Abstract
Violacein, a purple bacterial pigment, has a variety of biological activities, including cytotoxicity against tumour cells. To investigate the physiological properties of this pigment, we studied its inhibitory effect on protein kinases in vitro. We used phosphorylation experiments to assess the inhibitory effect of violacein on protein kinase activity. Violacein inhibited the classical-type protein kinase C (PKC) and some novel and atypical PKC enzymes. The catalytic subunits of protein kinase A (PKA) and classical-type PKC were strongly inhibited by violacein. Kinetic analysis of PKA inhibition displayed mixed-type inhibition with respect to ATP. Slight inhibition of Ca2+/calmodulin-dependent protein kinase was observed while protein tyrosine kinase (Src) was not inhibited by violacein. The inhibition of PKA and PKCs by violacein provided evidence that violacein binds to the catalytic subunit of enzymes. The protein kinase inhibition revealed here may be important to the mechanism of violacein cytotoxicity.
Public Interest Statement
Violacein is a pigment produced by some terrestrial and marine bacteria. The physiological role of violacein is unknown, but it has been reported to play a role in chemical defence against predators. Furthermore, violacein has strong antitumour activity against various types of cancer cells. In this study, we examined the inhibitory properties of violacein on the activity of protein kinases and showed that violacein could inhibit protein kinase C and protein kinase A. The findings of this study will help characterise the molecular mechanisms underlying violacein’s antitumour activity and may suggest possible medical applications of violacein.
Competing interests
The authors declare no competing interest.
1. Introduction
Violacein, a purple pigment, is a typical secondary metabolite produced by several groups of bacteria (Choi, Yoon, Lee, & Mitchell, Citation2015; Durán & Menck, Citation2001; Durán et al., Citation2012). Violacein has biological properties with possible medical applications, including antibacterial, antiparasitic, antifungal and antitumour activities (Choi et al., Citation2015; Durán et al., Citation2012). Since the discovery of violacein, the physiological significance of this pigment has remained unclear. However, Matz et al. (Citation2004) suggested that violacein is involved in chemical defence in bacteria.
Violacein also exhibits cytotoxicity to various tumour cell lines through the induction of apoptosis (Alshatwi, Subash-Babu, & Antonisamy, Citation2016; Bromberg et al., Citation2010; Ferreira et al., Citation2004; Kodach et al., Citation2006). However, the molecular mechanisms and target molecules involved in this toxicity have not been determined. Therefore, identification of the cellular components directly affected by violacein is essential to understand this pigment’s physiological and pharmacological properties.
Some natural products from bacteria have inhibitory effects on intracellular signalling enzymes, particularly protein kinases and protein phosphatases (Soliev, Hosokawa, & Enomoto, Citation2015; Stevenson et al., Citation2002; Tamaoki & Nakano, Citation1990). Staurosporine from Streptomyces sp. is a strong inhibitor of protein kinases including protein kinase C (PKC) (Tamaoki & Nakano, Citation1990), while scytonemin, a yellow-green pigment isolated from marine cyanobacteria, inhibited polo-like kinase and PKCβ (Stevenson et al., Citation2002). We recently reported that prodigiosin and its congeners isolated from marine bacteria strongly inhibited protein phosphatases, protein phosphatase 2A and protein tyrosine phosphatase 1B (Soliev et al., Citation2015). Violacein has been also reported to cause 30% inhibition of cytoplasmic protein phosphatase activity extracted from human lymphocytes (Bromberg, Justo, Haun, Durán, & Ferreira, Citation2005).
Here, we report the selective inhibitory effect of violacein on PKC and protein kinase A (PKA). This novel property of violacein may be involved in the cytotoxicity of the pigment.
2. Materials and methods
2.1. Chemicals and reagents
Violacein was purified from Pseudoalteromonas sp. strain 520P1 (accession number; NBRC107703), as described previously (Yada et al., Citation2008).
The PKA catalytic subunit from bovine heart, PKC catalytic subunit from rat brain and recombinant isozymes of PKC (α, βI, βII, γ, δ, ε, θ, η and ζ) were purchased from Sigma–Aldrich (St. Louis, MO, USA). Recombinant human Src kinase and Ca2+/calmodulin-dependent protein kinase II (CaM kinase) were purchased from Enzo Life Sciences (Plymouth Meeting, PA, USA). Kemptide, 3-sn-phosphatidyl-l-serine (PS) and 1,2-dioleoyl-sn-glycerol (DAG) were also obtained from Sigma-Aldrich. [Ser159]-PKCε fragment (amino acid (aa) 149–164) peptide, [Ser25]-PKC fragment (aa 19–31) peptide, autocamtide-2 peptide and calmodulin were also obtained from Enzo Life Sciences. The p60c-src substrate peptide was purchased from AnaSpec (Fremont, CA, USA). [γ-32P]ATP (NEG502A) was obtained from Perkin Elmer (Waltham, MA, USA).
2.2. Phosphorylation experiments
The PKA catalytic subunit was assayed according to the method of Toomik and Ek (Citation1997), with minor modifications. The phosphorylation reaction was performed in 100 μl of buffer containing 5 μl of violacein dissolved in dimethyl sulfoxide (DMSO), 25 mM Tris–HCl, pH 7.5, 9.4 mM MgCl2, 100 μM kemptide, 0.1 mg/ml ovalbumin, 10 units/ml PKA catalytic subunit and 190 μM [γ-32P]ATP (specific radioactivity, 1.0 KBq/nmol).
Phosphorylation experiments with PKC enzymes were carried out according to the manufacturer’s instructions, with some modifications. The PKC catalytic subunit was assayed in a final volume of 100 μl of buffer containing 5 μl of violacein dissolved in DMSO, 20 mM Tris–HCl pH 7.5, 10 mM MgCl2, 20 μM [Ser25]-PKC fragment (aa 19–31) peptide, PKC catalytic subunit and 100 μM [γ-32P]ATP (specific radioactivity, 2.8 KBq/nmol). Assays using the recombinant PKC subfamily of enzymes were conducted as described above, with the exception that 48 μM [Ser159]-PKCε fragment (aa 149–164) peptide substrate was used. For classical PKCs, 0.1 mM CaCl2, 5 μg/ml PS and 1 μg/ml DAG were added. For novel and atypical PKC enzymes, 0.1 mM ethylene glycol tetraacetic acid was added instead of 0.1 mM CaCl2. DAG was not included in the assay mixture for atypical PKCs.
Protein tyrosine kinase (Src kinase) and CaM kinase were assayed according to the manufacturer’s instructions, in a final volume of 50 μl of buffer containing 5 μl of violacein dissolved in DMSO, 50 mM 4-(2-hydroxylethyl)-1-piperazineethansulfonic acid (HEPES)-NaOH pH 7.4, 10 mM MgCl2, 1 mM ethylene glycol tetraacetic acid, 200 μM p60c-src substrate for Src kinase or 50 μM autocamtide for CaM kinase enzymes and 200 μM [γ-32P]ATP (specific radioactivity, 4.2 KBq/nmol). For the assay of CaM kinase, 5 mM CaCl2 and 30 μg/ml calmodulin were also added.
Phosphorylation reactions by all kinase types were performed in duplicate or triplicate at 30°C for either 10 or 20 min. The mean enzyme activity values within the 10% margin of error are presented in the figures. The reactions were initiated by the addition of 5 μl [γ-32P]ATP solution and stopped by the addition of 10 μl 800 mM H3PO4 solution. Phosphorylated peptides were recovered on P-81-type phosphocellulose paper, according to the method of Toomik and Ek (Citation1997). Incorporated radioactivity was measured for 10 min by Cerenkov counting in a Beckman LS 6500 scintillation counter (Beckman Instruments, Inc. Fullerton, CA, USA).
2.3. Protein phosphatase assay
The effect of violacein on protein phosphatase 2A activity was assayed as previously described (Soliev et al., Citation2015).
3. Results
Bromberg et al. (Citation2005) have previously shown that violacein at a concentration of 10 μM inhibited the activity of protein phosphatase extracted from human lymphocyte by 30%. We confirmed this inhibition of enzyme activity using recombinant human protein phosphatase 2A. The concentration for 50% inhibition of enzyme activity (IC50) was 15 μM violacein (data not shown) while 2-methyl-butylprodiginine, a prodigiosin family compound, displayed IC50 of 2 μM (Soliev et al., Citation2015). A higher concentration of violacein was necessary for the inhibition of protein phosphatase 2A compared to prodigiosin family compounds. We, therefore, examined the inhibitory effect of violacein on protein kinases.
Figure shows the effects of violacein on the enzymatic activity of PKC subfamily enzymes. PKC enzymes belong to a large family of enzymes consisting of classical (α, βI, βII, γ), novel (δ, ε, θ, η) and atypical (ζ, μ, τ, ι) subfamilies. Therefore, we examined whether violacein has inhibitory activity against these subtypes of enzymes. An assay of recombinant enzyme activity was conducted with the corresponding PKC activators and [Ser159]-PKCε fragment (aa 149–164) peptide substrate. Our results demonstrated that classical PKC enzymes, which require diacylglycerol (DAG) and Ca2+ for activation, were differentially inhibited by violacein (Figure (a)). The assay was also conducted using [Ser25]-PKC fragment (aa 19–31) peptide, another substrate that was used in the PKC catalytic subunit enzyme activity assay in Figure (b). A similar level of inhibition of classical PKC enzymes was observed, though PKCβII was only weakly inhibited (data not shown).
Figure 1. Inhibition of a subfamily of PKC enzymes by violacein. (a) Classical-type recombinant PKCs (α, βI, βII and γ, 7.5 ng of each) were assayed at 30°C for 10 min. Phosphorylations in the absence of violacein were 0.63 (PKCα), 0.34 (PKCβI), 0.76 (PKCβII) and 0.38 (PKCγ) nmol Pi, respectively. (b) Novel and atypical recombinant PKCs (δ, ε, θ, η and ζ, 6.6 ng of each) were assayed at 30°C for 10 min. Phosphorylations in the absence of violacein were 0.023 (PKCδ), 0.13 (PKCε), 0.020 (PKCη), 0.095 (PKCθ) and 0.13 (PKCζ) nmol Pi, respectively.
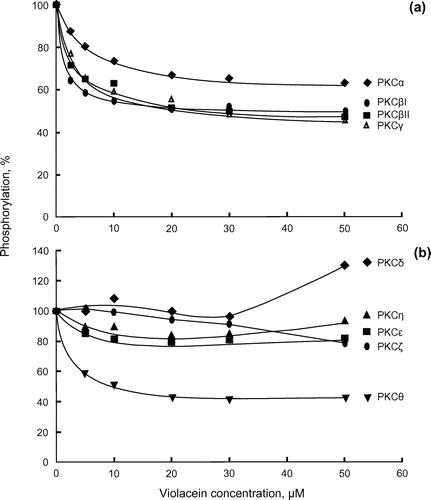
Figure 2. Inhibition of the catalytic subunits of PKA and PKC by violacein. (a) Catalytic subunits of PKA (1 unit) and (b) PKC (2.1 ng) were assayed at 30°C for 10 and 20 min, respectively, in the presence of the indicated concentrations of violacein.
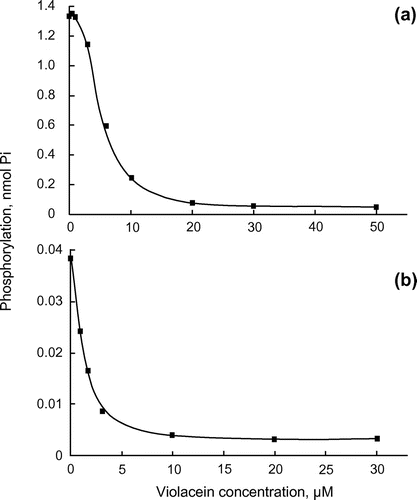
PKCζ, which belongs to the atypical subfamily of PKCs and does not require DAG and Ca2+ for its activation, was only slightly inhibited by violacein (Figure (b)). Notably the PKCθ enzyme, which belongs to the novel subfamily of PKC and requires DAG, but not Ca2+, for its activation, was inhibited by violacein. PKCδ, PKCε and PKCη from the same subfamily showed no or only slight inhibition (Figure (b)).
We further examined the enzyme inhibition by violacein using the catalytic subunits of PKC and PKA to know whether violacein inhibits the enzyme activity by interacting with the catalytic site of the enzyme (Figure ). Phosphorylation of kemptide, a synthetic peptide specific for PKA, was measured with [γ-32P]ATP in an assay with PKA (Figure (a)). Increasing the concentration of violacein from 0.4 to 50 μM caused a gradual decrease in the catalytic activity of PKA, indicating that the inhibition of enzyme activity was dependent on the concentration of violacein. The IC50 value was approximately 6 μM. To examine whether violacein inhibits enzyme activity by competing with ATP, the km values of PKA for ATP were compared in the presence and absence of violacein using an Eadie–Hofstee plot (Figure ). Violacein showed a mixed-type inhibition with respect to ATP. In mixed-type inhibition, an inhibitor binds to the enzyme regardless of whether it has already bound the substrate. When the inhibitor has a higher affinity for the enzyme than for the enzyme–substrate complex, the maximum velocity and the apparent affinity for the substrate decrease. In the presence of violacein, the km value increased from 24 to 42 μM, and the maximum velocity of the reaction decreased by approximately 50%. These results indicated that the inhibition of protein kinase activity by violacein is not simply based on its competition with ATP.
Figure 3. Enzyme kinetics of the PKA catalytic subunit. Eadie–Hofstee plot of kinetic experiments with PKA. PKA was assayed without violacein (closed square) and in the presence of 6-μM violacein (closed circle).
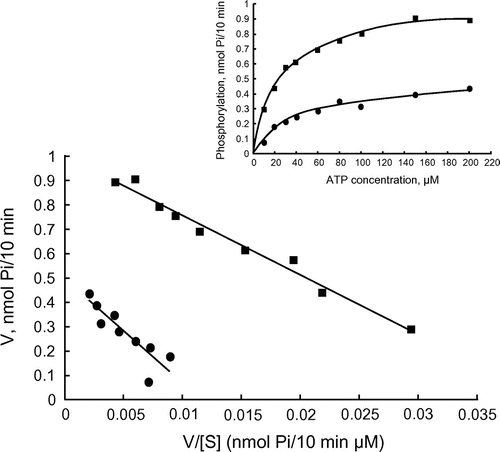
Figure (b) shows the inhibitory effects of violacein on the catalytic subunit activity of PKC from rat brain. The catalytic subunit is a fragment of PKC, from which the regulatory region has been removed by partial proteolysis. This subunit does not, therefore, require the PKC activators, DAG, Ca2+ and phosphatidylserine (PS), for its activation. Phosphorylation of [Ser25]-PKC fragment (aa 19–31), a modified PKCα pseudosubstrate peptide, was assayed with [γ-32P]ATP, using a similar method to that used for PKA. Violacein clearly inhibited the enzymatic activity of the catalytic subunit with an IC50 value of 2 μM.
Figure shows the effects of violacein on Src kinase and CaM kinase, respectively. CaM kinase was slightly inhibited by violacein while inhibition of Src kinase was barely perceptible. The extent of violacein-mediated inhibition was dependent on the type of protein kinase analysed.
Figure 4. Effects of violacein on Src kinase and CaM kinase. Src kinase (20 ng) and CaM kinase (15 ng) were assayed at 30°C for 20 min. Phosphorylations in the absence of violacein were 0.047 (Src kinase) and 0.54 (CaM kinase) nmol Pi, respectively.
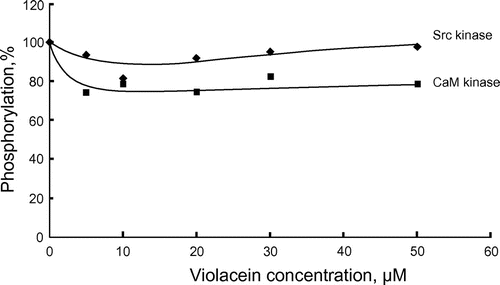
These results clearly showed that violacein has a selective inhibitory effect on protein kinases A and C, which may cause metabolic interruption in eukaryotic cells.
4. Discussion
Violacein has a range of physiological properties, such as antibacterial, antifungal, anti-trypanosome and antimalarial activities (Choi et al., Citation2015; Durán & Menck, Citation2001; Durán et al., Citation2012). Violacein has also been reported to show toxicity against a number of different types of tumour cell lines (Alshatwi et al., Citation2016; Bromberg et al., Citation2010; Ferreira et al., Citation2004; Kodach et al., Citation2006). A recent study suggested that the toxicity of violacein is essential for chemical defence against eukaryotes that predate violacein-producing bacteria (Matz et al., Citation2004). It is likely that the toxicity of violacein is related to its ability to induce apoptosis in eukaryotic cells. Although there are several reports relating to the toxic effects of violacein (Choi et al., Citation2015; Durán et al., Citation2012), the target molecules on which violacein acts have yet to be determined.
In our study, violacein inhibited the catalytic subunits of PKA and PKC with IC50 values of 6 and 2 μM, respectively. Kinetic analysis of the PKA catalytic subunit showed mixed inhibition with respect to ATP. Well-known non-selective inhibitor of protein kinases, staurosporine, has been shown to inhibit protein kinases by a mixed-type inhibition mechanism, while its analogue K252a inhibits protein kinases in a manner that is competitive with ATP (Kase et al., Citation1987; Ward & O’Brian, Citation1992). In this respect, the mode of protein kinase inhibition by violacein was similar to that of staurosporine. The catalytic subunit of PKC from rat brain, in which classical-type PKCs are expressed (Mellor & Parker, Citation1998), was the most effectively inhibited. This result was consistent with the observation that violacein inhibited all of the classical-type PKCs examined. Enzyme inhibition by violacein varied between different protein kinases, with inhibition observed for PKA, and most PKC enzymes tested. PKCζ, PKCε and PKCη were only slightly inhibited, and PKCδ was not inhibited by violacein (Figure (b)). It remains unclear why violacein does not affect all enzymes in the same subfamily, but this observation suggests that the violacein binding sites of these enzymes may be different. A similar differential inhibition has been reported for UCN-01, a staurosporine analogue, which more potently inhibited classical PKC enzymes, than novel and atypical PKC enzymes (Mizuno et al., Citation1995; Seynaeve, Kazaniets, Blumberg, Sausville, & Worland, Citation1994).
Furthermore, when the protein kinases were activated by DAG and PS at relatively high concentrations (20 and 100 μg/ml, respectively), the inhibitory activity of violacein decreased. We believe that this was caused by the hydrophobic interaction of violacein with these lipid molecules. The inhibition of PKA and PKC by violacein provided evidence that violacein binds to the catalytic subunit of enzymes. The recombinant PKC enzymes consisting of both regulatory and catalytic domains require DAG and PS for activation. However, it seems that excess DAG and PS not only bind to the enzyme but also interact with violacein. This seems consistent with the experimental data, which indicated that the PKC catalytic subunit was inhibited to a greater extent than the recombinant isozyme itself. It should be also noted that the potency of violacein-dependent inhibition is not determined only by the interaction of violacein with DAG and PS. PKCθ, a member of a novel PKC subfamily that requires DAG for its activity, was inhibited by violacein (Figure (b)). However, other members (PKCδ, PKCε and PKCη) belonging to the same subfamily and PKCζ, an atypical PKC that does not need DAG, were at most only slightly inhibited by violacein. These results suggest that the violacein binding sites of these enzymes have different affinity for violacein and, therefore, different potencies of violacein-dependent inhibition.
In this study, we also examined the inhibitory effect of violacein on other families of protein kinases, namely: Src kinase, a non-receptor protein tyrosine kinase, and CaM kinase, a kinase activated by Ca2+ and calmodulin. These kinases were only slightly inhibited by violacein and are therefore unlikely to be major targets of the pigment. Therefore, it is likely that violacein inhibits protein kinases belonging to AGC group of protein kinases which includes PKC and PKA. The AGC group contains Akt (also known as PKB) and PDK1, which play a pivotal role in intracellular signalling. However, it has yet to be determined whether these enzymes could be targets for violacein.
An operon consisting of five synthetic enzyme genes, vioA–vioE, is involved in the biosynthesis of violacein (Balibar & Walsh, Citation2006; Hoshino, Citation2011; Sánchez, Braña, Méndez, & Salas, Citation2006). The first enzyme, encoded by the vio gene operon, VioA, converts l-tryptophan to indole-3-pyruvic acid imine (IPA imine). The second enzyme, VioB, catalyses the conversion of the IPA imine into an unidentified compound X, which may be an IPA imine dimer. Compound X then undergoes successive reactions catalysed by the enzymes VioE, VioD and VioC to produce violacein. The pathway catalysed by VioA and VioB is common to the biosynthesis of other antibiotics, such as rebeccamycin and staurosporine (Sánchez et al., Citation2006), indicating that these different biosynthetic pathways may have evolved from the same origin. It is, therefore, interesting to note that both staurosporine and violacein are able to inhibit protein kinases.
In this study, we have shown the inhibitory effect of violacein on PKA and PKC and have provided insight into the possible molecular interactions involved in its toxicity to eukaryotic cells.
5. Conclusions
The present study demonstrated that violacein inhibited the enzymatic activities of PKA and PKC. This inhibition was shown to be caused by the interaction of violacein with the catalytic subunits of PKA and PKC. Kinetic analysis of PKA inhibition revealed mixed-type inhibition with respect to ATP. Violacein exhibited an inhibitory effect on all of the classical PKC enzymes, but its inhibition potency differed depending on the types of novel and atypical PKC enzymes. Violacein only slightly inhibited CaM kinase and Src kinase. This indicates that violacein selectively inhibited the protein kinases that belong to the AGC group of protein kinases.
Additional information
Funding
Notes on contributors
Keiichi Enomoto
Kakushi Hosokawa, Azamjon B. Soliev and Aki Kajihara joined this research project as graduate students at Kochi University of Technology under the supervision of Keiichi Enomoto. Enomoto’s group has focused its attention on marine bacteria that produce bacterial pigments including violacein and prodigiosin. Prior to this study, the authors had isolated pigment-producing marine bacteria, purified and identified bacterial pigments and isolated the gene cluster for violacein synthesis. Further research is being conducted to clarify the regulatory mechanism of violacein biosynthesis in marine bacteria and the molecular mechanism underlying violacein’s antitumour activity. This study was conducted as a research project related to the molecular mechanism of violacein’s physiological properties.
References
- Alshatwi, A. A., Subash-Babu, P., & Antonisamy, P. (2016). Violacein induces apoptosis in human breast cancer cells through up regulation of BAX, p53 and down regulation of MDM2. Experimental and Toxicologic Pathology, 68, 89–97.10.1016/j.etp.2015.10.002
- Balibar, C. J., & Walsh, C. T. (2006). In vitro biosynthesis of violacein from l -tryptophan by the enzymes VioA−E from Chromobacterium violaceum. Biochemistry, 45, 15444–15457.10.1021/bi061998z
- Bromberg, N., Dreyfuss, J. L., Regatieri, C. V., Palladino, M. V., Durán, N., Nader, H. B., … Justo, G. Z. (2010). Growth inhibition and pro-apoptotic activity of violacein in Ehrlich ascites tumor. Chemico-Biological Interactions, 186, 43–52.10.1016/j.cbi.2010.04.016
- Bromberg, N., Justo, G. Z., Haun, M., Durán, N., & Ferreira, C. V. (2005). Violacein cytotoxicity on human blood lymphocytes and effect on phosphatases. Journal of Enzyme Inhibition and Medicinal Chemistry, 20, 449–454.10.1080/14756360500273052
- Choi, S. Y., Yoon, K., Lee, J. I., & Mitchell, R. J. (2015). Violacein: properties and production of a versatile bacterial pigment. BioMed Research International, 2015, 465056. doi:10.1155/2015/465056
- Durán, N., & Menck, C. F. M. (2001). Chromobacterium violaceum: A review of pharmacological and industrial perspectives. Critical Reviews in Microbiology, 27, 201–222.10.1080/20014091096747
- Durán, M., Ponezi, A. N., Faljoni-Alario, A., Teixeira, M. F. S., Justo, G. Z., & Durán, N. (2012). Potential applications of violacein: a microbial pigment. Medicinal Chemistry Research, 21, 1524–1532.10.1007/s00044-011-9654-9
- Ferreira, C. V., Bos, C. L., Versteeg, H. H., Justo, G. Z., Durán, N., & Peppelenbosch, M. P. (2004). Molecular mechanism of violacein-mediated human leukemia cell death. Blood, 104, 1459–1464.10.1182/blood-2004-02-0594
- Hoshino, T. (2011). Violacein and related tryptophan metabolites produced by Chromobacterium violaceum: biosynthetic mechanism and pathway for construction of violacein core. Applied Microbiology and Biotechnology, 91, 1463–1475.10.1007/s00253-011-3468-z
- Kase, H., Iwahashi, K., Nakanishi, S., Matsuda, Y., Yamada, K., Takahashi, M., Murakata, C., … Kaneko, M. (1987). K-252 compounds, novel and potent inhibitors of protein kinase C and cyclic nucleotide-dependent protein kinases. Biochemical and Biophysical Research Communications, 142, 436–440.10.1016/0006-291X(87)90293-2
- Kodach, L. L., Bos, C. L., Durán, N., Peppelenbosch, M. P., Ferreira, C. V., & Hardwick, J. C. H. (2006). Violacein synergistically increases 5-fluorouracil cytotoxicity, induces apoptosis and inhibits Akt-mediated signal transduction in human colorectal cancer cells. Carcinogenesis, 27, 508–516.10.1093/carcin/bgi307
- Matz, C., Deines, P., Boenigk, J., Arndt, H., Eberl, L., Kjelleberg, S., & Jürgens, K. (2004). Impact of violacein-producing bacteria on survival and feeding of bacterivorous nanoflagellates. Applied and Environmental Microbiology, 70, 1593–1599.10.1128/AEM.70.3.1593-1599.2004
- Mellor, H., & Parker, P. J. (1998). The extended protein kinase C superfamily. Biochemical Journal, 332, 281–292.10.1042/bj3320281
- Mizuno, K., Noda, K., Ueda, Y., Hanaki, H., Saido, T. C., Ikuta, T., … Ohno, S. (1995). UCN-01, an anti-tumor drug, is a selective inhibitor of the conventional PKC subfamily. FEBS Letters, 359, 259–261.10.1016/0014-5793(95)00042-8
- Sánchez, C., Braña, A. F., Méndez, C., & Salas, J. A. (2006). Reevaluation of the violacein biosynthetic pathway and its relationship to indolocarbazole biosynthesis. ChemBioChem, 7, 1231–1240.10.1002/cbic.v7:8
- Seynaeve, C. M., Kazaniets, M. G., Blumberg, P. M., Sausville, E. A., & Worland, P. J. (1994). Differential inhibition of protein kinase C isozymes by UCN-01, a staurosporine analogue. Molecular pharmacology, 45, 1207–1214.
- Soliev, A. B., Hosokawa, K., & Enomoto, K. (2015). Effects of prodigiosin family compounds from Pseudoalteromonas sp. 1020R on the activities of protein phosphatases and protein kinases. Journal of Enzyme Inhibition and Medicinal Chemistry, 30, 533–538.10.3109/14756366.2014.951347
- Stevenson, C. S., Capper, E. A., Roshak, A. K., Marquez, B., Grace, K., Gerwick, W. H., … Marshall, L. A. (2002). Scytonemin—A marine natural product inhibitor of kinases key in hyperproliferative inflammatory diseases. Inflammation Research, 51, 112–114.10.1007/BF02684014
- Tamaoki, T., & Nakano, H. (1990). Potent and specific inhibitors of protein Kinase C of microbial origin. Nature Biotechnology, 8, 732–735.10.1038/nbt0890-732
- Toomik, R., & Ek, P. (1997). A potent and highly selective peptide substrate for protein kinase C assay. Biochemical Journal, 322, 455–460.10.1042/bj3220455
- Ward, N. E., & O’Brian, C. A. (1992). Kinetic analysis of protein kinase C inhibition by staurosporine: Evidence that inhibition entails inhibitor binding at a conserved region of the catalytic domain but not competition with substrates. Molecular pharmacology, 41, 387–392.
- Yada, S., Wang, Y., Zou, Y., Nagasaki, K., Hosokawa, K., Osaka, I., … Enomoto, K. (2008). Isolation and characterization of two groups of novel marine bacteria producing violacein. Marine Biotechnology, 10, 128–132.10.1007/s10126-007-9046-9