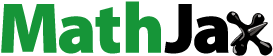
Abstract
Invasive Burmese pythons (Python bivittatus) succumbed to weather-induced mortality in the Florida Everglades in January 2010. We use a mechanistic bioenergetics model to calculate body temperature in various-sized pythons for the successive months of December and January from 2009 to 2014 using daily weather data for the Everglades area. We incorporate python thermal behaviors judged to mitigate weather effects on body temperature. These models suggest that for at least one month in every year except 2013 pythons experienced body temperatures that would subject them to significant physiological stress. However, estimated body temperatures as low as those reported for pythons that succumbed to exposure occurred only in January and December 2010. Results demonstrate the importance of weather variability on survival.
Public Interest Statement
It has been reported in the popular media that the climate along the Eastern seaboard and in many southern states of the United States would allow for the potential expansion of the invasive Burmese python population from the Florida Everglades into these regions. We demonstrate that extremes in weather using data from the Florida Everglades area would likely preclude expansion outside the current range using a more detailed evaluation of the temperature effect on the core body temperature of pythons. In general, temperature extremes are more likely to constrain invasive species expansion and are better estimates for establishment of reptiles than average temperature, which is commonly used.
Competing Interests
The authors declare no competing interest.
1. Introduction
The invasive Burmese python (Python bivittatus) has been established in Southern Florida, USA, in the vicinity of Everglades National Park for around a quarter century (Meshaka, Loftus, & Steiner, Citation2000; Snow, Krysko, Enge, & Oberhoffer, Citation2007). The python population in Florida is attributed to illegal pet releases, although the highly destructive Hurricane Andrew in 1992 may also have released many from captive breeding and holding facilities (Bilger, Citation2009; Engeman, Jacobson, Avery, & Meshaka, Citation2011; Snow et al., Citation2007; Willson, Dorcas, & Snow, Citation2011). The origin of the individuals available in the pet trade at the inception and early growth of the population came from a subset of the native range, initially Thailand near Bangkok and subsequently Vietnam near Ho Chi Minh City after 1994 (Barker & Barker, Citation2008a, Citation2008b). The numbers of pythons that founded the population and the ability of these pythons to expand into the temperate regions of the US are largely unknown but attributed to a small founder population (Dorcas, Willson, & Gibbons, Citation2011; Snow et al., Citation2007; Willson et al., Citation2011).
Rodda, Jarnevich, and Reed (Citation2009, Citation2011) and Pyron, Burbrink, and Guiher (Citation2008) applied climate data from the native ranges of the Burmese python and the closely related Indian python (Python molurus) to evaluate possible range expansion of the Burmese python in the contiguous US as the combined native range of these species covers both tropical and temperate regions in Asia (Groombridge & Luxmoore, Citation1991; Whitaker & Captain, Citation2004; Zhao & Alder, Citation1993). Climate matching has been used extensively to predict establishment of an invasive population by contrasting the climate in the species’ home range with the local climate of the site of invasion (e.g. Bomford, Kraus, Barry, & Lawrence, Citation2009). This has often been the only consistent predictor of invasion success across biological groups (Hayes & Barry, Citation2008) when combined with previous history of invasion success and the numbers of released individuals. The approach is sensitive to both climate parameterization and the statistical methods used in evaluating the likelihood of the climates being comparable between the home range and the new range being evaluated for expansion (Rodda et al., Citation2011). For example, Rodda et al. (Citation2009) predicted a possible range expansion encompassing the southern tier of the US from the Delmarva Peninsula in the east to portions of California in the west, while Pyron et al. (Citation2008) identified only southern portions of Florida and Texas as suitable for expansion. Both approaches used mean monthly minimum and maximum air temperatures at large spatial scales as part of the parameterization of the climate-matching modeling.
Climate matching does not account for the effect of weather on the body temperature of an organism nor does it directly couple the energy environment resulting from weather to the organism as modified by thermal behavior. As a result, there is no way to mechanistically determine what aspects of a climate preclude site occupancy in a landscape. This can be accomplished by calculating an energy budget for an organism applying physical mechanisms of energy exchange between an organism and its environment.
There are four fundamental physical mechanisms, derived from first principles, used to estimate the rates of energy exchange (fluxes) between an organism and its environment: radiation, latent heat, conduction, and sensible heat transfer (Montieth & Unsworth, Citation2013). To elucidate; radiation is energy transmitted as light and is associated with sunlight but has a thermal component in the infrared and longer wavelengths; sensible heat exchange occurs as a result of a state change for example when water evaporates; conduction occurs at an interface between two surfaces, and is moderated by a boundary layer resistance; sensible heat transfer accounts for the energy flows in a medium, as in air or soil, diurnally.
As an example, the magnitude of these four mechanisms, calculated for the average global mean energy budget under contemporary climate conditions results in a net positive energy imbalance of 0.6 W m−2, with solar radiation accounting for 161 W m−2, sensible heat loss corresponding to 20 W m−2, latent heat loss contributing 84 W m−2, and conduction having a net loss of 56 W m−2 (Hartmann et al., Citation2013). It is this energy imbalance that results in the observed increase in surface temperature globally. These same mechanisms are used internationally to predict freeze damage when coupled to organism physiology (Snyder & De Melo-Abreu, Citation2005) as the mechanisms allow for the calculation of an organism’s temperature. Reviews of the application of these four mechanisms to individual animals can be found in Campbell (Citation1977), Campbell and Norman (Citation1998), and Montieth and Unsworth (Citation2013). For poikilotherms, organisms that do not use metabolism to maintain body temperature, it is possible to use this approach to predict when an organism’s body temperature falls outside some empirically determined lethal limit and can be used to identify periods when local weather precludes site occupancy. In contrast, climate match, as a result of the parameters used, can never identify when an organism’s body temperature falls outside a lethal limit and thus can never identify weather events that result in mortality. Additionally, these mechanisms can be coupled to organism behavior on short time scales to evaluate behavioral effects on body temperature.
Thermoregulatory behaviors involve changing body position (Johnson, Citation1972, Citation1973) and the use of particular microclimates to improve heat balance (Pearson, Shine & Williams, Citation2003; Shine & Fitzgerald, Citation1996; Shine & Madsen, Citation1996; Slip & Shine, Citation1988) and to maintain body temperature (Shine, Citation1981; Storey & Storey, Citation1992). The use of burrows by captive pythons during cold spells was reported by Dorcas et al. (Citation2011), and the practice is widely reported in related species (Dorcas & Willson, Citation2011). This phenotypic attribute is set in early developmental stages and may not be plastic, preventing the snake from adapting to extremes in climate variation (Aubret & Shine, Citation2010; Shine, Madsen, Elphick, & Harlow, Citation1997). The sum of thermoregulatory tactics, physiological adaptation to the cold, and behavioral strategies are mechanisms by which pythons may survive exposure to weather extremes.
Within Everglades National Park, pythons prefer saline glades and mangroves (Meshaka et al., Citation2000; Snow et al., Citation2007) and broadleaf and coniferous forest with bordering marsh (Walters, Mazzotti, & Fitz, Citation2016) but avoid marsh and open water. Pythons maintained in semi-natural enclosures in the Upper Coastal Plain of South Carolina containing aquatic, terrestrial, arboreal, and underground refuges exhibited strong seasonal shifts in habitat use, moving from aquatic habitat use in the late summer to terrestrial and underground refuges in the fall and winter (Dorcas et al., Citation2011).
Many species of pythons are reported to shift use of habitat diurnally to moderate body temperature (Avery et al., Citation2010; Dorcas et al., Citation2011; Mazzotti et al., Citation2011; Pearson, Shine, & Williams, Citation2003; Shine & Fitzgerald, Citation1996; Shine & Madsen, Citation1996; Slip & Shine, Citation1988). Pythons are also reported to shift body posture to increase heat exchange with their environment, adopting positions that are outstretched, loosely coiled, tightly coiled, and tightly coiled with the head positioned under the coils (Johnson, Citation1972, Citation1973; Pearson et al., Citation2003). In carpet pythons, Pearson et al. (Citation2003) measured significant changes in body temperature ranging from 24.0°C stretched out, 25.1°C tightly coiled, to 26.1°C loosely coiled. During cold periods in Florida, pythons were often observed coiled along river banks or canal banks (Dorcas & Willson, Citation2011).
We calculated an energy budget to estimate body temperatures of a python basking tightly coiled, and either exposed to ambient climatic conditions on the ground or in a burrow or refuge 30 cm below the soil surface. This modeling exercise allowed us to investigate the utility of adopting a fixed-heat conserving body position as a thermoregulatory behavior in one of two microclimates on body temperature. We chose these scenarios as unusually cold, overcast weather in January 2010 contributed to weather-induced mortality among Burmese pythons in captive and free-ranging populations (Avery et al., Citation2010; Dorcas et al., Citation2011; Mazzotti et al., Citation2011). Avery et al. (Citation2010) and Dorcas et al. (Citation2011) observed captive pythons basking in a coiled body position, a thermal regulatory behavior, at ambient air conditions determined to be lethal near or below 0°C. Additionally, Avery et al. (Citation2010) reported that captive pythons left heated refuges to bask during this cold spell but that behavioral change proved maladaptive.
In calculating the radiation input into the energy budget for a python, we followed the approach of Campbell (Citation1977) and defined short-wave radiation as energy having wavelengths between 300 and 4,000 nm and long-wave radiation having wavelengths between 4,000 and 80,000 nm. Sunlight produces a radiant flux density of 1.36 kW/m2 at the edge of the Earth’s atmosphere (Campbell, Citation1977). During the daylight hours short-wave radiation has a large impact on body temperature due to efficient absorption of this energy associated with the dark coloration of a python above ground. The use of a refuge shifts the energy balance from radiative and conductive fluxes at the surface to sensible heat transfer from the surface to the refuge and then long-wave radiation fluxes from the refuge walls coupled with conduction from the air in the refuge.
We model body temperatures for pythons using temperature data available for Everglades National Park for each day in the months of December 2009 and January 2010 and for the next four December–January periods. We examine ambient air temperature in the context of being a conductive mechanism for energy transfer and demonstrate its impact on body temperature. We summarize this approach over the sequential two month periods to assess the frequency with which low body temperatures occur. Duration of body temperature falling outside a lethal limit and the frequency of occurrence of these events underlie the importance of weather in restricting site occupancy and determine the limitations for python range expansion.
2. Methods
Diurnal fluctuations in the body temperature of a python are calculated using a biophysical energy-flux model from Campbell (Citation1977) and Campbell and Norman (Citation1998). The body temperature of a python results from the energy flux between its body and its environment. The energy budget for the python that allows for the calculation of body temperature is described as follows (Equation (7.19) in Campbell, Citation1977):(1)
(1)
where λE is evaporative heat loss, M is energy produced as a result of metabolism, ρcp is the product of air density and the specific heat capacity of air, Tb is core body temperature, Te is equivalent environmental temperature (corresponding to the energy radiating from a black body cavity at this temperature), rb is whole-body thermal resistance, and re is the sum of parallel resistances at the body surface to radiative heat loss (rr) and convective heat loss (ra). For a poikilotherm, it is assumed that Μ − λΕ = 0. The equation predicting a python’s core body temperature mathematically simplifies to Tb = Te.
The environmental temperature is calculated as (Equation (7.17) in Campbell, Citation1977):(2)
(2)
where εσTa4 is the long-wave radiation energy loss, ε is the surface emissivity, σ is the Stephan-Boltzman constant, and Ta refers to ambient temperature. Ta was calculated from Equations (2.2) and (2.3) in Campbell and Norman (Citation1998) for air temperature. For the soil temperature at 30 cm depth, Ta was calculated from Equation (2.8) in Campbell (Citation1977). Rabs is total energy absorbed from both long-wave and short-wave radiation.
The equation that describes Rabs at night is (Equation (7.11) in Campbell, Citation1977):(3)
(3)
where aL is the long-wave absorptivity of the python, and ε is the average emissivity of the surroundings. This equation includes a short-wave radiation component during the day which has the following form (Equation (7.12) in Campbell, Citation1977):(4)
(4)
The terms Sp and Sd are projected and diffuse short-wave irradiance incident to the snake. The projected short-wave irradiance is calculated as a ratio of the projected surface area of the python normal to the incident radiation divided by the total surface area of the python as a product of the short-wave absorptivity of the python, as. Sp varies throughout the day in a sinusoidal manner following Equations (5.8)–(5.10) in Campbell (Citation1977). Diffuse radiation is direct solar radiation attenuated by clouds, if present, and calculated from Sp using Equation (5.11) in Campbell (Citation1977). As the short-wave radiation term is impacted by the size of an animal, simulations with snakes coiled with circular diameters of 0.092, 0.127, 0.376, and 0.81 m were evaluated.
Monthly summaries of daily weather data (Monthly Climate Data F6 Product) collected by the National Weather Service at the Miami Weather Station (http://w2.weather.gov/climate/index.php?wfo=mfl, Accessed 4/30/2014) were used to parameterize the biophysical model for the scenario where the pythons are lying in a coil, above ground (exposed) for the months of December and January in the years spanning 2009–2014. The daily maximum and minimum air temperatures (Table ), as well as the average daily wind speed and cloud cover, are used to parameterize the model.
Table 1. The monthly mean air and soil temperatures for December or January 2009 to 2014 in the data sets used in the models
Data from the National Resource Conservation Service soil-monitoring network are used to parameterize the second scenario in which pythons are in a burrow 30 cm below the soil surface and is based on depth to ground water at the Everglades station (www.NRCS.USDA.gov; SCAN site FL Everglades). The soil temperature data are measured at a soil depth of 10 cm. The daily maximum and minimum soil temperatures from each day (Table ) and the average difference between these values are used to calculate the temperature amplitude in the soil temperature calculation. Soil moisture, measured at the same location, is used to calculate damping depth for the soil temperature calculation. The soil texture at the monitoring site is loam, the damping depth (the depth at which surface temperature fluctuations will be reduced by a fixed amount) based on soil moisture is set to 0.105 m, following Campbell (Citation1977).
The model calculates snake body temperature at hourly time steps over a 24 h interval for both the above-ground scenario and the burrow scenario for each day in a month. We examine model output for each scenario to record the number of hours in a given 24 h interval that a python’s body temperature is either <5°C or >5°C but <10°C. The average body temperature for each temperature range is also determined. The average number of hours in a month where a python’s body temperature meets one of these two criteria is also determined. The models are programmed in MATLAB (Mathworks, Natick, MA, USA).
3. Results
January (13.2°C) and December (10.6°C) 2010 had the lowest mean daily temperatures over the five-year period examined. To illustrate the effect of extreme cold on predicted body temperature, we present data from January 2010 when mortality in captive pythons was reported by Avery et al. (Citation2010) and in telemetered pythons by Mazzotti et al. (Citation2011). For 9 January 2010, when the recorded high temperature was 17.2°C, the low was 3.3°C, and the sky was cloudy, model-simulation results reveal that air temperature exceeds python body temperature before sunrise (1:00–7:00 h) and after sunset (17:00–24:00 h; Figure ). The air temperatures provide a positive energy flux from conduction. However, the python’s energy balance is dominated by long-wave radiation in the dark, and the snakes are radiating more heat into the environment than they are receiving from it, due in part to the cold night sky. Python body temperature rises above air temperature from 7:00 to 17:00 h due to radiation fluxes and the efficient absorption of short-wave radiation with larger snakes having higher body temperatures because their larger surface area allows for greater absorption of short-wave radiation. On 9 January 2010, there is a 14 h period (body temperatures falling below the horizontal grey line in Figure ) when a python’s body temperature would have been <10°C and at least a 7 h period (body temperatures falling below the horizontal black line in Figure ) when the body temperature would have been close to the lower lethal limit for a python (<5°C; Jacobson et al., Citation2012). This is consistent with Dorcas et al. (Citation2011) reporting mortality at body temperatures between 5 and 10°C in captive pythons.
Figure 1. Hourly air temperature for 9 January 2010 compared to the estimated body temperature of Burmese pythons arranged in a coil of varying dimension exposed above ground to ambient conditions on the same date. Body temperature is dependent on size as the energy balance is dominated by solar short-wave radiation.
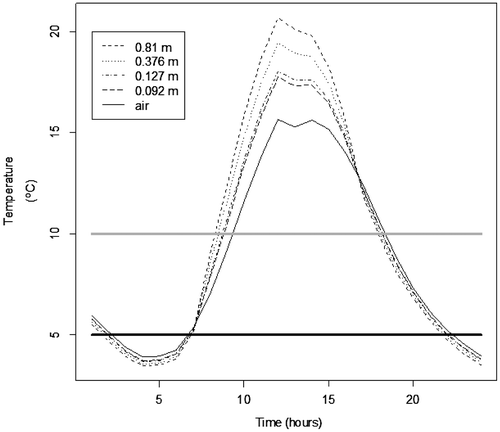
In both January and December 2010, there were four or more days when pythons would have experienced predicted body temperatures <5°C for an average interval greater than four hours/day across all size classes evaluated by us (Table ). Pythons are projected to experience body temperatures >5 and <10°C for one or more days for one or more size classes every month evaluated except for January and December 2013 (Table ). January and December 2010 were the harshest months, with pythons experiencing body temperatures between 5 and 10°C from 12 to 19 days, depending on body size. Pythons with largest body size were projected to experience the largest number of days with these low body temperatures (19 days in December 2010).
Table 2. The number days per month, the average number of hours per day, and the average body temperature of Burmese pythons were estimated to fall between 5 and 10°C for December or January, 2009 to 2014 when the pythons were exposed above ground to ambient climatic conditions
For pythons in a burrow 30 cm below the soil surface, our modeling predicts that body temperature is unaffected by either body size or air temperature on 9 January 2010. The diel fluctuation in body temperature, irrespective of python body size, ranges from a minimum of 10.3°C to a maximum of 10.9°C while air temperature ranges from a minimum of 3.9°C to a maximum of 15.6°C on this date (data not shown). The bioenergetics of an organism in a burrow are dominated by long-wave radiation from the surrounding soil and are not sensitive to surface area. For this particular day, the body temperature predictions of the pythons remain above 10°C. Soils moderate surface-ambient temperature fluctuations, via sensible heat transfer, as illustrated by the daily average air temperature for January 2010 and the corresponding average soil temperature at 30 cm (Figure ).
Figure 2. Daily average air temperature for January 2010 compared to daily soil temperature on the same date at a soil depth of 30 cm.
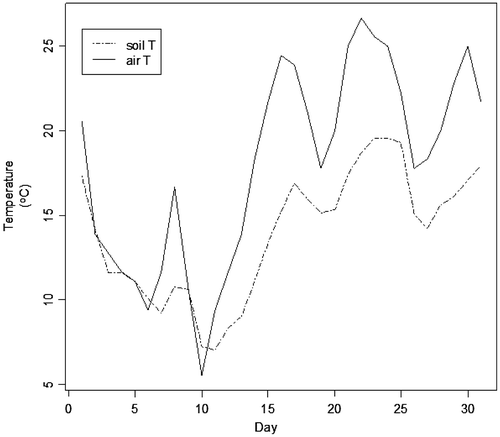
January 2014 was the only month in which python body temperatures are predicted to fall below 5°C in the burrow scenario. It is predicted to have occurred on a single day for a period of 11 h with a mean body temperature of 0.5 ± 0.7°C, irrespective of body size. The month of January 2014 had only 25 days data collected at a soil depth of 10 cm by the monitoring station. Times which predicted python temperatures were >5 and <10°C in the years of 2010, 2013, and 2014 ranged from two to six days/month.
4. Discussion
For pythons above ground, in all size classes, there are extended periods of time when body temperature is predicted to fall below 5 or 10°C. Dorcas et al. (Citation2011) reported minimum body temperatures for 8 out of 10 snakes succumbing at temperatures higher than 4.1°C, predicted by our model to have occurred for 9 January 2010. Mazzotti et al. (Citation2011) also reported mortality in 9 of 10 telemetered pythons in the Everglades with body temperatures falling below 10°C prior to 9 January 2010. Pearson et al. (Citation2003) reported body temperatures as low as 10°C in female carpet pythons in a region at the limit of their known thermal range for portions of the day. The body temperatures calculated by the model are not at or below freezing, and mortality was not likely associated with the formation of ice crystals in inter or intracellular spaces (Storey & Storey, Citation1992). More likely, the inability to generate energy at low temperatures and a corresponding decreased respiration rate and associated hypoxia (Davies & Bennett, Citation1981) are contributing factors to mortality at the body temperatures predicted by the model. Burmese pythons are reported to maintain a low energy metabolism (Dorcas & Willson, Citation2011), and cold may exacerbate this low energy state.
Although a burrow or refuge at a depth of 30 cm is expected to have a more stable temperature, the soil temperatures predicted by the model would result in body temperatures fatal in pythons held in captivity in South Carolina during this time period (Dorcas et al., Citation2011). Moreover, considerable evidence suggests that Burmese pythons are not behaviorally programmed to seek shelter in cooler temperatures, but rather to bask, even in lethally cold air temperatures (Avery et al., Citation2010; Barker, Citation2008; Jacobson et al., Citation2012). This behavioral pattern of leaving a refuge to bask is reported in other python species that experience winter temperatures similar to those observed in Florida (Pearson et al., Citation2003; Shine et al., Citation1997). Mazzotti et al. (Citation2011) reported maximum body temperatures in telemetered pythons above 30°C which they attributed to basking during this cold period.
Basking under extreme cold may reflect the thermal history of the pythons in FL and the current climatic conditions they are experiencing whereby phylogenetic inertia leads to maladaptive behavior in severe cold. Use of a refuge moderates thermal heat loss but does not prevent it. If the objective is to regulate body temperature, then remaining in a refuge as it cools will not be a successful strategy. In the absence of a metabolic means to increase body temperature, increasing exposure to solar radiation by basking is the only option, and adopting a tightly coiled body posture helps maintain body temperature.
Monthly mean maximum and minimum air temperatures are poor predictors of an ectotherm’s body temperature (Tables and ), as the use of microclimates and modifications to body position allows snakes to achieve body temperatures above ambient (Avery et al., Citation2010; Engeman, Avery, & Jacobson, Citation2014; Engeman et al., Citation2011; Jacobson et al., Citation2012). Furthermore, the mean monthly minimum air temperature is 7 to 10°C higher than the lowest modeled average body temperature for pythons in January and December 2010. The importance of this is that weather observed in 2010 is unusual, climatically, in that it is the only year in which body temperatures predicted by the models for snakes above ground were associated with death from exposure, with significant mortality reported during January 2010 in both captive and wild populations (Avery et al., Citation2010; Dorcas et al., Citation2011; Mazzotti et al., Citation2011).
The average temperatures predicted by the models for snakes 30 cm below ground in the Everglades area were not cold enough to kill pythons, in contrast to the conditions reported by Dorcas et al. (Citation2011) and this may be attributed to the difference in depth to ground water, a change in soil texture and soil moisture content. Soils provide a high degree of thermal insulation, and considerable thermal moderation is predicted by our model to occur over the month of January 2010 (Figure ). Soils temperatures lag air temperatures temporally, and short-term extremes in air temperature are buffered with increasing soil depth. Soil temperatures lag air temperature and Dorcas et al. (Citation2011, Figure 4) present trends in air and refuge temperatures illustrating this on a longer time scale in a semi-natural refuge.
Snakes with larger body sizes are predicted to have experienced a larger number of days with low body temperatures in 2010 could have implications for population dynamics. Python size is directly related to breeding potential, with females averaging larger than males (De Vosjoli, Citation1991; Shine et al., Citation1997). Thus, exposed breeding females would be most at risk of cold-induced mortality. As such, a cold spell might not only suppress populations, but could also immediately diminish breeding potential among the surviving population. However, body-size effects may be mitigated by use of other microclimates potentially available in the landscape (Pearson et al., Citation2003).
The role of microclimates as moderate weather may be reflected in the results of aerial surveys conducted by Mazzotti et al. (Citation2011) between 2 January and 4 February 2010, whereby they located a total of 104 non-telemetered pythons, of which 60 were found alive. This result does not represent a survival rate as the surveys were conducted over a month’s time and there is no information available on the total population this subsample represents (Engeman et al., Citation2014). However, in the context of the two scenarios we modeled, the majority (84%) of these pythons were located in artificial habitats identified as levees, canals, and roads (Mazzotti et al., Citation2011). Anthropogenic structures have distinct microclimates that may increase survival, particularly as they are often elevated, drier, and warmer sites in the landscape. Insufficient detail is presented in Mazzotti et al. (Citation2011) to assess how the behaviors associated with use of these anthropogenic structures impacted the energy budgets of the pythons that were detected over this month-long time frame. Based on the results of the model, we would anticipate that access to refuges at depths greater than 30 cm, as might exist in canal banks or levees, would provide a more stable thermal environment and may not have elicited behavioral changes such as basking to increase body temperature during the coldest periods. Extensive use of anthropogenic structures by carpet pythons was reported by Shine and Fitzgerald (Citation1996), with an associated increase in population in a mosaic rural landscape, contrasting with a general population decline in other parts of Australia.
Changes in the energetic physiology of pythons under the temperature extremes observed in Florida in 2010 would likely be required to ensure survival. The generally moderate climate in Florida may preclude opportunities for these attributes to develop (Aubret & Shine, Citation2010). Rapid weather changes, as observed in 2010, may preclude the development of metabolic adaptations, as this requires several weeks in reptiles (Davies & Bennett, Citation1981). Additionally, these temperature changes occurred rapidly enough that changes in body position or microclimate use would not preclude the onset of potentially lethal physiological responses.
An important limitation to using a mechanistic energy budget for the calculation of body temperature in a poikilotherm is that body temperature does not mechanistically determine mortality unless temperatures correspond to those at which freezing of tissues occurs. The body temperatures calculated from a mechanistic energy budget must be compared with those empirically measured in pythons. The critical thermal minimum is especially important in this context and has not been definitively determined for pythons (Avery, Citation1982; Huey, Citation1982; Jacobson et al., Citation2012). The role of frequency of exposure must also be empirically determined as the effects of repeated exposure have not been accessed as compared to continuous exposure. The weather events evaluated over the five-year period by applying a mechanistic bioenergetics model to predict body temperature in pythons demonstrate the rarity of the severely cold weather observed in 2010 in the Everglades and the importance of climate variability as contrasted with an average representation of climate used to predict invasion risk for this species.
5. Conclusion
We use a mechanistic bioenergetics modeling approach to investigate the effect of cold weather conditions during the successive months of December and January from 2009 to 2014 on Burmese python body temperature. The results predict body temperatures for pythons that would result in significant physiological stress for all size classes investigated in at least one month for all years we evaluated except 2013 (Jacobson et al., Citation2012). The model results predict critically low body temperatures across all size classes in 2010 for periods long enough to result in likely death based on minimum body temperatures reported for pythons (Dorcas et al., Citation2011; Mazzotti et al., Citation2011). Our approach is unique in that it is mechanistic and couples the environment to the body temperature of a python and allows for the inclusion of thermoregulatory behaviors exhibited by pythons in the wild. Over the period examined in this study, the bioenergetics approach predicts body temperatures low enough to result in mortality as compared to body temperatures empirically determined in pythons that were reported to have died as a result of exposure. This approach could be applied to other locations to evaluate the possible range expansion of the Burmese python through Florida and into other locations in the contiguous US.
Acknowledgments
We would like to thank Fred Kraus and two anonymous reviewers for their comments and suggestions on earlier versions of this manuscript.
Additional information
Funding
Notes on contributors
Randal S. Stahl
Current research addresses issues focused on understanding the potential for the spread of vertebrate invasive species and developing tools to prevent or mitigate the spread of vertebrate invasive species through human activities. Recent work has examined climate constraints on the survival of the Burmese python in Florida and developing repellents that can be applied to cargo to prevent the transport of the brown treesnake from Guam.
References
- Aubret, F., & Shine, R. (2010). Thermal plasticity in young snakes: how will climate change affect the thermoregulatory tactics of ectotherms? Journal of Experimental Biology, 213, 242–248.10.1242/jeb.035931
- Avery, M. L., Engeman, R. M., Keacher, K. L., Humphrey, J. S., Bruce, W. E., Mathies, T. C., & Mauldin, R. E. (2010). Cold weather and the potential range of invasive Burmese pythons. Biological Invasions, 12, 3649–3652.10.1007/s10530-010-9761-4
- Avery, R. A. (1982). Field studies of body temperatures and thermoregulation. In C. Gans & F. H. Poug (Eds.), Biology of the Reptilia (Vol. 12), (pp. 93–166). New York, NY: Academic Press.
- Barker, D. G. (2008). Will they come in out of the cold? Observations of large constrictors in cool and cold conditions. Bulletin of the Chicago Herpetological Society, 43, 93–97.
- Barker, D. G., & Barker, T. M. (2008a). The distribution of the Burmese python, Python molurus bivittatus. Bulletin of the Chicago Herpetological Society, 43, 33–38.
- Barker, D. G., & Barker, T. M. (2008b). Comments on a flawed herpetological paper and an improper and damaging news release from a government agency. Bulletin of the Chicago Herpetological Society, 43, 45–47.
- Bilger, B. (2009, April 20). Swamp things. The New Yorker, pp. 80–89.
- Bomford, M., Kraus, F., Barry, S. C., & Lawrence, E. (2009). Predicting establishment success for alien reptiles and amphibians: A role for climate matching. Biological Invasions, 11, 713–724.10.1007/s10530-008-9285-3
- Campbell, G. S. (1977). An introduction to environmental biophysics. New York, NY: Springer-Verlag.10.1007/978-1-4684-9917-9
- Campbell, G. S., & Norman, J. M. (1998). An introduction to environmental biophysics (2nd ed.). New York, NY: Springer.10.1007/978-1-4612-1626-1
- Davies, P. M. C., & Bennett, E. L. (1981). Non-acclimatory latitude-dependent metabolic adaptation to temperature in juvenile natricine snakes. Journal of Comparative Physiology ? B, 142, 489–494.10.1007/BF00688980
- De Vosjoli, P. (1991). The general care and maintenance of burmese pythons. Lakeside, CA: Advanced Vivarium Systems.
- Dorcas, M. E., & Willson, J. D. (2011). Invasive pythons in the United States ecology of an introduced predator. Athens GA: The Univerity of Georgia Press.
- Dorcas, M. E., Willson, J. D., & Gibbons, J. W. (2011). Can invasive Burmese pythons inhabit temperate regions of the southeastern United States? Biological Invasions, 13, 793–802.10.1007/s10530-010-9869-6
- Engeman, R. M., Avery, M. L., & Jacobson, E. (2014). Weighing empirical and hypothetical evidence for assessing potential invasive species range limits: A review of the case of Burmese pythons in the USA. Environmental Science and Pollution Research, 21, 11973–11978.10.1007/s11356-014-3173-4
- Engeman, R. M., Jacobson, E., Avery, M. L., & Meshaka, Jr, W. E. (2011). The aggressive invasion of exotic reptiles in Florida with a focus on prominent species: A review. Current Zoology, 57, 599–612.10.1093/czoolo/57.5.599
- Groombridge, B., & Luxmoore, R. (1991) Pythons in south-east Asia. A review of distribution, status, and trade in three selected species. Secretariat of the Convention on International Trade in Endangered Species of Wild Fauna and Flora, Lausanne.
- Hartmann, D. L., Klein Tank, A. M. G., Rusticucci, M., Alexander, L. V., Brönnimann, S., Charabi, Y., … Zhai, P. M. (2013). Observations: Atmosphere and surface. In T. F. Stocker, D. Qin, G.-K. Plattner, M.Tignor, S. K. Allen, J. Boschung, … P. M. Midgley (Eds.), Climate Change 2013: The Physical Science Basis. Contribution of Working Group I to the Fifth Assessment Report of the Intergovernmental Panel on Climate Change (pp. 159–254). Cambridge and New York, NY: Cambridge University Press.
- Hayes, K. R., & Barry, S. (2008). Are there any consistent predictors of invasion success? Biological Invasions, 10, 483–506.10.1007/s10530-007-9146-5
- Huey, R. B. (1982). Temperature, physiology, and the ecology of reptiles. In C. Gans & F. H. Pough (Eds.), Biology of the reptilia (Vol. 12, pp. 25–91). Academic Press.
- Jacobson, E. R., Barker, D. G., Barker, T. M., Mauldin, R., Avery, M. L., Engeman, R., & Secor, S. (2012). Environmental temperatures, physiology and behavior limit the range expansion of invasive Burmese pythons in southeastern USA. Integrative Zoology, 7, 271–285.10.1111/inz.2012.7.issue-3
- Johnson, C. R. (1972). Thermoregulation in pythons—I. Effect of shelter, substrate type and posture on body temperature of the australian carpet python, Morelia spilotes variegata. Comparative Biochemistry and Physiology Part A: Physiology, 43, 271–278.10.1016/0300-9629(72)90185-5
- Johnson, C. R. (1973). Thermoregulation in pythons—II. Head-body temperature differences and thermal preferenda in australian pythons. Comparative Biochemistry and Physiology Part A: Physiology, 45, 1065–1087.10.1016/0300-9629(73)90343-5
- Mazzotti, F. J., Cherkiss, M. S., Hart, K. M., Snow, R. W., Rochford, M. R., Dorcas, M. E., & Reed, R. N. (2011). Cold-induced mortality of invasive Burmese pythons in south Florida. Biological Invasions, 13, 143–151.10.1007/s10530-010-9797-5
- Meshaka, Jr, W. E., Loftus, W., & Steiner, T. (2000). The herptofauna of Everglades National Park. Florida Scientist, 63, 84–103.
- Montieth, J. L., & Unsworth, M. (2013). Principles of environmental physics. Plants, animals and the atmosphere (4th ed.). New York, NY: Academic Press.
- Pearson, D., Shine, R., & Williams, A. (2003). Thermal biology of large snakes in cool climates: a radio-telemetric study of carpet pythons (Morelia spilota imbricata) in south-western Australia. Journal of Thermal Biology, 28, 117–131.10.1016/S0306-4565(02)00048-7
- Pyron, R. A., Burbrink, F. T., & Guiher, T. J. (2008). Claims of Potential Expansion throughout the U.S. by Invasive Python Species Are Contradicted by Ecological Niche Models. PLoS ONE, 3, e2931. doi:10.1371/journal.pone.002931
- Rodda, G. H., Jarnevich, C. S., & Reed, R. N. (2009). What parts of the US mainland are climatically suitable for invasive alien pythons spreading from Everglades National Park? Biological Invasions, 11, 241–252.10.1007/s10530-008-9228-z
- Rodda, G. H., Jarnevich, C. S., & Reed, R. N. (2011). Challenges in identifying sites climatically matched to the native ranges of animal invaders. PLoS ONE, 6, e14670. doi:10.1371/journal.pone.0014670
- Shine, R. (1981). Venomous snakes in cold climates: Ecology of the Australian genus Drysdalia (Serpentes: Elapidae). Copeia, 1981, 14–25.10.2307/1444037
- Shine, R., & Fitzgerald, M. (1996). Large snakes in a mosaic rural landscape: The ecology of carpet pythons Morelia spilota (serpentes: Pythonidae) in coastal eastern Australia. Biological Conservation, 76, 113–122.10.1016/0006-3207(95)00108-5
- Shine, R., & Madsen, T. (1996). Is thermoregulation unimportant for most reptiles? An example using water pythons (Liasis fuscus) in Tropical Australia. Physiological Zoology, 69, 252–269.10.1086/physzool.69.2.30164182
- Shine, R., Madsen, T. R., Elphick, M. J., & Harlow, P. S. (1997). The influence of nest temperatures and maternal brooding on hatchling phenotypes in water pythons. Ecology, 78, 1713–1721.10.1890/0012-9658(1997)078[1713:TIONTA]2.0.CO;2
- Slip, D. J., & Shine, R. (1988). Thermoregulation of free-ranging diamond pythons, Morelia spilota (Serpentes, Boidae). Copeia, 1988, 984–995.10.2307/1445722
- Snow, R. W., Krysko, K. L., Enge, E. M., & Oberhoffer, A. (2007). Introduced populations of Boa constrictor (Boidae) and Python molurus bivittatus (Pythonidae) in southern Florida. In R. W. Henderson & R. Powell (Eds.), The Biology of Boas and Pythons (pp. 416–438). UT: Eagle Mountain.
- Snyder, R. L., & De Melo-Abreu, J. P. (2005). Frost protection: Fundamentals, practice and economics (Vol. 1). Rome: Food and Agriculture Organization of the United Nations.
- Storey, K. B., & Storey, J. M. (1992). Natural freeze tolerance in ectothermic vertebrates. Annual Review of Physiology, 54, 619–637.10.1146/annurev.ph.54.030192.003155
- Walters, T. M., Mazzotti, F. J., & Fitz, H. C. (2016). Habitat selection by the invasive species burmese python in Southern Florida. Journal of Herpetology, 50, 50–56.10.1670/14-098
- Whitaker, R., & Captain, A. (2004). Snakes of India: The field guide. Chengapattu: Draco Books.
- Willson, J. D., Dorcas, M. E., & Snow, R. W. (2011). Identifying plausible scenarios for the establishment of invasive Burmese pythons (Python molurus) in Southern Florida. Biological Invasions, 13, 1493–1504.10.1007/s10530-010-9908-3
- Zhao, E., & Alder, K. (1993). Herpetology of China. St Louis, MO: Society for the Study of Amphibians and Reptiles.