Abstract
We investigated sleep quality and visual symptoms in 30 adults who spent two hours before bedtime using a tablet device with and without the advanced technology of large-scale integration for blue-light reduction and color management. Dry eye- and eye fatigue-related symptom scores were significantly better with than without blue-light reduction. Sleepiness and saliva melatonin during the task were greater with blue-light reduction, however, overnight melatonin secretion and sleep quality parameters were similar in both conditions. In conclusion, tablet devices using large-scale integration for blue-light reduction increased sleepiness and reduced eye fatigue and dryness during tasks before bedtime.
Keywords:
Public Interest Statement
This scientific paper describes large-scale integration (LSI) built in tablet PC for blue-light reduction maintained natural sleepiness and reduced eye fatigue and dryness at night. In modern society, many people use portable devices at night and even in bed before sleep. Ordinary tablet emits much blue-light component and may induce sleep disorder and eye symptoms by disturbing biological clock and optical property of the eye. The authors examined 30 healthy adults viewing tablet PC during tasks before bedtime. We found new technology enabled the subjects to do tasks with minimum disadvantages for sleep, dry eye symptoms, and eye fatigue. We believe LSI should be a promising solution for busy mobile user to maintain systemic and eye health.
Competing Interests
The authors declare no competing interest.
1. Introduction
There is growing evidence that exposure to light emitted from a portable device before bedtime disrupts sleep and daytime function (Chang, Aeschbach, Duffy, & Czeisler, Citation2015; Higuchi, Motohashi, Liu, & Maeda, Citation2005). In this respect the more recently available portable devices have become a major problem, unlike the conventional displays of desktop computers and ambient lighting, since they are manipulated at a very short distance and even very young users are using them late at night for several hours (Bartel, Gradisar, & Williamson, Citation2015; Figueiro & Overington, Citation2015; Gradisar et al., Citation2013). Eyewear, software, and films have been introduced to filter and control the blue light emitted from displays (Ayaki et al., Citation2015; Burkhart & Phelps, Citation2009; Heath et al., Citation2014; Ide, Toda, Miki, & Tsubota, Citation2015; van der Lely et al., Citation2015); however, these methods have some disadvantages including decreased visual quality with darkness and yellowness that may be potentially linked to eye fatigue, decreased performance and concentration, and increased errors. The recently developed large-scale integration (LSI) IROMI® engine (Dai Nippon Printing Co. Ltd., Tokyo, Japan), designed to provide acceptable image quality even under reduced blue-light conditions, enables the user to choose a blue-light filter and color management to optimize visual quality.
In addition to the well-known sleep and circadian rhythm disorders caused by the use of portable displays at night, there is also a risk of ophthalmological problems. Eye fatigue and dryness are the most common ocular symptoms in patients visiting ophthalmology clinics due to work-related issues or the habitual use of portable devices late at night. Difficulty focusing and reduced blinking frequency are closely associated with eye fatigue during computer display tasks and the symptoms of patients with dry eye disease (DED) worsen in the evening (Tsubota & Nakamori, Citation1993; Uchino et al., Citation2013; Walker, Lane, Ousler, & Abelson, Citation2010). These symptoms may not be detected in adolescents since difficulty focusing and DED are not common in young people.
To date, there has been no ophthalmic assessment of adults using blue-light control technology at night. Therefore, in the present study, we focused on whether innovative display technology could reduce eye fatigue and DED-related symptoms. We used two ocular examinations that can be performed by untrained participants at night in their bedroom. The first test, of functional visual acuity (FVA), reflects an individual’s visual performance and detects impairment of visual function in various eye diseases including DED, with normal visual acuity measured by standard examination (Kaido, Dogru, Ishida, & Tsubota, Citation2007; Kaido et al., Citation2015). FVA may decrease with disruption of the stable tear-film layer over the surface of the cornea. The second test, maximum blinking interval (MBI), is useful for detecting and quantifying DED because differences in the mean MBI have been reported between normal subjects (8.9 ± 4.0 s) and patients with DED (4.2 ± 2.4 s) (Tsubota et al., Citation1996).
Here, we describe a sleep and ophthalmological study conducted in adults to explore whether blue-light control using LSI can deliver acceptable visual performance and sleep quality against the effects of blue-light pollution from self-luminous portable devices used at night.
2. Methods
2.1. Participants and ethics approval
Participants were recruited via an advertisement in two research centers. The selection criteria were habitual use of a portable tablet device at night, normal vision, and good general health according to a workplace health check by attending physicians. Normal vision was confirmed by a board-certified ophthalmologist for all participants before they completed the study. Exclusion criteria were a history of shift work, systemic illness, use of medication, and any history of psychiatric illness. Participants maintained their usual weekday lifestyle before the study period and were asked to refrain from drinking alcohol, smoking, and consuming caffeine during the study period. The study was conducted during June (20 subjects), October (3 subjects), and November (7 subjects) 2015 in Tokyo, Japan, where the latitude is 35.68° N and day length varies by 4–6 h over the year, according to the 1981–2010 averages reported by the Japan Meteorological Agency.
The study was approved by the institutional review board of Shinseikai Toyama Hospital and was carried out in accordance with the approved guidelines. All participants provided written informed consent.
2.2. Study design and use of self-luminous portable devices
Participants were asked to stay in a dark room from 22:00 to 24:00 doing tasks and reading Japanese literature of their choice on a portable self-luminous device either with or without LSI for blue-light management. They were asked to go to bed at about midnight and sleep for seven hours in the same dark room. Participants were required to send e-mail alerts and submit three reports during the study to confirm awakeness. A cross-over study design was used, so the use of LSI for blue-light management was changed in the second phase (after two weeks). Participants and investigators were masked either with or without LSI for blue-light management.
The self-luminous devices used were 10.1 inch IROMI® Tablet devices (DNP, Co., Ltd., Tokyo, Japan) emitting approximately 410 cd/m2 of visible light; they were held less than 25 cm from the participants’ eyes and participants were instructed to set or hold it within their elbow length. The tasks performed using these devices were reading literature chosen by the participant and/or writing about health and habits. Participants were monitored by actigraphy and email alerts to ensure that they stayed awake during the 2-h task period.
2.3. Specification of LSI
Blue-light reduction and color control LSI (IROMI®, Dai Nippon Printing Co. Ltd., Tokyo, Japan) are an image-processing LSI that has functions of color management, contrast enhancement, and blue-light control. It is built into the IROMI® Tablet device and study participants were masked for its function in the present study. The color filter controls the amount of the three primary colors of light, red (R), green (G), and blue (B), in every pixel from the transmitted image to manage the color display. The color resolution is determined by the data width. For example, in the case of each 8-bit RGB (256 × 256 × 256), it is possible to display 16.77 million colors and in the case of each 6-bit RGB (64 × 64 × 64), it is possible to display 262,000 colors. To reduce blue light, the B component can be reduced, but when only B is reduced the color balance collapses and the color turns yellowish. Because the IROMI® engine can adjust RGB individually, IROMI® Tablet devices adjust R and G to display the correct color. The blue-light reduction rate was reduced by 20.1% at a peak wavelength of 434 nm in the present study and reduced by 14.2% in total irradiance (Figure ). As such, under the blue-light reduction mode, IROMI® LSI controlled the emitted blue light by optimizing color and contrast to acceptable levels (Figure ).
Figure 1. The spectral component of white light emitted from the display of the IROMI® Tablet device with (dotted line) and without large-scale integration for blue-light control (solid line). Blue-light reduction rate is 20.1% (@ 434 nm).
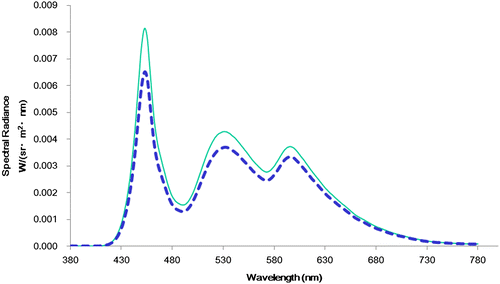
Figure 2. Screen images from the tablet device with and without large-scale integration (LSI) for blue-light control. (a) Original screen image without LSI for blue-light control; (b) Screen image with LSI for blue-light reduction only; (c) Screen image with LSI for blue-light reduction and color control. Note the display is yellowish with blue-light reduction only whereas the image is acceptable with color control by LSI.
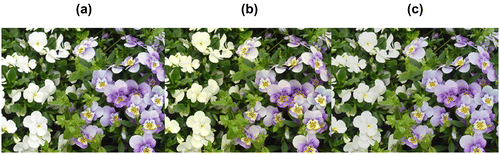
2.4. Ophthalmological examinations
FVA, MBI, and questionnaires regarding visual quality and symptoms related to dry eye were evaluated. The FVA test consisted of a 30–60 s continuous measurement of visual acuity under the best corrected condition, measured at 24:00 with a vision chart on the display downloaded from a free software website to measure FVA (https://itunes.apple.com/jp/app/dryeyekt/id781168068?mt=8). The result was expressed as the mean visual acuity of several measurements during the 30–60 s examination. MBI was expressed as the number of seconds the eyes were kept open, and was measured once at 24:00. Visual quality (clarity, sharpness, eye fatigue, and hue), with or without LSI for blue-light reduction, was measured using a visual analogue scale ranging from 5 (best) to 1 (worst) compared with their own portable device. Dry eye-related symptoms (dryness, irritation, fatigue, blurring) were evaluated as the sum of the symptoms present at 24:00. These symptoms are the most common ocular symptoms included in validated questionnaires (Gulati et al., Citation2006; Sakane et al., Citation2013).
2.5. Actigraphy and questionnaires for measurement of sleepiness and sleep quality
Participants completed a validated questionnaire (Karolinska Sleepiness Scale [KSS]) before and after the task (22:00 and 24:00, respectively). Each participant’s sleep/wake cycle was monitored by a micro-motion logger (AMI, New York, NY, USA) during the study period. Micro-motion data were analyzed using the Cole–Kripke algorithm (Cole, Kripke, Gruen, Mullaney, & Gillin, Citation1992).
2.6. Urine melatonin
First morning-void urine samples were collected from all participants at about 7:00 on the day following each intervention. The samples were frozen immediately and stored at −20°C until testing was undertaken. Urinary 6-sulfatoxymelatonin levels were measured by enzyme-linked immunosorbent assay (ELISA) using a Melatonin-Sulfate Urine ELISA kit (IBL International GmbH, Hamburg, Germany). The sensitivity of this assay was 0.41 ng/mL, and the intra-assay and inter-assay coefficients of variation were 1.2 and 5.7%, respectively. To adjust for variation in the dilution of urine, 6-sulfatoxymelatonin concentration was expressed as urine 6-sulfatoxymelatonin/urine creatinine using LabAssay Creatinine (Jaffe method) (Wako Pure Chemical Industries, Ltd, Tokyo, Japan) (Oba, Nakamura, Sahashi, Hattori, & Nagata, Citation2008).
2.7. Statistical analyses
Data of each phase of the study were analyzed and, where appropriate, given as mean ± standard deviation. Sleep indices, ocular parameters, and melatonin levels obtained during the use of tablet devices with LSI for blue-light reduction (Figure (c)) and without LSI for blue-light reduction (Figure (a)) were compared. All analyses were performed using StatFlex (Atech, Osaka, Japan) and SPSS version 21 (SPSS Inc, Chicago, IL). A p value less than 0.05 was considered significant.
3. Results
Thirty healthy Japanese adults (age range, 24–58 years; mean age, 35.4 ± 8.9 years; 3 women) participated in the study.
FVA tended to be better with (0.02 ± 0.22, LogMAR scale) than without LSI for blue-light reduction (0.13 ± 0.21, LogMAR scale; p = 0.08, paired t test; Figure ). MBI was significantly greater with (22.2 ± 23.0 s) than without LSI for blue-light reduction (18.1 ± 18.5 s; p = 0.03). The dry eye symptom score was improved with the use of LSI for blue-light reduction (0.46 ± 0.80), compared to without it (0.79 ± 0.89; p = 0.01). Visual quality, in terms of eye fatigue, was also significantly better when LSI for blue-light reduction was used, rather than not used (p = 0.04; Table ).
Figure 3. The ocular parameters of functional visual acuity (left panel), maximum blinking interval (center panel), and number of dry eye symptoms (right panel) are shown for participants while they performed tasks for two hours, from 22:00 to 24:00, on self-luminous devices with large-scale integration (LSI) for blue-light reduction and without LSI (control).
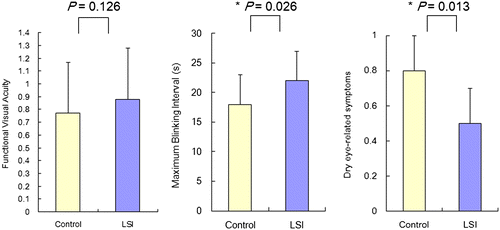
Table 1. Visual qualityTable Footnotea with and without large-scale integration for blue-light reduction
The increase in sleepiness from 22:00 to 24:00, measured with the KSS corrected by normalizing to that at the start of the study, was greater with LSI for blue-light reduction (2.26 ± 2.18, mean KSS from 4.2 to 6.5) than without LSI for blue-light reduction (1.52 ± 2.40, mean KSS from 4.8 to 6.3; p = 0.11) (Figure ). Urine melatonin and sleep parameters measured by actigraphy were not different between conditions. Specifically, for control and LSI, respectively, the mean sleep efficacy was 94.3 ± 6.1% and 93.7 ± 7.6%, sleep latency was 5.18 ± 1.54 min and 5.64 ± 1.11 min, and wake-up time after sleep onset was 26.8 ± 12.0 min and 24.7 ± 9.5 min (all p > 0.05).
4. Discussion
Our present results demonstrate that incorporating LSI for blue-light control in the newly developed tablet devices preserves acceptable image quality and provides better visual function and ocular comfort. In addition, the LSI addition does not appear to alter sleep quality compared with the same tablet device without LSI for blue-light reduction. However, all the participants in our study were adults and many confounding factors might interact with the results, including light transmission into the eye (Cuthbertson, Peirson, Wulff, Foster, & Downes, Citation2009; Turner & Mainster, Citation2008) and light sensitivity (Higuchi, Nagafuchi, Lee, & Harada, Citation2014). Sleepiness was only measured during the time spent watching the screen at night, whereas daytime activity and many other factors can affect melatonin secretion. Thus, the apparent inconsistency between sleepiness and overnight urine melatonin does not exclude the possible advantages of blue-light control LSI for sleep quality, although we did not analyze saliva melatonin during the task since preliminary results showed wide variations among participants not fit for statistical analysis. The discrepancy between melatonin and sleep might therefore be due to daytime activity, sunlight exposure, chronotype, and individual difference.
One investigation reported that 60-min exposures to a tablet screen before bedtime did not affect sleep even in adolescences (Heath et al., Citation2014). The blue-light reduction rate in this study was 20% and thus might not be enough to enhance melatonin secretion. Interestingly, the present results were different from our previous results investigating the effect of blue-light filter eyewear on melatonin secretion and sharpness of image with two hours of tablet device use before bedtime (Ayaki et al., Citation2015). Taken together, eyewear and LSI for blue-light management have unique functions and abilities in reducing the blue-light effect at night in terms of melatonin secretion, sleepiness, vision, and dry eye-related symptoms. LSI for blue-light reduction has no side effects and is not complicated to use. Although further investigations are warranted, this new technology allows users to easily manage blue-light exposure according to their purpose and preference.
Several studies have revealed health and ocular problems caused by the evening use of tablet devices and smartphones. Decreased liquid tear secretion (Srinivasan, Chan, & Jones, Citation2007) and decreased blinking (Tsubota & Nakamori, Citation1993) can exacerbate dry eye symptoms in the evening. Blue light emitted from displays can also induce eye fatigue by light scattering (Kaido et al., Citation2016). Intrinsically photosensitive retinal ganglion cells are implicated in ocular pain when exposed to blue light (Matynia et al., Citation2015) and younger subjects are more sensitive to light in terms of melatonin suppression and headache (Harle & Evans, Citation2004; Higuchi et al., Citation2014; Wilkins, Patel, Adjamian, & Evans, Citation2002). Tinted eyewear has been proven effective for blue-light control during day and night (Ide et al., Citation2015; Kaido et al., Citation2016), and sleep studies have revealed a distinct effect of blue-light filter eyewear in maintaining healthy sleep (Ayaki et al., Citation2016; van der Lely et al., Citation2015).
Blue-light reduction LSI presented better images and less scattering on the display, and these effects induced an increased blinking interval that could be likened to better FVA and less dry eye-related symptoms. MBI, FVA, and dry eye-related questionnaires are clinically proven to be useful for evaluating ocular condition (Gulati et al., Citation2006; Kaido et al., Citation2015; Tsubota et al., Citation1996) and our results favored LSI for maintaining ocular health. Taken together, we speculate that the new LSI could contribute to ocular health with a reduction in the blue light and alteration to the spectral composition.
This study has several limitations. The sample size was small, and the sleep quality data we collected should be extended to a larger number of participants to cover variations in age, level of light sensitivity, and genetic background. Sleep should be evaluated by polysomnography and sleepiness should be measured by saliva melatonin in an environment that is strictly controlled for light level. Also, the study period was short, so longer observation periods should be used in future research to determine the effect of blue-light control LSI on circadian rhythms. A control night during which participants slept the entire night would have been useful because most of the measured values were so variable that the statistical analysis provided few significant findings. Further investigation should be performed with more strict protocols including a control night and experimentally prepared LSI since the LSI used in the present study was a commercial product.
In conclusion, LSI for blue-light reduction was effective for vision and ocular health during a task before bedtime. A longer MBI, reduced dry eye-related symptoms, and the tendency for a better FVA all support the substantial advantages of LSI technology for users. Additionally, hue, sharpness, and clarity of the display using LSI for blue-light reduction were comparable to no blue-light reduction, while eye fatigue was relieved with LSI for blue-light control.
Funding
This work was supported by the Dai Nippon Printing Co., Ltd.
Author contributions
Masahiko Ayaki, Atsuhiko Hattori, and Yusuke Maruyama collected and analyzed the data. Masahiko Ayaki wrote the manuscript. Masahiko Ayaki, Atsuhiko Hattori, Kazuno Negishi, and Kazuo Tsubota designed the study. Masahiko Ayaki, Atsuhiko Hattori, Yusuke Maruyama, Kazuno Negishi, and Kazuo Tsubota reviewed and approved the final version of the manuscript.
Ethical approval
The research project was approved by the Ethics Committee of the institution within which the work was undertaken, and conforms with the provisions of the 1995 Declaration of Helsinki (as revised in Edinburgh, 2000).
Acknowledgments
We thank Mr Michitaka Yoshimura for his valuable suggestions and Dr Takayuki Abe for help with statistical analyses. We acknowledge Inter-Biotech (http://www.inter-biotech.com) for assistance with the English language editing of this manuscript.
Additional information
Notes on contributors
Masahiko Ayaki
Our research team has three subgroups; refraction, cataract, and sleep research. Currently ongoing major projects include progression of myopia and presbyopia, happiness and eye diseases, and driving performance and visual function. Please visit our HP for details: http://ophthal.med.keio.ac.jp/research/index.html
References
- Ayaki, M., Hattori, A., Maruyama, Y., Nakano, M., Yoshimura, M., Kitazawa, M., ... Tsubota, K. (2015). Protective effect of blue-light shield eyewear for adults against light pollution from self-luminous devices used at night. Chronobiology International, 33, 134–139.
- Ayaki, M., Kawashima, M., Negishi, K., Kishimoto, T., Mimura, M., & Tsubota, K. (2016). Sleep and mood disorders in dry eye disease and allied irritating ocular diseases. Scientific Reports, 6, 22480.10.1038/srep22480
- Bartel, K. A., Gradisar, M., & Williamson, P. (2015). Protective and risk factors for adolescent sleep: A meta-analytic review. Sleep Medicine Reviews, 21, 72–85.10.1016/j.smrv.2014.08.002
- Burkhart, K., & Phelps, J. R. (2009). Amber lenses to block blue light and improve sleep: A randomized trial. Chronobiology International, 26, 1602–1612.
- Chang, A. M., Aeschbach, D., Duffy, J. F., & Czeisler, C. A. (2015). Evening use of light-emitting eReaders negatively affects sleep, circadian timing, and next-morning alertness. Proceedings of the National Academy of Sciences, 112, 1232–1237.10.1073/pnas.1418490112
- Cole, R. J., Kripke, D. F., Gruen, W., Mullaney, D. J., & Gillin, J. C. (1992). Automatic sleep/wake identification from wrist activity. Sleep, 15, 461–469.
- Cuthbertson, F. M., Peirson, S. N., Wulff, K., Foster, R. G., & Downes, S. M. (2009). Blue light–filtering intraocular lenses: Review of potential benefits and side effects. Journal of Cataract & Refractive Surgery, 35, 1281–1297.10.1016/j.jcrs.2009.04.017
- Figueiro M., & Overington D. (2015, May 6). Self-luminous devices and melatonin suppression in adolescents. Lighting Res Technol. Article ID: 1477153515584979. doi: 10.1177/1477153515584979
- Gradisar, M., Wolfson, A. R., Harvey, A. G., Hale, L., Rosenberg, R., & Czeisler, C. A. (2013). The sleep and technology use of Americans: Findings from the National Sleep Foundation’s 2011 Sleep in America poll. Journal of Clinical Sleep Medicine, 9, 1291–1299.
- Gulati, A., Sullivan, R., Buring, J. E., Sullivan, D. A., Dana, R., & Schaumberg, D. A. (2006). Validation and repeatability of a short questionnaire for dry eye syndrome. American Journal of Ophthalmology, 142, 125–131.10.1016/j.ajo.2006.02.038
- Harle, D. E., & Evans, B. J. (2004). The optometric correlates of migraine. Ophthalmic and Physiological Optics, 24, 369–383.10.1111/opo.2004.24.issue-5
- Heath, M., Sutherland, C., Bartel, K., Gradisar, M., Williamson, P., Lovato, N., & Micic, G. (2014). Does one hour of bright or short-wavelength filtered tablet screenlight have a meaningful effect on adolescents’ pre-bedtime alertness, sleep, and daytime functioning? Chronobiology International, 31, 496–505.10.3109/07420528.2013.872121
- Higuchi, S., Motohashi, Y., Liu, Y., & Maeda, A. (2005). Effects of playing a computer game using a bright display on presleep physiological variables, sleep latency, slow wave sleep and REM sleep. Journal of Sleep Research, 14, 267–273.10.1111/jsr.2005.14.issue-3
- Higuchi, S., Nagafuchi, Y., Lee, S. I., & Harada, T. (2014). Influence of light at night on melatonin suppression in children. The Journal of Clinical Endocrinology & Metabolism, 99, 3298–3303.10.1210/jc.2014-1629
- Ide, T., Toda, I., Miki, E., & Tsubota, K. (2015). Effect of blue light–reducing eye glasses on critical flicker frequency. Asia-Pacific Journal of Ophthalmology, 4, 80–85.10.1097/APO.0000000000000069
- Kaido, M., Dogru, M., Ishida, R., & Tsubota, K. (2007). Concept of functional visual acuity and its applications. Cornea, 26, S29–S35.10.1097/ICO.0b013e31812f6913
- Kaido, M., Kawashima, M., Yokoi, N., Fukui, M., Ichihashi, Y., Kato, H., ... Tsubota, K. (2015). Advanced dry eye screening for visual display terminal workers using functional visual acuity measurement: The Moriguchi study. British Journal of Ophthalmology, 99, 1488–1492.10.1136/bjophthalmol-2015-306640
- Kaido, M., Toda, I., Oobayashi, T., Kawashima, M., Katada, Y., & Tsubota, K. (2016). Reducing short-wavelength blue light in dry eye patients with unstable tear film improves performance on tests of visual acuity. PLoS ONE, 11, e0152936.10.1371/journal.pone.0152936
- Matynia, A., Parikh, S., Deot, N., Wong, A., Kim, P., Nusinowitz, S., & Gorin, M. B. (2015). Light aversion and corneal mechanical sensitivity are altered by intrinscally photosensitive retinal ganglion cells in a mouse model of corneal surface damage. Experimental Eye Research, 137, 57–62.
- Oba, S., Nakamura, K., Sahashi, Y., Hattori, A., & Nagata, C. (2008). Consumption of vegetables alters morning urinary 6-sulfatoxymelatonin concentration. Journal of Pineal Research, 45, 17–23.10.1111/jpi.2008.45.issue-1
- Sakane, Y., Yamaguchi, M., Yokoi, N., Uchino, M., Dogru, M., Oishi, T., ... Ohashi, Y. (2013). Development and Validation of the Dry Eye–Related Quality-of-Life Score Questionnaire. JAMA Ophthalmology, 131, 1331–1338.10.1001/jamaophthalmol.2013.4503
- Srinivasan, S., Chan, C., & Jones, L. (2007). Apparent time-dependent differences in inferior tear meniscus height in human subjects with mild dry eye symptoms. Clinical and Experimental Optometry, 90, 345–350.10.1111/cxo.2007.90.issue-5
- Tsubota, K., Hata, S., Okusawa, Y., Egami, F., Ohtsuki, T., & Nakamori, K. (1996). Quantitative videographic analysis of blinking in normal subjects and patients with dry eye. Archives of Ophthalmology, 114, 715–720.10.1001/archopht.1996.01100130707012
- Tsubota, K., & Nakamori, K. (1993). Dry eyes and video display terminals. New England Journal of Medicine, 328, 584.10.1056/NEJM199302253280817
- Turner, P. L., & Mainster, M. A. (2008). Circadian photoreception: Ageing and the eye’s important role in systemic health. British Journal of Ophthalmology, 92, 1439–1444.10.1136/bjo.2008.141747
- Uchino, M., Yokoi, N., Uchino, Y., Dogru, M., Kawashima, M., Komuro, A., ... Tsubota, K. (2013). Prevalence of dry eye disease and its risk factors in visual display terminal users: The Osaka study. American Journal of Ophthalmology, 156, 759–766.10.1016/j.ajo.2013.05.040
- van der Lely, S., Frey, S., Garbazza, C., Wirz-Justice, A., Jenni, O. G., Steiner, R., ... Schmidt, C. (2015). Blue blocker glasses as a countermeasure for alerting effects of evening light-emitting diode screen exposure in male teenagers. Journal of Adolescent Health, 56, 113–119.10.1016/j.jadohealth.2014.08.002
- Walker, P. M., Lane, K. J., Ousler, 3rd., G. W., & Abelson, M. B. (2010). Diurnal variation of visual function and the signs and symptoms of dry eye. Cornea, 29, 607–612.10.1097/ICO.0b013e3181c11e45
- Wilkins, A. J., Patel, R., Adjamian, P., & Evans, B. J. (2002). Tinted spectacles and visually sensitive migraine. Cephalalgia, 22, 711–719.10.1046/j.1468-2982.2002.00362.x