Abstract
Birds, including members of the families Anatidae (waterfowl) and Laridae (gulls and terns), serve as the major reservoir of influenza A viruses (IAVs). The ecogeographic contributions of gulls to global IAV dynamics, in terms of geographic scale and virus movements, are important and are distinct from those of waterfowl. Gulls primarily carry the H13 and H16 subtypes, yet can be infected by additional subtypes. Also, gulls are frequently infected by IAVs that contain mixtures of genes from different geographic phylogenetic lineages (e.g. North American and Eurasian). The present analysis examines a variety of viruses isolated from gulls and terns across the world that exhibit particularly high phylogenetic affinities to viruses found in other hosts. This illustrates the potential for gulls to act as highly pathogenic virus carriers, disseminators of viruses over long distances, and contributors in the genesis of pandemic strains. The historical evolution of an entirely Eurasian gull virus isolated in North America was also traced and indicates the Caspian Sea, in southwestern Asia, was an important area for the generation of this virus, and analysis of IAVs from terns also points to this region as relevant for the generation of novel strains.
Public Interest Statement
Influenza A viruses are most well known for their effects on human health and the agriculture industry. However, the movement of these viruses among their natural reservoir species, which are wild birds, plays an important role in these human society-affecting outcomes. Within wild birds, gulls are one important host group and the dynamics of influenza A virus transmission and evolution within this group need to be considered alongside the dynamics in other host groups. This paper presents an analysis of gull viruses and gull biology relevant to this topic.
Competing Interests
The authors declare no competing interest.
1. Introduction
Wild birds, including waterfowl, shorebirds, and gulls, harbor the vast majority of known influenza A virus (IAV) genetic diversity. Within these influenza IAVs, there is clear distinction between Eurasian and North American gene pools (Olsen et al., Citation2006) and there is increasing evidence of additional phylogeographic lineages (Gonzalez-Reiche & Perez, Citation2012; Hurt et al., Citation2014; Pereda et al., Citation2008). However, bird movements lead to inter-regional transmission of IAV genes and their establishment in new regions. Intercontinental transmission of IAV genes from Eurasia to America has been frequently observed, and isolation of reassortant viruses that contain gene segments of different continental origins is particularly common from gulls in these regions, which is presumably facilitated by gull movements across the oceans. The North Atlantic Ocean is one location where gull and IAV movements between North America and Europe have been documented (Wille, Robertson, Whitney, Ojkic, & Lang, Citation2011), and intercontinental IAV reassortants have also been identified in high proportions (Huang et al., Citation2014). Additionally, both entirely Eurasian and North American gull IAVs have been found in Iceland (Dusek et al., Citation2014), suggesting this location as important for the mixing of viruses between regions. An equivalent position has also been proposed for Greenland (Shoham & Rogers, Citation2006). In North America, only two wholly Eurasian avian IAVs have been found to date. One was an H16N3 virus from an American herring gull (Larus smithsonianus) in eastern Canada (Huang et al., Citation2014). The other was the highly pathogenic avian influenza (HPAI) H5N8 virus that recently emerged in China, spread to South Korea, Japan, and Siberia, and is proposed to have been transmitted through the Beringia region by migratory waterfowl into Pacific North America (Lee et al., Citation2015). This H5N8 arrival ended the long speculation about whether or not virulent Asian avian strains, such as H5N1, could be transmitted into the Americas by migrating birds. There is also a third case, involving H9N2 viruses from Alaska that were nearly identical (>99%) to viruses in China and South Korea, which were proposed to be carried to Alaska by migratory waterfowl (Ramey et al., Citation2015). In this case, however, the gene segments of the viruses showed mixed geographic origins.
In addition to geographic divisions in IAV genes, distinct host group lineages are found. These include mainly the avian, gull, human, swine, and equine lineages (Olsen et al., Citation2006). These distinctions make it possible to recognize that inter-species transmissions of IAVs between gulls and other hosts have occurred. Although most IAVs from gulls contain gull-lineage gene segments, and most are of the hemagglutinin (HA) subtypes H13 and H16, various viruses found in gulls contain HA genes and other gene segments that do not appear to be gull-specific, but rather fall within avian clades and are closely related to waterfowl viruses in phylogenetic analyses (Hall et al., Citation2013; Van Borm et al., Citation2012; Wille et al., Citation2011). The opportunities for these transmissions certainly exist, as gulls share terrestrial, freshwater, and marine habitats with other birds and mammals, and therefore gulls could be important for moving IAVs among ecosystems and for contributing to the transmission of highly pathogenic IAVs to other hosts.
The present analysis is an investigation of the potential for gulls to act as carriers of highly pathogenic viruses, disseminators of viruses over long distances, and contributors in the genesis of pandemic strains. This is done through examination of a variety of gull and tern viruses isolated from different locations that exhibit particularly high phylogenetic affinities to viruses found in other hosts.
2. Results and discussion
2.1. Ecogenetic aspects of gull IAVs
Avian IAVs constitute the major, and likely the primal, component within the total IAV gene pool worldwide (Olsen et al., Citation2006; Webster, Bean, Gorman, Chambers, & Kawaoka, Citation1992). Distinct phylogeographic lineages, such as those representing Eurasia and North America, can be identified within avian IAVs, mainly as a result of partial segregation of migratory birds (Olsen et al., Citation2006). The first indication that gulls might represent a distinct reservoir for IAVs came from surveillance work on the Atlantic coast of North America, with the identification of a new HA antigen, H13, distinct from the previous 12 HA antigens identified in other avian species (Hinshaw et al., Citation1982). Two decades later, an additional novel HA subtype, H16, was identified and characterized from gulls in Sweden (Fouchier et al., Citation2005). Continued surveillance and research have shown that gulls are predominantly infected by these H13 and H16 subtypes (Figure ), and these subtypes are not often found outside of gulls (other than in shorebirds) (Arnal et al., Citation2015). We performed phylogenetic and time of most recent common ancestor (TMRCA) analyses of H13 and H16 nucleotide sequences. These showed three distinct clades for each subtype that were estimated to originate from a common ancestor in the 1930s for the H13 lineage (Figure ) and in the 1920s for the H16 lineage (Figure ), similar to previous estimates (Worobey, Han, & Rambaut, Citation2014). Both trees show some segregation of sequences according to geographic origin as well as clades that contain mixtures of viruses from both Eurasia and North America. These gull lineage HA genes have been transferred to waterfowl, poultry, and marine mammals but there is no evidence of persistence of these sequences in these other host groups.
Figure 1. Distribution of IAV subtypes isolated from gulls.
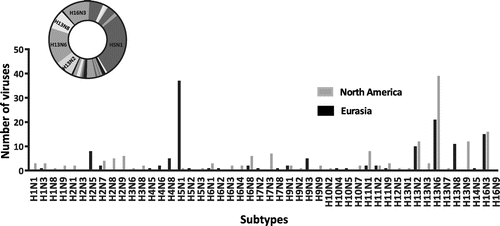
Figure 2. Time-clock Bayesian inference analysis of H13 nucleotide sequences from North America and Eurasia.
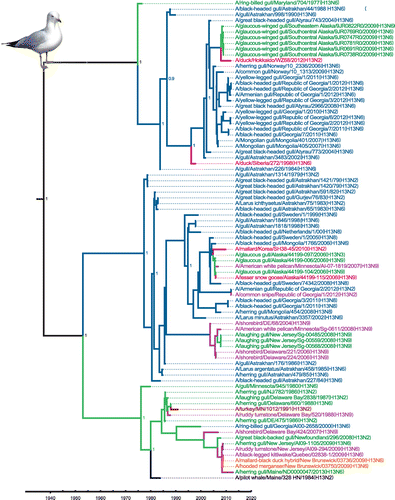
Figure 3. Time-clock Bayesian inference analysis of H16 nucleotide sequences from North America and Eurasia.
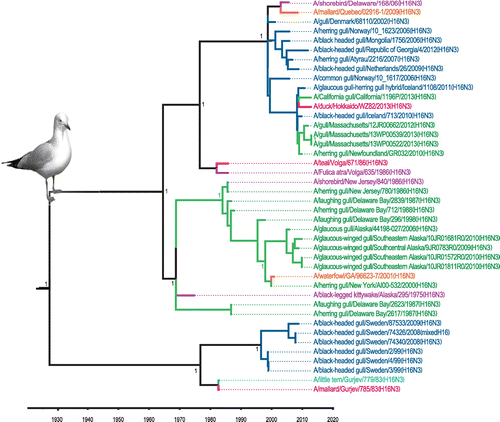
Inter-hemispheric movements of avian IAV genes from Eurasia to America have repeatedly been documented (Dusek et al., Citation2014; Fries et al., Citation2013; Ip et al., Citation2008). However, observations of IAV movements from America to Eurasia have been less common. Possible explanations for this include a higher rate of infection in birds during movements from Eurasia to America, differences in net avian biomass traveling in the two directions, incompatibility of the Eurasian IAV gene pool with North American genes introduced into Eurasia, or uneven virological monitoring. Regardless, the North American avian IAV gene pool appears appreciably permissive for Eurasian genes, which are frequently assimilated (J. Bahl, Vijaykrishna, Holmes, Smith, & Guan, Citation2009). This is exemplified by the case of the H6 subtype, where it was shown that IAV movement from Eurasia to North America led to the replacement of the North American H6 subtype, continent-wide, within a decade of its introduction (Zu Dohna, Li, Cardona, Miller, & Carpenter, Citation2009). However, this is an extreme case, and Eurasian gene introductions do not usually appear to be so dramatic and successful. As mentioned above, there have been only two discoveries of entirely Eurasian IAVs in North America, the H16N3 gull virus and the highly pathogenic H5N8 virus. Examining the evolutionary histories of the entirely Eurasian H16N3 gull virus’ genes reveals that the Caspian Sea appears to be a source for the generation of this virus and others. Indeed, genetic analyses illustrate the spatiotemporal distribution of viruses that traces its gene segment origins from Astrakhan, in Russia, in 1983 to Ukraine in 2005, Scandinavia between 2006–2009, then to Iceland and Eastern Canada in 2010 (Figure and Table S1). Therefore, it appears that the Eurasian isolate from Eastern Canada has been generated through the gradual accumulation of different genes (through reassortment events) at different locations from 1983 to 2010, although we recognize that there are limitations in the consistency of availability of data through time and from all regions. This was presumably facilitated through the migration of gulls across these regions and the spatiotemporal pattern suggests a predominantly east to west movement and evolutionary trend. While only single gene segments were found closely related to the Eurasian gull virus preceding Iceland, this recent virus from Iceland showed a close relationship to the virus from Eastern Canada for four gene segments (HA, PB2, PA, and NP). It is also notable that an H16 segment similar to the North American virus was detected three years later in a duck in Japan, which points towards an active inter-hemispheric gull-duck interface (Table S1). Globally, the H16N3 North American gull virus is presently the first and only whole IAV of which a trans-Atlantic ecophylogenetic course has been concretely traced. Its origin and spatiotemporal phylogenetic history are analogous to the apparent natural history of the H14 viruses that were originally isolated from a gull and mallard (Anas platyrhynchos) in Astrakhan, and subsequently recovered 28 years later in ducks in North America (Fries et al., Citation2013). The H14 subtype was originally isolated in 1982 from both European herring gulls (Larus argentatus) and mallards sampled in Central Asia along the northern shore of the Caspian Sea. Subsequent identifications of H14 viruses have occurred solely in North and Central America (USA and Guatemala) during 2010 to 2012 (Boyce et al., Citation2013; Ramey et al., Citation2014). These viruses originated from sea ducks and dabbling ducks, which are most frequently associated with marine and freshwater habitats, respectively. This unusual spatiotemporal profile might indicate a role played by gulls in inter-hemispheric and marine-freshwater avifauna IAV transmission. The gap in H14 virus isolation from 1982 to 2010 could be due to a lack of fortuitous sampling during those periods and the same applies for the large gap between the identification of gene segments similar to the North American H16N3 gull virus. Regardless, the overall movements of the involved host species are compatible with the viral migration routes. Broadly, such analyses might be useful for detailed reconstruction of evolutionary processes in other IAVs.
Figure 4. Putative geographic progression of gull virus genes that contributed to the genesis of the Eurasian H16N3 gull virus identified in Newfoundland, Canada.

2.2. Ecogeographic aspects of gull IAVs
The global distribution of gulls is vast and nearly unlimited worldwide (K. M. Olsen & Larsson, Citation2004). They breed on every continent, from the margins of Antarctica to the high Arctic. Many species breed in coastal colonies and although they are less common on tropical islands, where terns are often very common, a few species do inhabit islands such as the Galapagos and New Caledonia. There is considerable variety within the group, and species may breed and feed in marine, freshwater and/or terrestrial habitats. Many gull species migrate, but the extent to which they migrate varies among species. Some species migrate long distances, such as Franklin’s gull (Leucophaeus pipixcan) that migrates from Canada to wintering grounds in southern South America. Two examples illustrating inter-hemispheric distribution are black-headed gull (Chroicocephalus ridibundus) and laughing gull (Leucophaeus atricilla). The black-headed gull mostly breeds in Europe and Asia, and most of the population is migratory, wintering further south, but some birds in the milder westernmost areas of Europe are non-migratory. Some birds also spend the winter in northeastern North America. Additionally, black-headed gulls have an impressive ability to adapt to man-made environments. The laughing gull is largely a species of North and South America although it also occurs as a rare vagrant to western Europe. Herring gulls exhibit intercontinental distribution and connectivity and there is overlap in the ranges of closely related species/subspecies within a larger species complex that includes American herring gull, European herring gull, and East Siberian gull (Larus vegae) (Sangster, Collinson, Knox, Parkin, & Svensson, Citation2007). However, it is likely that migratory connectivity exists among these related American, East Eurasian, and European populations, and this may serve as a platform for virus movements across both the Pacific and Atlantic Oceans.
2.3. Clinical and subclinical IAV infections of gulls
Historically, IAVs have been classified as virulent in gulls and terns in only two natural episodes. These are the first wild bird IAV isolate, A/Tern/South Africa/61(H5N3) (Becker, Citation1966), and the Asian highly pathogenic H5N1 lineage that infects many species of mammals and birds (Amonsin et al., Citation2007; Chen et al., Citation2005; Claas et al., Citation1998; Mushtaq et al., Citation2008; Subbarao et al., Citation1998). However, an H13 virus circulating in a Canadian colony of dense-breeding ring-billed gulls (Larus delawarensis) caused inflammation of the heart, kidney, pancreas, and liver in chicks but no clinical symptoms (Velarde, Calvin, Ojkic, Barker, & Nagy, Citation2010). Details from some of the experimental infection studies involving gulls or other hosts infected with avian strains or with gull viruses are summarized in Tables , and some details are expanded below.
Table 1. Experimental infections of gulls with avian strains
Table 2. Experimental infections of avian and mammalian hosts with gull strains
Table 3. Gull virus binding assays on avian and human tissues (Lindskog et al., Citation2013)
Experimental infection with the A/chicken/Hong Kong/220/97(H5N1) strain produced mild disease in laughing gulls (Perkins & Swayne, Citation2002), whereas severe disease and mortality were reported in laughing gulls after infection with highly pathogenic H5N1 strains from 2001 and 2006 (Brown, Stallknecht, Beck, Suarez, & Swayne, Citation2006). Another highly pathogenic H5 strain, A/Chicken/Penn/1370/83(H5N2), which caused 80% mortality in chickens in a Pennsylvania outbreak, produced little to no clinical signs in experimentally infected ring-billed gulls (Wood, Webster, & Nettles, Citation1985). Franklin’s gulls experimentally infected with a turkey virus that was pathogenic for turkeys did not develop signs of disease, yet were capable of shedding the virus (Bahl & Pomeroy, Citation1977). These studies suggest that gulls could be infected with virulent strains from poultry and serve as asymptomatic carriers for subsequent virus transmission. In another study, the virus A/laughing gull/NJ/AI08–1460/2008(H13N9) was used to experimentally infect ring-billed gulls by the respiratory route, and high susceptibility to the virus was observed without developing any clinical symptoms (Brown et al., Citation2012). Most importantly, the virus was excreted via the oropharynx and cloaca for several days. Collectively, in vivo experimental infection studies show fairly consistent clinical sensitivity of gulls to different highly pathogenic viruses, and subclinical sensitivity to low pathogenicity viruses, with consistent evidence of virus shedding.
Studies involving experimental infections of mammals with gull viruses have been limited, but several are of particular interest. In one study (Hinshaw et al., Citation1982), the H13 gull virus A/gull/Massachusetts/26/1980(H13N6) was used in experimental infection of ferrets, which are generally considered as the best animal model for human influenza infection. When challenged intranasally, the ferrets contracted the virus and shed it in nasal secretions. Additionally, attachment of the strain A/black-headed gull/Sweden/2/99(H16N3) to human, gull, and mallard tissues was investigated using tissue microarrays and virus histochemistry, using mallard and human viruses as references (Lindskog et al., Citation2013). The H16N3 virus attached more readily to the human respiratory tract than the other avian IAVs, and it could also bind to the human cornea and conjunctiva. The human virus, A/Netherlands/213/03(H3N2), also attached to the trachea of both gull species investigated, which demonstrates the presence of the first necessary characteristic for respiratory transmission. It was consequently suggested that H16 viruses might present a different cell tropism in humans and mallards than other bird-origin IAVs (Lindskog et al., Citation2013). Elsewhere, it was shown that several gull species display α2,6-linked sialic acid receptors, to which human IAVs usually bind, on the surface of their tracheal epithelium (Jourdain et al., Citation2011). Those findings indicate the potential for either direct or indirect gull-human IAV interface. Viruses from gulls were also among those identified in wild birds as encoding proteins that differed at relatively few amino acid positions from the 1918 pandemic strain (Watanabe et al., Citation2014), with gull viruses found that had <15 amino acid differences in their PB2, PB1, PA, NP, M1 or NS1 proteins. A virus identified in wild birds that contained such segments showed higher pathogenicity than typical avian viruses and was able to gain mammalian respiratory drop transmission after acquiring as few as three substitutions in the HA protein (Watanabe et al., Citation2014). This supports the notion that the potential to generate a pandemic virus exists within wild birds and, although the sequences used in the experimental study were not from gull viruses, gulls are a relevant part of this scenario.
2.4. Inter-species transmission of gull IAVs
Although the level of active infection found is much lower, seroprevalence for IAV in gulls is high, reaching 45–92% (Brown et al., Citation2010; Huang et al., Citation2014; Toennessen et al., Citation2011; Velarde et al., Citation2010). As mentioned above, gulls also exhibit notable capacity in terms of inter-hemispheric virus transmission (Hall et al., Citation2013; Huang et al., Citation2014; Perkins & Swayne, Citation2002; Ratanakorn et al., Citation2012; Van Borm et al., Citation2012; Wille, Robertson, Whitney, Bishop, et al., Citation2011, Wille et al., Citation2011). If gull-imported Eurasian gene segments persist within the American gene pool, they could subsequently be transmitted to domestic hosts such as chickens, turkeys and pigs. In consideration of experimental studies that frequently show subclinical infections of gulls by highly pathogenic avian viruses, these hosts should be regarded as candidates for inter-hemispheric transmission of highly pathogenic viruses. The following sections will evaluate whether gulls could play a major role in inter-species and long-range transmission of IAVs and specific IAV genes and whether they could be considered as contributors to the transmission of HPAI viruses.
2.4.1. The gull-poultry IAV interface
The first evidence of poultry infection by a gull IAV came from a study in which H13N2 viruses were isolated in North America from subclinically infected domestic turkey flocks living near accessible pond water and gulls (Sivanandan, Halvorson, Laudert, Senne, & Kumar, Citation1991). The H13 sequence of one of the resultant viruses, A/turkey/MN/1012/1991(H13N2), is included within the North American gull AIV lineage (Figure ). These turkey H13N2 viruses did exhibit marked pathogenicity in experimentally infected turkeys (Laudert, Sivanandan, Halvorson, Shaw, & Webster, Citation1993). However, various H13 gull viruses used for experimental challenges of various poultry species infrequently resulted in infection and appeared only subclinical (Table ). There have been no reports of H16 viruses infecting poultry. A low pathogenicity H7N7 gull strain was widely invasive and caused systemic, though subclinical, infection in experimental studies with chickens (Otsuki, Kawaoka, Nakamura, & Tsubokura, Citation1982). Another low pathogenicity gull virus of the H7 subtype, A/Laughing gull/DE/42/06(H7N3), is believed to have been naturally transmitted to chickens, with subsequent evolution to a highly pathogenic form therein (Krauss et al., Citation2007). This virus was also fully infectious, although mostly subclinically, in experimentally challenged chickens (Krauss et al., Citation2007). Inter-species transmission between gulls and chickens was also observed with A/brown-head gull/Thailand/vsmu-4/2008(H5N1), being highly similar to two highly pathogenic chicken isolates identified from the same region (Figure ). Further sampling in turkey and chicken flocks, especially where there are potential interactions with gulls, would help confirm whether gulls could play a significant role in IAV transmission to poultry.
Figure 5. Phylogenetic analysis of gull H5 and H7 nucleotide sequences.
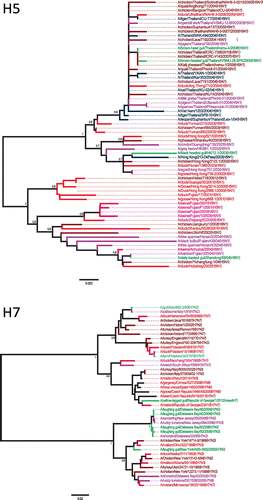
2.4.2. The gull-waterfowl IAV interface
Sympatry exists among many gull species and waterfowl across freshwater and marine habitats. In addition to the H14 viruses discussed above, particularly high gene sequence identity can be found between various gull and duck viruses (Figures and , Table S2). These include both wild (mostly mallard) and domestic duck viruses in America and Eurasia. These diverse affinities substantiate an ongoing, reciprocal gull-waterfowl interface, worldwide, which spans both natural ecosystems and farms containing domestic ducks. Additionally, one study showed that H16N3 gull viruses isolated in Russia readily replicated in respiratory and intestinal tissues in challenged ducks (Iamnikova et al., Citation2009).
2.4.3. The gull-marine mammal IAV interface
The movement of IAV between gulls and mammals was identified for the first time in 1981, when it was shown that all genomic segments from a seal H7N7 virus were highly similar to viral gene segments from avian species, including gulls (Webster et al., Citation1981). Interestingly, the seal isolate replicated poorly in avian species but well in mammals such as ferrets, pigs, and cats, which indicated this isolate had probably accumulated mammalian-adaptive mutations. Phylogenetic analysis of some seal and whale viruses has shown that these are frequently closely related to gull and shorebird viruses (Groth et al., Citation2014). More specifically, relatedness between NP gene segments of the strains A/gull/Maryland/704/77(H13N6) and A/whale/Maine/328/84(H13N2) from the Atlantic Coast of the USA was found (Mandler et al., Citation1990). Similarly, the HA gene segment from the same whale isolate falls within a North American gull H13 lineage, and presumably originated from gulls (Figure , Table S2). High sequence identity was also found between the H5 gene segments from a gull isolate, A/black_headed_gull/HK/12.1/2003(H5N1), and a human isolate, A/Hong_Kong/213/2003(H5N1), both obtained in Asia (Figure , Table S2).
2.4.4. The gull-swine IAV interface
Swine are considered one of the most important mixing vessels of IAV, as genetic reassortment between avian and mammalian viruses occurs in this host. Many of the viruses found in swine also contain genes that originated from human and bird viruses. H1N1, H1N2, and H3N2 viruses are endemic in the swine population worldwide (Brown, Citation2000), which also represent the subtypes circulating in the human population and the presence of those viruses or their genes within gulls might be important in terms of transmission of viruses across distances and host species. To date, several H1 viruses have been found in the gull population in North America and they all fall within a North American lineage that mainly comprises waterfowl viruses. Although no direct evidence of AIV transmission between gulls and swine has been found to date, we identified gull viruses that do exhibit phylogenetic affinities to swine viruses, in conjunction with related waterfowl and poultry viruses. One swine virus from 2002, A/swine/Saskatchewan/18789/02(H1N1), which was closely related to waterfowl viruses (Karasin, West, Carman, & Olsen, Citation2004), was also close to two gull viruses obtained in 2002 from the United States for the HA gene segment (Figure ).
Figure 6. Phylogenetic analysis of gull H1 and H3 nucleotide sequences.
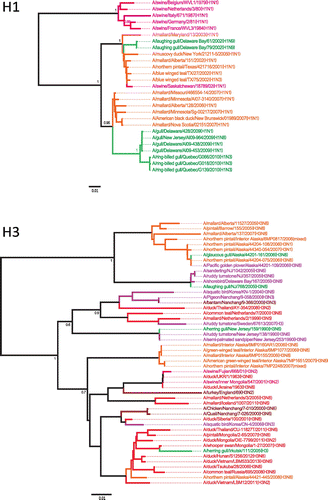
The H3N2 viruses currently found in swine are believed to have originated mostly from reassortment events involving avian and swine viruses or avian, swine and human viruses (Brown, Citation2000) and the triple reassortant virus has now become predominant in the swine population (Richt et al., Citation2003). Sequence analysis of H3 genes from gull viruses showed that two viruses from America and Asia, A/herring gull/New Jersey/159/1990(H3N6) and A/herring gull/Irkutsk/111/2005(H3), which belong to a Eurasian avian lineage, were related to swine and poultry viruses from England and China (Figure ). Additionally, H3 gene segments from shorebirds related to A/herring gull/New Jersey/159/1990(H3N6) and found in North America are falling within a Eurasian lineage, which further illustrate the potential role of gulls in inter-hemispheric movement of IAVs (J. Bahl et al., Citation2013).
2.5. The role of gulls as contributors to the transmission of HPAI viruses
Transmission of IAV from wild birds to poultry and swine is a major concern for the agriculture industry and must also be considered with respect to the possible generation of novel pandemic viruses. H5 and H7 subtypes have been commonly associated with high mortality in poultry (Stech & Mettenleiter, Citation2013). Analysis of H5 sequences from gull viruses showed that most of them are closely related to highly pathogenic H5N1 virus isolated from waterfowl, poultry, and swine (Figure ). Specifically, the virus A/slaty-backed gull/Shandong/59/04(H5N1) is identical to the avian virus A/babbler/Fujian/320/04(H5N1) and groups closely with a swine strain, A/swine/Anhui/ca/2004(H5N1), with all these isolates from 2004. This shows that there has been frequent inter-species transmission of this virus between avian species, including gulls, and swine. Similarly, phylogenetic analysis of H7 sequences from gulls showed that gull viruses from both North America and Eurasia are closely related to viruses associated with lethal H7 outbreaks in poultry (Figure ).
The movements of viruses among gulls, waterfowl, poultry, and mammals indicate that gulls need to be considered as important contributors to IAV evolutionary dynamics and ecology. It is also important to recognize the potential contribution of gulls to the generation of pandemic viruses, which always carry gene segments of bird origin, and that could contribute to the emergence of pathogenic strains.
2.6. The role of terns in global IAV transmission, and relationships of tern IAVs to those from other hosts
Terns are the most closely related taxon to gulls; they exhibit shared ecological features and can also be sympatric with gulls. It is also noteworthy that the first isolate of IAV from wild birds was from terns, with A/tern/South Africa/1961(H5N3) representing a HPAI H5 virus that caused many deaths among terns in South Africa (Becker, Citation1966). Close relationships of viruses from terns to those from other hosts can also be found. A low pathogenicity tern isolate, A/tern/Potsdam/342–6/1979(H7N7), is similar to a virus that infected chickens in Germany and subsequently accrued several mutations to become a highly pathogenic virus that caused a severe outbreak in chickens (Röhm, Süss, Pohle, & Webster, Citation1996). A connection from terns to marine mammals has also been previously documented with the NP gene segment of the tern isolate A/tern/Turkmenistan/18/1972(H3N3) closely related to the whale isolate A/whale/Pacific Ocean/19/1976(H1N3) (Mandler et al., Citation1990).
Phylogenetic analyses with tern virus sequences showed high relatedness of these viruses to viruses identified in gulls, ducks, turkeys, and whales from around the world (Figures S1 and S2, Table S3). Broadly, these sequence comparisons reveal a series of IAVs isolated from two partially sympatric and highly migratory tern species, the common tern (Sterna hirundo) and the Arctic tern (Sterna paradisaea). In particular, the common tern isolate from South Africa showed inter-hemispheric reassortment, as well as affinities to isolates from turkey, seal, whale, and another common tern strain (Figure S2). This isolate from Turkmenistan is one of a group of common tern viruses from a region of Central Asia that show affinities to North American gull isolates, a European mallard isolate, a tufted duck (Aythya fuligula) isolate from southern Siberia, and some duck isolates from Taiwan. The phylogenetic analysis of M gene segments also showed significant homology between an Arctic tern virus (A/arctic tern/Alaska/300/1975(H5N3)) and gull and turkey viruses from North America (Figure S1). Finally, the three polymerase gene segments of the same A/arctic tern/Alaska/300/1975(H5N3) isolate also exhibit affinities to these same North American gull isolates, and the PB2 gene segment is also highly related to gull isolates from Astrakhan, Asia (Figure S1). Overall, these analyses show that terns also constitute an important IAV host. They share many characteristics and display migratory connectivity with gulls, and they should also be considered as contributors to IAV dynamics, diversity and transmission to different hosts.
2.7. Ecobiological aspects of gull IAVs
Two aspects of the ecobiology of gulls are particularly relevant for their role in IAV dynamics. These concern their utilization of both marine and freshwater habitats and their potential role in zoonotic transmission pathways. Gulls are generalist feeders and eat both live-captured prey and opportunistically scavenge, including on carcasses of birds and mammals. Presumably, one means by which gulls could potentially contract IAVs from other species in aquatic habitats is by eating IAV-infected dead birds or mammals. The utilization of both marine and freshwater habitats, and interactions with different species in both environments, raises the possibility for gulls to be exposed to viruses from both freshwater and marine sources. The ecology of IAVs is fairly well understood in freshwater habitats, but this has been explored less in marine environments. However, alongside gulls, additional marine birds such as murres and puffins (members of the family Alcidae) and sea ducks (family Anatidae, tribe Mergini) are known to host IAVs and be sympatric with gulls (Ip et al., Citation2008). Although some IAVs do not appear very stable in elevated salinity (Stallknecht, Kearney, Shane, & Zwank, Citation1990), marine birds drink seawater and it must be presumed that effective fecal–oral transmission can take place in seawater. Viral circulation within marine environments also involves seals and whales, which are known to host avian-derived IAVs and apparently mostly those originating from gulls and terns as our phylogenetic and phylogeographic findings concerning both gull and tern viruses indicate relationships with viruses from marine mammals globally.
There is also an important zoonotic dimension for IAV circulation in gulls. While the important role played by waterfowl as facilitators of the emergence and dispersal of epizootic and pandemic strains is soundly established, gulls are both cosmopolitan migrants and salient synanthropic birds adjacent to the duck-poultry-swine-human IAV interface. It has previously been articulated that there is a crucial epidemiological position occupied by gulls among wildlife, domestic birds as well as humans (Arnal et al., Citation2015), and the potential for gulls to act in transmission of micro-organisms from sewage plants or outflows to domestic animals has also been described (Teale, Citation2002). Natural interfaces between gull viruses and those from ducks, turkeys, chickens, and swine have been identified in the present analysis, either in terms of interchangeability of certain gene segments, or whole viruses, and IAV-related interactions of gulls with ducks, turkeys, chickens, and mammals, phylogenetically and ecologically, might contribute to emergence and spread of epizootic and pandemic strains. It is also of note that a connection was found between marine mammal and human infections with the 2009 H1N1 human pandemic strain, which naturally infected and spread, subclinically, among seals in California (Goldstein et al., Citation2013; Ohishi et al., Citation2004). However, it is not known if gulls or terns were directly involved in transmission of this virus.
3. Concluding remarks
Within the enormous spectrum of IAVs hosted by avian species, gull IAVs constitute an important component, somewhat parallel to waterfowl viruses, of the global ecophylogenetic system defining this protean virus. Gulls affect, both directly and indirectly, the evolutionary dynamics of low and high pathogenicity avian viruses and various mammalian IAVs worldwide. Analysis of the evolutionary history of an entirely Eurasian virus isolated in North America allowed the reconstruction of the gradual evolutionary process that generated this virus, starting in Central Asia, moving northwest, and ending in Iceland, with subsequent transmission to Canada. The origin of this virus appears to be associated with a region of Central Asia that also appears to have given rise to an important H14 strain that involved both gull and duck viruses. Such reverse ecophylogenetic analysis is useful for attaining a broadened perspective of the evolutionary history of a given virus and can allow identification of the presumed strains, genes, and hosts involved in the evolutionary and spatiotemporal pathways that gave rise to a strain in question. Additional gull viruses that were identified as phylogenetically interesting in this analysis were also from the same Central Asian geographic region. A similar scenario was found when analyzing a series of common tern viruses that were obtained in the same general region of Central Asia, where those viruses exhibited clear affinities to gull, duck, and whale viruses. Our analyses of the tern viruses reveal a broad phylogeographic contribution for these viruses beyond Central Asia, with gene transactions extending to Europe, South Africa, North America, and the Pacific Ocean.
The traits contributing to the importance of gulls as IAV hosts include their cosmopolitan distributions, migratory behaviors, synanthropic associations with humans, use of both marine and freshwater habitats, prevalence as a host of many different IAVs of a large variety of host group origins (including farm animals), and predominance of subclinical infections. As shown in the present study, the summary of these properties defines an important IAV host group that needs to be considered in the context of the perpetuation and emergence of enzootic, epizootic, and pandemic strains.
Funding
JEB and ANKK were partially supported by fellowships from the Memorial University School of Graduate Studies. Funding from the Newfoundland and Labrador Forestry and Agrifoods Agency and a Grant (341561) from the Natural Sciences and Engineering Research Council (NSERC) of Canada to ASL supported this research.
Supplementary_Material.docx
Download MS Word (8 MB)Acknowledgments
We thank M. Canuti for comments on the manuscript.
Additional information
Notes on contributors
Andrew S. Lang
Research on influenza A viruses in the laboratory of Andrew Lang is focused on identification and characterization of viruses from wild birds. There is a particular emphasis on the study of gulls and seabirds as hosts of influenza A viruses, and this research is focused in the North Atlantic region of North America. These viruses are analyzed for their evolutionary relationships with those from other species and locations to follow virus transmission among different hosts and regions.
References
- Altschul, S. F., Gish, W., Miller, W., Myers, E. W., & Lipman, D. J. (1990). Basic local alignment search tool. Journal of Molecular Biology, 215, 403–410. doi:10.1016/S0022-2836(05)80360-2
- Amonsin, A., Songserm, T., Chutinimitkul, S., Jam-on, R., Sae-Heng, N., Pariyothorn, N., ... Poovorawan, Y. (2007). Genetic analysis of influenza A virus (H5N1) derived from domestic cat and dog in Thailand. Archives of Virology, 152, 1925–1933. doi:10.1007/s00705-007-1010-5
- Arnal, A., Vittecoq, M., Pearce-Duvet, J., Gauthier-Clerc, M., Boulinier, T., & Jourdain, E. (2015). Laridae: A neglected reservoir that could play a major role in avian influenza virus epidemiological dynamics. Critical Reviews in Microbiology, 41, 508–519. doi:10.3109/1040841X.2013.870967
- Bahl, A. K., & Pomeroy, B. S. (1977). Experimental exposure of Franklins' gulls (Larvus pipixcan) and mallards (Anas platyrhynchos) to a turkey influenza A virus A/Turkey/Minn/BF/72 (Hav6Neq2). Journal of Wildlife Diseases, 13, 420–426.10.7589/0090-3558-13.4.420
- Bahl, J., Vijaykrishna, D., Holmes, E. C., Smith, G. J., & Guan, Y. (2009). Gene flow and competitive exclusion of avian influenza A virus in natural reservoir hosts. Virology, 390, 289–297. doi:10.1016/j.virol.2009.05.002
- Bahl, J., Krauss, S., Kühnert, D., Fourment, M., Raven, G., Pryor, S. P., ... Webster, R. G. (2013). Influenza A virus migration and persistence in North American wild birds. PLoS Pathogens, 9, e1003570. doi:10.1371/journal.ppat.1003570
- Bao, Y., Bolotov, P., Dernovoy, D., Kiryutin, B., Zaslavsky, L., Tatusova, T., ... Lipman, D. (2008). The Influenza Virus Resource at the National Center for Biotechnology Information. Journal of Virology, 82, 596–601. doi:10.1128/jvi.02005-07
- Becker, W. B. (1966). The isolation and classification of Tern virus: Influenza virus A/Tern/South Africa/1961. Journal of Hygiene, 64, 309–320.10.1017/S0022172400040596
- Boyce, W. M., Schobel, S., Dugan, V. G., Halpin, R. A., Lin, X., Wentworth, D. E., ... Plancarte, M. (2013). Complete genome sequence of a reassortant H14N2 avian influenza virus from California. Genome Announcements, 1, e00543–00513. doi:10.1128/genomeA.00543-13
- Brown, I. H. (2000). The epidemiology and evolution of influenza viruses in pigs. Veterinary Microbiology, 74, 29–46.10.1016/S0378-1135(00)00164-4
- Brown, J. D., Luttrell, M. P., Berghaus, R. D., Kistler, W., Keeler, S. P., Howey, A., ... Stallknecht, D. E. (2010). Prevalence of antibodies to type A influenza virus in wild avian species using two serologic assays. Journal of Wildlife Diseases, 46, 896–911. doi:10.7589/0090-3558-46.3.896
- Brown, J. D., Stallknecht, D. E., Beck, J. R., Suarez, D. L., & Swayne, D. E. (2006). Susceptibility of North American ducks and gulls to H5N1 highly pathogenic avian influenza viruses. Emerging Infectious Diseases, 12, 1663–1670.10.3201/eid1211.060652
- Brown, J. D., Stallknecht, D. E., & Swayne, D. E. (2008). Experimental infections of herring gulls (Larus argentatus) with H5N1 highly pathogenic avian influenza viruses by intranasal inoculation of virus and ingestion of virus-infected chicken meat. Avian Pathology, 37, 393–397. doi:10.1080/03079450802216595
- Brown, J., Poulson, R., Carter, D., Lebarbenchon, C., Pantin-Jackwood, M., Spackman, E., ... Stallknecht, D. (2012). Susceptibility of avian species to North American H13 low pathogenic avian influenza viruses. Avian Diseases, 56, 969–975.10.1637/10158-040912-Reg.1
- Chen, H., Smith, G. J., Zhang, S. Y., Qin, K., Wang, J., Li, K. S., ... Guan, Y. (2005). Avian flu: H5N1 virus outbreak in migratory waterfowl. Nature, 436, 191–192. doi:10.1038/nature03974
- Claas, E. C., Osterhaus, A. D., van Beek, R., De Jong, J. C., Rimmelzwaan, G. F., Senne, D. A., ... Webster, R. G. (1998). Human influenza A H5N1 virus related to a highly pathogenic avian influenza virus. The Lancet, 351, 472–477. doi:10.1016/s0140-6736(97)11212-0
- Curran, J. M., Robertson, I. D., Ellis, T. M., Selleck, P. W., & O’Dea, M. A. (2013). Variation in the responses of wild species of duck, gull, and wader to inoculation with a wild-bird–origin H6N2 low pathogenicity avian influenza virus. Avian Diseases, 57, 581–586. doi:10.1637/10458-112712-Reg.1
- Daoust, P. Y., van de Bildt, M., van Riel, D., van Amerongen, G., Bestebroer, T., Vanderstichel, R., ... Kuiken, T. (2013). Replication of 2 subtypes of low-pathogenicity avian influenza virus of duck and gull origins in experimentally infected mallard ducks. Veterinary Pathology, 50, 548–559. doi:10.1177/0300985812469633
- Drummond, A. J., Suchard, M. A., Xie, D., & Rambaut, A. (2012). Bayesian phylogenetics with BEAUti and the BEAST 1.7. Molecular Biology and Evolution, 29, 1969–1973. doi:10.1093/molbev/mss075
- Dusek, R. J., Hallgrimsson, G. T., Ip, H. S., Jónsson, J. E., Sreevatsan, S., Nashold, S. W., ... Hall, J. S. (2014). North Atlantic migratory bird flyways provide routes for intercontinental movement of avian influenza viruses. PLoS ONE, 9, e92075. doi:10.1371/journal.pone.0092075
- Fereidouni, S., Harder, T. C., Globig, A., & Starick, E. (2014). Failure of productive infection of mallards (Anas platyrhynchos) with H16 subtype of avian influenza viruses. Influenza and Other Respiratory Viruses, 8, 613–616. doi:10.1111/irv.12275
- Fouchier, R. A. M., Munster, V., Wallensten, A., Bestebroer, T. M., Herfst, S., Smith, D., ... Osterhaus, A. D. M. E. (2005). Characterization of a novel influenza A virus Hemagglutinin subtype (H16) obtained from black-headed gulls. Journal of Virology, 79, 2814–2822.10.1128/JVI.79.5.2814-2822.2005
- Fries, A. C., Nolting, J. M., Danner, A., Webster, R. G., Bowman, A. S., Krauss, S., ... Wang, Y. (2013). Evidence for the circulation and inter-hemispheric movement of the H14 subtype influenza A virus. PLoS ONE, 8, e59216. doi:10.1371/journal.pone.0059216
- Goldstein, T., Mena, I., Anthony, S. J., Medina, R., Robinson, P. W., Greig, D. J., ... Boyce, W. M. (2013). Pandemic H1N1 influenza isolated from free-ranging northern elephant seals in 2010 off the Central California Coast. PLoS ONE, 8, e62259. doi:10.1371/journal.pone.0062259
- Gonzalez-Reiche, A. S., & Perez, D. R. (2012). Where do avian influenza viruses meet in the Americas? Avian Diseases, 56, 1025–1033. doi:10.1637/10203-041412-Reg.1
- Groth, M., Lange, J., Kanrai, P., Pleschka, S., Scholtissek, C., Krumbholz, A., ... Zell, R. (2014). The genome of an influenza virus from a pilot whale: Relation to influenza viruses of gulls and marine mammals. Infection Genetics and Evolution, 24, 183–186. doi:10.1016/j.meegid.2014.03.026
- Hall, J. S., TeSlaa, J. L., Nashold, S. W., Halpin, R. A., Stockwell, T., Wentworth, D. E, ... Ip, H. S. (2013). Evolution of a reassortant North American gull influenza virus lineage: Drift, shift and stability. Virology Journal, 10, 179. doi:10.1186/1743-422X-10-179
- Hinshaw, V. S., Air, G. M., Gibbs, A. J., Graves, L., Prescott, B., & Karunakaran, D. (1982). Antigenic and genetic characterization of a novel hemagglutinin subtype of influenza A viruses from gulls. Journal of Virology, 42, 865–872.
- Huang, Y., Wille, M., Benkaroun, J., Munro, H., Bond, A. L., Fifield, D. A., ... Lang, A. S. (2014). Perpetuation and reassortment of gull influenza A viruses in Atlantic North America. Virology, 456-457, 353–363. doi:10.1016/j.virol.2014.04.009
- Hurt, A. C., Vijaykrishna, D., Butler, J., Baas, C., Maurer-Stroh, S., Silva-de-la-Fuente, M. C., & Gonzalez-Acuna, D. (2014). Detection of evolutionarily distinct avian influenza A viruses in Antarctica. mBio, 5, e01098–01014. doi: 10.1128/mBio.01098-14
- Iamnikova, S. S., Gambarian, A. S., Aristova, V. A., L’Vov, D. K., Lomakina, N. F., Munster, V., & Foucher, R. A. (2009). A/H13 and A/H16 influenza viruses: Different lines of one precursors. Voprosy virusologii, 54, 10–18.
- Ip, H. S., Flint, P. L., Franson, J. C., Dusek, R. J., Derksen, D. V., Gill, R. E., Jr, ... Rothe, T. C. (2008). Prevalence of influenza A viruses in wild migratory birds in Alaska: Patterns of variation in detection at a crossroads of intercontinental flyways. Virology Journal, 5, 71. doi:10.1186/1743-422x-5-71
- Jourdain, E., van Riel, D., Munster, V. J., Kuiken, T., Waldenström, J., Olsen, B., & Ellström, P. (2011). The pattern of influenza virus attachment varies among wild bird species. PLoS ONE, 6, e24155. doi:10.1371/journal.pone.0024155
- Karasin, A. I., West, K., Carman, S., & Olsen, C. W. (2004). Characterization of avian H3N3 and H1N1 influenza a viruses isolated from pigs in Canada. Journal of Clinical Microbiology, 42, 4349–4354. doi:10.1128/jcm.42.9.4349-4354.2004
- Koçer, Z. A., Krauss, S., Zanin, M., Danner, A., Gulati, S., Jones, J. C., ... Webster, R. G. (2015). Possible basis for the emergence of H1N1 viruses with pandemic potential from avian hosts. Emerging Microbes & Infections, 4, e40. doi:10.1038/emi.2015.40
- Krauss, S., Obert, C. A., Franks, J., Walker, D., Jones, K., Seiler, P., ... Webster, R. G. (2007). Influenza in migratory birds and evidence of limited intercontinental virus exchange. PLoS Pathogens, 3, e167. doi:10.1371/journal.ppat.0030167
- Laudert, E., Sivanandan, V., Halvorson, D., Shaw, D., & Webster, R. G. (1993). Biological and molecular characterization of H13N2 influenza type A viruses isolated from turkeys and surface water. Avian Diseases, 37, 793–799.10.2307/1592031
- Lee, D.-H., Swayne, D. E., Torchetti, M. K., Winker, K., Ip, H. S., & Song, C.-S. (2015). Intercontinental spread of asian-origin H5N8 to North America through Beringia by migratory birds. Journal of Virology, 89, 6521–6524. doi:10.1128/JVI.00728-15
- Lindskog, C., Ellström, P., Olsen, B., Pontén, F., van Riel, D., Munster, V. J., ... Jourdain, E. (2013). European H16N3 gull influenza virus attaches to the human respiratory tract and eye. PLoS ONE, 8, e60757. doi:10.1371/journal.pone.0060757
- Mandler, J., Gorman, O. T., Ludwig, S., Schroeder, E., Fitch, W. M., Webster, R. G., & Scholtissek, C. (1990). Derivation of the nucleoproteins (NP) of influenza A viruses isolated from marine mammals. Virology, 176, 255–261. doi:10.1016/0042-6822(90)90250-U
- Mushtaq, M. H., Juan, H., Jiang, P., Li, Y., Li, T., Du, Y., & Mukhtar, M. M. (2008). Complete genome analysis of a highly pathogenic H5N1 influenza A virus isolated from a tiger in China. Archives of Virology, 153, 1569–1574. doi:10.1007/s00705-008-0145-3
- Ohishi, K., Kishida, N., Ninomiya, A., Kida, H., Takada, Y., Miyazaki, N., ... Maruyama, T. (2004). Antibodies to human-related H3 influenza A virus in Baikal seals (Phoca sibirica) and ringed seals (Phoca hispida) in Russia. Microbiology and Immunology, 48, 905–909.10.1111/mim.2004.48.issue-11
- Olsen, K. M., & Larsson, H. (2004). Gulls of North America, Europe, and Asia. Princeton: Princeton University Press.
- Olsen, B., Munster, V. J., Wallensten, A., Waldenstrom, J., Osterhaus, A. D., & Fouchier, R. A. (2006). Global patterns of influenza A virus in wild birds. Science, 312, 384–388. doi:10.1126/science.1122438
- Otsuki, K., Kawaoka, Y., Nakamura, T., & Tsubokura, M. (1982). Pathogenicity for chickens of avian influenza viruses isolated from whistling swans and a black-tailed gull in Japan. Avian Diseases, 26, 314–320.10.2307/1590100
- Pereda, A. J., Uhart, M., Perez, A. A., Zaccagnini, M. E., La Sala, L., Decarre, J., ... Perez, D. R. (2008). Avian influenza virus isolated in wild waterfowl in Argentina: Evidence of a potentially unique phylogenetic lineage in South America. Virology, 378, 363–370. doi:10.1016/j.virol.2008.06.010
- Perkins, L. E. L., & Swayne, D. E. (2002). Susceptibility of laughing gulls (Larus atricilla) to H5N1 and H5N3 highly pathogenic avian influenza viruses. Avian Diseases, 46, 877–885. doi:10.1637/0005-2086(2002)046[0877:SOLGLA]2.0.CO;2
- Ramey, A. M., Poulson, R. L., González-Reiche, A. S., Perez, D. R., Stallknecht, D. E., & Brown, J. D. (2014). Genomic characterization of H14 subtype influenza A viruses in New World waterfowl and experimental infectivity in mallards (Anas platyrhynchos). PLoS ONE, 9, e95620. doi:10.1371/journal.pone.0095620
- Ramey, A. M., Reeves, A. B., Sonsthagen, S. A., TeSlaa, J. L., Nashold, S., Donnelly, T., ... Hall, J. S. (2015). Dispersal of H9N2 influenza A viruses between East Asia and North America by wild birds. Virology, 482, 79–83. doi:10.1016/j.virol.2015.03.028
- Ramis, A., van Amerongen, G., van de Bildt, M., Leijten, L., Vanderstichel, R., Osterhaus, A. D., & Kuiken, T. (2014). Experimental infection of highly pathogenic avian influenza virus H5N1 in black-headed gulls (Chroicocephalus ridibundus). Veterinary Research, 45, 84. doi:10.1186/s13567-014-0084-9
- Ratanakorn, P., Wiratsudakul, A., Wiriyarat, W., Eiamampai, K., Farmer, A. H., Webster, R. G., ... Puthavathana, P. (2012). Satellite tracking on the flyways of brown-headed gulls and their potential role in the spread of highly pathogenic avian influenza H5N1 virus. PLoS ONE, 7, e49939. doi:10.1371/journal.pone.0049939
- Richt, J. A., Lager, K. M., Janke, B. H., Woods, R. D., Webster, R. G., & Webby, R. J. (2003). Pathogenic and antigenic properties of phylogenetically distinct reassortant H3N2 swine influenza viruses cocirculating in the United States. Journal of Clinical Microbiology, 41, 3198–3205.10.1128/JCM.41.7.3198-3205.2003
- Röhm, C., Süss, J., Pohle, V., & Webster, R. G. (1996). Different hemagglutinin cleavage site variants of H7N7 in an influenza outbreak in chickens in Leipzig, Germany. Virology, 218, 253–257. doi:10.1006/viro.1996.0187
- Sangster, G., Collinson, J. M., Knox, A. G., Parkin, D. T., & Svensson, L. (2007). Taxonomic recommendations for British birds: Fourth report. Ibis, 149, 853–857. doi:10.1111/j.1474-919X.2007.00758.x
- Shoham, D., & Rogers, S. (2006). Greenland as a plausible springboard for trans-Atlantic avian influenza spread. Medical Hypotheses, 67, 1460–1461. doi:10.1016/j.mehy.2006.05.039
- Sivanandan, V., Halvorson, D. A., Laudert, E., Senne, D. A., & Kumar, M. C. (1991). Isolation of H13N2 influenza A virus from turkeys and surface water. Avian Diseases, 35, 974–977. doi:10.2307/1591638
- Stallknecht, D. E., Kearney, M. T., Shane, S. M., & Zwank, P. J. (1990). Effects of pH, temperature, and salinity on persistence of avian influenza viruses in water. Avian Diseases, 34, 412–418.10.2307/1591429
- Stech, J., & Mettenleiter, T. C. (2013). Virulence determinants of high-pathogenic avian influenza viruses in gallinaceous poultry. Future Virology, 8, 459–468. doi:10.2217/fvl.13.27
- Subbarao, K., Klimov, A., Katz, J., Regnery, H., Lim, W., Hall, H., & Cox, N. (1998). Characterization of an avian influenza A (H5N1) virus isolated from a child with a fatal respiratory illness. Science, 279, 393–396.10.1126/science.279.5349.393
- Tamura, K., Stecher, G., Peterson, D., Filipski, A., & Kumar, S. (2013). MEGA6: Molecular evolutionary genetics analysis version 6.0. Molecular Biology and Evolution, 30, 2725–2729. doi:10.1093/molbev/mst197
- Teale, C. J. (2002). Antimicrobial resistance and the food chain. Journal of Applied Microbiology, 92, 85s–89s.10.1046/j.1365-2672.92.5s1.20.x
- Toennessen, R., Germundsson, A., Jonassen, C. M., Haugen, I., Berg, K., Barrett, R. T., & Rimstad, E. (2011). Virological and serological surveillance for type A influenza in the black-legged kittiwake (Rissa tridactyla). Virology Journal, 8, 21. doi:10.1186/1743-422x-8-21
- Tønnessen, R., Valheim, M., Rimstad, E., Jonassen, C. M., & Germundsson, A. (2011). Experimental inoculation of chickens with gull-derived low pathogenic avian influenza virus subtype H16N3 causes limited infection. Avian Diseases, 55, 680–685.10.1637/9701-030411-ResNote.1
- Van Borm, S., Rosseel, T., Vangeluwe, D., Vandenbussche, F., van den Berg, T., & Lambrecht, B. (2012). Phylogeographic analysis of avian influenza viruses isolated from Charadriiformes in Belgium confirms intercontinental reassortment in gulls. Archives of Virology, 157, 1509–1522. doi:10.1007/s00705-012-1323-x
- Velarde, R., Calvin, S. E., Ojkic, D., Barker, I. K., & Nagy, E. (2010). Avian influenza virus H13 circulating in ring-billed gulls (Larus delawarensis) in southern Ontario, Canada. Avian Diseases, 54, 411–419. doi:10.1637/8808-040109-Reg.1
- Watanabe, T., Zhong, G., Russell, C. A., Nakajima, N., Hatta, M., Hanson, A., ... Kawaoka, Y. (2014). Circulating avian influenza viruses closely related to the 1918 virus have pandemic potential. Cell Host & Microbe, 15, 692–705. doi:10.1016/j.chom.2014.05.006
- Webster, R. G., Hinshaw, V. S., Bean, W. J., Van Wyke, K. L., Geraci, J. R., St. Aubin, D. J., & Petursson, G. (1981). Characterization of an influenza A virus from seals. Virology, 113, 712–724. doi:10.1016/0042-6822(81)90200-2
- Webster, R. G., Bean, W. J., Gorman, O. T., Chambers, T. M., & Kawaoka, Y. (1992). Evolution and ecology of influenza A viruses. Microbiological Reviews, 56, 152–179.
- Wille, M., Robertson, G. J., Whitney, H., Bishop, M. A., Runstadler, J. A., & Lang, A. S. (2011). Extensive geographic mosaicism in avian influenza viruses from gulls in the Northern Hemisphere. PLoS ONE, 6, e20664. doi:10.1371/journal.pone.0020664
- Wille, M., Robertson, G. J., Whitney, H., Ojkic, D., & Lang, A. S. (2011). Reassortment of American and Eurasian genes in an influenza A virus isolated from a great black-backed gull (Larus marinus), a species demonstrated to move between these regions. Archives of Virology, 156, 107–115. doi:10.1007/s00705-010-0839-1
- Wood, J. M., Webster, R. G., & Nettles, V. F. (1985). Host range of A/Chicken/Pennsylvania/83 (H5N2) influenza virus. Avian Diseases, 29, 198–207.10.2307/1590708
- Worobey, M., Han, G. Z., & Rambaut, A. (2014). A synchronized global sweep of the internal genes of modern avian influenza virus. Nature, 508, 254–257. doi:10.1038/nature13016
- Zu Dohna, H., Li, J., Cardona, C. J., Miller, J., & Carpenter, T. E. (2009). Invasions by Eurasian avian influenza virus H6 genes and replacement of its North American clade. Emerging Infectious Diseases, 15, 1040–1045. doi:10.3201/eid1507.090245