamine (NDEA) dissolved in water (0.1 mg/ml), second group was allowed to feed on water having NDEA (0.1 mg/ml) plus EGCG (5 mg/ml), third group was allowed to feed on water having NDEA (0.1 mg/ml) plus EGCG (10 mg/ml), fourth group was allowed to feed on water having NDEA (0.1 mg/ml) plus EGCG (15 mg/ml), fifth group served as a control (normal drinking water), sixth, seventh, and eight group were allowed to feed on water having EGCG at final concentration of 5, 10 and 15 mg/ml, respectively. Ninth group was taken as a negative control and was allowed to feed on water having a dimethysulphoxide (DMSO) (3 μl/ml). EGCG was dissolved in 0.03% DMSO and in drinking water the final concentration of 5, 10, and 15 mg/ml were established. The rats were allowed to feed ad libitum for 21 days and were sacrificed under mild ether anaesthesia. All care and animal ethics were taken in consideration while performing the experiments and the study was approved by ethical committee.
2.3. Histological evaluation of the liver
A portion of liver was removed and washed thoroughly with 0.9% saline. The tissue was kept in 10% buffered neutral formalin (BNF) for 24 h. Then the fixed liver specimens from each group were embedded in paraffin and processed for light microscopy by staining individual sections with haematoxylin-eosin stain.
2.4. Biochemical analysis
The blood samples were collected directly by cardiac puncture in a vacutainer having a clot activator (AKÜret, Medkit). The serum was collected for the biochemical analysis of serum Aspartate amino transferase (AST), serum alanine transaminase (ALT), alkaline phosphatase (ALP) and lactate dehydrogenase (LDH). The levels of the enzymes were estimated according to the method described in the commercial kits (Crest Biosystems, India).
2.5. Preparation of liver homogenate
The homogenate was prepared according to the procedure described by Singh, Kaur, Sandhir, and Kiran (Citation2008) with little modification. The livers were washed thoroughly with chilled 0.9% saline. The final wash was given with chilled homogenizing buffer (pH 7.5). The homogenizing buffer was prepared by adding 0.024 M EDTA, 0.075 M NaCl and DMSO 10% in 1 litre of distilled water. After weighing, the liver was mixed, suspended in chilled homogenizing buffer at a concentration of 1 g/ml, and was homogenized on ice using homogenization at 300 g. The homogenate was then centrifuged at 5,500 g for 10 min at 4°C. The supernatant was removed and kept at −20°C for further analyses.
2.6. Estimation of lipid peroxidation
The method described by Siddique, Ara, and Afzal (Citation2012) was used for the estimation of lipid peroxidation in liver tissue. Reagent1 (R1) was prepared by dissolving 0.064 g of 1-methyl-2-phenylindole into 30 ml of acetonitrile. The preparation of 37% HCl served as the reagent R2. About 200 μl of diluted liver homogenate (protein concentration of approx 1 mg/ml) was mixed with 300 μl of R1. Then 300 μl of R2 was added to the tube, vortexed and incubated at 45°C for 40 min. After incubation, the tubes were cooled in ice and centrifuged at 11,000 g at 4°C. The absorbance was read at 586 nm.
2.7. Estimation of protein carbonyl content
The protein carbonyl content was estimated according to the protocol described by Hawkins, Morgan, and Davies (Citation2009). The liver homogenate was diluted to a protein concentration of approx 1 mg/ml. About 250 μl of each diluted homogenate was taken in eppendorf centrifuge tubes separately. To it 250 μl of 10 mM 2, 4-dinitrophenyl hydrazine (dissolved in 2.5 M HCl) was added, vortexed and kept in dark for 20 min. About 125 μl of 50% (w/v) trichloroacetic acid (TCA) was added, mixed thoroughly and incubated at −20°C for 15 min. The tubes were then centrifuged at 4°C for 10 min at 9,000 g. The supernatant was discarded and the pellet obtained was washed twice by ice cold ethanol:ethylacetate (1:1). Finally the pellets were re-dissolved in 1 ml of 6 M guanidine hydrochloride and the absorbance was read at 370 nm.
2.8. Micronucleus assay
Micronucleus assay was performed according to the method of Igarashi and Shimada (Citation1997). A drop of tissue homogenate put at one end of the pre-cleaned, grease-free microscopic slides and was spread using coverslip held at an angle of 45° into a smooth layer. Before staining, the slides were allowed to air-dried in a dust-free environment for at least 12 h. The slides were then stained for 2 min in May-Grunwald stain (0.25% in methanol) followed by staining with 10% Giemsa for 10 min. The slides were then rinsed twice in distilled water, dried and finally rinsed with methanol. The slides were then cleared in xylene and mounted in DPX. A total of 500 cells were counted per rat for the presence of micronuclei using light microscope at 40X.
2.9. Comet assay
Comet assay was performed according to the method described by Singh, McCoy, Tice, and Schneider (Citation1988) and with modification as suggested by Dhawan et al. (Citation2002). Frosted microscopic slides were dipped in 1% normal melting agarose and the underside was wiped to remove the agarose (dissolved in PBS). The slides were allowed to dry for 24 h. Next day for the liver cells 40 μl of the cell suspension was mixed with 60 μl of 0.5% low melting agarose (dissolved in PBS) was layered on the prepared base slides. For the separation of blood lymphocytes 20 μl of whole blood in 1 ml of RPMI 1640 and 100 μl of Ficoll histopaque was added and centrifuged at 1500 rpm for 15 min and finally the obtained pellet was re-suspended in 70 μl of LMPA. A cover slip was placed for the even spread of the suspension. The slides were allowed for solidifying at 4°C for 10 min. The cover slip was removed and a third layer of 1% low melting agarose was added and allowed to solidify on ice pack for 5 min. The slides were then immersed in cold lysis solution (2.5 M NaCl, 100 mM EDTA, 10 mM Tris and 1% triton X-100, pH 10) at 4°C for overnight. Next day the slides were kept for 30 min in alkaline electrophoretic buffer (300 mM NaOH, 1 mM EDTA; pH > 13) for unwinding of the DNA. Electrophoresis was performed at 4°C at 24 volts for 45 min. The slides were then washed with neutralization buffer (0.4 M Tris) and stained with ethidium bromide (20 μg/ml). Three slides were prepared per rat and a total of 50 randomly captured comets per slide under fluorescence microscope were analyzed for scoring comet tail length using comet 1.5 software (TriTek Corporation).
2.10. Statistical analysis
All data were expressed as the mean ± standard error and student’s t-test was used for the analysis. Statistical significance was estimated at the 5% level.
3. Results
The histomorphological study of the liver sections revealed a normal structure of the hepatocytes in control group (Figure (a)). The exposure of rats to NDEA (0.1 mg/ml) showed sinusoidal dilution and swollen hepatocytes. The degeneration in liver cells is evident by the formation of swollen nucleus and vacuoles (Figure (b)). Figure (c) shows the liver exposed to NDEA (0.1 mg/ml) along with 15 mg/ml of EGCG for 21 days. The significant increase in the serum enzymes is an indicator of damage in the liver plasma membrane due to oxidation of polyunsaturated fatty acids in the plasma membrane by ROS generated by the metabolism of NDEA. The result obtained for the AST activity is shown in Figure . The rats exposed to NDEA (0.1 mg/ml) were associated with almost 4-fold increase, compared to the control (p < 0.05) (Figure ). A dose-dependent decrease in the mean values for AST was observed in the rats exposed to EGCG along with NDEA (Figure ). A 3.3-fold increase in the ALT (U/ml) was observed as compared to control (p < 0.05) in rats exposed to NDEA (0.1 mg/ml) (Figure ). A dose-dependent decrease in the mean value of ALT (U/L) was observed in rats exposed to NDEA along with various doses of EGCG (Figure ). A 2.45-fold increase in the ALP (U/L) was observed in rats exposed to NDEA (0.1 mg/ml) as compared to control (Figure ). A dose-dependent decrease in the mean value of ALP (U/L) was observed in rats exposed to NDEA along with various doses of EGCG (Figure ). A 1.78-fold increase in LDH (U/L) was observed in rats exposed to only NDEA (0.1 mg/ml) (Figure ). A dose-dependent decrease in the mean value of LDH (U/L) was observed in rats exposed to various doses of EGCG (Figure ).
Figure 1. Rat liver sections stained with haematoxylin-eosin (200× magnification) (a) control; (b) rats exposed to NDEA (0.1 mg/ml) for 21 days, and (c) rats exposed to 0.1 mg/ml of NDEA along with 15 mg/ml of epigallocatechin gallate.
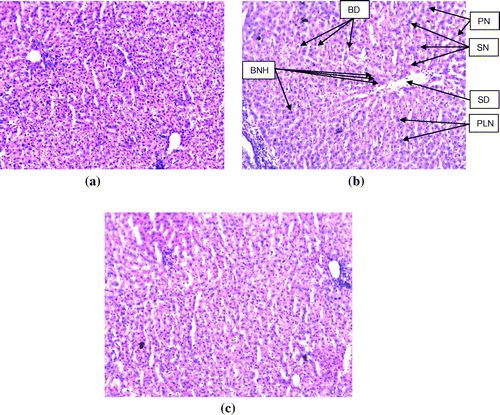
Figure 2. Aspartate aminotransferase (AST) activity measured after 21 days of treatment of N-nitrosodiethylamine separately and along with different doses of epigallocatechin gallate in rats.
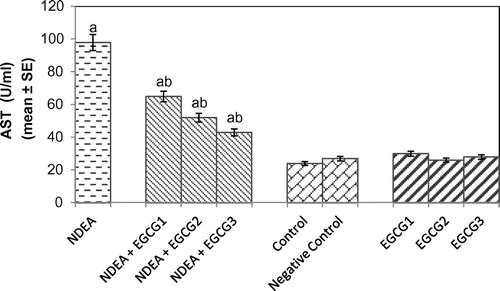
Figure 3. Alanine transaminase (ALT) activity measured after 21 days of treatment of N-nitrosodiethylamine separately and along with different doses of epigallocatechin gallate in rats.
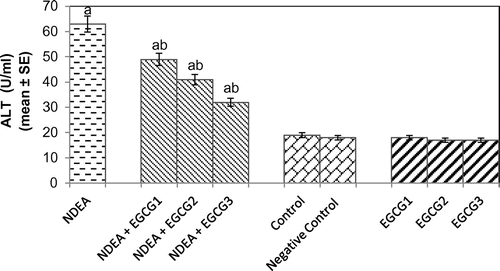
Figure 4. Alkaline phosphatase (ALP) activity measured after 21 days of treatment of N-nitrosodiethylamine separately and along with different doses of epigallocatechin gallate in rats.
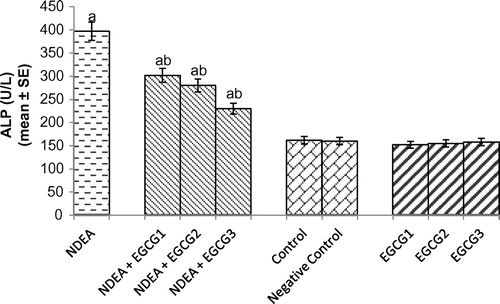
Figure 5. Lactate dehydrogenase (LDH) activity measured after 21 days of treatment of N-nitrosodiethylamine separately and along with different doses of epigallocatechin gallate in rats.
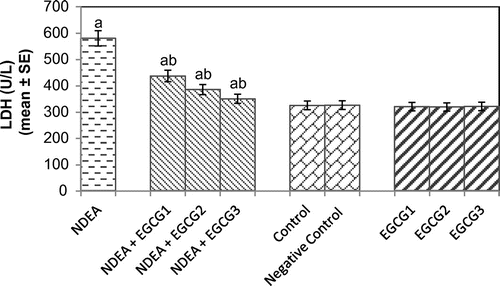
Lipid peroxidation is a measure of oxidative stress resulting from the production of reactive oxygen species (ROS) that cause damage to lipid, DNA and protein. The results obtained for the lipid peroxidation assay are shown in Figure . The rats exposed to NDEA (0.1 mg/ml) showed a 3.76-fold increase in the mean absorbance value as compared to control (p < 0.05) (Figure ). A dose-dependent decrease was observed in rats exposed to various doses of EGCG along with 0.1 mg/ml of NDEA as compared to NDEA treatment alone (p < 0.05) (Figure ).
Figure 6. Lipid peroxidation measured in rat liver after 21 days of treatment with NDEA alone and together with different amounts of epigallocatechin gallate.
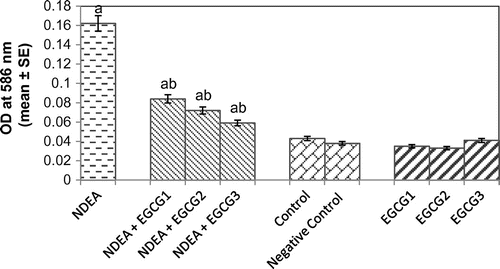
Protein carbonyl content gives us an estimation of the oxidation of proteins. The result obtained for protein carbonyl content is shown in Figure . An almost 2.55-fold increase in the mean absorbance value was observed in rats exposed to NDEA (0.1 mg/ml) alone as compared to treated (p < 0.05) (Figure ). A dose-dependent significant decrease in the mean absorbance value for PC content was observed in rats exposed to various doses of EGCG along with NDEA (0.1 mg/ml) (Figure ). The micronucleus assay is a useful end point for bio-monitoring studies and has found a wide application in the assessment of chromosomal damage in vitro and in vivo caused by different mutagens. The formation of micronucleus in rat hepatocytes is shown in Figure (a) and (b). The results obtained for micronucleus frequency in hepatocytes is shown in Figure (c). Exposure of rats to NDEA (0.1 mg/ml) produced a mean value of 0.052 ± 0.98/cell (p < 0.05). The exposure of rats to NDEA (0.1 mg/ml) together with of EGCG was associated with 0.040 ± 0.82, 0.034 ± 0.70 and 0.024 ± 0.65, respectively (Figure (c)). Figure (a)–(c) shows the comet assay performed on hepatocytes. A dose-dependent significant decrease in the mean tail length was observed in the rat hepatocytes exposed to various doses of EGCG along with 0.1 mg/ml of NDEA (Figure (d)) (p < 0.05). Figure (a)–(c) shows the comet assay performed on blood lymphocytes. A dose-dependent significant decrease in the mean tail length was observed in rat blood lymphocytes exposed to various doses of EGCG along with 0.1 mg/ml of NDEA (Figure (d)) (p < 0.05).
Figure 7. Protein carbonyl content measured in rat liver after 21 days of treatment with NDEA alone and together with different amounts of epigallocatechin gallate.
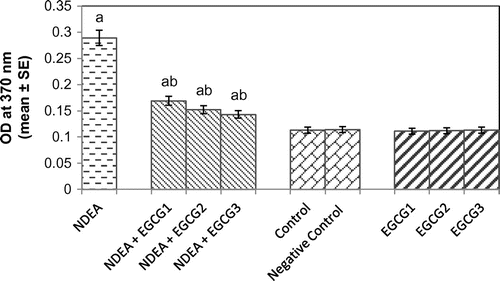
Figure 8. (a) Hepatocytes with control, (b) treated with NDEA (0.1 m/ml), and (c) Micronucleus formation in hepatocytes after 21 days of treatment of rats with NDEA alone and together with different amounts of epigallocatechin gallate.
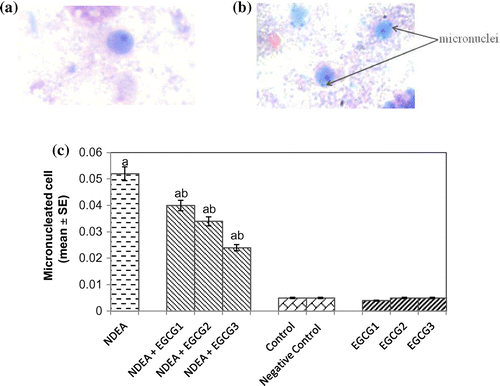
Figure 9. (a) Control, (b) treated with NDEA (0.1 mg/ml), and (c) Rats exposed to 0.1 mg/ml of NDEA along with 15 mg/ml of epigallocatechin gallate (d) Comet tail length in rat hepatocytes after 21 days of treatment of rats with NDEA alone and together with different amounts of epigallocatechin gallate.
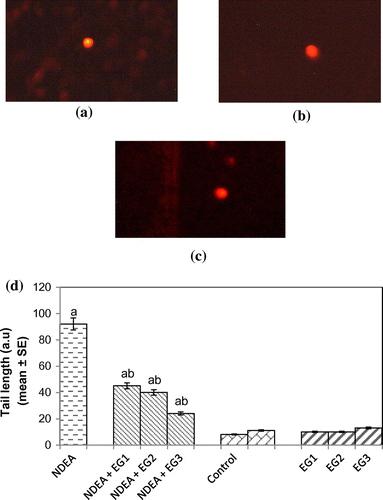
Figure 10. (a) Control, (b) treated with NDEA (0.1 mg/ml), and (c) Rats exposed to 0.1 mg/ml of NDEA along with 15 mg/ml of epigallocatechin gallate (d) Comet tail length in rat blood lymphocytes after 21 days of treatment of rats with NDEA alone, or together with different amounts of epigallocatechin gallate.
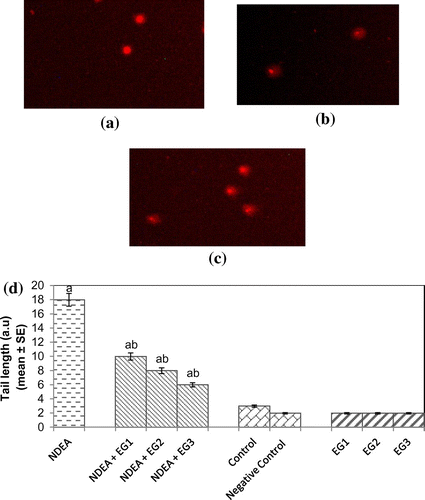
4. Discussion
The results obtained in the present study reveal the protective effect of EGCG against the toxicity induced by NDEA in rats. In liver the metabolic activation of NDEA leads to the formation of active ethyl radical metabolite (CH3CH2+) (Verna, Whysner, & Williams, Citation1996). The active ethyl radical metabolite causes oxidative stress and cellular injury by generating reactive oxygen species (Bansal, Trivedi, Soni, & Bhatnagar, Citation2000). As a result there is an increase in the serum indices of liver function. Many polyphenolic compounds have been demonstrated as an anticarcinogenic, anticlastogenic, antigenotoxic and antiapoptotic in animals as well as in in vitro models (Ali, Rahul, Naz, & Jyoti, Citation2014; Yang et al., Citation1997; Roy et al., Citation2003; Shin et al., Citation2014). A number of polyphenolic compounds possess antioxidant as well as other biological activities (Yang et al., Citation1997). Among various tea polyphenols EGCG is a potent-free radical scavenger as it curtailed the damaging effects of UVA by inhibiting the generation of superoxide anions and lipid peroxidation (Shin et al., Citation2014). In our present study, a decrease in the protein carbonyl content and lipid peroxidation also suggests the role of EGCG as a free radical scavenger. The antigenotoxic and anticlastogenic role of EGCG has been well documented (Kuroda & Hara, Citation1999). In our present study, the treatment of EGCG results in a reduction in the frequency of micronucleated cells and DNA damage in liver cells in a dose-dependent manner, induced by NDEA. The exposure of rats at various doses of EGCG also showed a dose-dependent increase in the GSH content. This increase in GSH content may play a role in combating the toxic effects induced by NDEA in our present study. The exposure of rats to NDEA and EGCG in combination showed a decrease in the GST activity. Now-a-days, an emphasis has been given towards the herbal bio-enhancers which themselves do not possess inherent pharmacological activity of their own but can modulate the activity of other compounds (Alexander et al., Citation2014). The oral administration of EGCG even at 2,000 mg/kg to mice and administering 1,200 mg/kg/day of EGCG for 10 days did not induce bone marrow cell micronuclei and produced plasma EGCG concentrations comparable to those reported in human studies (Isbrucker et al., Citation2006). In our present study, the selected doses of EGCG were found to be potent in modulating the activity of NDEA. After oral absorption the rapid methylation of EGCG is catalyzed by liver cytosolic catechol-O-methyltransferase and further sulphation/glucuronidation leads to the elimination of methylated EGCG (Mereles & Hunstein, Citation2011). At higher doses EGCG has been reported to be hepatotoxic (Lambert et al., Citation2010). However, the hepatotoxicity has been linked with the consumption of high doses of tea-based dietary supplements (10–29 mg/kg/day) (Bonkovsky, Citation2006). The use of endosulfan in a green tea plantations was considered as an additive factor for the hepatotoxicity (Bonkovsky, Citation2006; Mereles & Hunstein, Citation2011). The micronucleus assay is being considered as a useful endpoint for the assessment of chromosomal damage in vitro and in vivo caused by different mutagens (Garaj-Vrhovac, Đurinec, Kopjar, & Oreščanin, Citation2008). MN arises during cell division either from chromosomes that are lagging in anaphase or from chromosome fragments (Roy et al., Citation2003).
In our present study, micronucleus formation was evaluated in liver cells of treated and control rats. The data obtained clearly demonstrate the protective effect of EGCG against NDEA. The selected doses of EGCG did not induce significant MN compared to the MN level in the untreated rats. The comet assay performed on rat hepatocytes and blood lymphocytes showed the DNA damage by the exposure of NDEA. The treatment of EGCG showed a dose-dependent reduction in the DNA damage by NDEA in the hepatocytes as well as blood lymphocytes. Green tea extract has been reported to improve liver enzymes activity, antioxidant status, as well as suppress DNA fragmentation and the expression of the caspase-3 and Bax proteins against copper nanoparticles induced hepatotoxicity in rats (Ibrahim, Khalaf, Galal, Ogaly, & Hassan, Citation2015). Single cell gel electrophoresis (SCGE) or comet assay is a sensitive method to detect single strand breaks. (Singh et al., Citation1988). Metabolically active nitrosamines are capable of initiating DNA damage in cells as is evident in MN as well as comet assay (Taniguchi, Yasutake, Takedomi, & Inoue, Citation1999).
ROS may play an important role in the process of carcinogenesis by damaging DNA, altering the expression of gene, growth and differentiation (Cerutti, Citation1988; Feig, Reid, & Loeb, Citation1994). Inhibition of nitrosation by tea extract has been demonstrated in vitro and in humans, thereby preventing the various cancers by nitroso compounds (Stich, Citation1992). Although the anti genotoxic and anti carcinogenic activities of tea extract are well documented in various animal and in vitro models but the similar activity in humans are still warranted (Yang et al., Citation1997).
EGCG along with ascorbic acid showed protective effect against the genotoxic damage induced in mice by endogenous nitrosation (Abraham & Khandelwal, Citation2013). The antigenotoxic potential of EGCG and black tea polyphenols against heterocyclic amines has also been studied by using comet assay (Dhawan et al., Citation2002). A reduction in the frequency of micronuclei induced by chromium trioxide in polychromatic erythrocytes of mouse peripheral blood was observed by the treatment of EGCG (del Carmen García-Rodríguez, Nicolás-Méndez, Montaño-Rodríguez, & Altamirano-Lozano, Citation2014). EGCG protected the hepatocytes against D-Galactosamine (Gal N) induced cytotoxicity in cultured rat hepatocytes (Moravcova, Cervinkova, Kucera, Mezera, & Lotkova, Citation2014). Green tea extract has been reported to decrease the oxidative and nitrative stress induced by pro inflammatory enzymes during non alcoholic steatohepatitis (NASH) in obese mice (Chung, Park, Manautou, Koo, & Bruno, Citation2012). EGCG has been reported to reduce the metabolism and toxicity of acetaminophen in rats (Yao, Yang, Chang, Yang, & Yin, Citation2015). In other study, EGCG has been reported to remove intracellular labile iron pool (ILP) and reactive oxygen species (ROS) thus preventing the cellular and mitochondrial damage in cultured hepatocytes (Srichairatanakool, Kulprachakarn, Pangjit, Pattanapanyasat, & Fuchaeron, Citation2012). EGCG has been reported to prevent hepatocellular carcinoma (HCC) by restoring heparan-sulphate proteoglycans (HSPGs) receptors in rats induced by thioacetamide (Darweish, Abbas, Ebrahim, & Al-Gayyar, Citation2014). EGCG has been reported as a new anti-hepatitis C virus (anti-HCV) molecule and its effect has been extensively reviewed by Calland, Dubuisson, Rouillé, & Séron, Citation2012. Administration of EGCG to sodium fluoride intoxicated rats significantly retrieve the distorted biochemical indices, DNA damage and pathological changes in liver tissues due to the free radical scavenging, antioxidant, and antigenotoxic properties of EGCG (Thangapandiyan & Miltonprabu, Citation2013). The green tea polyphenols have also been reported to prevent the carbon tetrachloride-induced hepatotoxicity in mice by down regulating the nitric oxide derived pro-oxidants (Chen et al., Citation2004). A reduction in the level of blood serum enzymes (SGOT, SGPT, ALP and LDH) with the treatment of EGCG is in correlation with the study carried out by Thangapandiyan and Miltonprabu (Citation2013). EGCG has also been reported to have antitumour properties against a wide range of skin tumors (Agarwal, Katiyar, Zaidi, & Mukhtar, Citation1992).
4. Conclusion
A number of adverse health effects have been associated with consumption of amines. The formation of nitrite from the ingested nitrate leads to the formation of carcinogenic nitroso compounds. Any process/compound that could prevent the contact between nitrites and nitrosating compounds in food may be an effective way of reducing the concentrations of nitroso compounds, thereby reducing the toxic effects. Both the properties i.e. ROS scavenging and down regulating the production of inducible nitric oxide derived pro-oxidants have been attributed to EGCG. Since green tea has been consumed over long periods without any known side effects, its possible role as an adjunct therapeutic agent against the hepatotoxicity induced by nitroso compounds cannot be ruled out.
Acknowledgement
We are thankful to the Chairman, Department of Zoology, AMU, Aligarh for providing laboratory facilities.
Additional information
Funding
Notes on contributors
Yasir Hasan Siddique
Dr Siddique is an assistant professor in the Department of Zoology, Aligarh Muslim University, Aligarh. His research interest includes Toxicology and Neurodegenerative disorders. He has an experience of working with animal models (mice, rat, Drosophila) and in vitro cell culture.
Fahad Ali
Fahad Ali (ICMR-JRF) is working as a PhD student. Our study suggests that EGCG is effective in reducing the toxic effects induced by NDEA and could play a role of therapeutic agent for various kinds of liver ailments.
References
- Abraham, S. K., & Khandelwal, N. (2013). Ascorbic acid and dietary polyphenol combinations protect against genotoxic damage induced in mice by endogenous nitrosation. Mutation Research/Genetic Toxicology and Environmental Mutagenesis, 757, 167–172.10.1016/j.mrgentox.2013.08.004
- Agarwal, R., Katiyar, S. K., Zaidi, S. I. A., & Mukhtar, H. (1992). Inhibition of skin tumour promoter-caused induction of epidermal ornithine decarboxylase in SENCAR mice by polyphenolic fraction isolated from green tea and its individual epicatechin derivatives. Cancer Research, 52, 3582–3588.
- Ahmad, R., & Ahmad, A. (2012). Understanding the mechanism of hepatic fibrosis and potential therapeutic approaches. Saudi Journal of Gastroenterology, 18, 155–167.10.4103/1319-3767.96445
- Ahmad, N., Katiyar, S. K., & Mukhtar, H. (1998). Cancer chemoprevention by tea polyphenols. Nutrition and Chemical Toxicity, 301–343.
- Alexander, A., Qureshi, A., Kumari, L., Vaishnav, P., Sharma, M., Saraf, S., & Saraf, S. (2014). Role of herbal bioactives as a potential bioavailability enhancer for Active Pharmaceutical Ingredients. Fitoterapia, 97, 1–14.
- Ajiboye, T. O., Komolafe, Y. O., Oloyede, O. B., Ogunbode, S. M., Adeoye, M. D., Abdulsalami, I. O., & Nurudeen, Q. O. (2013). Polyphenolic extract of Sorghum bicolor grains enhances reactive oxygen species detoxification in N-nitrosodiethylamine-treated rats. Food Science and Human Wellness, 2, 39–45.10.1016/j.fshw.2013.02.001
- Ali, F., Rahul, F., Naz, F., & Jyoti, S. (2014). Protective effect of apigenin against N-nitrosodiethylamine (NDEA)-induced hepatotoxicity in albino rats. Mutation Research/Genetic Toxicology and Environmental Mutagenesis, 767, 13–20.10.1016/j.mrgentox.2014.04.006
- Ali, F., Rahul, F., Naz, F.q, & Jyoti, S. (2015). Protective effect of Genistein against N-nitrosodiethylamine (NDEA)-induced hepatotoxicity in Swiss albino rats. Journal of Pharmaceutical Analysis, 5, 51–57.10.1016/j.jpha.2014.07.003
- Amin, A. R., Kucuk, O., Khuri, F. R., & Shin, D. M. (2009). Perspectives for cancer prevention with natural compounds. Journal of Clinical Oncology, 27, 2712–2725.10.1200/JCO.2008.20.6235
- Bansal, A. K., Trivedi, R., Soni, G. L., & Bhatnagar, D. (2000). Hepatic and renal oxidative stress in acute toxicity of N-nitrosodiethylamine in rats. Indian journal of experimental biology, 38, 916–920.
- Bonkovsky, H. L. (2006). Hepatotoxicity associated with supplements containing chinese green tea (Camellia sinensis). Annals of Internal Medicine, 144, 68–71.10.7326/0003-4819-144-1-200601030-00020
- Cabrera, C., Artacho, R., & Giménez, R. (2006). Beneficial effects of green tea—a review. Journal of the American College of Nutrition, 25, 79–99.10.1080/07315724.2006.10719518
- Calland, N., Dubuisson, J., Rouillé, Y., & Séron, K. (2012). Hepatitis C virus and natural compounds: A new antiviral approach? Viruses, 4, 2197–2217.10.3390/v4102197
- Cerutti, P. A. (1988). Mechanisms of action of oxidant carcinogens. Cancer detection and prevention, 14, 281–284.
- Chen, J. H., Tipoe, G. L., Liong, E. C., So, H. S., Leung, K. M., Tom, W. M., … Nanji, A. A. (2004). Green tea polyphenols prevent toxin-induced hepatotoxicity in mice by down-regulating inducible nitric oxide–derived prooxidants. The American Journal of Clinical Nutrition, 80, 742–751.
- Chung, M. Y., Park, H. J., Manautou, J. E., Koo, S. I., & Bruno, R. S. (2012). Green tea extract protects against nonalcoholic steatohepatitis in ob/ob mice by decreasing oxidative and nitrative stress responses induced by proinflammatory enzymes. The Journal of Nutritional Biochemistry, 23, 361–367.10.1016/j.jnutbio.2011.01.001
- Darweish, M. M., Abbas, A., Ebrahim, M. A., & Al-Gayyar, M. M. (2014). Chemopreventive and hepatoprotective effects of Epigallocatechin-gallate against hepatocellular carcinoma: role of heparan sulfate proteoglycans pathway. Journal of Pharmacy and Pharmacology, 66, 1032–1045.10.1111/jphp.2014.66.issue-7
- Dhawan, A., Anderson, D., de Pascual-Teresa, S., Santos-Buelga, C., Clifford, M. N., & Ioannides, C. (2002). Evaluation of the antigenotoxic potential of monomeric and dimeric flavanols, and black tea polyphenols against heterocyclic amine-induced DNA damage in human lymphocytes using the Comet assay. Mutation Research/Genetic Toxicology and Environmental Mutagenesis, 515, 39–56.10.1016/S1383-5718(01)00347-3
- Feig, D. I., Reid, T. M., & Loeb, L. A. (1994). Reactive oxygen species in tumorigenesis. Cancer Research, 54, 1890s–1894s.
- Furukawa, A., Oikawa, S., Murata, M., Hiraku, Y., & Kawanishi, S. (2003). (−)-Epigallocatechin gallate causes oxidative damage to isolated and cellular DNA. Biochemical Pharmacology, 66, 1769–1778.10.1016/S0006-2952(03)00541-0
- Garaj-Vrhovac, V., Đurinec, M., Kopjar, N., & Oreščanin, V. (2008). A survey on the cytogenetic status of the Croatian general population by use of the cytokinesis-block micronucleus assay. Mutation Research/Genetic Toxicology and Environmental Mutagenesis, 649(1-2), 91–100.10.1016/j.mrgentox.2007.08.008
- del Carmen García-Rodríguez, M., Nicolás-Méndez, T., Montaño-Rodríguez, A. R., & Altamirano-Lozano, M. A. (2014). Antigenotoxic effects of (–)-epigallocatechin-3-gallate (EGCG), quercetin, and rutin on chromium trioxide-induced micronuclei in the polychromatic erythrocytes of mouse peripheral blood. Journal of Toxicology and Environmental Health, Part A, 77, 324–336.10.1080/15287394.2013.865006
- Hawkins, C. L., Morgan, P. E., & Davies, M. J. (2009). Quantification of protein modification by oxidants. Free Radical Biology and Medicine, 46, 965–988.10.1016/j.freeradbiomed.2009.01.007
- Hong, Y. H., Jung, E. Y., Shin, K. S., Kim, T. Y., Yu, K. W., Chang, U. J., & Suh, H. J. (2012). photoprotective effects of a formulation containing tannase-converted green tea extract against UVB-induced oxidative stress in hairless mice. Applied Biochemistry and Biotechnology, 166, 165–175.10.1007/s12010-011-9413-x
- Hou, Z., Lambert, J. D., Chin, K. V., & Yang, C. S. (2004). Effects of tea polyphenols on signal transduction pathways related to cancer chemoprevention. Mutation Research/Fundamental and Molecular Mechanisms of Mutagenesis, 555, 3–19.10.1016/j.mrfmmm.2004.06.040
- Ibrahim, M. A., Khalaf, A. A., Galal, M. K., Ogaly, H. A., & Hassan, A. H. (2015). Ameliorative influence of green tea extract on copper nanoparticle-induced hepatotoxicity in rats. Nanoscale Research Letters, 10, 1–9.
- Igarashi, M., & Shimada, H. (1997). An improved method for the mouse liver micronucleus test. Mutation Research/Genetic Toxicology and Environmental Mutagenesis, 391, 49–55.10.1016/S0165-1218(97)00031-1
- Isbrucker, R. A., Bausch, J., Edwards, J. A., & Wolz, E. (2006). Safety studies on epigallocatechin gallate (EGCG) preparations. Part 1: Genotoxicity. Food and Chemical Toxicology, 44, 626–635.10.1016/j.fct.2005.07.005
- Katiyar, S., & Mukhtar, H. (1996). Tea in chemoprevention of cancer. International Journal of Oncology, 8, 221–238.
- Katiyar, S. K., Agarwal, R., Wood, G. S., & Mukhtar, H. (1992). Inhibition of 12-O-tetradecanoylphorbol-13-acetate-caused tumor promotion in 7, 12-dimethylbenz [a] anthracene-initiated SENCAR mouse skin by a polyphenolic fraction isolated from green tea. Cancer Research, 52, 6890–6897.
- Kuroda, Y., & Hara, Y. (1999). Antimutagenic and anticarcinogenic activity of tea polyphenols. Mutation Research/Reviews in Mutation Research, 436, 69–97.10.1016/S1383-5742(98)00019-2
- Lambert, J. D., Kennett, M. J., Sang, S., Reuhl, K. R., Ju, J., & Yang, C. S. (2010). Hepatotoxicity of high oral dose (−)-epigallocatechin-3-gallate in mice. Food and Chemical Toxicology, 48, 409–416.10.1016/j.fct.2009.10.030
- Long, L. H., Clement, M. V., & Halliwell, B. (2000). Artifacts in cell culture: Rapid generation of hydrogen peroxide on addition of (−)-epigallocatechin, (−)-epigallocatechin gallate, (+)-catechin, and quercetin to commonly used cell culture media. Biochemical and Biophysical Research Communications, 273, 50–53.10.1006/bbrc.2000.2895
- Moravcova, A., Cervinkova, Z., Kucera, O., Mezera, V., & Lotkova, H. (2014). Antioxidative effect of epigallocatechin gallate against D-galactosamine-induced injury in primary culture of rat hepatocytes. Acta Medica, 57, 3–8.
- Mereles, D., & Hunstein, W. (2011). Epigallocatechin-3-gallate (EGCG) for clinical trials: more pitfalls than promises? International Journal of Molecular Sciences, 12, 5592–5603.10.3390/ijms12095592
- Oguni, I., Chen, S. J., Lin, P. Z., & Hara, Y. (1992). Protection against cancer risk by Japanese green tea. Preventive Medicine, 21, 332.
- Prasad, M. P., & Krishnaswamy, K. (1994). N-nitrosamines in Indian beers. Indian Journal of Medical Research, 100, 299–301.
- Roy, M., Chakrabarty, S., Sinha, D., Bhattacharya, R. K., & Siddiqi, M. (2003). Anticlastogenic, antigenotoxic and apoptotic activity of epigallocatechin gallate: a green tea polyphenol. Mutation Research/Fundamental and Molecular Mechanisms of Mutagenesis, 523-524, 33–41.10.1016/S0027-5107(02)00319-6
- Shin, S., Wang, L. X., Zheng, X. Q., Xiang, L. P., & Liang, Y. R. (2014). Protective effect of (-)-epigallocatechin gallate against photo-damage induced by ultraviolet a in human skin fibroblasts. Tropical Journal of Pharmaceutical Research, 13, 1079–1084.10.4314/tjpr.v13i7.10
- Siddique, Y. H., Ara, G., & Afzal, M. (2012). Estimation of lipid peroxidation induced by hydrogen peroxide in cultured human lymphocytes. Dose-Response, 10, 1–10.10.2203/dose-response.10-002.Siddique
- Singh, M., Kaur, P., Sandhir, R., & Kiran, R. (2008). Protective effects of vitamin E against atrazine-induced genotoxicity in rats. Mutation Research/Genetic Toxicology and Environmental Mutagenesis, 654, 145–149.10.1016/j.mrgentox.2008.05.010
- Singh, N. P., McCoy, M. T., Tice, R. R., & Schneider, E. L. (1988). A simple technique for quantitation of low levels of DNA damage in individual cells. Experimental Cell Research, 175, 184–191.10.1016/0014-4827(88)90265-0
- Srichairatanakool, S., Kulprachakarn, K., Pangjit, K., Pattanapanyasat, K., & Fuchaeron, S. (2012). Green tea extract and epigallocatechin 3-gallate reduced labile iron pool and protected oxidative stress in iron-loaded cultured hepatocytes. Advances in Bioscience and Biotechnology, 03, 1140.10.4236/abb.2012.38140
- Stich, H. F. (1992). Teas and tea components as inhibitors of carcinogen formation in model systems and man. Preventive Medicine, 21, 377–384.10.1016/0091-7435(92)90045-J
- Taniguchi, M., Yasutake, A., Takedomi, K., & Inoue, K. (1999). Effects of N-nitrosodimethylamine (NDMA) on the oxidative status of rat liver. Archives of Toxicology, 73, 141–146.10.1007/s002040050598
- Thangapandiyan, S., & Miltonprabu, S. (2013). Epigallocatechin gallate effectively ameliorates fluoride-induced oxidative stress and DNA damage in the liver of rats. Canadian Journal of Physiology and Pharmacology, 91, 528–537.10.1139/cjpp-2012-0347
- Verna, L., Whysner, J., & Williams, G. M. (1996). N-Nitrosodiethylamine mechanistic data and risk assessment: Bioactivation, DNA-adduct formation, mutagenicity, and tumor initiation. Pharmacology & Therapeutics, 71, 57–81.10.1016/0163-7258(96)00062-9
- Wang, Z. Y., Agarwal, R., Khan, W. A., & Mukhtar, H. (1992). Protection against benzo[ α ]pyrene- and N -nitrosodiethylamineinduced lung and forestomach tumorigenesis in A/J mice by water extracts of green tea and licorice. Carcinogenesis, 13, 1491–1494.10.1093/carcin/13.8.1491
- Wang, Z. Y., Wan, L. D., Lee, M. J., Ho, C. T., Huang, M. T., Conney, A. H., & Yang, C. S. (1995). Inhibition of N-nitrosomethylbenzylamine-induced esophageal tumorigenesis in rats by green and black tea. Carcinogenesis, 16, 2143–2148.10.1093/carcin/16.9.2143
- Yang, C. S., Lee, M. J., Chen, L., & Yang, G. Y. (1997). Polyphenols as inhibitors of carcinogenesis. Environmental Health Perspectives, 105, 971.10.1289/ehp.97105s4971
- Yang, C. S., & Wang, Z. Y. (1993). Tea and cancer. JNCI Journal of the National Cancer Institute, 85, 1038–1049.10.1093/jnci/85.13.1038
- Yao, H.T., Yang, Y. C., Chang, C. H., Yang, H. T., &Yin, M. C. (2015). Protective effects of (-)-epigallocatechin-3-gallate against acetaminophen-induced liver injury in rats). BioMedicine, 5, 16–21.
- Zhang, X., Wu, Z., & Weng, P. (2014). Antioxidant and Hepatoprotective Effect of (−)-Epigallocatechin 3- O -(3- O -Methyl) gallate (EGCG3″Me) from Chinese Oolong Tea. Journal of Agricultural and Food Chemistry, 62, 10046–10054.10.1021/jf5016335