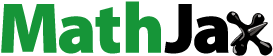
Abstract
The study demonstrates the temporal pattern of expression of melatonin bio-synthesizing enzyme genes (Tph1, Aanat1, Aanat2, and Hiomt) in the brain and gut of a tropical carp (Catla catla) on a daily and seasonal basis under natural photo-thermal conditions. The measurement of melatonin in brain and gut (both in vivo and in vitro) demonstrated a higher content in gut, with evidence of melatonin bio-synthesizing machinery in both tissues. All melatonin bio-synthesizing gene expressions in these two tissues were negatively correlated (except Aanat1 in brain and Hiomt in the gut) with the water temperature on an annual cycle. The higher expression of Aanat2 gene, rather than Aanat1, signifies the importance of Aanat2 isoform in the melatonin production in tropical carp. Furthermore, the Aanat2 and Hiomt genes are highly expressed in the gut. Analysis of the rhythm and acrophase of expression of these genes in the brain and gut imply a pineal-independent melatonin synthesizing machinery in these two organs, possibly involving both environmental and endogenous cues for the regulation of melatonin rhythm to synchronize the physiology of the animal.
Public Interest Statement
This article provides information about the melatonin bio-synthesizing machinery in the brain and gut of a tropical fish. The production of melatonin, an indole amine, is directly linked to the environmental photo-thermal conditions. This study also gives an idea about the variation and/or changes in the rhythmic expression of the melatonin bio-synthesizing genes under natural environmental conditions. Moreover, a relationship between the brain (central) and gut (peripheral) has also been focused in respect to the production of melatonin. The present finding is an opening toward the brain–gut axis and its synchronization with respect to melatonin in fish.
Competing Interests
The authors declare no competing interest.
1. Introduction
Melatonin (5-Methoxy-N-acetyltryptamine), an indole amine, is primarily synthesized in the pineal gland of vertebrates. It is also synthesized from retina, liver, gut, and brain (Chowdhury & Maitra, Citation2012; Jimenez-Jorge et al., Citation2007). Among these, the gut is the richest source of melatonin (Bubenik, Citation2002) in various groups of vertebrates, including fish (Bubenik & Brown, Citation1997; Bubenik & Pang, Citation1997; Munoz, Ceinos, Soengas, & Miguez, Citation2009). The limited data on brain melatonin in vertebrates suggest the presence of least amount of melatonin in the cerebral structures (Jimenez-Jorge et al., Citation2007; Uz, Qu, Sugaya, & Manev, Citation2002). Melatonin is formed from tryptophan by four sequential enzymatic reactions. Tryptophan hydroxylase (TPH) catalyzes the hydroxylation of tryptophan, and decarboxylation is done by aromatic amino acid decarboxylase, forming serotonin, an important neurotransmitter in the functioning of body physiology. It has already been reported that many parts of brain perform these two reactions where serotonin is formed (Appelbaum & Gothilf, Citation2006). The remaining two steps, N-acetylation by arylalkylamine N-acetyltransferase (AANAT) and O-methylation by hydroxyindole-O-methyltransferase (HIOMT), are known to occur predominantly in the pineal gland and retina, and therefore are considered as pineal-/retina-specific enzymes (Appelbaum & Gothilf, Citation2006). In teleost, the existence of three TPH isoforms (TPH1a, TPH1b, and TPH2), (Lillesaar, Citation2011), two AANAT subfamilies AANAT1 (AANAT1a and/or AANAT1b) and AANAT2 (Paulin et al., Citation2015), and two isoforms of HIOMT (Velarde, Cerda-Reverter et al., Citation2010) has been reported.
The circadian organization and the regulatory system of melatonin are shown to be dependent on environmental photo-thermal conditions resulting in a peak at midnight in the pineal gland of mammals (Reiter, Citation1991b), fish (Falcón, Migaud, Muñoz-Cueto, & Carrillo, Citation2010; Iigo et al., Citation2007), and carp (Seth & Maitra, Citation2010). In this way, melatonin acts as the internal neurohormonal signal of darkness and plays a role as a “zeitgeber” (Falcón, Citation1999; Reiter, Citation1991a; Seth & Maitra, Citation2011). The involvement of suprachiasmatic nucleus (SCN) in maintaining biological rhythms in mammals is well known (Korf, Schomerus, & Stehle, Citation1998). Studies on fish and amphibians have given the indication of involvement of brain in photo neuroendocrine system beside the pineal gland and retina (Falcón et al., Citation2010). The diencephalic cells of Rana perezi (including SCN cells) show the expression of Aanat1 (Isorna et al., Citation2006) which leads to the hypothesis that some parts of the brain can concentrate photosensitivity (Falcón et al., Citation2010). However, pinealectomized (brain only) rat demonstrated the reduction of brain melatonin (Jimenez-Jorge et al., Citation2007). It has also been evidenced that the developing brain (without pineal gland) of rat can produce its own melatonin rhythmically with higher nocturnal content than the diurnal concentration (Jimenez-Jorge et al., Citation2007). The study on zebrafish showed that circadian rhythms can be initiated and maintained in the absence of SCN and other tissues in the ventral brain, though the SCN may play a deciding role in the regulation of amplitude of rhythms in the absence of environmental cues (Noche, Lu, Goldstein-Kral, Glasgow, & Liang, Citation2011). Moreover, a recent study on zebrafish also demonstrated the expression pattern of melatonin bio-synthesizing enzymes in the whole brain (Khan et al., Citation2016) in different photic conditions.
The presence of melatonin in the gut of many vertebrates, including fish, is reported (Bubenik & Brown, Citation1997; Kulczykowska, Kalamarz, Warne, & Balment, Citation2006). Based on the high expression levels of its synthesizing enzymes, some studies pointed out its local synthesis in the gut (Fernández-Durán et al., Citation2007; Stefulj et al., Citation2001). The study on goldfish informed us of the gAanat2 mRNA expression in both foregut and hindgut (Velarde, Cerda-Reverter et al., Citation2010). Rhythmic AANAT activity is also reported in the foregut of the same fish (Nisembaum et al., Citation2013). A recent study on trout also demonstrated a variation in melatonin content and the expression of Aanat1, Aanat2, and Hiomt in the mucosa and wall of the gut (Munoz-Perez et al., Citation2016). Stable presence of melatonin in the lower gut in a pinealectomized animal is indicative of local production of this indole amine (Chen, Fichna, Bashashati, Li, & Storr, Citation2011). Melatonin level in gut showed a significant difference at the species level in fish, amphibians, and reptiles (Bubenik & Pang, Citation1997). Recently, a study on the seasonal and diurnal profiles of melatonin (serum, gut, retinal, and pineal) and expression of AANAT in natural and altered photic conditions in same species gave an idea about the presence of gut melatonin in any tropical carp (Mukherjee & Maitra, Citation2015; Mukherjee, Moniruzzaman, & Maitra, Citation2014). In fish, there is no difference in the melatonin content in various parts of the gut (Mukherjee & Maitra, Citation2015). The study on aged mice depicted the protective role of melatonin and its precursor serotonin in the gut (Bertrand, Bertrand, Camello, & Pozo, Citation2010). Presence of melatonin in the hepatobiliary–gastrointestinal tract (Messner, Huether, Lorf, Ramadori, & Schwörer, Citation2001) may contribute to reduce pathophysiology in humans (Reiter, Tan, Manchester, & Gitto, Citation2010) and gut motility in fish through its receptor or bio-synthesizing enzyme (AANAT) (Nisembaum et al., Citation2013; Velarde, Delgado, & Alonso-Gómez, Citation2010).
Information about the expression of melatonin bio-synthesizing enzyme genes and its temporal pattern of expression at a transcriptional level along with the concentration, in brain and gut, is not available. Therefore, the present study is the first attempt to show diurnal and seasonal variations and/or rhythms of melatonin bio-synthesizing enzyme genes (Tph1, Aanat1, Aanat2, and Hiomt) at the transcriptional level in brain (without pineal) and gut in any tropical carp (Catla catla), under natural photo-thermal conditions. Moreover, measurement of melatonin in vivo and in culture provided the evidence of the melatonin production unit in these two organs.
2. Materials and methods
2.1. Animals, housing conditions, and sample collection
An economically important surface-dwelling adult major carp of both sexes of Indian subcontinent, C. catla (n = 3) weighing between 700 and 900 g was used for the sampling in this study continuously for three years. The fish were collected from local fish farm located in Kodompokpi, Imphal. After transportation to the laboratory, the fish were acclimatized in cemented tanks in identical photo-thermal conditions, as they were, for a week (Chattoraj, Seth, & Maitra, Citation2009). Artificially prepared balanced food comprising 35% fish meal, 28% mustard oil cake, 28% rice bran, 2% each sunflower and cod liver oils, 5% carboxy methyl cellulose, and multivitamin–multimineral tablets (“Becozyme Forte”, Glaxo India Ltd., 25 tabs/kg food) were given two times daily ad libitum. Laboratory care of fish and adopted study schedules were in agreement with ethical standards journal (Portaluppi, Smolensky, & Touitou, Citation2010). The ethical clearance was obtained from the Institutional Animals Ethical Committee constituted as per the recommendations of Committee for the Purpose of Control and Supervision of Experiments on Animals (CPCSEA), Government of India. Samples were collected in preparatory, pre-spawning, spawning, and post-spawning phases in Imphal valley (latitude 24°44′N, longitude 93°58′E), Manipur, with an elevation of 786 m above sea level. This region of the world is under the Indo–Burma biodiversity hotspot. The area is having a seasonal, tropical monsoonal climate, with a cool dry season (~November–April) and warm wet season (~May–October). From winter to summer, the temperature varies from 4 to 32°C and the photoperiod ranges from 11 to 13 h in a year. Animals were anesthetized (0.1% Tricaine, SIGMA; pH 7.00 of the media) and euthanized before tissues were collected. Samplings at dark were carried out in dim red light. The brain tissues without the end vesicle of pineal and whole gut were collected. The previous studies on the morphoanatomy of this carp clearly demonstrated that the end vesicle part of pineal organ is mainly considered as the unit for photoreception and production of melatonin. This end vesicle is very much distant from the dorsal sac and connected by a long pineal stalk (Figure 1 of Dey, Bhattacharya, Maitra, & Banerji, Citation2003 and Figure 2 of Chattoraj, Bhattacharyya, Dey, Seth, & Maitra, Citation2006). The gut content was removed from whole gut tissues prior to storage in −80°C freezer. Both the tissues were collected in an identical method and time points (8:00, 12:00, 16:00, 20:00, 24:00, 4:00 h) in preparatory (January; duration of Natural Photoperiod, NP: 11 h 18 min; Water Temperature, WT: maximum 15°C, minimum 14°C), pre-spawning (April; NP: 13 h; WT: maximum 28.3°C, minimum 26.3°C), spawning (August; NP: 13 h; WT: maximum 26°C, minimum 25°C), and post-spawning phases (October; NP: 10 h 50 min; WT: maximum 18.5°C, minimum 17°C) (Table ). There was no change in the feeding conditions and timing before the euthanization of fish. Each phase is designated by characterized profile of germ cells in the gonad; serum melatonin varies in its amplitude and pattern (Bhattacharya, Chattoraj, & Maitra, Citation2007; Dey, Bhattacharya, & Maitra, Citation2005; Hasan, Moniruzzaman, & Maitra, Citation2014; Maitra & Chattoraj, Citation2007) in the mentioned period. Immediately after isolation, tissues were put in TRIzol and frozen at −80°C until further processing.
Table 1. The average day length and water temperature in four seasons from where fish were collected (latitude 24°44′N, longitude 93°58′E)
2.2. Gene expression study
Gene-specific primers were used for quantitative PCR, listed in Table . Total RNA was isolated using “PureLink® RNA Mini Kit” (Ambion) as recommended by manufacturer’s protocol. RNA quality and quantity were assessed using a spectrophotometer (BioSpec-nano, Shimadzu). RNA integrity was checked by taking 200 ng of total RNA loaded onto 1% denaturing agarose gel electrophoresis, stained with GelRed™ Nucleic Acid Gel Stain (Biotium Inc, USA). Genomic DNA contamination was removed by treating 10 μg of total RNA with “TURBO DNA-free™ Kit (Ambion)”. Using “High Capacity cDNA Reverse Transcription Kit” (Applied Biosystems), 2 μg DNase-treated total RNA was reverse transcribed into cDNA following manufacturer’s protocol in “ProFlex™ PCR System” (Applied Biosystems). The thermal cycling conditions for cDNA synthesis were 25°C for 10 min, followed by 37°C for 2 h, 85°C for 5 min, and final incubation at 4°C. Furthermore, the cDNA was diluted five times for downstream process. This cDNA was amplified using Tph1, Aanat1, Aanat2, Hiomt, and β-actin primers followed by clean up using QIAquick PCR Purification Kit (Qiagen) and quantified. A serial dilution of concentration ranges from 0.001 to 100 pg/μl was made and used as template to generate a standard curve. Quantitative PCR was performed in “StepOnePlus Real-Time PCR System” (Applied Biosystems) using GoTaq® qPCR Master Mix (Promega) following manufacturer-recommended protocol. The PCR cycling conditions were as follows: 95°C for 10 min followed by 40 cycles of 95°C for 15 s, and 60°C for 1 min. Primers were blast against NCBI Primer blast tool to check specificity. Amplified products were also assessed in 2% agarose gel to check whether single amplicon was amplified or not. No template control and reverse transcriptase negative control were setup by replacing cDNA with water and DNase-treated total RNA, respectively. After quantitative PCR amplification, standard curve was prepared and sample quantification was done. The quantified amount was then normalized (Piesiewicz, Kedzierska, Turkowska, Adamska, & Majewski, Citation2015) using β-actin (Kumari, Pathakota, Annam, Kumar, & Krishna, Citation2015; Munoz-Perez et al., Citation2016) and the gene expression level was represented as the number of mRNA copies per 106 copies of β-actin mRNA. The quantified concentrations using standard curve were converted into copy number using the following equation (Whelan, Russell, & Whelan, Citation2003),
Table 2. Details about primer sequences used for RT-qPCR for Tph1, Aanat1, Aanat2, and Hiomt in the brain and gut of Catla catla
2.3. Brain and gut tissue culture and measurement of melatonin
Adult C. catla (n = 3) of similar weight were taken. After euthanization, brain and gut tissues were taken out from the fish at 08.00 h (light phase) and 20.00 h (dark phase). Each tissue was incubated in culture medium (M199, SIGMA) for four hours (up to 12.00 h and 24.00 h respectively) and kept immediately at −80°C for further melatonin measurement. The tissues were maintained with 95% O2/5% CO2 at 28°C in a CO2 Incubator (Thermo Scientific). The incubator was fitted with a florescent lamp (300 lux) with automated timer (12 h light: 12 h Dark). The light was on at 05.00 h and off at 17.00 h. The M199 medium was supplemented with 10 mm HEPES, 0.1% BSA, 0.1 mM phenyl-methyl-sulfonyl fluoride (PMSF), penicillin (100 U/ml), and Streptomycin (100 μg/ml) (Chattoraj et al., Citation2005; Chowdhury et al., Citation2010; Khan et al., Citation2016). For in vivo melatonin estimation, each tissue was collected in phosphate buffer (PBS; pH 7.4) at 08.00 and 20.00 h from adult fish. Utmost care was given to the identical body weight of the fish for either in vitro or in vivo assay. For both in vivo and in vitro measurements of melatonin, 0.3 g of the homogenized tissues were taken in 500 μl of PBS (pH 7.4) and sonicated followed by centrifugation at 3000 rpm until a clear supernatant was obtained. Finally, 50 μl of the supernatant was taken for the measurement of melatonin by a commercially available ELISA kit (Gen Asia Biotech Co., Ltd, China) according to the manufacturer’s protocol. The assay was measured at an absorbance of 450 nm wavelength (Thermo, Multiskan spectrum).
2.4. Statistical analysis
Gene expressions shown here are in copy number per 106 copies of β-actin. All statistical analyses were performed in Statgraphics Centurion XVI (Statpoint Inc, USA). Seasonal change in expression was done by comparing gene expression level of the sample taken at three different time points during light and dark phases of different seasons. One-way ANOVA followed by Tukey HSD or Kruskal–Wallis test followed by Bonferroni (if the data-set doesn’t follow normal distribution even after log transformation) was performed to analyze these seasonal significant changes. Normal distribution of data was assisted using Shapiro–Wilk test.
Diurnal rhythmic changes in expression of these genes were done using COSINOR PERIODOGRAM Version 3.1 (Refinetti, Cornélissen, & Halberg, Citation2007) based on cosinor-rhythmometry (Nelson, Tong, Lee, & Halberg, Citation1979). A nonlinear regression curve was fitted to the data using the formula “Y = Mesor + Amplitude Cos (Frequency X + Acrophase)” with GraphPad Prism (Version 6.01, GraphPad Software, Inc., USA). Two-way ANOVA followed by Student–Newman–Keuls was performed to analyze significant changes in the melatonin production in vitro and in vivo conditions. All statistical analyses were conducted at 0.05 level of significance.
3. Result
3.1. Expression of Tph1 in brain and gut
In the brain, the average transcripts of Tph1 in all seasons were approximately 125 (Figure (A)). The highest expression of Tph1 in the brain was found in post-spawning while lowest was during the preparatory dark phase (Figure (A)). Significant day–night variation in the expression of Tph1 was only found during preparatory and pre-spawning phases (Kruskal–Wallis Test statistic = 17.9, p-value = 0.01; Figure (A)). The Cosinor analysis also revealed the insignificant diurnal rhythm of brain Tph1 transcript (Figure ). Highest rhythm percentage was detected in preparatory followed by pre-spawning, post-spawning, and spawning phases (Figure ). This change is inverse according to the changes in the water temperature (Tables and ).
Figure 1. Seasonal and light–dark phase changes in the expression level of (A) Tph1 in Brain, (B) Tph1 in Gut and (C) Aanat1 in Brain, (D) Aanat1 in Gut were compared by taking the mean value of three time points during the light phase (represented by empty circle) and the dark phase (represented by filled circle).
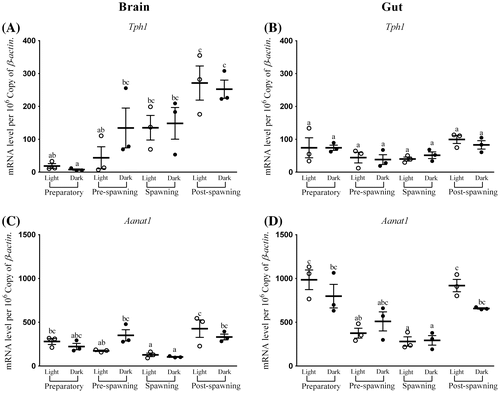
Figure 2. Seasonal and light–dark phase changes in the expression level of (A) Aanat2 in Brain, (B) Aanat2 in Gut and (C) Hiomt in Brain, (D) Hiomt in Gut were compared by taking the mean value of three time points during the light phase (represented by empty circle) and the dark phase (represented by filled circle).
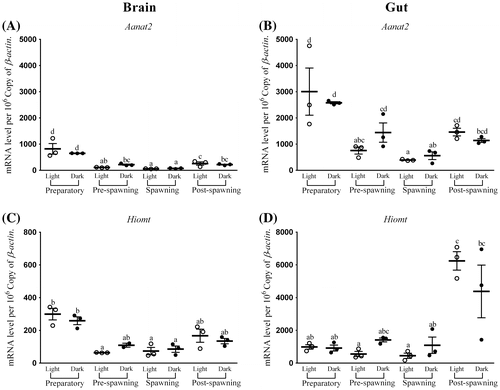
Figure 3. Changes in the expression of Tph1 in six different time points (8, 12, 16, 20, 24, and 4 h) in different seasons (n = 3) were fitted to nonlinear regression curve using the formula “Y = Mesor + Amplitude Cos (Frequency X + Acrophase)”.
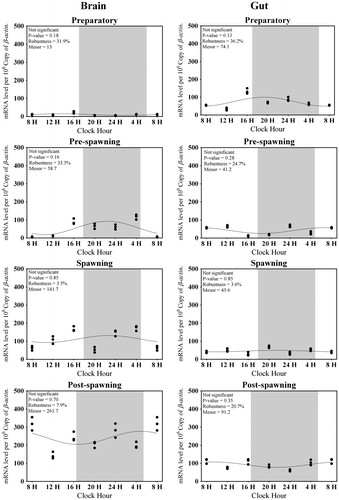
Table 3. Correlations study between water temperature and expression level
The average Tph1 transcripts in the gut in all four seasons were observed to be around 60 (Figure (B)). One-way ANOVA followed by Tukey HSD test and Cosinor analysis displayed insignificant changes in the expression of Tph1 in the gut in all seasons as well as in the light–dark phases (F-ratio = 1.9, p-value = 0.14; Figure (B)). Like the brain, Cosinor analysis also portrayed insignificant diurnal rhythm of gut Tph1 transcript in all the phases (Figure ). Percentage of rhythm was lowest in spawning and then started to increase as it moved to post-spawning and became highest at preparatory; afterward, it started to fall in pre-spawning (Figure ). This phenomenon was also negative with the water temperature or photic length (Table ).
3.2. Expression of Aanat1 in the brain and gut
Average brain Aanat1 transcripts were approximately 250 in all the seasons (Figure (C)). The highest expression level of brain Aanat1 was during the day time of post-spawning and lowest in the spawning phase in the brain (Figure (C)). There was a significant change in the expression level of Aanat1 in the brain between light and dark (F-ratio = 10.1, p-value < 0.01; Figure (C)) phases in all seasons, except spawning. Significant rhythm percentage was found in all the seasons, except post-spawning (the lowest) with the highest value in the preparatory phase (Figure ). It is interesting that the acrophase of expression was shifted from late light phase to early light phase (spawning to preparatory phases) and finally moved to dark in the pre-spawning phase (Figure ).
Figure 4. Changes in the expression of Aanat1 in six different time points (8, 12, 16, 20, 24, and 4 h) in different seasons (n = 3) were fitted to nonlinear regression curve using the formula “Y = Mesor + Amplitude Cos (Frequency X + Acrophase)”.
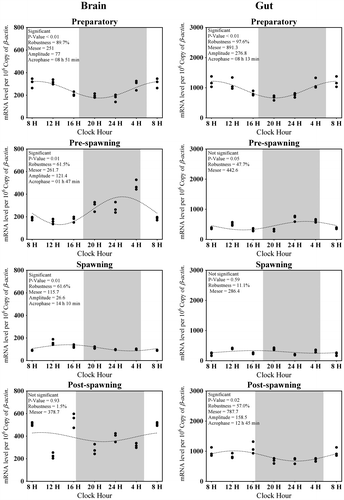
The average Aanat1 transcript level detected in the gut was around 600 in all seasons (Figure (D)). The highest Aanat1 expression in the gut was displayed during the daytime of preparatory and post-spawning phases and lowest during the spawning phase (F-ratio = 10.5, p-value < 0.01; Figure (D)). Except the spawning phase, a day–night variation was observed in the expression of gut Aanat1 in all phases (Figure (D)). A significant rhythmic expression of gut Aanat1 was observed in preparatory and post-spawning phases (Figure ). There was an increase in the percentage of rhythm in the expression of gut Aanat1 from spawning to preparatory phases and decreased at the pre-spawning phase (Figure ).
3.3. Expression of Aanat2 in brain and gut
Brain Aanat2 transcripts, on an average in four seasons, were about 300 (Figure (A)). There was no significant variation observed in the expression of brain Aanat2 between light and dark in preparatory (highest) and spawning phases (lowest) (Figure (A)). The other two phases exhibited a day–night variation (F-ratio = 39.4, p-value < 0.01; Figure (A)). A significant rhythm during pre-spawning and post-spawning phases (highest) (Figure ) was found. Except preparatory, the acrophase of brain Aanat2 was found in dark (Figure ).
Figure 5. Changes in the expression of Aanat2 in six different time points (8, 12, 16, 20, 24, and 4 h) in different seasons (n = 3) were fitted to nonlinear regression curve using the formula “Y = Mesor + Amplitude Cos (Frequency X + Acrophase)”.
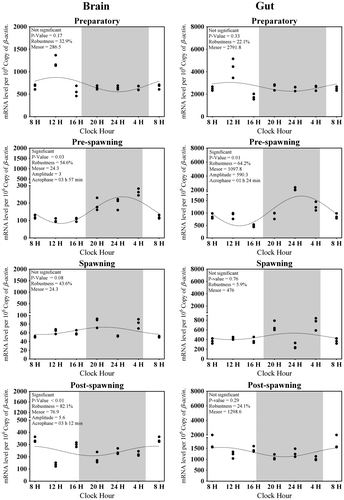
Highest Aanat2 expression in the gut was observed during the preparatory phase while lowest was during spawning (F-ratio = 13, p-value < 0.01; Figure (B)) and its overall average transcripts in four seasons were approximately 1,415 (Figure (B)). Except the preparatory phase, there was a significant difference in the expression of gut Aanat2 in other three phases, amongst which pre-spawning and spawning phases were giving the highest value in dark (Figure (B)). The rhythm percentage of Aanat2 in gut gradually increased from spawning to preparatory and ultimately became significantly rhythmic in pre-spawning phases (Figure ). Expression of Aanat1 and Aanat2 in both organs was negatively related to the environmental photo-thermal conditions (Tables and ).
It has also been revealed that any one of the isoforms of Aanat was rhythmic in their expression in a particular season and in a particular organ, except in the spawning season in the gut (Figures and ) where both isoforms were arrhythmic in their expression. Moreover, except the post-spawning phase, there was almost an identical acrophase of Aanat1 and Aanat2 in both brain and gut (Figures and ) in all the phases.
3.4. Expression of Hiomt in the brain and gut
The average transcripts of Hiomt mRNA in the brain were around 160 (Figure (C)). The level of expression was highest in the preparatory phase and the light phase of pre-spawning was with the lowest value (Figure (C)). There was no significant difference in the expression of brain Hiomt in all phases except pre-spawning (F-ratio = 9.3, p-value < 0.01; Figure (C)). The highest rhythm was observed only in the pre-spawning phase (Figure ).
Figure 6. Changes in the expression of Hiomt in six different time points (8, 12, 16, 20, 24, and 4 h) in different seasons (n = 3) were fitted to nonlinear regression curve using the formula “Y = Mesor + Amplitude Cos (Frequency X + Acrophase)”.
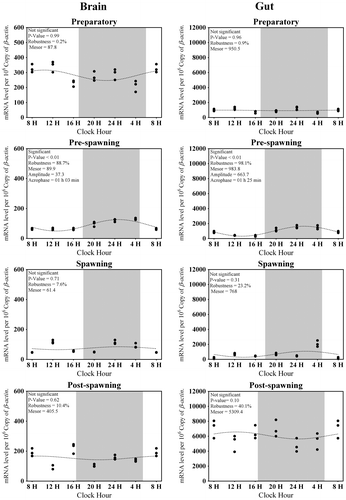
In all four seasons, the average gut Hiomt mRNA transcripts were approximately 2,000 (Figure (D)). The expressivity of gut Hiomt was almost similar in all seasons with no or very less significant differences between light and dark phases, but at the post-spawning phase, suddenly, the overall expression was elevated (F-ratio = 9.4, p-value < 0.01; Figure (D)). Like the brain, the expression of Hiomt gave a significant rhythm only during the pre-spawning phase (Figure ). This was also following a negative trend of relation with environmental photo-thermal conditions (Tables and ).
3.5. Correlation between water temperature and melatonin synthesizing enzyme genes
A significant negative correlation was found between the expression of melatonin synthesizing enzyme genes and water temperature from the correlation co-efficient analysis (Table ) in all four seasons in both brain and gut, except for Aanat1 (brain) and Hiomt (gut). In brain, the expression of Tph1 in the preparatory phase did not reveal a negative relationship with the water temperature (Table ).
3.6. Measurement of melatonin in vivo and in vitro
The brain melatonin level was found to have significant differences between the light and dark phases as well as between in vivo and in vitro as revealed by two-way ANOVA (in vivo/in vitro F-ratio = 8.6, p-value = 0.03; light/dark F-ratio = 16.5, p-value < 0.01). In vitro melatonin level was found to be more as compared to in vivo in both light and dark conditions (Figure (A)).
Figure 7. In vivo and in vitro melatonin synthesis in (A) Brain and (B) Gut tissues was analyzed by two-way analysis of variance followed by Student–Newman–Keuls.
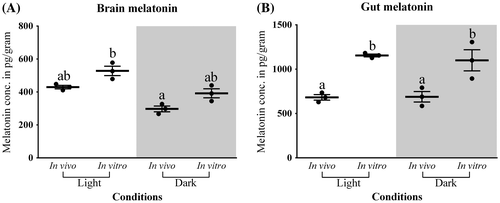
The gut melatonin level does not show any significant variation between light and dark phases in both in vivo and in vitro but the in vitro level was found to be higher than in vivo (in vivo/in vitro F-ratio = 41.5, p-value < 0.01; light/dark F-ratio = 0.1, p-value = 0.67; Figure (B)). Moreover, a considerable amount of melatonin was also detected during the light phase in both the tissues (Figures (A) and (B)).
4. Discussion
The present communication demonstrates the presence of melatonin bio-synthesizing machinery both in adult brain and gut of any tropical carp with a daily and seasonal variation at the transcript level. Our data also validate the production of this indole amine both in vivo and in vitro cultures in these two organs. The variation in the mRNA of melatonin-synthesizing genes according to the environmental cues indicates the importance of brain and gut melatonin in the maintenance of annual physiology of this tropical carp.
4.1. Expression of Tph1 and Hiomt in brain and gut
Tissue-specific expression of Tph has been reported in zebrafish; Tph1 (a and b) is involved mainly in the areas of photoreception, phototransduction, and production of melatonin (Bellipanni, Rink, & Bally-Cuif, Citation2002). The cloning, sequencing, and phylogenetic analyses of Tph and Hiomt from pineal gland and retina in C. catla (unpublished data from our group) identified them as Tph1 and Hiomt, respectively, unlike goldfish g-Hiomt-1 and g-Hiomt-2 (Velarde, Cerda-Reverter et al., Citation2010).
The mRNA of Tph1 in both brain and gut is giving a significant negative correlation with the water temperature (Table ) and photoperiod (data not shown) in its expression. It is noteworthy that the preparatory phase expression of Tph1 in the brain is not giving the same trend with photo-thermal conditions as it is with other genes. On the other hand, the level of expression of Tph1 is very less in gut, though it has a negative correlation with the photo-thermal conditions. This low level of Tph1 and comparatively higher levels of other melatonin synthesizing genes as well as higher melatonin content in gut (Figures (A), (B) and ) in comparison to brain may be due to the possible existence of another source of serotonin in the gut as gut serotonin is an important signaling molecule that helps in regulating the gastrointestinal functions (Gershon & Tack, Citation2007). More serotonin in the brain is required for neurotransmission (Sugden, Citation2003). The tissue-specific distribution of Tph1 revealed a weak and arrhythmic circadian expression in rat brain (Malek, Dardente, Pevet, & Raison, Citation2005), which is in support of the present finding (Figure (A)). This pattern of expression is also supporting the arrhythmic production of a trace amount of melatonin in the brain, as reported in the rat (Jimenez-Jorge et al., Citation2007). Further, the present study also reveals the presence of melatonin in both brain and gut at light phase, signifying a pineal-independent mechanism of expression of this gene in both brain and gut as suggested from the AANAT activity in rat brain (Jimenez-Jorge et al., Citation2007) and study on carp (Seth & Maitra, Citation2010).
On the other hand, the level of expression of Hiomt is very less in the brain as compared to gut (Figures (C) and (D)). The expression of Hiomt in gut portrays an insignificant negative trend of correlation with water temperature, though in the brain it is significant (Table ). The rich melatonin content in fish gut found in this study (Figure ) is also supportive of previous findings (Mukherjee & Maitra, Citation2015), and may be explained with the ample copy number of Hiomt mRNA, observed in this study. A recent study on rainbow trout is also showing a high transcription of Hiomt in gut (Muñoz-Pérez et al., Citation2016). This particular observation may be linked to the notion that Hiomt acts as the key regulator in melatonin production (Liu & Borjigin, Citation2005). A lack of correlation of the expression of Hiomt in the gut with environmental factors (Table ) also indicates a non-photo-thermal regulation of melatonin production (Mukherjee & Maitra, Citation2015; Velarde et al., Citation2009; Velarde, Delgado et al., Citation2010) in gut. Moreover, high expression of Hiomt in brain (Figures (C) and ) during preparatory (phase of preparation for reproduction) is a sign of involvement of brain melatonin in the modulation of seasonal reproductive parameters through hypothalamo–pituitary–gonadal axis (Chattoraj et al., Citation2005; Falcón, Besseau, Sauzet, & Boeuf, Citation2007). Earlier studies on the same fish also demonstrated the role of environmental photo-thermal conditions with the development of gonads through melatonin (Bhattacharya et al., Citation2007; Dey et al., Citation2005; Maitra, Chattoraj, Mukherjee, & Moniruzzaman, Citation2013).
4.2. Expression of Aanat1 and Aanat2 in brain and gut
This is the first evidence for the presence of both isoforms of Aanat transcripts (Aanat1 and Aanat2) in brain and gut in any tropical fish. Study on rainbow trout recently showed the transcription of Aanat1 and Aanat2 in gut (Muñoz-Pérez et al., Citation2016). The expression of Aanat mRNA has been demonstrated in various parts of the rat brain (Uz et al., Citation2002). The present study denotes an inverse relationship of the expression of Aanat1 and Aanat2 in both brain and gut with the environmental photo-thermal conditions in all phases, though in brain, Aanat1 gave an insignificant value (Table ). Involvement of environmental cues in the regulation of these enzymes is valid for both brain and gut in fish as evidenced from previous studies (Falcón et al., Citation2010). In both tissues, the expressivity of Aanat2 is more than Aanat1, signifying the importance of Aanat2 in the regulation of melatonin synthesis in carp (Figures (C) and (D), (A) and (B)). Presence of Val115 and Val161 is important for the temperature-dependent activity of AANAT2 (Cazamea-Catalan et al., Citation2013). This indicates AANAT2 might play a role in temperature regulation of melatonin bio-synthesis. Higher expression of Aanat2 in the gut is also proportionate to the copy number of Hiomt and higher content of melatonin in the same tissue (Figures (B) and (D); Figure ). Interestingly, in the brain, in every season, any one of the isoforms is rhythmic in their expression, but during the spawning phase, Aanat expression became arrhythmic (Figures and ). Similar kinds of observations are available in pike, where Aanat1 is giving a peak at light in retina and Aanat2 in dark in pineal (Coon, Begay, Deurloo, Falcon, & Klein, Citation1999), which may be explained through the proteasomal proteolysis of AANAT by light at the downstream of the clock (Gastel, Roseboom, Rinaldi, Weller, & Klein, Citation1998). The current finding demonstrates the alternation (season wise) of Aanat1 and Aanat2 rhythmicity and this further explains the probable reasons to have two isoforms of Aanat in fish. Both isoforms cannot remain rhythmic in same photo-thermal conditions in both brain and gut, except in the pre-spawning of the brain (Figures and ) which might be due to the difference in their optimum photo-thermal conditions. This mechanism may help the system react immediately to the length of photoperiod which cannot be anticipated by the endogenous clock (Coon et al., Citation1999). Insignificant variation of melatonin content between light and dark phases (Figure ) suggests more involvement of endogenous cues in the regulation of melatonin synthesis in gut. The regulation of expression of Aanat through external cues (Table ) supports the hypothesis of its chronobiological importance in the central nervous system (Uz et al., Citation2002). Study on the melatonin synthesizing machinery in the gut of rainbow trout also indicated a control by environmental light cycle and is likely to be under circadian regulation (Muñoz-Pérez et al., Citation2016).
Moreover, findings on goldfish (Velarde et al., Citation2009; Velarde, Cerda-Reverter et al., Citation2010) and mammals (Konturek, Brzozowski, & Konturek, Citation2011) suggest that the rhythm of Aanat2 and Hiomt expression and melatonin production can be regulated by peripheral clocks entrained by non-photic cues (Liu & Borjigin, Citation2005). On the contrary, very recent studies indicate the synchronization of physiological rhythm in various parts of the body or even in the microbial population of the gut (Paulose, Wright, Patel, & Cassone, Citation2016) may be harmonized by melatonin. Further, these physiological processes of entrainment and/or synchronization are influenced by seasonal changes (Falcón et al., Citation2010; Maitra et al., Citation2013).
The recent study from our group has demonstrated that the synchronization of melatonin synthesizing genes and clock-associated genes in central (brain) and peripheral organs (ovary) of zebra fish is dependent on environmental photic conditions (Khan et al., Citation2016), supporting the “orchestra” model (Richards & Gumz, Citation2012). It was demonstrated earlier that the self-sustaining circadian oscillators exist in several peripheral organs in fish (Whitmore, Foulkes, Strahle, & Sassone-Corsi, Citation1998). The present work indicates a seasonal and diurnal variation of four major melatonin synthesizing enzyme genes in an annual cycle of a tropical carp in brain and gut. It is evident from the statistical analysis that the rhythm of transcription of all these genes is mostly insignificant in daily basis, but an overall variation can be seen depending on the photo-thermal conditions. Future study involving alteration of photo-thermal setting and pattern of expression of clock-associated genes will clarify the synchronization of melatonin synthesizing machinery in brain (central) and gut (peripheral) in this tropical carp.
Funding
The work was supported by IBSD (PhD JRF Programme); Department of Biotechnology, Ministry of Science and Technology [grant number BT/407/NE/U-Excel/2013]; CSIR-JRF Programme [grant number (09/1044(0002)/2012-EMR-I(14/11/2012)]; Science and Engineering Research Board [grant number SR/FT/LS-179/2010].
Acknowledgments
Authors are grateful to the Director, IBSD India, We are thankful to Mr L. Umakanta Singh, Mr S. Surjit Singh, Mr W. Rahul, and Mr H. Bishorjit for their continuous support in the collection and maintenance of fish in laboratory conditions. Our sincere thanks to all of our colleagues of Fish Biology and Insect Biology Laboratories for their kind cooperations during the study. Our sincere gratitude to every Indian tax payer for their contribution to the funding for research. The authors are really grateful to three distinguished reviewers for their valuable comments and suggestions. The IBSD, Imphal manuscript number is 2015008.
Additional information
Notes on contributors
Haobijam Sanjita Devi
The research group led by Asamanja Chattoraj is mainly focused on the synchronization of the environmental cues with body physiology in relation to the “chronobiotic” molecule melatonin and clock-associated genes. The abiotic parameters of the nature are changing abruptly; fish are the best model to study the variation and possible reason in the alteration of body physiology. The research interest concerns about the relationship between the central and peripheral clock mechanisms and their orientation either through “master-slave” or “orchestra”. The findings can lead to develop a sustainable strategy for the maintenance of the species population in a favorable niche as well as tools for their conservation. Moreover, several lifestyle diseases are emerging from the circadian rhythm disruption. We are trying to address this inappropriate harmonization between environmental cues and body functioning with special emphasis on clock-associated genes and “melatonin” using fish as model.
References
- Appelbaum, L., & Gothilf, Y. (2006). Mechanism of pineal-specific gene expression: The role of E-box and photoreceptor conserved elements. Molecular and Cellular Endocrinology, 252, 27–33. doi:10.1016/j.mce.2006.03.021
- Bellipanni, G., Rink, E., & Bally-Cuif, L. (2002). Cloning of two tryptophan hydroxylase genes expressed in the diencephalon of the developing zebrafish brain. Mechanisms of Development, 119, S215–S220.10.1016/S0925-4773(03)00119-9
- Bertrand, P. P., Bertrand, R. L., Camello, P. J., & Pozo, M. J. (2010). Simultaneous measurement of serotonin and melatonin from the intestine of old mice: The effects of daily melatonin supplementation. Journal of Pineal Research, 49, 23–34. doi:10.1111/j.1600-079X.2010.00760.x
- Bhattacharya, S., Chattoraj, A., & Maitra, S. K. (2007). Melatonin in the regulation of annual testicular events in carp Catla catla : Evidence from the studies on the effects of exogenous melatonin, continuous light, and continuous darkness. Chronobiology International, 24, 629–650. doi:10.1080/07420520701534665
- Bubenik, G. A. (2002). Gastrointestinal melatonin: Localization, function, and clinical relevance. Digestive Diseases and Sciences, 47, 2336–2348.10.1023/A:1020107915919
- Bubenik, G. A., & Brown, G. M. (1997). Pinealectomy reduces melatonin levels in the serum but not in the gastrointestinal tract of rats. Neurosignals, 6, 40–44.10.1159/000109107
- Bubenik, G. A., & Pang, S. F. (1997). Melatonin levels in the gastrointestinal tissues of fish, amphibians, and a reptile. General and Comparative Endocrinology, 106, 415–419. doi:10.1006/gcen.1997.6889
- Cazamea-Catalan, D., Magnanou, E., Helland, R., Besseau, L., Boeuf, G., Falcon, J., & Jorgensen, E. H. (2013). Unique arylalkylamine N-acetyltransferase-2 polymorphism in Salmonids and profound variations in thermal stability and catalytic efficiency conferred by two residues. The Journal of experimental biology. doi:10.1242/jeb.080960
- Chattoraj, A., Bhattacharyya, S., Basu, D., Bhattacharya, S., Bhattacharya, S., & Maitra, S. K. (2005). Melatonin accelerates maturation inducing hormone (MIH): Induced oocyte maturation in carps. General and Comparative Endocrinology, 140, 145–155. doi:10.1016/j.ygcen.2004.10.013
- Chattoraj, A., Bhattacharyya, S., Dey, R., Seth, M., & Maitra, S. K. (2006). The pineal organ and reproduction in fish. Proceedings of the Zoological Society (Calcutta), 59, 35–60.
- Chattoraj, A., Seth, M., & Maitra, S. K. (2009). Localization and dynamics of Mel1a melatonin receptor in the ovary of carp Catla catla in relation to serum melatonin levels. Comparative Biochemistry and Physiology Part A: Molecular & Integrative Physiology, 152, 327–333. doi:10.1016/j.cbpa.2008.11.010
- Chen, C. Q., Fichna, J., Bashashati, M., Li, Y. Y., & Storr, M. (2011). Distribution, function and physiological role of melatonin in the lower gut. World Journal of Gastroenterology, 17, 3888–3898. doi:10.3748/wjg.v17.i34.3888
- Chowdhury, I., & Maitra, S. K. (2012). Melatonin in the promotion of health. In W. RR (Ed.), Melatonin time line: From discovery to therapy (pp. 1–60). Boca Raton, FL: Taylor and Francis.
- Chowdhury, V. S., Yamamoto, K., Ubuka, T., Bentley, G. E., Hattori, A., & Tsutsui, K. (2010). Melatonin stimulates the release of gonadotropin-inhibitory hormone by the avian hypothalamus. Endocrinology, 151, 271–280. doi:10.1210/en.2009-0908
- Coon, S. L., Begay, V., Deurloo, D., Falcon, J., & Klein, D. C. (1999). Two arylalkylamine N-acetyltransferase genes mediate melatonin synthesis in fish. Journal of Biological Chemistry, 274, 9076–9082.10.1074/jbc.274.13.9076
- Dey, R., Bhattacharya, S., & Maitra, S. K. (2005). Importance of photoperiods in the regulation of ovarian activities in indian major carp Catla catla in an annual cycle. Journal of Biological Rhythms, 20, 145–158. doi:10.1177/0748730404272925
- Dey, R., Bhattacharya, S., Maitra, S. K., & Banerji, T. K. (2003). The morpho‐anatomy and histology of the pineal complex in a major indian carp, Catla catla : Identification of the pineal photoreceptor cells and their responsiveness to constant light and constant darkness during different phases of the annual reproductive cycle. Endocrine Research, 29, 429–443.10.1081/ERC-120026949
- Falcón, J. (1999). Cellular circadian clocks in the pineal. Progress in Neurobiology, 58, 121–162.10.1016/S0301-0082(98)00078-1
- Falcón, J., Besseau, L., Sauzet, S., & Boeuf, G. (2007). Melatonin effects on the hypothalamo-pituitary axis in fish. Trends in Endocrinology & Metabolism, 18, 81–88. doi:10.1016/j.tem.2007.01.002
- Falcón, J., Migaud, H., Muñoz-Cueto, J. A., & Carrillo, M. (2010). Current knowledge on the melatonin system in teleost fish. General and Comparative Endocrinology, 165, 469–482. doi:10.1016/j.ygcen.2009.04.026
- Fernández-Durán, B., Ruibal, C., Polakof, S., Ceinos, R. M., Soengas, J. L., & Míguez, J. M. (2007). Evidence for arylalkylamine N-acetyltransferase (AANAT2) expression in rainbow trout peripheral tissues with emphasis in the gastrointestinal tract. General and Comparative Endocrinology, 152, 289–294. doi:10.1016/j.ygcen.2006.12.008
- Gastel, J. A., Roseboom, P. H., Rinaldi, P. A., Weller, J. L., & Klein, D. C. (1998). Melatonin production: Proteasomal proteolysis in serotonin N-acetyltransferase regulation. Science, 279, 1358–1360.10.1126/science.279.5355.1358
- Gershon, M. D., & Tack, J. (2007). The serotonin signaling system: From basic understanding to drug development for functional GI disorders. Gastroenterology, 132, 397–414. doi:10.1053/j.gastro.2006.11.002
- Hasan, K. N., Moniruzzaman, M., & Maitra, S. K. (2014). Melatonin concentrations in relation to oxidative status and oocyte dynamics in the ovary during different reproductive phases of an annual cycle in carp Catla catla. Theriogenology, 82, 1173–1185. doi:10.1016/j.theriogenology.2014.08.001
- Iigo, M., Abe, T., Kambayashi, S., Oikawa, K., Masuda, T., Mizusawa, K., ... Yanagisawa, T. (2007). Lack of circadian regulation of in vitro melatonin release from the pineal organ of salmonid teleosts. General and Comparative Endocrinology, 154, 91–97. doi:10.1016/j.ygcen.2007.06.013
- Isorna, E., Besseau, L., Boeuf, G., Desdevises, Y., Vuilleumier, R., Alonso-Gómez, A. L., ... Falcón, J. (2006). Retinal, pineal and diencephalic expression of frog arylalkylamine N-acetyltransferase-1. Molecular and Cellular Endocrinology, 252, 11–18. doi:10.1016/j.mce.2006.03.032
- Jimenez-Jorge, S., Guerrero, J. M., Jimenez-Caliani, A. J., Naranjo, M. C., Lardone, P. J., Carrillo-Vico, A., ... Molinero, P. (2007). Evidence for melatonin synthesis in the rat brain during development. Journal of Pineal Research, 42, 240–246. doi:10.1111/j.1600-079X.2006.00411.x
- Khan, Z. A., Yumnamcha, T., Rajiv, C., Devi, H. S., Mondal, G., Devi, S. D., & Chattoraj, .A. (2016). Melatonin biosynthesizing enzyme genes and clock genes in ovary and whole brain of zebrafish (Danio rerio): Differential expression and a possible interplay. General and Comparative Endocrinology, 233, 16–31. doi:10.1016/j.ygcen.2016.05.014
- Konturek, P. C., Brzozowski, T., & Konturek, S. J. (2011). Gut clock: Implication of circadian rhythms in the gastrointestinal tract. Journal of Physiology and Pharmacology : An Official Journal of the Polish Physiological Society, 62, 139–150.
- Korf, H. W., Schomerus, C., & Stehle, J. H. (1998). The pineal organ, its hormone melatonin, and the photoneuroendocrine system. Advances in anatomy, embryology, and cell biology, 146, 1–8.10.1007/978-3-642-58932-4
- Kulczykowska, E., Kalamarz, H., Warne, J. M., & Balment, R. J. (2006). Day–night specific binding of 2-[125I]Iodomelatonin and melatonin content in gill, small intestine and kidney of three fish species. Journal of Comparative Physiology B, 176, 277–285. doi:10.1007/s00360-005-0049-4
- Kumari, K., Pathakota, G.-B., Annam, P.-K., Kumar, S., & Krishna, G. (2015). Characterisation and validation of house keeping gene for expression analysis in Catla catla (Hamilton). Proceedings of the National Academy of Sciences, India Section B: Biological Sciences, 85, 993–1000. doi:10.1007/s40011-014-0482-9
- Lillesaar, C. (2011). The serotonergic system in fish. Journal of Chemical Neuroanatomy, 41, 294–308. doi:10.1016/j.jchemneu.2011.05.009
- Liu, T., & Borjigin, J. (2005). N-acetyltransferase is not the rate-limiting enzyme of melatonin synthesis at night. Journal of Pineal Research, 39, 91–96. doi:10.1111/j.1600-079X.2005.00223.x
- Maitra, S., & Chattoraj, A. (2007). Role of photoperiod and melatonin in the regulation of ovarian functions in Indian carp Catla catla: Basic information for future application. Fish Physiology and Biochemistry, 33, 367–382.10.1007/s10695-007-9174-1
- Maitra, S. K., Chattoraj, A., Mukherjee, S., & Moniruzzaman, M. (2013). Melatonin: A potent candidate in the regulation of fish oocyte growth and maturation. General and Comparative Endocrinology, 181, 215–222. doi:10.1016/j.ygcen.2012.09.015
- Malek, Z. S., Dardente, H., Pevet, P., & Raison, S. (2005). Tissue-specific expression of tryptophan hydroxylase mRNAs in the rat midbrain: Anatomical evidence and daily profiles. European Journal of Neuroscience, 22, 895–901. doi:10.1111/j.1460-9568.2005.04264.x
- Messner, M., Huether, G., Lorf, T., Ramadori, G., & Schwörer, H. (2001). Presence of melatonin in the human hepatobiliary-gastrointestinal tract. Life Sciences, 69, 543–551.10.1016/S0024-3205(01)01143-2
- Mukherjee, S., & Maitra, S. K. (2015). Gut melatonin in vertebrates: Chronobiology and physiology. Frontiers in endocrinology, 6, 112. doi:10.3389/fendo.2015.00112
- Mukherjee, S., Moniruzzaman, M., & Maitra, S. K. (2014). Daily and seasonal profiles of gut melatonin and their temporal relationship with pineal and serum melatonin in carp Catla catla under natural photo-thermal conditions. Biological Rhythm Research, 45, 301–315. doi:10.1080/09291016.2013.817139
- Muñoz, J. L., Ceinos, R. M., Soengas, J. L., & Míguez, J. M. (2009). A simple and sensitive method for determination of melatonin in plasma, bile and intestinal tissues by high performance liquid chromatography with fluorescence detection. Journal of Chromatography B, 877, 2173–2177. doi:10.1016/j.jchromb.2009.06.001
- Muñoz-Pérez, J. L., López-Patiño, M. A., Álvarez-Otero, R., Gesto, M., Soengas, J. L., & Míguez, J. M. (2016). Characterization of melatonin synthesis in the gastrointestinal tract of rainbow trout (Oncorhynchus mykiss): Distribution, relation with serotonin, daily rhythms and photoperiod regulation. Journal of Comparative Physiology B, 186, 471–484. doi:10.1007/s00360-016-0966-4
- Nelson, W., Tong, Y. L., Lee, J. K., & Halberg, F. (1979). Methods for cosinor-rhythmometry. Chronobiologia, 6, 305–323.
- Nisembaum, L. G., Tinoco, A. B., Moure, A. L., Alonso Gómez, A. L., Delgado, M. J., & Valenciano, A. I. (2013). The arylalkylamine-N-acetyltransferase (AANAT) acetylates dopamine in the digestive tract of goldfish: A role in intestinal motility. Neurochemistry International, 62, 873–880. doi:10.1016/j.neuint.2013.02.023
- Noche, R. R., Lu, P. N., Goldstein-Kral, L., Glasgow, E., & Liang, J. O. (2011). Circadian rhythms in the pineal organ persist in zebrafish larvae that lack ventral brain. BMC Neuroscience, 12, 7. doi:10.1186/1471-2202-12-7
- Paulin, C. H., Cazamea-Catalan, D., Zilberman-Peled, B., Herrera-Perez, P., Sauzet, S., Magnanou, E., & Besseau, L. (2015). Subfunctionalization of arylalkylamine N-acetyltransferases in the sea bass Dicentrarchus labrax: Two-ones for one two. Journal of Pineal Research, 59, 354–364. doi:10.1111/jpi.12266
- Paulose, J. K., Wright, J. M., Patel, A. G., & Cassone, V. M. (2016). Human gut bacteria are sensitive to melatonin and express endogenous circadian rhythmicity. Plos One, 11, e0146643. doi:10.1371/journal.pone.0146643
- Piesiewicz, A., Kedzierska, U., Turkowska, E., Adamska, I., & Majewski, P. M. (2015). Seasonal postembryonic maturation of the diurnal rhythm of serotonin in the chicken pineal gland. Chronobiology International, 32, 59–70. doi:10.3109/07420528.2014.955185
- Portaluppi, F., Smolensky, M. H., & Touitou, Y. (2010). Ethics and methods for biological rhythm research on animals and human beings. Chronobiology International, 27, 1911–1929. doi:10.3109/07420528.2010.516381
- Refinetti, R., Cornélissen, G. C., & Halberg, F. (2007). Procedures for numerical analysis of circadian rhythms. Biological Rhythm Research, 38, 275–325. doi:10.1080/09291010600903692
- Reiter, R. J. (1991a). Melatonin synthesis: Multiplicity of regulation. Advances in Experimental Medicine and Biology, 294, 149–158.10.1007/978-1-4684-5952-4
- Reiter, R. J. (1991b). Melatonin: The chemical expression of darkness. Molecular and Cellular Endocrinology, 79, C153–C158.10.1016/0303-7207(91)90087-9
- Reiter, R. J., Tan, D.-X., Manchester, L. C., & Gitto, E. (2010). Gastrointestinal tract and melatonin: Reducing pathophysiology. Polish Gastroenterology, 17, 213–218.
- Richards, J., & Gumz, M. L. (2012). Advances in understanding the peripheral circadian clocks. The FASEB Journal, 26, 3602–3613. doi:10.1096/fj.12-203554
- Seth, M., & Maitra, S. K. (2010). Importance of light in temporal organization of photoreceptor proteins and melatonin-producing system in the pineal of carp Catla catla. Chronobiology International, 27, 463–486. doi:10.3109/07420521003666416
- Seth, M., & Maitra, S. K. (2011). Neural regulation of dark-induced abundance of arylalkylamine N -acetyltransferase (AANAT) and melatonin in the carp ( Catla catla ) pineal: An in vitro study. Chronobiology International, 28, 572–585. doi:10.3109/07420528.2011.590913
- Stefulj, J., Hortner, M., Ghosh, M., Schauenstein, K., Rinner, I., Wolfler, A., & Liebmann, P. M. (2001). Gene expression of the key enzymes of melatonin synthesis in extrapineal tissues of the rat. Journal of Pineal Research, 30, 243–247.10.1034/j.1600-079X.2001.300408.x
- Sugden, D. (2003). Comparison of circadian expression of tryptophan hydroxylase isoform mRNAs in the rat pineal gland using real-time PCR. Journal of Neurochemistry, 86, 1308–1311.10.1046/j.1471-4159.2003.01959.x
- Uz, T., Qu, T., Sugaya, K., & Manev, H. (2002). Neuronal expression of arylalkylamine N-acetyltransferase (AANAT) mRNA in the rat brain. Neuroscience Research, 42, 309–316.10.1016/S0168-0102(02)00011-1
- Velarde, E., Cerdá-Reverter, J. M., Alonso-Gómez, A. L., Sánchez, E., Isorna, E., & Delgado, M. J. (2010). Melatonin-synthesizing enzymes in pineal, retina, liver, and gut of the goldfish ( Carassius ): mRNA expression pattern and regulation of daily rhythms by lighting conditions. Chronobiology International, 27, 1178–1201. doi:10.3109/07420528.2010.496911
- Velarde, E., Delgado, M. J., & Alonso-Gómez, A. L. (2010). Serotonin-induced contraction in isolated intestine from a teleost fish (Carassius auratus): Characterization and interactions with melatonin. Neurogastroenterology & motility, 22, e364–e373. doi:10.1111/j.1365-2982.2010.01605.x
- Velarde, E., Haque, R., Iuvone, P. M., Azpeleta, C., Alonso-Gomez, A. L., & Delgado, M. J. (2009). Circadian clock genes of goldfish, Carassius auratus: cDNA Cloning and rhythmic expression of Period and Cryptochrome transcripts in retina, liver, and gut. Journal of Biological Rhythms, 24, 104–113. doi:10.1177/0748730408329901.
- Whelan, J. A., Russell, N. B., & Whelan, M. A. (2003). A method for the absolute quantification of cDNA using real-time PCR. Journal of Immunological Methods, 278, 261–269. doi:10.1016/S0022-1759(03)00223-0
- Whitmore, D., Foulkes, N. S., Strahle, U., & Sassone-Corsi, P. (1998). Zebrafish clock rhythmic expression reveals independent peripheral circadian oscillators. Nature neuroscience, 1, 701–707. doi:10.1038/3703