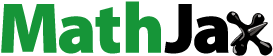
Abstract
Seed quality is one of the most important factors for stand establishment in cotton (Gossypium sp.), and the use of good quality seeds is therefore essential to obtain an optimum plant population. Conditions prevailing during seed formation can affect the quality of seed produced, and hence crop establishment in the next growing season. These conditions can affect the germination of the seeds and the ability of the seedlings to emerge from soil, these being the most critical stages during the life cycle of cotton plant. Field experiments were conducted to investigate the effect of nitrogen (N), phosphorus (P), potassium (K), foliar application of zinc (Zn) and calcium (Ca), the use of plant growth retardants (PGR’s) [e.g. 1,1-dimethyl piperidinium chloride (MC); 2-chloroethyl trimethyl ammonium chloride (CC); or succinic acid 2,2-dimethyl hydrazide (SADH)], during square initiation and boll setting stage, on growth, seed yield, seed viability, and seedling vigor of cotton.
Public Interest Statement
Stand establishment of cotton seedlings is one of the most critical stages in cotton production. Cotton-seed quality is affected, to a large extent, by the indeterminate growth habit of the cotton plant, which allows seed to set and develop across an extended period of time. Seed vigor and viability are important components influencing seedling establishment, crop growth, and productivity. Any factor that negatively affects seed vigor and viability during seed development will have adverse consequences on crop production. Plant nutrition using a balanced fertilization programmer with both macro- and micro-nutrients has become very important in the production of high quality seed. Plant growth retardants (PGR’s) represent diverse chemistries and mode of action, and provide numerous possibilities for altering crop growth and development, provide farmers with a new management tool for controlling undesirable vegetative growth, and to balance vegetative and reproductive growth as well as to improve yield and its quality.
Competing Interests
The author declare no competing interests.
1. Introduction
Sowing is a critical time in the life cycle of any crop and the seeds are frequently exposed to adverse conditions that may compromise the establishment of seedlings in the field (de Figueiredo e Albuquerque, Citation2003). Stand establishment of cotton seedlings is one of the most critical stages in cotton production. Cotton-seed quality is affected, to a large extent, by the indeterminate growth habit of the cotton plant, which allows seed to set and develop across an extended period of time. Seed vigor and viability are important components influencing seedling establishment, crop growth, and productivity. Any factor (biotic and/or environmental) that negatively affects seed vigor and viability during seed development will have adverse consequences on crop production, especially when seeds are sown under environmentally stressful conditions (Welch, Citation1995). Both size and number of seeds, produced by maternal plants, are most likely determined by their nutritional status at the time of flowering and bud initiation. Furthermore, the most important single determinant of mineral nutrient reserves in seeds is the mineral nutrient availability to the maternal plant during reproductive development, with increasing supplies of a particular mineral nutrient enhancing the nutrient concentration in the mature seed (Fenner, Citation1992).
Plant nutrition using a balanced fertilization programmer with both macro- and micro-nutrients has become very important in the production of high quality seed. Many management practices and breeding efforts have allowed plants to partition more carbohydrates into bolls and less into vegetative growth. Mineral nutritional status of plants has a considerable impact on partitioning of carbohydrates and dry matter between shoots and roots. Often, the number of sink organs is the yield component that is affected mostly by mineral nutrients. The positive effect of mineral nutrient supply on the number of sink organs may result not only from an increase in mineral nutrient supply, but also from an increase in photosynthate supply to the sink sites or from hormonal effects (Borowski, Citation2001).
1.1. Nitrogen
In cotton culture, N have the most necessity role in production inputs, which controls growth and prevents abscission of squares and bolls, essential for photosynthetic activity (Reddy, Reddy, Padjung, & Hodges, Citation1996), and stimulates the mobilization and accumulation of metabolites in newly developed bolls, thus increasing their number and weight. Additionally, with a dynamic crop like cotton, excess N serves to delay maturity, promote vegetative tendencies, and usually results in lower yields (Rinehardt, Edmisten, Wells, & Faircloth, Citation2004). Therefore, errors made in N management that can impact the crop can be through either deficiencies or excesses. With a dynamic crop like cotton, excess N serves to delay maturity, promote vegetative tendencies, and usually results in lower yields (McConnell, Baker, & Frizzell, Citation1996; Rinehardt et al., Citation2004). Therefore, errors made in N management that can impact the crop can be through either deficiencies or excesses. If an N deficiency is developing in a cotton crop, it is not particularly difficult to diagnose and correct. Excess N fertility levels, which, can be damaging to final crop productivity, are subtler to detect, and are difficult to correct (Silvertooth & Norton, Citation1997).
1.2. Phosphorus
Phosphorus (P) is the second most limiting nutrient in cotton production after nitrogen Response to P fertilizer, however, is often difficult to predict, even with soil test-based applications (Bronson, Onken, Keeling, Booker, & Torbert, Citation2001). The high soil pH (>7.6) and the high quantities of CaCO3 result in precipitation of P, which reduces the soluble P supply. Its deficiency tends to limit the growth of cotton plants, especially when plants are deprived of phosphorus at early stages than later stages of growth (Hearn, Citation1981). P is also involved in cell division and development of meristematic tissues (Russell, Citation1973). Moreover, on a whole-plant scale, P plays a decisive role in carbon assimilate transport and metabolic regulation (Bisson, Cretenet, & Jallas, Citation1994). Phosphorus deficiencies lead to a reduction in the rate of leaf expansion and photosynthesis per unit leaf area (Rodríguez et al., Citation1998). The high soil pH (>7.6) and the high quantities of CaCO3 result in precipitation of P, which reduces the soluble P supply. Sasthri, Thiagarajan, Srimathi, Malarkodi, and Venkatasalam (Citation2001) found that application of 2% diammonium phosphate to cotton plants increased seed yield, seed germination, root length, vigor index, and dry matter production.
1.3. Potassium
The physiological role of K during fruit formation and maturation periods is mainly expressed in carbohydrate metabolism and translocation of metabolites from leaves and other vegetative organs to developing bolls. K increases the photosynthetic rates of crop leaves, CO2 assimilation and facilitating carbon movement (Sangakkara, Frehner, & Nosberger, Citation2000). At least 60 enzymes are known to be activated by this ion. The enzyme pyruvate kinas (more correctly referred to as ATP: pyruvate phosphotransferase), which participates in glycolysis (Glass ADM: Plant Nutrition, Citation1989). The high concentration of K+ is thought to be essential for normal protein synthesis. Potassium role in this process is considered to be the maintenance of a proper association between t RNA molecules and ribosomes during the translation of mRNA (Glass ADM: Plant Nutrition, Citation1989). Potassium also acts as an activator for several enzymes involved in carbohydrates metabolism. The requirement of cotton for K increases with the beginning of bud formation stage. A greater accumulation of sugars and starch in leaves under K-deficient conditions adversely affects development of bolls due to deficiency of metabolites. K deficiency during the reproductive period can limit the accumulation of crop biomass (Colomb, Bouniols, & Delpech, Citation1995), markedly changes the structure of fruit-bearing organs, and decreases yield and quality. Pettigrew (Citation1999) stated that the elevated carbohydrate concentrations remaining in source tissue, such as leaves, appear to be part of the overall effect of K deficiency in reducing the amount of photosynthate available for reproductive sinks and thereby producing the changes in yield and quality seen in cotton.
1.4. Calcium
Ca is essential in cell nucleus matrix. It activates enzymes, particularly those that are membrane-bound (Rensing & Cornelius, Citation1980). Calcium is important in membrane permeability, maintenance of cell integrity, and in ion uptake. Calcium deficiency may also decrease the basipetal transport of auxin (dela Fuente & Leopold, Citation1973). Addicott and Lyon (Citation1973) listed Ca deficiency as one of the causes of abscission and suggested this plus the role of Ca in the middle lamella (Ca pectates) as the possible reason. It is thought that Ca is important in the formation of cell membranes and lipid structures. Ma and Sun (Citation1997), suggested that Ca might be involved in light signal transduction chain for phototropism. Ca also plays an important role in plant growth as a major component of the middle lamella (calcium pectate). A likely reason was that Ca deficiency affected translocation of carbohydrates, causing accumulation in the leaves and a decline in stems and roots.
1.5. Zinc
Although only small amounts of Zn are removed from the field by a cotton crop (0.5 oz per bale), Zn is critical for several key enzymes in the plant (Sharma, Sharma, Bisht, & Nautiyal, Citation1982). Zinc influences electron transfer reactions, including those of the Kreb cycle, and thereby affecting the plant’s energy production. Zinc binds tightly to Zn-containing essential metabolites in vegetative tissues, e.g. Zn-activated enzymes such as carbonic anhydrase (Welch, Citation1995). Zn deficiency has been shown to affect growing sink organs; it adversely affects the development and viability of pollen grains (Sharma, Chatterjee, Agarwala, & Sharma, Citation1990). Zinc deficiency occurs on high-pH soils, particularly where topsoil has been removed in preparing fields for irrigation and thereby exposing the Zn-deficient subsoil. Also, Zn deficiencies have occurred where high rates of P are applied. The high P rates in the plant interfere with the utilization of Zn (Oosterhuis, Hake, & Burmester, July Citation1991).
1.6. Plant growth retardants
Plant growth retardants (PGR’s) represent diverse chemistries and mode of action, and provide numerous possibilities for altering crop growth and development (Cothren, Citation1994). PGR’s [e.g. 1,1-dimethyl piperidinium chloride (MC); 2-chloroethyl trimethyl ammonium chloride (CC); or succinic acid 2,2-dimethyl hydrazide (SADH)]. provide farmers with a new management tool for controlling undesirable vegetative growth. An objective for using PGR in cotton is to balance vegetative and reproductive growth as well as to improve yield and its quality (Zhao & Oosterhuis, Citation2000). Visual growth-regulating activity of MC, CC, or SADH is similar (Stuart, Isbell, Wendt, & Abernathy, Citation1984; von Heyendorff-Scheel, Schott, & Rittig, Citation1983), being expressed as reduced plant height and width (shortened stem and branch internodes and leaf petioles), influence leaf chlorophyll concentration, structure and CO2 assimilation, and thicker leaves.
In Egypt, soil fertilization is the primary limiting factor affecting growth and production under intensive land use for two or more crops per year. Furthermore, recently released varieties have high yielding ability, which largely depends on ensuring the plant’s essential nutritional requirements (e.g. N, P, K, Ca; Zn). Considerable interest also exists in using PGR for cotton production because of their potential for altering crop growth and seed development (Cothren, Citation1994). All environmental factors and their interactions that influence plant growth can potentially influence the complicated and dynamic processes that control their seed initiation, development, and seed nutrient reserves. These factors can modify the ultimate vigor and viability of seeds (Sawan, Fahmy, & Yousef, Citation2011). The objectives of this study were to evaluate the effects of N and P, and K fertilization and foliar application of chelated Ca and Zn nutrients, and the PGR’s (e.g. MC, CC, or SADH) during square initiation and boll setting stage and to identify the best combination of these production treatments in order to improve seed yield, seed weight, and seed quality (as measured by seed viability, seedling vigor, and cool germination test) of Egyptian cotton (Gossypium barbadense).
2. Methods and measurements
Field experiments were conducted at the Agricultural Research Center (ARC), in Giza (30°N, 31°: 28′E and 19 m altitude). The soil type was a clay loam with an alluvial substratum. Average textural properties (Kilmer & Alexander, Citation1940) and chemical properties (Chapman & Pratt, Citation1961) of soil in both seasons are reported in Table . Range and mean values of the climatic factors recorded during the growing seasons are presented in Table . These data were obtained from the Agricultural Meteorological Station of the, ARC, Giza, Egypt. No rainfall occurred during the two growing seasons (Sawan, Fahmy, & Yousef, Citation2009). The experiments were arranged as a randomized complete block design in a factorial arrangement. The plot size was 1.95 m. (3 ridges, its ridge was 65 cm width) × 4 m (length), including three ridges (beds). Hills were spaced 25 cm apart on one side of the ridge (16 hills per ridge), and seedlings were thinned to two plants hill−1 6 weeks after sowing (AS), providing plant density of 123,000 plants ha−1. Total irrigation amount during the growing season (surface irrigation) was about 6,000 m3 ha−1. The first irrigation was applied 3 weeks AS, and the second one was 3 weeks later. Thereafter, the plots were irrigated every 2 weeks until the end of the season, thus providing a total of nine irrigations. In every experiment, fertilization along with pest and weed management was applied as needed during the growing season, according to local practices performed at the experimental station.
Table 1. Physical and chemical properties of the soil used in I and II seasons
Table 2. Range and mean values of the weather variables recorded during the growing seasons (April–October)
2.1. Experiments
2.1.1. Influence of N and Foliar-applied PGR’s and Zn on cotton seed yield, viability, and seedling vigor
2.1.1.1. Materials
A field experiment was conducted at the Agricultural Research Center, Ministry of Agriculture in Giza, Egypt using the cotton cultivar Giza 75 (G. barbadense L.) in the two seasons I and II. Each experiment included 16 treatments which were the combinations of:
(1) | Two N rates (farmer rate of 107 kg ha−1 N as control, and a higher rate of 161 kg ha−1 N) applied as ammonium nitrate (MH4NO3, 33.5% N) in two equal doses, six and eight weeks AS. | ||||
(2) | Three PGR’s: (1) 1,1-dimethyl piperidinium chloride (MC); (2) 2-chloroethyl trimethyl ammonium chloride (CC); and (3) succinic acid 2,2-dimethyl hydrazide (SADH). Each was foliar-sprayed once after 75 days after sowing (DAS) (during square initiation and boll formation stages) at a rate of 300 ppm. The volume of solution used for each one was 960 l ha−1. Water alone (0 PGR) was foliar-sprayed at 960 l ha−1 as the control or check treatment. | ||||
(3) | Two Zn rates (0 as control or 50 ppm Zn) in the chelated form (ethylene diamine tetra-acetic acid, EDTA) were applied. Each was foliar-sprayed two times, i.e. 80 and 95 DAS. The volume of solution used for each rate was 960 l ha−1 (Sawan, Gregg, & Yousef, Citation1998). |
2.2.1. Effect of P, Zn, and Ca on cotton seed yield, viability, and seedling vigor
2.2.1.1. Materials
A field experiment was conducted at the ARC in Giza, Egypt, using the cotton cultivar Giza 75 in the two seasons I and II. The experiment included 16 treatments, combinations of:
(1) | Two P rates (44 or 74 kg ha−1 P2O5), were applied (banded into soil) as calcium super phosphate (15% P2O5) three weeks AS, just before the first irrigation. General Farmer practice of applying 44 kg ha−1 P2O5 was used as the control treatment. | ||||
(2) | Two Zn rates (0 as control or 40 ppm Zn) were applied as the chelated form. Each rate was foliar-sprayed twice, at 75 and 90 DAS. Volume of solution applied was 960 l ha−1 (actual Zn applied in each spray was 0 or 38.4 g ha−1), | ||||
(3) | Four Ca rates (0 as control, 20, 40 or 60 ppm Ca) were applied as the chelated form. Each rate was foliar-sprayed twice, at 80 and 95 DAS. Volume of solution applied was 960 l ha−1 (actual Ca applied in each spray was 0, 19.2, 38.4, or 57.6 g ha−1) (Sawan, Gregg, & Yousef, Citation1999). |
2.3.1. Effect of N, K, and PGR’s on cotton growth, seed yield, seed viability, and seedling vigor
2.3.1.1. Materials
A field experiment was conducted at the ARC in Giza, Egypt using the cotton cultivar Giza 86 in the two seasons I and II. The factors studied were N fertilization, foliar application of potassium, and the PGR MC. Two N rates, 95.2 and 142.8 kg N ha−1, were applied as ammonium nitrate at two equal doses, six and eight weeks AS. Each application in the form of side dressing beside each hill was followed immediately by irrigation. Four K rates (0, 0.38, 0.77, 1.15 kg ha−1 K2O) were applied in a volume 960 l ha−1 as K sulfate (K2SO4, “48% K2O”) twice during the reproductive phase. The first application occurred 70 DAS during square initiation and the second at 95 DAS during boll development. The foliar PGR MC was applied twice during reproductive phase, both times delivered in of 960 l H2O ha−1. The first application (0.048 kg a.i. ha−1) occurred 75 DAS and the second (0.024 kg a.i. ha−1) 90 DAS. Control plots received no MC. The K2O and MC were both applied to the leaves with uniform coverage using a knapsack sprayer (Sawan et al., Citation2009).
2.4.1. Effect of K, Zn, and P on seed yield, seed viability, and seedling vigor of cotton
2.4.1.1. Materials
A field experiment was conducted at the ARC in Giza, Egypt using the cotton cultivar Giza 86 in the two seasons I and II. Each experiment included 16 treatments, which were the combinations of two K rates (0.0 and 47 kg ha−1 K), two Zn rates (0.0 or 58 g ha−1 Zn) (0 or 57.6 g of Zn ha−1) and four P rates (0.0, 576, 1,152, and 1,728 g ha−1 P) (0, 576, 1,152, and 1,728 g ha−1 P). The K was applied as K sulfate (K2SO4, “48% K2O”) eight weeks AS (as a band close to the seed ridge) and the application was followed immediately by irrigation. Zn was applied to the foliage in chelated form two times (70 and 85 DAS). Foliar application of P (calcium super phosphate, 15% P2O5) was made twice (80 and 95 DAS). The Zn and P were both applied to the leaves with uniform coverage at a solution volume of 960 l ha−1, using a knapsack sprayer (Sawan et al., Citation2011).
2.4.1.2. Measurements
At harvest, bolls of 10 randomly chosen plants from each plot were harvested (handpicking) and laboratory-ginned to determine seed yield in g per plant. Total seed cotton yield of each plot (including the 10-plant sub-sample) was lab-ginned to determine seed yield in kg ha−1. A random sample of 100 g of seeds from each plot was taken to determine seed weight (weight of 100 seed in g) and to evaluate seed quality in terms for seed viability, cool germination test performance, and seedling vigor (Sawan et al., Citation2009).
2.4.1.3. Seed viability
Germination was evaluated using the International Rules of Seed Testing (International Seed Testing Association, Citation1976) in the Seed Research Unit, Central Administration of Seed, ARC, Giza, Egypt. Aluminum dishes 17 cm in diameter and 3 cm deep were used, and the sand substratum was sieved/washed/sterilized and kept moistened to 50% of water-holding capacity. Fifty seeds were planted in each dish in sand depressions made with a standard puncher and then covered with a top layer of 2 cm of loose moist sand. Each of the four replicates of each treatment included two dishes for each replicate. Dishes were then incubated at 30 ± 1°C for 12 days. The following parameters were measured:
(1) | First germination count (germination velocity): percentage of seeds that sprouted after four days of incubation. | ||||
(2) | Second germination count: percentage of seeds that sprouted after eight days of incubation. This count was used to calculate germination rate index (GRI). | ||||
(3) | Total germination capacity (final count): total percentage of normal seedlings after 12 days of incubation. | ||||
(4) | GRI: was calculated according to Bartlett (Citation1937) as follows: |
where n = 3 is the number of times counts were taken (Sawan et al., Citation2009).
2.4.1.4. Cool germination test performance
In this test, the germination chamber was maintained at a constant temperature of 18 ± 1°C with sufficient humidity to prevent drying of the paper towel substratum (Bird & Reyes, Citation1967). Two hundred seeds (four replicates of 50 seeds each) were tested per field treatment. Four paper towels represented each of the four replicates of each treatment. In each of the four replicates, the 50 seeds were randomly placed on moist towels, as usually practiced in the standard germination test. Two towels were placed over the seeds before rolling. The towels were moistened, but not so wet that by pressing, a film of water formed around the finger. Rolled towel tests were then set upright in wire mesh baskets in the germinator. Additional moisture was not needed during the test period. Two counts of germination were made for germination on the fourth and seventh day under test conditions (Sawan et al., Citation2009).
2.4.1.5. Seedling vigor
Aluminum dishes, similar to those described regarding the seed viability test, were used to evaluate seedling vigor. Two dishes represented each of the four replicates of each treatment; in each dish, 50 seeds were planted. Ten seedlings were randomly taken from each dish after eight days of incubation at 30 ± 1°C to measure the following seedling vigor characters: (1) length (in cm) of hypocotyl, radicle, and entire seedling; and (2) fresh and dry weights (g) of 10 seedlings. The 10 seedlings were weighed immediately to record fresh weight, and then oven-dried for 72 h at 85°C to determine dry weight (Sawan et al., Citation2009).
2.4.1.6. Statistical analysis
Data for the studied characters observed were analyzed as a factorial experiment arranged in a randomized complete block design, and combined statistical analysis for the two years had been done, according to Snedecor and Cochran (Citation1980). The Least Significant Difference (LSD) test (t-test) at the 0.05 significance level was used to examine differences among treatment means (Sawan et al., Citation2009).
3. Analyzed data for measurements
3.1. Experiments
3.1.1. Influence of N and foliar-applied PGR and Zn on cotton seed yield, viability, and seedling vigor
3.1.1.1. Seed yield
Seed yield plant−1 and yield ha−1 significantly increased by raising the N-rate in both years (Table ) (Sawan et al., Citation1998). Nitrogen is an important nutrient which control growth and prevents abscission of squares and bolls, essential for photosynthetic activity (Reddy et al., Citation1996) and stimulate the mobilization and accumulation of metabolites in newly developed bolls and thus their number and weight are increased. Abdel-Malak, Radwan, and Baslious (Citation1997) stated that cotton yield was higher when N was applied at a rate of 190 kg ha−1 than at the rate of 143 kg ha−1. Palomo Gil and Chávez González (Citation1997) applied N at a rate ranging from 40 to 200 kg ha−1 to cotton plants and found highest yield was associated with high rates of applied N. Similar results were obtained by Sarwar Cheema, Akhtar, and Nasarullah (Citation2009) and Saleem, Bilal, Awais, Shahid, and Anjum (Citation2010) when N was applied at 120 kg ha−1. In both years, all three PGR increased seed yield plant−1 and ha−1, compared to untreated control. In I season, only MC and CC produced statistically significant increases, while in II season, increases were significant with all tested PGR. The highest numerically increase in seed yield was with MC, followed in order by CC, and SADH. Such increases may be due to increased photosynthetic activity of leaves when these substances are applied. Increased photosynthesis greatly increased flowering, boll retention, and boll weight (Kler, Raj, & Dhillon, Citation1989; Mauney, Fry, & Guinn, Citation1978). Abdel-Al (Citation1998) indicated that cotton yield significantly increased with MC treatment at a rate 11.90 ml (formulation) ha−1 at the beginning of flowering. Pípolo, Athayde, Pípolo, and Parducci (Citation1993) found that spraying cotton plants at an age of 70 d after emergence with CC at rates ranging from 25 to 100 g ha−1 resulted in yield increases. Sawan and Gregg (Citation1993) stated that application of CC and SADH, at rates ranging from 250 to 700 ppm 105 DAS increased cotton seed yield ha−1. Similar results were obtained by Sarwar Cheema et al. (Citation2009). Zinc application significantly increased seed yield plant−1 and ha−1 in both years, as compared with untreated plants. Zinc is required in the synthesis of tryptophan, which is a precursor of IAA synthesis which is the hormone that inhibits abscission of squares and bolls. Also, this nutrient has favorable effect on the photosynthetic activity of leaves and plant metabolism (Li, Ma, Wang, & Tai, Citation2004), which might account for higher accumulation of metabolites in reproductive organs (bolls). Similar results were obtained by Basilious, Abdel Malak, and Abdel Kader (Citation1991), by foliar spraying of 0.314 kg ha−1 Zn at flowering stage, Gomaa (Gomaa, Citation1991) when cotton was sprayed with 0.4 kg ha−1 ZnSO4. Zeng (Citation1996) stated that application of Zn to cotton plants on calcareous soil increased yield by 7.8–25.7% and Ibrahim, Bekheta, El-Moursi, and Gaafar (Citation2009).
Table 3. Effect of N-rate and foliar application of plant growth retardants and Zn on seed yield plant−1 and seed yield ha−1, and seed weight
3.1.1.2. Seed weight
Seed weight significantly increased by adding the high N-rate in both years (Table ). This may be partially due to enhanced photosynthetic activity (Abdel-Malak et al., Citation1997). Similar findings were obtained by Palomo Gil and Chávez González (Citation1997). Application of PGR increased seed weight over the untreated control in both years. The increase was significant for MC and CC in season I. In season II, the increase was significant for all the three PGR’s. Spraying plants with MC produced the highest numerically seed weight. Increased seed weight as a result of MC, CC, or SADH applications may be due to increase in photosynthetic activity, which stimulates photosynthetic activity and dry matter accumulation, and in turn increases formation of fully mature seed and increases seed weight. These agree with previous works of Kler et al. (Citation1989) when CC was applied at 58 ppm after 75 DAS, Sawan and Sakr (Citation1990) when MC was applied at 10–100 ppm once after 90 D or twice after 90 and 110 DAS, Sawan and Gregg (Citation1993) when CC or SADH was sprayed at 250, 500 or 750 ppm after 105 DAS. Results are confirmed by those of Carvalho et al. (Citation1994) by applying MC and CC and Abdel-Al (Citation1998), by applying MC. Seed weight significantly increased with Zn application, compared with the control in both years. This may be due to its favorable effects on photosynthetic activity, improving mobilization of photosynthesis and directly affect seed weight. In this connection, Ibrahim et al. (Citation2009) noted that seed weight increased due to the application of Zn.
3.1.1.3. Seed viability, seedling vigor, and cool germination test
Seed viability, seedling vigor, and cool germination test performance significantly increased by addition the high N-rate, as compared to the low rate. Also, the same pattern was true with the application of the three PGR or Zn in both years (Tables and ) (Sawan et al., Citation1998). However, the exceptions of this trend were in case of germination velocity, second germination count, cool germination test performance (four and seven day counts), radicle seedling length and seedling fresh and dry weights, which were not significantly increased when SADH was applied in season I. Results from MC application were numerically higher than from CC or SADH. GRI was not significantly affected in either year by N-rate, PGR, or Zn. The stimulatory effect of raising N-rate on these characters may be attributed to the increase in seed weight. These favorable effects of increased N-rate on seed viability and seedling vigor agreed with the results obtained by Sawan, Maddah El-Din, and Gregg (Citation1989). No information on the residual effects on N-rate on cool germination was noted in the available literature. Beneficial residual effects of PGR on seed viability, seedling vigor, and cool germination test performance may be due to their favorable effects on seed weight. Bartee and Kreig (Citation1974) reported that organic and inorganic materials available to germination seedlings were greater in high-density seed than in low-density seed. Sawan, Basyony, McCuistion, and El-Farra (Citation1993) found that application of CC or SADH (at 250, 500, or 750 ppm as foliar spray after 105 DAS) increased seed viability, seedling vigor, and cool germination test performance. Zinc’s stimulation of seed viability, seedling vigor, and cool germination performance, which are important in stand establishment, may also be associated with increased seed weight and changed composition, as Zn has favorable effects on metabolism of nucleic acids, proteins, vitamins, and growth substances (Vlasynk et al., Citation1978). These are manifested in metabolites formed in plant tissues, and directly influence growth and development processes. Favorable effects of Zn on seed viability and seedling vigor were mentioned by Sawan et al. (Citation1989) when Zn was applied at 12.5 ppm three times as foliar spray after 70, 85, and 100 DAS. No information on residual effects of Zn on cool germination test performance was found. The interaction between N-rate and application of PGR or zinc showed no significant effect on the investigated characters.
Table 4. Effect of N-rate and foliar application of plant growth retardants and Zn on seed viability and cool germination test performance
Table 5. Effect of N-rate and foliar application of plant growth retardants and Zn on seedling vigor
3.2.1. Effect of P, Zn, and Ca on cotton seed yield, viability, and seedling vigor
3.2.1.1. Seed yield
Seed yield plant−1 and plot−1, significantly increased when P was applied at the highest rate with the application of high P-rate in both years (Table ) (Sawan et al., Citation1999). Phosphorus as a constituent of cell nuclei is essential for cell division and development of meristematic tissue, and hence it should have a stimulating effect on the plants, increasing the number of flowers and bolls per plant. Further, P has a well-known impact in photosynthesis as well as synthesis of nucleic acids, proteins, lipids, and other essential compounds (Guinn, Citation1984), all of which are major factors affecting boll weight and consequently cottonseed. These results are confirmed by those of Abdel-Malak et al. (Citation1997), Ibrahim et al. (Citation2009), and Saleem, Shakeel, Bilal, Shahid, and Anjum (Citation2010). Application of Zn significantly increased Seed yield plant−1 and plot−1, as compared with the untreated control. This may be due to its favorable effect on photosynthetic activity, which improves mobilization of photosynthates and directly influences of boll weight (Glass ADM: Plant Nutrition, Citation1989). Also, Zn enhances the activity of tryptophan synthesis, which is involved in the synthesis of the growth control compound IAA, the major hormone that inhibits abscission of squares and bolls. The application of Zn increased the number of retained bolls plant−1. Similar results were obtained by Alikhanova and Tursunov (Citation1988) by application of Zn at 2.5–7.5 kg ha−1, by Sawan et al. (Sawan et al., Citation1989) when cotton was sprayed with Zn at 12.5 ppm and by Gomaa (Citation1991) when cotton was sprayed with 0.952 kg Zn SO4 ha−1. Results are confirmed by those of Zeng (Citation1996) and Ibrahim et al. (Citation2009). The three concentrations of Ca applied significantly exceeded the control (Table ) (Sawan et al., Citation1999). In general, the highest Ca concentration (60 ppm) was better than the other two. The role of Ca in increasing seed yield can possibly be ascribed to its involvement in the process of photosynthesis and the translocation of carbohydrates to young bolls. Calcium deficiency depressed the rate of photosynthesis (rate of CO2 fixation). Guinn (Guinn, Citation1984) stated that Ca deficiency would cause carbohydrates to accumulate in leaves and not in young bolls. The results obtained agree with those reported by Shui and Meng (Citation1990) and Wright et al. (Citation1995).
Table 6. Effect of P-rate and foliar application of Zn and Ca on seed yield plant−1 and seed yield plot−1, and seed weight
3.2.1.2. Seed weight
Application of P at the high rate of 74 kg ha−1 P2O5 and Zn at the concentration of 40 ppm, both significantly increased seed weight relative to the control in the two seasons (Table ) (Sawan et al., Citation1999). A possible explanation for increased seed weight due to the application of P at the higher rate is that this nutrient activated biological reactions in the cotton plants, particularly CO2 fixation and the synthesis of sugar, amino acids, protein, lipids, and other organic compounds. It also increased the translocation of assimilates from photosynthetic organs to the sink (Kosheleva, Bakhnova, Semenova, & Mil’Kevich, Citation1984). Similar results were obtained by El-Debaby, Hammam, and Nagib (Citation1995). Application of Zn significantly increased seed index, compared to the control. This may be due to its favorable effect on photosynthetic activity. Zinc improves mobilization of photosynthates and directly influences boll weight that coincide directly with increased seed index. These results are confirmed by those obtained by Ibrahim et al. (Citation2009). Calcium applied at all rates significantly increased seed index over the control. The highest rate of Ca (60 ppm) showed the highest numerical value of seed index. Similar results were obtained by Ibrahim et al. (Citation2009).
3.2.1.3. Seed viability, seedling vigor, and cool germination test
Seed viability, seedling vigor, and cool germination test performance were generally significantly increased by addition of P at high rate and by application of Zn at 40 ppm and Ca at different concentrations in both years (Tables and ) (Sawan et al., Citation1999). This may be attributed to the fact that P is required for production of high quality seed, since it occurs in coenzymes involved in energy transfer reactions. Energy is tapped in photosynthesis in the form of adenosine triphosphate (ATP) and nicotinamide adenine dinucleotide phosphate (NADP). This energy is then used in photosynthetic fixation of CO2 and in the synthesis of lipids and other essential organic compounds (Taiz & Zeiger, Citation1991). Also, this could be attributed to the increase in total photo assimilates (e.g. lipids) and the translocated assimilates to the sink as a result of applying zinc. The highest Ca-concentration (60 ppm) significantly increased total germination count. Ochiai (Citation1977) notes that Ca2+ can bridge phosphate and carboxylate groups of phospholipids and proteins; that it increases hydrophobicity of membranes; that it generally increases membrane stability and reduces water permeability. Certain hydrolase’s acting on macromolecular substrates (e.g. some @-amylases, phospholipase, and nucleases) requires Ca2+ for activity. Although Ca2+ probably has a structural role rather than catalytic (binds at sites other than catalytic, changing enzyme conformation), it is also possible that macromolecular substrates such as starch might require Ca2+ for bridge complexes (Scott & Peterson, Citation1979). Phosphorus-rates or application of Zn or Ca at different concentrations had no significant effect in either year on GRI.
Table 7. Effect of P-rate and foliar application of Zn and Ca on seed viability and cool germination test performance
Table 8. Effect of P-rate and foliar application of Zn and Ca on seedling vigor
3.3.1. Effect of N, K, and PGR on cotton growth, seed yield, seed viability, and seedling vigor
3.3.1.1. Plant growth and mineral contents
From Table , it follows that there were significant effects under the high N-rate regime (142.8 kg ha−1 N) on growth and nutrient content of cotton plants (105 DAS) compared with the lower rate (95.2 kg ha−1 N) (Sawan et al., Citation2009). These findings coincide with the fact that N is an essential nutrient in building a plant dry matter as well as many energy-rich compounds (ATP), which regulate photosynthesis. Under N deficiency, a considerably larger proportion of dry matter (photosynthates) is partitioned to roots than shoots, leading to reduced shoot/root dry weight ratios (Engels & Marschner, Citation1995). Shrivastava, Tomar, and Singh (Citation1993) found that an increase in N level (from 0 up to 120 kg N ha−1) application caused an increased uptake of N, P, and K. Perumai (Citation1999) stated that when cotton was given 0–120 kg N ha−1, an increase in an N level (from 0 up to 120 kg ha−1 N) significantly increased aboveground biomass production. Bronson et al. (Citation2001) found that petiole NO3-N readings were positively related to N rate when applied up to 112 kg N ha−1. According to the N-status in our experimental soil (Table ), it was classified as medium fertile for N. The K applied at all the three K concentrations (0.38, 0.77, 1.15 kg of K2O ha−1) significantly enhanced growth, N and K uptake of cotton plants as compared to control (0 kg ha−1 K2O). In this connection, (Fan, Yuzhang, and Chaojun (Citation1999) found that K content in petioles and total dry matter production in cotton increased by application of K. Gormus (Citation2002) indicated that the 0 kg ha−1 K2O plots (untreated control) had lower leaf K concentrations compared with the plots with 80, 160, and 240 kg ha−1 K2O. According to the K-status in our experimental soil (Table ), it classified as medium fertile for K. MC significantly increased dry matter yield and N and K uptake of cotton plants compared with plots not treated with MC. Hodges, Reddy, and Reddy (Citation1991) stated that application of MC increased canopy grass photosynthesis of cotton within 48 h, suggesting a direct effect of MC on photosynthesis. Zhao and Oosterhuis (Citation1999) stated that MC application improved leaf photosynthetic rate compared with the untreated control.
Table 9. Mean effects of N-rate and foliar application of potassium and the plant growth MC on dry matter yield and uptake of N and K by cotton plants (season II, sampled 105 days after planting)
3.3.1.2. Seed yield
An increase in N dose from 95.2 to 142.8 kg ha−1 N increased seed yield per plant and per ha (by 13.08 and 13.03%) (Table ) (Sawan et al., Citation2009). There is an optimal relationship between the nitrogen content in the plant and CO2 assimilation, where decreases in CO2 fixation are well documented for N-deficient plants. Nitrogen deficiency is associated with elevated levels of ethylene (which increase boll shedding), suggesting ethylene production in response to N-deficiency stress (Legé, Cothren, & Morgan, Citation1997). N is also an essential nutrient in creating plant dry matter, as well as many energy-rich compounds which regulate photosynthesis and plant production (Wu, Wu, & Xu, Citation1998), thus influencing boll development, increasing the number of bolls plant−1 and boll weight. Similar findings were obtained by McConnell & Mozaffari (Citation2004) when N fertilizer was applied at 120 kg ha−1, and Saleem, Bilal et al., (Citation2010) when N fertilizer was applied at 120 kg ha−1. Also, similar results were obtained by Sarwar Cheema et al. (Citation2009). On the other hand, Boquet (Citation2005) reported that increasing N from 90 to 157 kg ha−1 did not result in increased cotton yield in irrigated or rain-fed cotton. All the three K concentrations (0.38, 0.77, 1.15 kg ha−1 K2O) significantly increased seed yield per plant (by 10.28–16.45%) and per ha (by 10.02–16.26%) compared to the untreated control. This could be attributed to the fact that K significantly enhanced growth and N and K uptake of the plants (Table ) (Sawan et al., Citation2009). These increases could be due to the favorable effects of this nutrient on yield components such as number of opened bolls plant−1, boll weight, or both, leading to higher cotton yield. Zeng (Citation1996) indicated that K fertilizer affects abscission and reduced boll shedding and it certainly affects yield. Pettigrew (Citation1999) stated that, the elevated carbohydrate concentrations remaining in source tissue, such as leaves, appear to be part of the overall effect of K deficiency in reducing the amount of photosynthate available for reproductive sinks and thereby producing changes in boll weight. Cakmak, Hengeler, and Marschner (Citation1994) found that, the K nutrition had pronounced effects on carbohydrate partitioning by affecting either the phloem export of photosynthates (sucrose) or growth rate of sink and/or source organs. Results obtained here confirmed those obtained by Aneela, Muhammad, and Akhtar (Citation2003) when applying 200 kg K2O ha−1, Pervez, Ashraf, and Makhdum (Citation2004) under 62.5, 125, 250 kg K ha ha−1, and Pettigrew, Meredith, and Young (Citation2005) under K fertilizer (112 kg ha−1). MC significantly increased seed yield plant−1 (by 9.68%), and ha−1 (by 9.72%) compared with untreated plants. This could be attributed to the fact that MC significantly controlled new growth and N and K uptake of cotton plants compared to plots not treated with MC (Table ) (Sawan et al., Citation2009). Such increases could be due to the fact that, the application of MC restrict vegetative growth and thus enhance reproductive organs by allowing plants to direct more energy toward the reproductive structure (Pípolo et al., Citation1993). This means that bolls on treated cotton would have a larger photo synthetically supplied sink of carbohydrates and other metabolites than did those on untreated cotton (Wang, Yin, & Sun, Citation1995). Results agreed with those obtained by Ram, Prasad, and Pachauri (Citation2001) when MC was applied at 50 ppm, Mekki (Citation1999) when MC was applied at 100 ppm, and Kumar, Patil, and Chetti (Citation2004). Also, similar results were obtained by Sarwar Cheema et al. (Citation2009).
Table 10. Mean effects of N-rate and foliar application of potassium and the plant growth retardant MC on seed yield plant−1 and seed yield ha−1, and seed weight
3.3.1.3. Seed weight
Seed weight significantly increased with an increase in N from 95.2 to 142.8 kg ha−1 (Table ). This may be due to increased photosynthetic activity, which increases accumulation of metabolites, with direct impact on seed weight (Table ) (Sawan et al., Citation2009). This may be due to increased photosynthetic activity that increases accumulation of metabolites, with direct impact on seed weight. Reddy et al. (Citation1996), in a pot experiment under natural environmental conditions, where 20-day old cotton plants received 0, 0.5, 1.5, or 6 mM NO3, found that, net photosynthetic rates, stomatal conductance and transpiration were positively correlated with leaf N concentration. Similar findings were reported by Palomo Gil, Godoy Avila, and Chávez González (Citation1999), when N was applied at 40–200 kg ha−1, and Ali and El-Sayed (Citation2001), when N was applied at 95–190 kg ha−1. The K application, at all the three concentrations, increased seed weight compared with a control. Results from K application were more effective and significant when applied at the high concentration (1.15 kg ha−1 K2O) than those produced from the low concentration (0.38 kg ha−1 K2O). This may be due to its favorable effects on nutrient uptake, photosynthetic activity, improving its mobilization (Table ) (Sawan et al., Citation2009), which directly influence boll weight and increased seed weight (Pettigrew, Citation1999). Ghourab, Wassel, and Raya (Citation2000) and Ibrahim et al. (Citation2009) reported that, the application of K fertilizer MC significantly increased seed weight as compared to untreated control. Increased seed weight as a result of MC applications may be due to an increase in photosynthetic activity, which stimulates photosynthetic activity, and dry matter accumulation (Bednarz & Oosterhuis, Citation1999; Kumar et al., Citation2004), and in turn increases the formation of fully-mature seed and thus increases seed weight. Similar results to the present study were obtained by Ghourab et al. (Citation2000) and Lamas (Citation2001).
3.3.1.4. Seed viability, seedling vigor, and cool germination test performance
Seed viability, seedling vigor, and cool germination test performance were significantly increased by the high N rate as compared to the low rate and with the application of the three K concentrations and the PGR MC (Tables and ) (Sawan et al., Citation2009). Results from K application were more effective and significant when applied at the high concentration (1.15 kg ha−1 K2O) than those produced from the low concentration (0.38 kg ha−1 K2O) concerning its effect on seed viability and hypocotyl and entire seedling length. The GRI was not significantly affected by N rate, three K concentrations and the PGR MC. Effects of adding the high N-rate, application of potassium at different concentrations and MC on seed viability, seedling vigor and the cool germination test performance, which are important in stand establishment, may be attributed to the higher synthesis of assimilates (Table ) in the recovered leaves which were deviated toward bolls (Sawan et al., Citation2009). This caused an increase in seed weight associated with its changed composition (Taiz & Zeiger, Citation1991; Welch, Citation1995; Wiatrak, Wright, & Marois, Citation2006). These effects are manifested in metabolites formed in plant tissues, which have a direct impact through utilization in growth and development processes. This may be reflected in distinct changes in seed quality and weight. Speed, Krieg, and Jividen (Citation1996) stated that seed density was positively associated with germination capability at 15°C. Gadallah (Citation2000) indicated that viability, germination, and seedling emergence were directly related to seed density for the G. barbadense cultivars. He pointed out that high seed density of all cultivars usually exhibited faster and more uniform rates of radicle emergence than low seed density. Seedlings from heavier seeds had greater accumulation of fresh and dry weight, and quality and vigor indices were also higher than those from light seeds. N is essential for plant protein synthesis including chlorophyll, which is indispensable for photosynthesis and enzymes which act as catalysts in biochemical reactions, and seed reserve proteins (Bisson et al., Citation1994). Maiya, Basave Gowda, Gouda, and Khadi (Citation2001) indicated that the large- and medium-sized seeds (>4.75 mm) recorded higher field emergence (>65%) and produced vigorous seedlings compared to smaller seeds (<4.75 mm). The shoot and root length of seedlings increased with an increase in seed size. Thus, heavier cottonseed has a higher growth potential than lighter seed. Potassium application has favorable effects as an activator of several enzymes involved in carbohydrate metabolism and on the metabolism of nucleic acids, proteins, vitamins, and growth substances (Bednarz & Oosterhuis, Citation1999; Bisson et al., Citation1994). This may be reflected in distinct changes in seed weight and quality. Vasudevan, Virupakshappa, Venugopal, and Bhaskar (Citation1997) studied the response of sunflower to K, P, and Zn along with recommended doses of N, P and K, and found that those nutrients increased the seed-quality parameters such as higher germination after accelerated ageing, higher speed of germination, higher shoot and root lengths, higher vigor index and seedling-growth rate compared with the control. No information on residual effects of K on cool germination test performance was found in the available literature on cotton plants. Beneficial residual effects of MC on seed viability, seedling vigor, and cool germination test performance may be due to their favorable effects on seed weight (Kumar et al., Citation2004). Wang et al. (Citation1995) stated that the application of MC (at 50 or 100 mg kg−1) to the cotton plants at squaring decreased the partitioning of assimilates to the main stem, the branches and their growing points, and increased partitioning to the reproductive organs and roots. Also, they indicated that, from flower to boll setting, MC application was very effective in promoting the partitioning of assimilates into reproductive organs (seeds). Lamas and Athayde (Citation1999) indicated that seedling emergence and seedling dry matter increased with increasing MC rate, when applied at 50, 75, 100, or 125 g ha−1. Significant effects for the main treatments and years were detected, on all studied characters, with one exception for the GRI. Replications within years showed significant effects only on cotton seed yield plant−1 and ha−1, seed weight and first germination count. Boman and Westerman (Citation1994) found that no significant N × MC rate interactions on growth and yield of cotton when the plants received 0 to 227 kg ha−1 N and were sprayed at early flowering with 0, 25, or 50 g ha−1 MC.
Table 11. Mean effects of N-rate and foliar application of potassium and the plant growth retardant MC on seed viability and cool germination test performance
Table 12. Mean effects of N-rate and foliar application of potassium and the plant growth retardant MC on seedling vigor
3.4.1. Effect of K, Zn, and P on seed yield, seed viability, and seedling vigor of cotton
3.4.1.1. Effects of interactions among treatments
There were no significant interactions among K, Zn and P with respect to quantitative and qualitative characters under investigation, except for the following significant interaction effects (Sawan et al., Citation2011):
Table 13. Effect of interaction between K rate and foliar application of Zn on dry matter yield and uptake of K, Zn, and P by cotton plants (season II, sampled 105 days after planting)
Table 14. Effect of interaction between K rate and foliar application of P on chlorophyll and uptake of K and Zn and by cotton plants (season II, sampled 105 days after planting)
Table 15. Effect of interaction between Zn rate and foliar application of P on uptake of K and Zn by cotton plants (season II, sampled 105 days after planting)
Table 16. Effect of interactions between K rate, foliar application of Zn; P on chlorophyll and uptake of Zn by cotton plants (season II, sampled 105 days after planting)
Two factor interactions:
between K and Zn for dry matter yield of cotton plants (shoots) at 105 DAS, as well as for K, Zn, and P uptake (Table );
between K and P for total chlorophyll concentration, as well as for K, and Zn uptake (Table );
between Zn and P for K and Zn uptake (Table ).
Three factor interactions:
between K, Zn, and P for total chlorophyll concentration, as well as for Zn uptake (Table ). Application of the high K rate combined with Zn and or P application increased dry matter yield of cotton plants (shoots) at 105 DAS, as well as total chlorophyll concentration and K, Zn, and P uptake, over that obtained with the high K rate or Zn and or P alone.
3.5.1. Effects of main treatments
3.5.1.1. Plant growth and mineral content
Dry matter yield (shoots) at 105 DAS; total chlorophyll concentration, as well as K, Zn, and P content was determined to study the effect of applied K and foliar application of Zn and P on cotton growth and mineral uptake (Table ). Soil-applied K was associated with higher dry matter yield, higher chlorophyll concentration and higher contents of K, Zn and P (Sawan et al., Citation2011). Hiremath and Hunsigi (Citation1995) found that K application [presumably to soil] was associated with increased total dray matter and increased K concentration in petioles. Gormus (Citation2002) found that K content in petioles and total dry matter production increased by K applied to cotton plants. Gormus (Citation2002) indicated that control plots had lower leaf K concentrations, compared with the other plots, when applying K at the rates of 66.4, 132.8, and 199.2 kg ha−1. Aneela et al. (Citation2003) indicated that the K content significantly increased with increasing fertilizer K levels and was highest at 166 kg ha−1. The P content increased significantly with K application and was highest at 83 kg ha−1. According to the K-status in the experimental soil (Table ) (Sawan et al., Citation2011), it is classified as medium fertile for K. Foliar application of Zn was associated with higher dry matter yield, higher chlorophyll concentration, and higher contents of K, Zn, and P. This stimulation is due to a low level of available Zn in the soil (Table ). Because the pH value of the soil site was more than 2 units higher than 6, applied Zn almost certainly would give a profitable response (Benton, Wolf, & Mills, Citation1991). Cakmak (Citation2000) has speculated that Zn deficiency stress may inhibit the activities of a number of antioxidant enzymes, resulting in extensive oxidative damage to membrane lipids, proteins, chlorophyll, and nucleic acids. Applied at different concentrations significantly enhanced dry matter yield, chlorophyll concentration as well as Zn and K content in cotton plants. The highest increase in dry matter yield was obtained from the highest P application rate (1,728 g ha−1). In chlorophyll biosynthesis, P is required as a pyridoxal phosphate (Ambrose & Easty, Citation1977). The importance of P and Zn nutrition for Egyptian cotton (Sawan et al., Citation2011) was also confirmed by Mahmoud, Abdel Aziz, and Ashoub (Citation1985) who found a significant relationship between Zn uptake and P uptake by plants. This reflects the positive relationship that exists between the two elements in the nutrition of cotton plants. These results can be seen in the sense that both K and Zn are necessary for the biosynthesis of chlorophyll (Amberger, Citation1974). Therefore, the factors responsible for the green color of tissues (NPK and minor-elements) are themselves stimulators for chlorophyll biosynthesis. Data (Table ) also reveal that the uptake of P by cotton plants was increased significantly by the application of K, Zn, and P treatments, individually (Sawan et al., Citation2011). More and Agale (Citation1993) indicated that when applying P to cotton plants in the range of 10.9–32.7 kg P ha−1, plant uptake increased with increasing P fertilization, while total dry matter yield increased with increasing P levels up to 21.8 kg ha−1. Deshpande and Lakhdive (Citation1994) found that P application (10.9–21.8 kg ha−1) increased P uptake and content in stem, leaf, reproductive parts, and seed. Ahmad et al. (Citation2000) pointed out that P deficiency reduced biomass.
Table 17. Mean effects of K and foliar application of Zn and P on dry matter yield, chlorophyll and uptake of K, Zn, and P by cotton plants (season II, sampled 105 days after planting)
3.5.1.2. Seed yield
Seed yield plant−1, as well as plot−1, significantly increased when K was applied (by as much as 14.72, 13.13; 14.69, 13.26%, respectively) in both seasons (Table ) (Sawan et al., Citation2011). K would have a favorable impact on yield components, including number of opened bolls plant−1 and boll weight, leading to a higher cotton yield. Guinn (Citation1985) suggested that growth, flowering, and boll retention decrease when the demand for photosynthate increases and exceeds the supply. The obtained results of total chlorophyll (a; b) confirmed these findings (Table ) (Sawan et al., Citation2011). This means that an increase in photosynthesis should permit more bolls to be set before cutout. Sangakkara et al. (Citation2000) indicated that, K increases the photosynthetic rates of crop leaves, CO2 assimilation and facilitates carbon movement. Also, the role of K suggests that it affects abscission and reduced boll shedding and it certainly affects yield (Zeng, Citation1996). Mullins, Schwab, and Burmester (Citation1999) evaluated cotton yield in relation to long-term surface application of K at 49.8–149.4 kg ha−1 and found that K application increased yield. Results obtained here were similar to those of Aneela et al. (Citation2003), Gormus, (Citation2002), Ibrahim et al. (Citation2009), Pervez et al. (Citation2004), Pettigrew et al., (Citation2005), Sawan, Hafez, Basyony, and Alkassas (Citation2007), and Sawan, Mahmoud, and El-Guibali (Citation2006). Application of Zn significantly increased seed yield plant−1, and seed yield plot−1, as compared with the untreated control (by 10.22, 8.81; 10.07, 8.70%, respectively) in the two seasons (Table ) (Sawan et al., Citation2011). Zn could have a favorable effect on photosynthetic activity of leaves, which improves mobilization of photosynthesis coincidence the increases total chlorophyll (a; b) as shown in Table and directly influences boll weight. Further, Zn is required in the synthesis of tryptophan, a precursor of indole-3-acetic acid, which is the major hormone inhibits abscission of squares and bolls. Thus the number of retained bolls plant−1 and consequently seed yield ha−1 would be increased (Rathinavel & Dharmalingam, Citation2000). Similar results were obtained by Rathinavel and Dharmalingam (Citation2000), by soil application of 50 kg ha−1 ZnSO4, and Ibrahim et al. (Citation2009). Applying P significantly increased seed yield plant−1 and plot−1 in both seasons (Table ), as compared to the untreated plants, when treatment rate was increased from 576 up to 1728 g ha−1 (by 11.21–17.95, 8.31–16.39; 10.83–17.98, 8.03–16.20%, respectively). Generally seed yield plant−1 and plot−1 were the greatest when the highest P-concentration (1728 g ha−1) was applied. Such results reflect the pronounced improvement of yield components due to application of P which is possibly ascribed to its involvement in photosynthesis and translocation of carbohydrates to young bolls (Rodríguez et al., Citation1998). Phosphorus as a constituent of cell nucleus is essential for cell division and the development of meristematic tissue and hence it would have a stimulating effect on increasing the number of flowers and bolls plant−1 (Russell, Citation1973). These results agree with that reported by Katkar, Turkhede, Solanke, Wankhade, and Sakhare (Citation2002) Ibrahim et al. (Citation2009) and Saleem, Shakeel, et al. (Citation2010).
Table 18. Effect of K-rate and foliar application of Zn and P on seed yield plant−1 and seed yield plot−1, and seed weight
3.5.1.3. Seed weight
Seed weight significantly increased with applying K in both years (Table ). A possible explanation for the increased seed weight due to the application of K may be due in part to its favorable effects on photosynthetic activity rate of crop leaves and CO2 assimilation, which improves mobilization of photosynthates and directly influences boll weight which in turn directly affect seed weight (Ghourab et al., Citation2000). Result was similar to those obtained by Sabino, da Silva, and Kondo, (Citation1999); Sawan et al. (Citation2006, Citation2007), i.e. an increase in seed weight due to K application. Application of Zn significantly increased seed weight (Table ) coinciding with the increased total chlorophyll (a; b) (Table ) compared to the control in both seasons. The increased seed weight might be due to an increased photosynthesis activity resulting from the application of Zn (Welch, Citation1995) which improves mobilization of photosynthates and the amount of photosynthate available for reproductive sinks and thereby influences boll weight, factors that coincide with increased in seed weight. A similar result (in seed weight due to Zn application) was obtained by Rathinavel and Dharmalingam (Citation2000). The same pattern was found (Table ) with regard to application of the three P concentrations, which also brought about a significant increment in seed weight over the control in both seasons. This increase was significant for all P concentrations in the first season and for P at 1,152 and 1,728 g ha−1 in the second season. Spraying plants with P at 1,728 g ha−1 produced the highest seed weight. This increased seed weight may be due to the fact that P activated the biological reaction in cotton plant, particularly photosynthesis fixation of CO2 and synthesis of sugar, and other organic compounds (Welch, Citation1995; Wiatrak et al., Citation2006). This indicates that treated cotton bolls had larger photosynthetically supplied sinks for carbohydrates and other metabolites than untreated bolls.
3.5.1.4. Seed viability, seedling vigor, and cool germination test
Seed viability, cool germination test performance, and seedling vigor were significantly increased by application of K (47 kg ha−1), Zn (58 g ha−1) and P (576, 1,152; 1,728 g ha−1), as compared with the untreated plants (Tables and ) (Sawan et al., Citation2011). The differences between the effects of the three P rates were not significant, with the exception of the 1,728 g ha−1 dose, which significantly increased seed viability, seedling vigor and the cool germination test performance as compared with P applied at 576 g ha−1. Application of K, Zn, or P had no effect on GRI. Beneficial residual effects of K addition and application of Zn, and P at different concentrations on stimulating the seed viability, seedling vigor and cool germination test performance may be attributed to their favorable effects on increased seed weight associated with changes in the metabolism of nucleic acids, proteins, vitamins, and growth substances (Sharma et al., Citation1990; Taiz & Zeiger, Citation1991; Welch, Citation1995; Wiatrak, Wright, Marois, Koziara, & Pudelko, Citation2005). At least 60 enzymes are known to be activated by this ion. The enzyme pyruvate kinas (more correctly referred to as ATP: pyruvate phosphotransferase), which participates in glycolysis (Glass ADM: Plant Nutrition, Citation1989). The high concentration of K+ is thought to be essential for normal protein synthesis. Potassium role in this process is considered to be the maintenance of a proper association between t RNA molecules and ribosomes during the translation of mRNA (Glass ADM: Plant Nutrition, Citation1989). Potassium also acts as an activator for several enzymes involved in carbohydrates metabolism. The requirement of cotton for K increases with the beginning of bud formation stage. A greater accumulation of sugars and starch in leaves under K-deficient conditions adversely affects development of bolls due to deficiency of metabolites. The significance of the correlation coefficients between seed weight and the various performance measures are as follows: 0.9487**–0.9893** with seed viability, 0.8912**–0.9437** with seedling vigor; 0.6660**–0.9550** with cool germination test performance. Wang, Yang, Zhou, and Xu (Citation2003) studied the influence of physical characteristics of cotton seed on germination and emergence rates and found that germination rate and seed weight had an extreme positive correlation. Also found that seed weight had a positive correlation with emergence rate.
Table 19. Effect of K-rate and foliar application of Zn and P on seed viability and cool germination test performance
Table 20. Effect of K-rate and foliar application of Zn and P on seedling vigor
4. Conclusion
From the findings of this study, it seems rational to recommended application of N at a rate of 161 of kg ha−1, spraying of cotton plants with plant PGR, and application of Zn in comparison with the ordinary cultural practices adopted by Egyptian cotton producers, it is quite apparent that applications of such PGR, Zn, and increased N fertilization rates could bring about better impact on seed yield and seedling characters studied (Sawan et al., Citation1998).
Under the conditions of this study, it can be concluded that addition of P at 74 kg ha−1 P2O5 and spraying cotton plants with Zn at 40 ppm and also with Ca at 60 ppm can be recommended to improve cotton seed yield, viability, and seedling vigor (Sawan et al., Citation1999).
Application of N at the rate of 142.8 kg ha−1 and application of K (foliar, at the rate of 1.15 kg ha−1 K2O) and MC (at the rate of 0.048 + 0.024 kg ha−1 MC) should help achieve higher cotton seed productivity and quality (seed viability and seedling vigor) in comparison with the usual cultural practices adopted by Egyptian cotton procedures (Sawan et al., Citation2009).
From the findings of this study, the addition of K at 47 kg ha−1, spraying cotton plants with Zn twice (at 57 g ha−1), and also with P twice (especially the P concentration of 1728 g ha−1) along with the soil fertilization used P at sowing time have been proven beneficial to the quality and yield of cotton plants. These combinations appeared to be the most effective treatments, affecting cottonseed productivity and quality (as indicated by better seed viability, seedling vigor, and cool germination test performance) (Sawan et al., Citation2011).
In comparison with the ordinary cultural practices adopted by Egyptian cotton producers, it is apparent that the applications of such treatments could produce an improvement in cottonseed yield and quality.
Additional information
Funding
Notes on contributors
Zakaria M. Sawan
Effect of different rates and application systems of nitrogen fertilization and indole-3-butyric acid on Egyptian cotton growth and yield. Effect of organic fertilizer and micro-nutrient treatments on cotton agronomy and fiber characteristics of Giza 69. Effect of concentration and time of application of the defoliant Harvade on the lint, seed, protein and oil yields, and oil properties of cottonseed. Effect of 1-naphthalene acetic acid and Kinetin on yield and fiber properties, seed, protein, oil, and fatty acids of Egyptian cotton. Plant growth retardants, plant nutrients, and cotton production. Plant density; plant growth retardants: its direct and residual effects on cotton yield and fiber properties. Plant nutrition and plant growth retardants: their effects on cottonseed, protein, oil yields, and oil properties. Direct and Residual affects of Plant Nutrition’s and Plant Growth Retardants, on Cottonseed. Studying the nature relationships between climatic factors and cotton production by different applied statistical methods.
References
- Abdel-Al, M. H. (1998). Response of Giza 85 cotton cultivar to the growth regulators Pix and Atonic. Egyptian Journal of Agricultural Research, 76, 1173–1181.
- Abdel-Malak, K. I., Radwan, F. E., & Baslious, S. I. (1997). Effect of row width, hill spacing and nitrogen levels on seed cotton yield of Giza 83 cotton cultivar. Egyptian Journal of Agricultural Research, 75, 743–752.
- Addicott, F. T., & Lyon, J. L. (1973). Physiological ecology of abscission. In T. T. Kozlowski (Ed.), Shedding of plants parts (pp. 85–124). New York, NY: Academic Press.10.1016/B978-0-12-424250-0.50008-7
- Ahmad, Z., Gill, M. A., Qureshi, R. H., Aslam, M., Iqbal, J., & Nawas, S. (2000). Inter-cultivar variations of phosphorus-deficiency stress tolerance in cotton. Tropical Agricultural Research, 12, 119–127.
- Ali, S. A., & El-Sayed, A. E. (2001). Effect of sowing dates and nitrogen levels on growth, earliness and yield of Egyptian cotton cultivar Giza 88. Egyptian Journal of Agricultural Research, 79, 221–232.
- Alikhanova, O. I., & Tursunov, D. (1988). Efficiency of trace element fertilizers applied to cotton on reclaimed grey-brown stony soils in the Khodzha-Bakirgan massif. Agrokhimiya, 2, 72–77.
- Amberger, A. (1974). Micronutrients, dynamics in the soil and function in plant metabolism. Iron Proceedings Egypt Botanic Social Workshop 1 Cairo, 81–90.
- Ambrose, E. J., & Easty, D. M. (1977). Cell Biology. London: The English Language Book Society and Longman.
- Aneela, S., Muhammad, A., & Akhtar, M. E. (2003). Effect of potash on boll characteristics and seed cotton yield in newly developed highly resistant cotton varieties. Pakistan Journal of Biological Sciences, 6, 813–815.
- Bartee, S. N., & Krieg, D. R. (1974). Cotton seed density: Associated physical and chemical properties of ten cultivars. Agronomy Journal, 66, 433–435.10.2134/agronj1974.00021962006600030028x
- Bartlett, M. S. (1937). Some examples of statistical methods of research in agriculture and applied biology. Supplement to the Journal of the Royal Statistical Society, 4, 137–183.10.2307/2983644
- Basilious, S. I., Abdel Malak, K. K. I., & Abdel Kader, A. E. M. (1991). Response of cotton “Giza 83” to some micronutrients as affected by time of application of nitrogen levels. Assiut Journal of Agricultural Sciences, 22, 351–366.
- Bednarz, C. W., & Oosterhuis, D. M. (1999). Physiological changes associated with potassium deficiency in cotton. Journal of Plant Nutrition, 22, 303–313.10.1080/01904169909365628
- Benton, J. J., Wolf, B., & Mills, H. A. (1991). Plant analysis handbook. Georgia, ATL: Micro-Macro Pub.
- Bird, L. S., & Reyes, A. A. (1967). Effects of cottonseed quality on seed and seedling characteristics. In Proceedings Beltwide Cotton Conferences, 199–206.
- Bisson, P., Cretenet, M., & Jallas, E. (1994). Nitrogen, phosphorus and potassium availability in the soil-physiology of the assimilation and use of these nutrients by the plant. Challenging the Future. In G. A. Constable & N. W. Forrester (Eds.), Proceedings of the World Cotton Research Conference-1, Brisbane Australia, February 14–17 (pp. 115–124). Melbourne: CSIRO.
- Boman, R. K., & Westerman, R. L. (1994). Nitrogen and mepiquat chloride effects on the production of Nonrank, irrigated, short-season cotton. Journal of Production Agriculture, 7, 70–75.10.2134/jpa1994.0070
- Boquet, D. J. (2005). Cotton in ultra-narrow row spacing plant density and nitrogen fertilizer rates. Agronomy Journal, 97, 279–287.10.2134/agronj2005.0279
- Borowski, E. (2001). The effect of nitrogenous compounds on the growth, photosynthesis and phosphorus uptake of sunflowers. Annales Universitatis Mariae Curie-Sklodowska. Sectio EEE Horticultura, 9, 23–31.
- Bronson, K. F., Onken, A. B., Keeling, J. W., Booker, J. D., & Torbert, H. A. (2001). Nitrogen response in cotton as affected by tillage system and irrigation level. Soil Science Society of America Journal, 65, 1153–1163.10.2136/sssaj2001.6541153x
- Cakmak, I. (2000). Possible roles of zinc in protecting plant cells from damage by reactive oxygen species. New Phytologist, 146, 185–205.10.1046/j.1469-8137.2000.00630.x
- Cakmak, I., Hengeler, C., & Marschner, H. (1994). Changes in phloem export of sucrose in leaves in response to phosphorus, potassium and magnesium deficiency in bean plants. Journal of Experimental Botany, 45, 1251–1257.10.1093/jxb/45.9.1251
- Carvalho, L. H., Chiavegato, E. J., Cia, E., Kondo, J. I., Sabino, J. C., Pettinelli Júnior, A., ... Gallo, P. B. (1994). Plant growth regulators and pruning in the cotton crop. Bragantia, 53, 247–254.10.1590/S0006-87051994000200014
- Chapman, H. D., & Pratt, P. F. (1961). Methods of analysis for soils, plants and waters (pp. 60–61 and 150–179). Los Angeles, CA: University of California, Division of Agricultural Science.
- Colomb, B., Bouniols, A., & Delpech, C. (1995). Effect of various phosphorus availabilities on radiation-use efficiency in sunflower biomass until anthesis. Journal of Plant Nutrition, 18, 1649–1658.10.1080/01904169509365010
- Cothren, J. T. (1994). Use of growth regulators in cotton production. Challenging the Future. In G. A. Constable & N. W. Forrester (Eds.), Proceedings of the World Cotton Research Conference-1, Brisbane Australia, February 14–17 (pp. 6–24). Melbourne: CSIRO.
- Deshpande, R. M., & Lakhdive, B. A. (1994). Effect of plant growth substances and phosphorus levels on yield and phosphorus uptake by cotton. PKV Research Journal, 18, 118–121.
- de Figueiredo e Albuquerque, M. C. (2003). Effect of the type of environmental stress on the emergence of sunflower (Helianthus annuus L.), soybean (Glycine max (L.) Merril) and maize (Zea mays L.) seeds with different levels of vigor. Seed Science and Technology, 31, 465–479.10.15258/sst
- dela Fuente, R. K., & Leopold, A. C. (1973). A role for calcium in Auxin transport. Plant Physiology, 51, 845–847.10.1104/pp.51.5.845
- El-Debaby, A. S., Hammam, G. Y., & Nagib, M. A. (1995). Effect of planting date, N and P application levels on seed index, lint percentage and technological characters of Giza 80 cotton cultivar. Annals of Agricultural Science, Moshtohor, 33, 455–464.
- Engels, C., & Marschner, H. (1995). Plant uptake and utilization of nitrogen. In P. E. Bacan (Ed.), Nitrogen fertilization in the environment (pp. 41–81). New York, NY: Marcel Dekker.
- Fan, S., Yuzhang, X., & Chaojun, Z. (1999). Effects of nitrogen, phosphorus and potassium on the development of cotton bolls in summer. Acta Gossypii Sinica, 11, 24–30.
- Fenner, M. (1992). Environmental influences on seed size and composition. Horticultural Reviews, 13, 183–213.
- Gadallah, F. M. (2000). Seed density in relation to germination and seedling quality in cotton (Gossypium barbadense L.). Alexandria Journal of Agricultural Research, 45, 119–137.
- Ghourab, M. H. H., Wassel, O. M. M., & Raya, N. A. A. (2000). Response of cotton plants to foliar application of (Pottasin-P)TM under two levels of nitrogen fertilizer. Egyptian Journal of Agricultural Research, 78, 781–793.
- Glass ADM: Plant Nutrition. (1989). An introduction to current concepts. Boston, MA: Jones and Bartlett.
- Gomaa, M. A. (1991). Response of cotton plant to phosphatic and zinc fertilization. Annals of Agricultural Science, Moshtohor, Egypt, 29, 1051–1061.
- Gormus, O. (2002). Effects of rate and time of potassium application on cotton yield and quality in Turkey. Journal of Agronomy and Crop Science, 188, 382–388.10.1046/j.1439-037X.2002.00583.x
- Guinn, G. (1984). Boll abscission in cotton. In U. S. Gupta (Ed.), Crop physiology: Advancing frontiers (pp. 177–225). New Delhi: Oxford & IBH.
- Guinn, G. (1985). Fruiting of cotton. III. Nutritional stress and cutout. Crop Science, 25, 981–985.10.2135/cropsci1985.0011183X002500060020x
- Hearn, A. B. (1981). Cotton nutrition. Field Crop Abstracts, 34, 11–34.
- Hiremath, G. M., & Hunsigi, G. (1995). Effect of nitrogen and potash levels on concentration of nitrogen and potassium in petiole of two cotton hybrids (Gossypium sp.). Karnataka. Karnataka Journal of Agricultural Science, 8, 99–101.
- Hodges, H. F., Reddy, V. R., & Reddy, K. R. (1991). Mepiquat chloride and temperature effects on photosynthesis and respiration of fruiting cotton. Crop Science, 31, 1302–1308.10.2135/cropsci1991.0011183X003100050044x
- Ibrahim, M. E., Bekheta, M. A., El-Moursi, A., & Gaafar, N. A. (2009). Effect of arginine, prohexadione-Ca, some macro and micro-nutrients on growth, yield and fiber quality of cotton plants. World Journal of Agricultural Sciences, 5, 863–870.
- International Seed Testing Association. (1976). International rules for seed testing. Seed Science and Technology, 4, 51–177.
- Katkar, R. N., Turkhede, A. B., Solanke, V. M., Wankhade, S. T., & Sakhare, B. A. (2002). Effect of foliar sprays of nutrients and chemicals on yield and quality of cotton under rainfed condition. Research Crops, 3, 27–29.
- Kilmer, V. J., & Alexander, L. T. (1940). Methods of making mechanical analysis of soils. Soil Science, 68, 15.
- Kler, D. S., Raj, D., & Dhillon, G. S. (1989). Modification of micro-environment with cotton canopy for reduced abscission and increased seed yield. Environment and Ecology, 7, 800–802.
- Kosheleva, L. L., Bakhnova, K. V., Semenova, T. A., & Mil’Kevich, Z. A. (1984). Effect of phosphorus nutrition on metabolism of young fiber flax plants in relation to assimilate distribution in them. Referativnyi Zhurnal, 6, 533.
- Kumar, K. A. K., Patil, B. C., & Chetti, M. B. (2004). Effect of plant growth regulators on biophysical, biochemical parameters and yield of hybrid cotton. Karnataka Journal of Agricultural Science, 16, 591–594.
- Lamas, F. M. (2001). Comparative study of mepiquat chloride and chlormequat chloride application in cotton. Pesquisa Agropecuária Brasileira, 36, 265–272.10.1590/S0100-204X2001000200008
- Lamas, F. M., & Athayde, M. L. F. (1999). Effect of mepiquat chloride and thidiazuron on some characteristics of cotton seed. Pesquisa Agropecuária Brasileira, 34, 2015–2019.10.1590/S0100-204X1999001100006
- Legé, K. E., Cothren, J. T., & Morgan, P. W. (1997). Nitrogen fertility and leaf age effects on ethylene production of cotton in a controlled environment. Plant Growth Regulation, 22, 23–28.10.1023/A:1005854625641
- Li, L.-L., Ma, Z.-B., Wang, W.-L., & Tai, G.-Q. (2004). Effect of spraying nitrogen and zinc at seedling stage on some physiological characteristics and yield of summer cotton. Journal of Henan Agricultural University, 38, 33–35.
- Ma, L.-G., & Sun, D.-Y. (1997). The involvement of calcium in the light signal transduction chain for phototropism in sunflower seedling. Biologia Plantarum, 39, 569–574.
- Mahmoud, M. H., Abdel Aziz, I., & Ashoub, M. A. (1985). The relationship between phosphorous and zinc and cotton plant. Annals Agricultural Science, Faculty of Agriculture, Ain Shams Univeristy, Cairo, Egypt, 30, 1011–1030.
- Maiya, M. R., Gowda, B., Gouda, M. S., & Khadi, B. M. (2001). Effect of size grading on recovery and quality in naturally coloured cotton. Seed Research, 29, 248–250.
- Mauney, J. R., Fry, K. E., & Guinn, G. (1978). Relationship of photosynthetic rate to growth and fruiting of cotton, soybean, sorghum and sunflower. Crop Science, 18, 259–263.10.2135/cropsci1978.0011183X001800020016x
- McConnell, J. S., Baker, W. H., & Frizzell, B. S. (1996). Distribution of residual nitrate-N in longterm fertilization studies of an alfisol cropped for cotton. Journal of Environment Quality, 25, 1389–1394.10.2134/jeq1996.00472425002500060032x
- McConnell, J. S., & Mozaffari, M. (2004). Yield, petiole nitrate, and node development responses of cotton to early season nitrogen fertilization. Journal of Plant Nutrition, 27, 1183–1197.
- Mekki, B. B. (1999). Effect of mepiquat chloride on growth, yield and fiber properties of some Egyptian cotton cultivars. Arab Universities Journal of Agricultural Science, 7, 455–466.
- More, S. D., & Agale, B. N. (1993). Phosphate balance studies in irrigated cotton. Journal of the Indian Society of Soil Science, 41, 498–500.
- Mullins, G. L., Schwab, G. J., & Burmester, C. H. (1999). Cotton response to surface applications of potassium fertilizer: A 10-year summary. Journal of Production Agriculture, 12, 434–440.10.2134/jpa1999.0434
- Ochiai, E.-L. (1977). Bioinorganic chemistry (p. 515). Boston, MA: Allyn & Bacon.
- Oosterhuis, D., Hake, K., & Burmester, C. (1991, July ). Leaf feeding insects and mites. Cotton Physiology Today, 2, 1–7.
- Palomo Gil, A., & Chávez González, J. F. (1997). Response of the early cotton cultivar CIAN 95 to nitrogen fertilizer application. ITEA. Producción Vegetal, 93, 126–132.
- Palomo Gil, A., Godoy Avila, S., & Chávez González, J. F. (1999). Reductions in nitrogen fertilizers use with new cotton cultivars: Yield, yield components and fiber quality. Agrociencia, 33, 451–455.
- Perumai, N. K. (1999). Effect of different nitrogen levels on morpho-physiological characters and yield in rainfed cotton. Indian Journal of Plant Physiology, 4, 65–67.
- Pervez, H., Ashraf, M., & Makhdum, M. I. (2004). Influence of potassium rates and sources on seed cotton yield and yield components of some elite cotton cultivars. Journal of Plant Nutrition, 27, 1295–1317.
- Pettigrew, W. T. (1999). Potassium deficiency increases specific leaf weights of leaf glucose levels in field-grown cotton. Agronomy Journal, 91, 962–968.10.2134/agronj1999.916962x
- Pettigrew, W. T., Meredith, W. R., Jr., & Young, L. D. (2005). Potassium fertilization effects on cotton lint yield, yield components, and reniform nematode populations. Agronomy Journal, 97, 1245–1251.10.2134/agronj2004.0321
- Pípolo, A. E., Athayde, M. L. F., Pípolo, V. C., & Parducci, S. (1993). Comparison of different rates of chlorocholine chloride applied to herbaceous cotton. Pesquisa Agropecuária Brasileira, 28, 915–923.
- Ram, P., & Prasad, M., & Pachauri, D. K. (2001). Effect of nitrogen, chlormequat chloride and FYM on growth yield and quality of cotton (Gossypium hirsutum L.). Annals of Agricultural Research, 22, 107–110.
- Rathinavel, K., & Dharmalingam, C. (2000). Paneersel vam S: Effect of micronutrient on the productivity and quality of cotton seed cv. TCB 209 (Gossypium barbadense L.). Madrase. Madras Agricultural Journal, 86, 313–316.
- Reddy, A. R., Reddy, K. R., Padjung, R., & Hodges, H. F. (1996). Nitrogen nutrition and photosynthesis in leaves of Pima cotton. Journal of Plant Nutrition, 19, 755–770.10.1080/01904169609365158
- Rensing, L., & Cornelius, G. (1980). Biological membranes as components of oscillating systems. Biologische Rundschau, 18, 197–209.
- Rinehardt, J. M., Edmisten, K. L., Wells, R., & Faircloth, J. C. (2004). Response of ultra–narrow and conventional spaced cotton to variable nitrogen rates. Journal of Plant Nutrition, 27, 743–755.10.1081/PLN-120030379
- Rodríguez, D., Zubillaga, M. M., Ploschuk, E., Keltjens, W., Goudriaan, J., & Lavado, R. (1998). Leaf area expansion and assimilate prediction in sunflower growing under low phosphorus conditions. Plant Soil, 202, 133–147.10.1023/A:1004348702697
- Russell, E. W. (1973). Soil condition and plant growth (p. 448). London: The English Language Book Society and Longman.
- Sabino, N. P., da Silva, N. M., & Kondo, J. I. (1999). Components of production and fiber quality of cotton as a function of potassium and gypsum (pp. 703–706). Campina Grande: Empresa Brasileira de Pesquisa Agropecuáia, Embrapa Algodão.
- Saleem, M. F., Bilal, M. F., Awais, M., Shahid, M. Q., & Anjum, S. A. (2010). Effect of nitrogen on seed cotton yield and fiber quality of cotton (Gossypium hirsutum L.). The Journal Animal Plant Science, 20, 23–27.
- Saleem, M. F., Shakeel, A., Bilal, M. F., Shahid, M. Q., & Anjum, S. A. (2010). Effect of different phosphorus levels on earliness and yield of cotton cultivars. Soil Environ, 29, 128–135.
- Sangakkara, U. R., Frehner, M., & Nosberger, J. (2000). Effect of soil moisture and potassium fertilizer on shoot water potential, photosynthesis and partitioning of carbon in mungbean and cowpea. Journal of Agronomy and Crop Science, 185, 201–207.10.1046/j.1439-037x.2000.00422.x
- Sarwar Cheema, M., Akhtar, M., & Nasarullah, M. (2009). Effect of foliar application of mepiquat chloride under varying nitrogen levels on seed cotton yield and yield components. Journal of Agricultural Research, 47, 381–388.
- Sasthri, G., Thiagarajan, C. P., Srimathi, P., Malarkodi, K., & Venkatasalam, E. P. (2001). Foliar application of nutrient on the seed yield and quality characters of nonaged and aged seeds of cotton cv. MCU5. Madras Agricultural Journal, 87, 202–206.
- Sawan, Z. M., Basyony, A. E., McCuistion, W. L., & El-Farra, A. A. (1993). Effect of plant population densities and application of growth retardants on cottonseed yield and quality. Journal of the American Oil Chemists’ Society, 70, 313–317.10.1007/BF02545314
- Sawan, Z. M., Fahmy, A. H., & Yousef, S. E. (2009). Direct and residual effects of nitrogen fertilization, foliar application of potassium and plant growth retardant on Egyptian cotton growth, seed yield, seed viability and seedling vigor. Acta Ecologica Sinica, 29, 116–123.10.1016/j.chnaes.2009.05.008
- Sawan, Z. M., Fahmy, A. H., & Yousef, S. E. (2011). Effect of potassium, zinc and phosphorus on seed yield, seed viability and seedling vigor of cotton (Gossypium barbadense L.). Archives of Agronomy and Soil Science, 57, 75–90.10.1080/03650340903222328
- Sawan, Z. M., & Gregg, B. R. (1993). Influence of growth retardants and plant density on cotton yield and fiber properties. Ann Bot (Rome), 51, 33–42.
- Sawan, Z. M., Gregg, B. R., & Yousef, S. E. (1998). Influence of nitrogen fertilisation and foliar-applied plant growth retardants and zinc on cotton seed yield, viability and seedling vigour. Seed Science and Technology, 26, 393–404.
- Sawan, Z. M., Gregg, B. R., & Yousef, S. E. (1999). Effect of phosphorus, chelated zinc and calcium on cotton seed yield, viability and seedling vigour. Seed Science and Technology, 27, 329–337.
- Sawan, Z. M., Hafez, S. A., Basyony, A. E., & Alkassas, A. R. (2007). Nitrogen, potassium and plant growth retardant effects on oil content and quality of cotton seed. Grasas Y Aceites, 58, 243–251.
- Sawan, Z. M., Maddah El-Din, M. S., & Gregg, B. (1989). Effect of nitrogen fertilisation and foliar application of calcium and micro-elements on cotton seed yield, viability and seedling vigor. Seed Science and Technology, 17, 421–431.
- Sawan, Z. M., Mahmoud, M. H., & El-Guibali, A. H. (2006). Response of yield, yield components, and fiber properties of Egyptian cotton (Gossypium barbadense L.) to nitrogen fertilization and foliar-applied potassium and mepiquat chloride. Journal of Cotton. Science, 10, 224–234.
- Sawan, Z. M., & Sakr, R. A. (1990). Response of Egyptian cotton (Gossypium barbadense) yield to 1,1-dimethyl piperidinium chloride (Pix). The Journal of Agricultural Science, 114, 335–338.10.1017/S0021859600072725
- Scott, M. G., & Peterson, R. L. (1979). The root endodermis in Ranunculus acris. I. Structure and ontogeny. Canadian Journal of Botany, 57, 1040–1062.10.1139/b79-129
- Sharma, C. P., Sharma, P. N., Bisht, S. S., & Nautiyal, B. D. (1982). Zinc deficiency induced changes in cabbage. P. 601-606. In A. Scaife (Ed.), Proceeding of the 9th Plant Nutrition Colloquy, Warwick, England (pp. 22–27). Slough: Aug. Commonwealth Agric. Bur. Farnham House.
- Sharma, P. N., Chatterjee, C., Agarwala, S. C., & Sharma, C. P. (1990). Zinc deficiency and pollen fertility in maize (Zea mays). Plant and Soil, 124, 221–225.10.1007/BF00009263
- Shrivastava, U. K., Tomar, S. P. S., & Singh, D. (1993). Effect of N and Zn fertilization on uptake of nutrients and dry matter production. Indian Society Cotton Improvement, 18, 71–74.
- Shui, J.-G., & Meng, S.-F. (1990). Effects of lime application on cotton yield in red soil fields. China Cottons, 1, 26–29.
- Silvertooth, J. C., & Norton, E. R. (1997). Evaluation of a feedback approach to nitrogen and Pix application, Cotton, A College of Agriculture Report (Series P-112, 1998, pp. 469–475). Tucson, AZ: University of Arizona.
- Snedecor, G. W., & Cochran, W. G. (1980). Statistical Methods (7th ed., p. 305). Ames, Iowa, USA: Iowa State University Press.
- Speed, T. R., Krieg, D. R., & Jividen, G. (1996). Relationship between cotton seedling cold tolerance and physical and chemical properties. In Proceedings Beltwide Cotton Conferences, Nashville, TN, USA, January 9–12 (pp. 1170–1171). Memphis, TN: National Cotton Council.
- Stuart, B. L., Isbell, V. R., Wendt, C. W., & Abernathy, J. R. (1984). Modification of cotton water relations and growth with mepiquat chloride. Agronomy Journal, 76, 651–655.10.2134/agronj1984.00021962007600040034x
- Taiz, L., & Zeiger, E. (1991). Plant physiology: Mineral nutrition (pp. 100–119). Redwood City, CA: The Benjamin Cummings.
- Vasudevan, S. N., Virupakshappa, K., Venugopal, N., & Bhaskar, S. (1997). Response of sunflower (Helianthus annuus) to phosphorus, sulphur, micronutrients and humic acid under irrigated conditions on red sandy-loam soil. Indian Journal of Agricultural Science, 67, 110–112.
- Vlasynk, P. A., Zhidkov, V. A., Ivchenkov, V. I., Kibalenko, A. P., Klimovitskaya, Z. M., Okrim, M. F., & Rudakova, E. V. (1978). Trace elements in nutrient metabolism and productivity of plants. Fiziologia Biokimya kul’turnykh Rastenii, 10, 350–359.
- von Heyendorff-Scheel, R. C., Schott, P. E., & Rittig, F. R. (1983). Mepiquat chloride, a plant growth regulator for cotton. Zeitschrift fur Pflanzenkrankheiten und Pflanzenschutz, 90, 585–590.
- Wang, Z.-L., Yin, Y.-P., & Sun, X.-Z. (1995). The effect of DPC (N, N-dimethyl piperidinium chloride) on the 14CO2-assimilation and partitioning of 14C assimilates within the cotton plants interplanted in a wheat stand. Photosynthetica, 31, 197–202.
- Wang, Y.-Q., Yang, W.-H., Zhou, D.-Y., & Xu, H.-X. (2003). Study on the relationship between the nutrients of cottonseed and germination and emergence percentage. Cotton Science, 15, 109–112.
- Welch, R. M. (1995). Micronutrient nutrition of plants. Critical Reviews in Plant Sciences, 14, 49–82.10.1080/07352689509701922
- Wiatrak, P. J., Wright, D. L., Marois, J. J., Koziara, W., & Pudelko, J. A. (2005). Tillage and nitrogen application on cotton following wheat. Agronomy Journal, 97, 288–293.10.2134/agronj2005.0288
- Wiatrak, P. J., Wright, D. L., & Marois, J. J. (2006). Development and yields of cotton under two tillage systems and nitrogen application following white lupine grain crop. Journal Cotton Science, 10, 1–8.
- Wright, S. D., Munk, D., Munier, D., Vargas, R., Weir, B., Roberts, B., & Jimenez, M., Jr. (1995). Effect of aminofol/Boll-Set Plus calcium zinc on California cotton. In Proceedings Beltwide Cotton Conferences, San Antonio, TX, USA, January 4–7 (Vol. 2). Memphis, TN: National Cotton Council.
- Wu, F.-B., Wu, L.-H., & Xu, F.-H. (1998). Chlorophyll meter to predict nitrogen sidedress requirement for short-season cotton (Gossypium hirsutum L.). Field Crops Research, 56, 309–314.
- Zeng, Q.-F. (1996). Experimental study on the efficiency of K fertilizer applied to cotton in areas with cinnamon soil or aquic soil. China Cottons, 23, 12.
- Zhao, D.-L., & Oosterhuis, D. (1999). Physiological, growth and yield responses of cotton to Mepplus and mepiquat chloride. In Proceedings Beltwide Cotton Conferences, Orlando, Florida, USA, 3–7 January (pp. 599–602). Memphis, TN: National Cotton Council.
- Zhao, D.-L., & Oosterhuis, D. M. (2000). Pix plus and mepiquat chloride effects on physiology, growth, and yield of field-grown cotton. Journal of Plant Growth Regulation, 19, 415–422.10.1007/s003440000018