Abstract
Bisphosphonates are used to reduce pathological osteolysis in bone cancer patients. In addition to direct effects on tumor cells, these drugs may also alter the production of immune cell factors within the tumor microenvironment. Interferon-γ (IFN-γ) represents one such factor whose production by T lymphocytes is increased following bisphosphonate treatment. This study characterized the effects of alendronate (ALN), an aminobisphosphonate, and IFN-γ on viability, maturation, and osteoclastic factor production in human G292 osteosarcoma cells. Viability was assessed with a colorimetric assay; maturation by alkaline phosphatase (ALP) and mineralization via alizarin red. Receptor activator of nuclear factor kappa-Β ligand (RANKL) and monocyte chemoattractant protein-1 (MCP-1) production were quantified with ELISAs. ALN (5 nM) had no effect on viability. IFN-γ (1,000 U/mL) decreased this parameter alone and in the presence of ALN. ALN had a transient inhibitory effect on ALP. While IFN-γ increased this parameter, ALN inhibited this effect. Whereas ALN and IFN-γ each decreased RANKL, cotreatment with IFN-γ lessened the inhibitory effect of ALN. ALN decreased MCP-1 and attenuated IFN-γ induced increases. These studies suggest that bisphosphonates have direct effects on bone tumor cells and on the actions of cytokines in the tumor microenvironment and provide a basis for optimization of bone cancer therapy.
Public Interest Statement
Metastases of cancer to the bone have serious consequences for cancer patients. Bisphosphonates have been used extensively to reduce skeletal effects in many types of cancer. These drugs may prevent bone loss often associated with the disease and its treatment. Although the use of bisphosphonates is most often beneficial for patients, studies are needed to optimize the use of these drugs and to alleviate some of their potential side effects on bone. In addition to direct effects on tumor cells, bisphosphonates may also alter the production of factors by immune cells such as interferon-γ (IFN-γ) within the bone tumor interface. We focused here on the in vitro effects of alendronate, a commonly used bisphosphonate, on human osteosarcoma cells. We studied alendronate alone as well as in combination with IFN-γ and found that there are interactions potentially important for consideration in optimization of the drug’s effects on bone cancers.
Competing interests
The authors declare no competing interest.
1. Introduction
Osteosarcoma (OS) is a skeletal lesion consisting of mutated osteoblastic cells with abnormal/heterogeneous phenotypes and altered gene expression profiles (Zhu et al., Citation2015). Normal bone homeostasis in vertebrates depends on a fine balance between the activities of matrix-synthesizing osteoblasts and bone-resorbing osteoclasts. However, in patients with primary bone tumors or malignant tumors that have metastasized to osseous tissues, this balance is disrupted via the production of various osteoclastic and pro-tumor factors by OS cells (Kansara, Teng, Smyth, & Thomas, Citation2014; Lopes-Júnior et al., Citation2016). When these factors are released into the bone tumor microenvironment, they alter the gene expression profiles of resident osteoblastic and bone marrow stromal cells, priming them to secrete additional osteoclastic and pro-tumor factors (Nannuru & Singh, Citation2010). Ultimately, the net result of these events is progressive osteolysis due to unrestricted osteoclast-mediated bone resorption. The accompanying pathological bone loss can have dire consequences for OS patients as it predisposes them to skeletal fractures, neurologic compression, and hypercalcemia (Goblirsch, Zwolak, & Clohisy, Citation2005). Despite progress in the development of multimodal treatment regimens, approximately 40% of OS patients continue to experience treatment failure within 5 years of diagnosis (Mirabello, Troisi, & Savage, Citation2009). This warrants the investigation of new therapeutic approaches, which should consider targeting the bone tumor microenvironment in addition to the tumor itself.
Bisphosphonate are currently the major class of drugs used to prevent and treat skeletal complications associated with tumor-induced osteolysis (Giger, Castagner, & Leroux, Citation2013; Van Poznak et al., Citation2011). In a clinical study involving patients with inoperable benign bone tumors, bisphosphonate-treated lesions were reported to show improved bone mineral density with a partial or complete vanishing of osteolytic activity (Cornelis et al., Citation2014). Moreover, a recent evaluation by the Children’s Oncology Group determined that the bisphosphonate zoledronic acid can be safely combined with conventional chemotherapy for patients with metastatic OS (Goldsby et al., Citation2013). Although these studies provide important information regarding the feasibility and efficacy of bisphosphonates for treating OS, the effects of these drugs on the molecular interplay between the different cell types of the bone tumor microenvironment remain unclear.
Bisphosphonates are synthetic analogues of inorganic pyrophosphate that strongly adsorb to hydroxyapatite crystals and suppress osteoclast-mediated bone resorption. While they were initially thought to act primarily on osteoclasts, recent investigations have demonstrated that bisphosphonates can modulate the production of specific osteoclastic factors by both osteoblastic and OS cells (Kobayashi, Hirano, & Makino, Citation2005; Ohba et al., Citation2014; Pan et al., Citation2004). These drugs have also been shown to be capable of stimulating the proliferation of γδ T cells—a subpopulation of cytotoxic T lymphocytes that is most abundant in the peripheral blood supply—and promoting their differentiation toward an anti-tumor and anti-resorptive phenotype (Kunzmann et al., Citation2000; Li, Peng, Xu, & Ye, Citation2012; Mariani et al., Citation2005; Sato et al., Citation2005). It is therefore postulated that bisphosphonates can indirectly reduce tumor-induced osteolysis not only by modulating the production of osteoclastic factors by both osteoblastic and OS cells, but also by stimulating the proliferation and differentiation of γδ T cells.
Two key osteoclastic factors suggested to be responsible for the progression of tumor-induced osteolysis are: receptor activator of nuclear factor kappa-Β (RANKL) and monocyte chemoattractant protein-1 (MCP-1) (Cote, Citation2015; Lu et al., Citation2007). A clinical study reported that elevated RANKL expression in biopsies taken from patients with localized, high-grade OS is correlated with poorer prognosis and lower chance of survival (Lee et al., Citation2011). Moreover, a recent in vitro study reported a positive correlation between the aggressive potential of murine and human OS cell lines and MCP-1 expression (Ohba et al., Citation2014). Taken together, these findings justify the development of therapies targeted against RANKL and MCP-1 expression in OS cells.
Another mechanism suggested to be responsible for the progression of tumor-induced osteolysis is the elevated concentrations of immunosuppressive cytokines at the bone/tumor interface and their inhibitory effects on γδ T cells (Fournier, Chirgwin, & Guise, Citation2006). These particular lymphocytes are specialized at recognizing tumor cells and are an important source of interferon-γ (IFN-γ), which is an immunomodulatory cytokine with a pleiotropic role in bone metabolism (Gao et al., Citation2003; Kabelitz, Wesch, & He, Citation2007). In some cases, IFN-γ strongly suppresses osteoclastogenesis by interfering with the binding interaction between RANKL and its cognate receptor RANK, which is expressed on the surface of osteoclast precursors (Takayanagi, Citation2000). In other cases, IFN-γ appears to promote osteoclastogenesis by initiating a signaling cascade that stimulates T cells to produce RANKL (Kotake et al., Citation2005; Sato et al., Citation2006). Based on these observations, it is clear that the effects of IFN-γ are complex and probably depend on the momentary state of other cytokine systems within the bone tumor microenvironment.
Attempting to elucidate the molecular mechanisms underlying the osteoprotective effects of bisphosphonate treatment, numerous studies have focused on the ability of these drugs to alter the gene expression profiles of different cell types within the bone tumor microenvironment (Bukowski, Dascher, & Das, Citation2005). In this context, recent studies suggest that bisphosphonates may reduce the incidence of osteolytic processes by inhibiting tumor expression of osteoclastic activators (Kobayashi et al., Citation2005; Ohba et al., Citation2014). Additionally, preclinical findings indicate that after being activated in vivo via intravenous bisphosphonate treatment, γδ T cells from OS patients begin to increase their production of IFN-γ (Dieli et al., Citation2003). It therefore seems plausible that the inhibitory action of bisphosphonates on osteolytic processes may be partly related to their modulatory effect on the concentrations of particular signaling molecules at the bone/tumor interface. To our knowledge, the combined effects of bisphosphonates and IFN-γ on OS cells have not yet been thoroughly described. It is also possible that the observed effects may differ, depending on the bisphosphonate studied and the experimental model used. The purpose of the present study was to explore the combined effects of alendronate (ALN), a nitrogen-containing bisphosphonate, and IFN-γ on the production of alkaline phosphatase (ALP), receptor activator of nuclear factor-κβ ligand (RANKL), and monocyte chemoattractant protein-1 (MCP-1) by human osteosarcoma (HOS) cells with specific emphasis on G292 cells, a well-characterized osteosarcoma cell line. We also tested the effects of these agents on osseous mineralization by these cells.
2. Materials and methods
2.1. Chemicals and reagents
ALN was purchased from United States Pharmacopeia (Rockville, MD). Human recombinant IFN-γ was purchased from R&D Systems (Minneapolis, MN). Stock solutions of ALN and IFN-γ were prepared by dissolving the substances in distilled deionized water. All other chemicals and reagents used were purchased from Sigma-Aldrich (St. Louis, MO) unless otherwise stated.
2.2. Cell culture conditions
The human osteosarcoma-derived cell line G292 was obtained from the American Type Culture Collection (Manassas, VA) and grown in 75 cm2 plastic culture flasks (Falcon, Oxnard, CA) containing minimum essential medium-alpha (MEM-α; Gibco Life Technologies, Grand Island, NY) supplemented with 10% fetal bovine serum (Gibco Life Technologies) and a 1% anti-mycotic solution (Gibco Life Technologies). Cultures were maintained at 37°C in a humidified incubator with 5% CO2. The media was refreshed every 2 to 3 days, and cells were passaged 1 to 2 times per week. Conditioned media with the desired experimental concentrations of ALN (5 nM) and IFN-γ (1,000 U/mL) was prepared by diluting the stock solutions with serum-free MEM not containing phenol red. Cells untreated with either ALN or IFN-γ, but with equivalent amounts of serum free –free MEM, served as control groups.
2.3. Cell viability assay
Cell viability at two different time points (24 and 48 h) was assayed by reduction of yellow tetrazolium dye (MTT) to violet formazan crystals by mitochondrial enzymes. Cells were seeded onto two 24-well plates in 500 μL of conditioned media, either treated with ALN (5 nM) and/or IFN-γ (1,000 U/mL) or with equivalent amounts of serum-free MEM for controls. A separate plate was used for each experimental time period. At each time point, 50 μL of MTT reagent was added to the culture media, and the plate was incubated for 2 h at 37°C. Following this, the precipitated formazan crystals were dissolved by adding 500 μL of 0.1 N HCl/isopropanol to each well, and the plate was incubated for an additional 30 min at 37°C. The plate was then vigorously shaken on a microplate shaker (VWR, Bridgeport, NJ) for 15 min at room temperature to ensure that the formazan crystals were adequately dissolved. Finally, two aliquots (200 μL) of supernatant from each well were transferred to a 96-well plate, and the optical densities of the samples were measured by absorbance at 570 nm in a microplate reader (FlexStation 3 Multi-Mode Microplate Reader, Molecular Devices, Sunnyvale, CA).
2.4. Alkaline phosphatase (ALP) activity assay
Cellular ALP activity at three different time points (24, 48, and 72 h) was assayed by conversion of colorless ρ-nitrophenyl phosphate (ρNPP) to yellowish ρ-nitrophenol (ρNp). G292 cells were seeded onto three 96-well plates in 200 μL of conditioned media, either with ALN (5 nM) and/or IFN-γ (1,000 U/mL) or with equivalent amounts of serum-free MEM for controls. A separate plate was used for each experimental time period (24, 48, and 72 h). At each time point, conditioned media was aspirated and the cells were incubated in 105 μL of 1% (v/v) Triton X-100 (Avantor Performance Materials, Center Valley, PA) for 1 h at 4°C. Following this, 50 μL of aminomethyl propanol (AMP) and 50 μL of ρNpp reagent were added to each well. The cells were then incubated for 30 min at 37°C, during which time the enzymatic reaction proceeded. The reaction was stopped by adding 50 μL of 0.5 N NaOH to each well, and ALP activity was measured by absorbance at 405 nm in a microplate reader (Molecular Devices). The enzyme activity (nmol/min/mg) was calibrated with a ρNPP standard curve and normalized with the total protein content in aliquots (5 μL) of the same samples, which were collected and transferred to a new 96-well plate prior to the addition of AMP and ρNPP reagent. Total protein content was determined with the Bio-Rad DC Protein Assay (Bio-Rad Laboratories, Rockford, IL) for detergent-solubilized cells and calculated according to a series of bovine serum albumin (BSA) standards.
2.5. Mineralization assay
Mineralized nodule formation was quantified after a 7-day incubation period using alizarin red S (ARS) staining and cetylpyridinium chloride (CPC) extraction based on the protocol described by Gregory et al. Cells were seeded onto a 24-well plate in 500 μL of conditioned media, either treated with ALN (5 nM) and/or IFN-γ (1,000 U/mL or with equivalent amounts of serum-free MEM for controls. After seven days, the G292 cells were washed with Dulbecco’s phosphate-buffered saline (DPBS, Mediatech Inc., Manassas, VA) and fixed with ice-cold 70% (v/v) ethanol for 1 h at 4°C. The ethanol was then removed and the cells were washed twice with molecular biology grade water (Mediatech Inc.) before being incubated in 500 μL of 40 mM ARS solution, pH 4.2, for 10 min at room temperature with gentle agitation. After absorbing the dye, the cells were washed five times with molecular biology grade water and once with DPBS to remove any residual stain. The plates were stored overnight at −20°C prior to dye extraction. ARS was solubilized by incubating the cells in 500 μL of 10% (w/v) CPC solution, pH 7.0, for 1 h at room temperature with shaking. Finally, two aliquots (200 μL) of supernatant from each well were transferred to a 96-well plate, and mineralized nodule formation was quantified by absorbance at 550 nm in microplate reader (Molecular Devices).
2.6. Enzyme-linked immunosorbent assay (ELISA)
G292 cells were seeded onto a 96-well plate in 200 μL of conditioned media, either treated with ALN (5 nM) and/or IFN-γ (1,000 U/mL) or with equivalent amounts of serum-free MEM for controls. Following a 24 h incubation period, the culture supernatants were tested with quantitative colorimetric ELISAs for human MCP-1 (R&D Systems) and human RANKL (Boster Biological Technology, Pleasanton, CA) according to the respective manufacturer’s directions.
2.7. Analysis of data
Results are presented as the means ± standard error of mean (SEM). Statistical computations were performed using Microsoft® Excel software (Microsoft Corporation, Redmond, WA). After verifying normality with a Fisher’s exact test, a two-sided Student’s t-test for paired samples was used to test for differences within groups during time course experiments, and one-way analysis of variance (ANOVA) was used to test for differences among groups. Statistical significance was accepted for p values ≤ 0.01.
3. Results
3.1. Evaluation of cell viability
A reduction in MTT measurement indicates a loss in cell viability and can also be interpreted as representing cytotoxicity (Fotakis & Timbrell, Citation2006). With that in mind, the individual and combined effects of ALN and IFN-γ on the viability of G292 cells were examined at 24 and 48 h. Collectively, under both control and experimental conditions, Figure shows that cell viability significantly decreased between 24 and 48 h. In comparison among the treatment groups at each of the indicated time points, it was found that incubating the cells in the presence of ALN only weakly increased their viability at 24 h and had no effect at 48 h, while incubating them with IFN-γ significantly reduced it at both 24 and 48 h (Figure ). Interestingly, when applied together, ALN and IFN-γ did not affect cell viability at 24 h, but the combination significantly reduced it at 48 h (Figure ).
Figure 1. Cell viability assay. G292 osteosarcoma cells were incubated for 24 or 48 h with ALN (5 nM) and/or IFN-γ (1,000 U/mL) under the conditions described in the text. Controls were incubated under identical conditions without added agents. Viability was measured with the MTT assay and expressed as absorbance at 570 nm. Results are expressed as the mean absorbance ± SEM with n = 4 samples per group. ALN added alone produced no significant effects at either time period; IFN-γ produced significant decreases when added alone at 24 and 48 h and in the presence of ALN at 48 h.
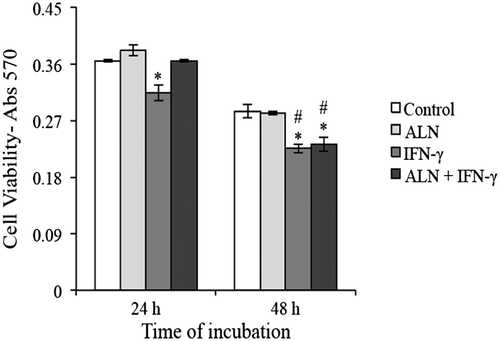
3.2. Evaluation of ALP activity
The level of ALP activity exhibited by G292 cells in response to treatment with ALN and/or IFN-γ was examined over a 72 h incubation period. Figure shows that during the course of the experiment, a similar trend in ALP activity was present under all treatment conditions although the inhibitory effect of ALN alone was significant only at 24 h (Figure ). In comparing the level of ALP activity among the treatment groups at each of the indicated time points, substantial variations were detected in the presence of IFN-γ. Notably, it was found that exposing the cells to IFN-γ significantly enhanced ALP activity at each of the indicated time points, as compared to levels in the presence of either ALN or in control medium (Figure ). On the other hand, while costimulation with ALN plus IFN-γ did not significantly enhance ALP activity compared to control conditions at 24 h, it significantly increased it at 48 and 72 h (Figure ). The results also suggest that ALN inhibits the stimulatory effects of IFN-γ on ALP activity. At all time periods tested (24, 48, and 72 h), ALN plus IFN-γ, applied together, significantly attenuated the stimulatory effect induced by IFN-γ alone on ALP activity (Figure ). However, compared to the control group, no difference in ALP activity was detected in the presence of ALN alone at 48 or 72 h, indicating that ALN-induced inhibition of ALP activity in G292 cells is probably temporal and begins to diminish following 48 h of incubation (Figure ).
Figure 2. ALP assay. G292 osteosarcoma cells were incubated for 24, 48 or 72 h with ALN (5 nM) and/or IFN-γ (1,000 U/mL) under the conditions described in the text. Controls were incubated under identical conditions without added agents. ALP activity was measured with the pNPP assay; values normalized per mg protein measured with a Bio-Rad DC Protein Assay and expressed as nmoles/min/mg protein. Results are expressed as the mean ± SEM with n = 4 samples per group. ALN alone produced significant decreases at 24 h and IFN-γ alone significant increases at all three time periods but the presence of ALN resulted in significant decreases in the IFN-γ effect.
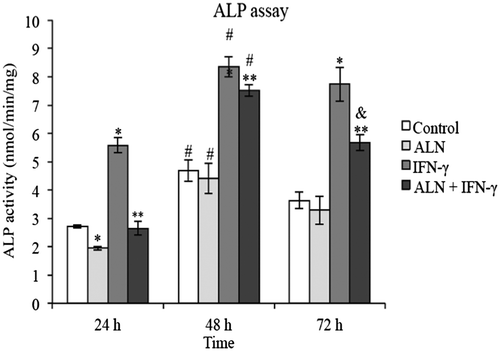
3.3. Assessment of mineralization
Extracellular matrix calcification in G292 cells was evaluated using ARS staining. After 7 days in culture, mineralized nodules were detected in each of the treatment groups. Figure shows that the level of mineralization demonstrated by the control group was significantly less than the experimental groups. Meanwhile, IFN-γ treatment was observed to significantly elevate mineralization, with IFN-γ treated cells showing the highest degree of mineralization (Figure ). However, this amplifying effect was significantly diminished in the presence of ALN, suggesting that ALN might have an inhibitory effect on IFN-γ stimulated mineralization (Figure ). Furthermore, while ALN treated cells showed significantly less mineralization than IFN-γ treated cells, they showed significantly greater mineralization than the cells treated with ALN in combination with IFN-γ (Figure ). This suggests that ALN and IFN-γ might counteract one another’s stimulatory effect on mineralization in G292 cells.
Figure 3. ARS mineralization assay. G292 osteosarcoma cells were incubated for 7 days with ALN (5 nM) and/or IFN-γ (1,000 U/mL) under the conditions described in the text. Controls were incubated under identical conditions without added agents. Mineralization was measured with alizarin red staining and expressed as absorbance at 550 nm. Results are expressed as the mean absorbance ± SEM with n = 4 samples per group. ALN incubated alone produced a significant increase in mineralization compared to controls as did incubation with IFN-γ alone. However, incubation with the combination of ALN and IFN-γ significantly decreased these effects.
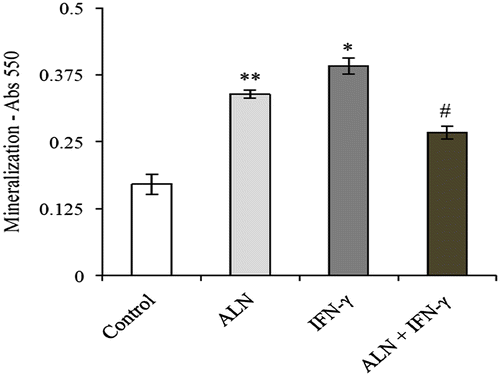
3.4. Production of osteoclastic factors
The effects of ALN and IFN-γ on the secretion of RANKL and MCP-1 by G292 cells were assayed by ELISA. After 24 h of incubation, measurable amounts of both these osteoclastic factors were detected in the culture supernatants of each treatment group.
Figure (A) shows that the amount of RANKL secreted by the control cells was significantly greater compared to that of the cells in the other treatment groups. ALN treatment alone resulted in significantly less RANKL production compared to the other experimental groups (Figure ). IFN-γ treatment alone was also observed to significantly reduce the amount of RANKL secreted compared to that of the control cells, but the inhibitory effect was significantly less than that observed following ALN alone treatment (Figure (A)). Interestingly, no significant difference was observed in the amount of RANKL secreted by cells stimulated with IFN-γ and cells costimulated with ALN plus IFN-γ, suggesting that IFN-γ may lessen the magnitude of ALN induced inhibition of RANKL secretion (Figure (A)).
Figure 4. Production of osteoclastic factors. G292 osteosarcoma cells were incubated for 24 h with ALN (5 nM) and/or IFN-γ (1,000 U/mL) under the conditions described in the text. Controls were incubated under identical conditions without added agents. (A) RANKL Production. Measurements were made with a ELISA assay specific for RANKL and results are expressed as the mean pg/mL ± SEM with n = 4 samples per group. Both ALN and IFN-γ alone produced decreases compared to controls with ALN having a slightly greater decrease. The combination treatment of ALN and IFN-γ did not modify the decrease observed with IFN-γ alone. *p < 0.01 vs. untreated control. **p < 0.01 vs. in the presence of ALN alone. (B) MCP-1 Production. Measurements were made with a ELISA assay specific for MCP-1 and results are expressed as the mean pg/mL ± SEM with n = 4 samples per group. ALN alone produced significant decreases compared to controls while IFN-γ alone produced significant increases. The combination of ALN and IFN-γ resulted in a significant attenuation of the IFN-γ effect. *p < 0.01 vs. untreated control. **p < 0.01 vs. in the presence of IFN-γ alone.
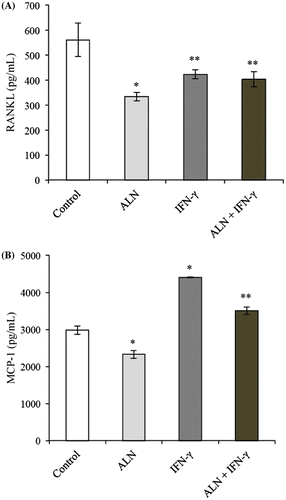
Figure (B) shows that ALN significantly attenuated control MCP-1 secretion while IFN-γ had a robust stimulatory effect. In addition, it was found that costimulation with ALN and IFN-γ significantly reduced the amount of MCP-1 secreted compared to stimulation with IFN-γ alone, suggesting that ALN may attenuate the stimulatory effect induced by IFN-γ on MCP-1 secretion (Figure (B)).
4. Discussion
The physiochemical mechanisms underlying the antitumor and antiresorptive effects of bisphosphonates remain unclear. It is hypothesized that these drugs can inhibit the progression of tumor-induced osteolysis through their interactions with multiple cell types at the bone/tumor interface (Bukowski et al., Citation2005). During such interactions, the gene expression profile of these cell types becomes altered, leading to shifts in the local concentrations of particular cytokines. The aim of the present study was to describe the effects of ALN and IFN-γ on the viability, ALP activity, mineralization capacity and RANKL, and MCP-1 expression in the HOS cell line G292.
Although previous studies on the effects of ALN in G292 cells do not appear to have been published, studies with another HOS cell line, MG-63, found biphasic effects with ALN at doses from 10 nM to 10 μM with inhibitory effects on proliferation at the high dose and optimal stimulatory effects at 10 nM (Im, Qureshi, Kenney, Rubash, & Shanbhag, Citation2004). In order to understand possible interactions with the immune cell factors and ALN, it was our goal to test ALN at a concentration that did not result in cytotoxic effects over the course of at least 48 h when no other factors were added. Since we observed that ALN, at a dose of 5 nM, does not have a cytotoxic effect on G292 cells when tested under these incubation conditions, all of the experiments reported here were conducted at this dose. While ALN significantly reduced the ALP activity in these cells at 24 h, it was not observed to have a significant effect thereafter, neither at 48 nor 72 h. With regard to effects of IFN-γ on G292 cells, we observed that IFN-γ can decrease viability and increase ALP activity in these cells, and that these effects are attenuated in the presence of ALN. Moreover, we found that IFN-γ has similar effects as high dosages of ALN on both the viability and ALP activity in G292 cells. While it is possible that these effects may become additive in the presence of IFN-γ and high concentrations of ALN, these effects may vary depending on the HOS cell line studied. In support of this notion, Beresford et al. studied the effects of IFN-γ (at doses of 5–1,000 U/mL) on ALP activity in the HOS cell line TE 85 and found that it significantly reduced ALP activity in these cells, to the same extent across all the doses evaluated (Beresford, Taylor, & Teiffitt, Citation1990). In vivo, given that bisphosphonates are preferentially incorporated into the bone remodeling compartment and the capacity of these drugs to activate immunosuppressed γδ T cells, high local concentration of ALN and IFN-γ would be expected to be found at the bone/tumor interface in OS patients. Along this line, additional studies need to be conducted on the combined effects of both these agents, when applied at high dosages, on HOS cells.
In evaluating the mineralization capacity of G292 cells incubated in the presence of ALN and/or IFN-γ, we found that ALN and IFN-γ added separately stimulated mineralization, but, when applied together, reduced it. Contradictory to our findings, Huang et al. showed that the aminobisphosphonate zoledronate significantly reduced mineralization in both the G292 and MG-63 HOS cell lines (Huang, Cheng, Chuang, & Yang, Citation2015). However, the effects they reported were shown to be dose-dependent, thereby suggesting that they can be expected to disappear at lower concentrations of zoledronate. While high concentrations of bisphosphonates may be useful in that they could abate the production of abnormal mineralized matrix by HOS cells, they have also been demonstrated to have a harmful effect on the survival and function of human osteoblasts (Hofstaetter et al., Citation2013; Huang et al., Citation2015). In this light, it would seem that bisphosphonate treatment would be ineffective in impeding the progressive loss of bone associated with tumor-induced osteolysis. However, clinical findings indicate that bisphosphonates can stimulate bone formation in OS patients suffering from bone pain due to osteolysis, thereby suggesting that the aforementioned in vitro findings are unlikely to represent an entirely accurate model for what’s transpiring at the bone/tumor interface in vivo (Cornelis et al., Citation2014). Regarding our data on the effects of IFN-γ on mineralization in G292 cells, we found that IFN-γ had a stimulatory effect similar to that of ALN, but, when applied together, the stimulatory effect was less robust than either agent individually. To our knowledge, the vitro effects of IFN-γ on mineralization in HOS cells have yet to be thoroughly investigated. On this basis, our results suggest that increased local concentrations of IFN-γ at the bone-tumor interface could be deleterious in that it appears to promote matrix mineralization in HOS cells. Further studies need to be conducted on the combined effects of ALN and IFN-γ to determine whether or not they are translatable to what may be occurring in vivo at the bone/tumor interface.
Finally, we evaluated the effects of ALN and IFN-γ on the secretion of RANKL and MCP-1 by G292 cells. Although several studies report on not being able to detect the presence of RANKL in the culture supernatants of HOS cells, our results from the present study show that we were able to detect measurable of amounts of RANKL under both the control and experimental conditions. In that regard, we found that both ALN and IFN-γ attenuated the secretion of RANKL by G292 cells, but the effect was greater for ALN. In agreement with the notion of bisphosphonate-induced inhibition of RANKL secretion, Ohba et al. found that zoledronate downregulated the expression of RANKL mRNA in the HOS cell lines TE85 and 143B (Ohba et al., Citation2014). Moreover, akin to what we observed in the present study, with ALN being able to attenuate MCP-1 secretion, these authors found that zoledronate also attenuated MCP-1 expression by HOS cells. Both the attenuation of RANKL and MCP-1 are likely to provide substantial therapeutic benefits in the treatment of OS. The reason for this is because RANKL facilitates osteoclast maturation and subsequent bone resorption, while MCP-1 promotes connective tissue damage, which, in turn, promotes OS cell invasiveness (Ohba et al., Citation2014). Regarding the effects of IFN-γ on the secretion of these osteoclastic factors, we observed decreases in RANKL with increases in MCP-1 in the culture supernatants of G292 cells exposed to this cytokine. Furthermore, while the magnitude of the inhibitory effect of IFN-γ on RANKL secretion was unchanged in the presence of ALN, the level of IFN-γ-stimulated MCP-1 secretion was significantly less in G292 cells exposed to ALN plus IFN-γ. Regarding the therapeutic benefits associated with increases in local concentrations of IFN-γ at the bone/tumor interface, while a decrease in the amount of RANKL is favorable, it is unlikely to outweigh the cons of increases in the amount of MCP-1. This is because MCP-1 is a chemokine that promotes connective tissue damage, which, in turn, promotes OS cell metastasis by facilitating bone destruction. With that in mind, it is possible that ALN-induced inhibition of IFN-γ-stimulated MCP-1 production could outweigh the cons of enhanced MCP-1 secretion due to increases in local concentrations of IFN-γ at the bone/tumor interface.
In conclusion, the studies presented here suggest that bisphosphonates such as ALN may have direct effects on bone tumor cells to modulate their activity and differentiation including their ability to secrete factors with effects on osteolysis. The effect of the interactions of a noncytotoxic concentration of ALN with IFN-γ presented here suggests that this bisphosphonate may also modulate the actions of local cytokines known to be present at the bone/tumor interface. These results may contribute to a better understanding of the interaction of bisphosphonates and local cytokines in the bone tumor microenvironment and development of approaches to optimize the beneficial effects of this class of drugs.
Funding
Funding for this research was from UBSDM Oral Biology departmental general support as well as for Andrew Barone via the Summer Research Program at UBSDM funded by the Dean’s Office.
Acknowledgements
The authors wish to thank Dr Dinh Nguyen of the Department of Oral Biology at the University at Buffalo for his technical assistance with the maintenance of the G292 cells.
Additional information
Notes on contributors
Andrew W. Barone
Andrew Barone is pursuing a DDS at the University at Buffalo as well as conducting research in the laboratory of Rosemary Dziak, PhD. His research interests have focused on bone regeneration with particular interests in tissue engineering of osteoconductive scaffolds as well as regulatory events in the microenvironment of osteosarcomas.
Gabriela Fernandes
Gabriela Fernandes participated in this study as a post-doctoral fellow in the Department of Oral Biology at the University at Buffalo. She received her MS in Oral Sciences from that Institution having obtained a BDS from YCMM & RDF Dental College. Her research interests have been in tissue engineering and regulation of skeletal remodeling.
Rosemary Dziak
Rosemary Dziak, PhD, is a professor of Oral Biology at the University at Buffalo. Her research is focused on osteoblastic cell mediated events. She has studied the mechanism of action of many agents that have effects on pathological and physiological bone metabolism.
References
- Beresford, J. N., Taylor, G. T., & Teiffitt, J. T. (1990). Interferons and bone. The FEBS Journal, 193(2), 589–597. doi:10.1111/j.1432-1033.1990.tb19377.x
- Bukowski, J. F., Dascher, C. C., & Das, H. (2005). Alternative bisphosphonate targets and mechanisms of action. Biochemical and Biophysical Research Communications, 328(3), 746–750. doi:10.1016/j.bbrc.2004.11.075
- Cornelis, F., Truchetet, M. E., Amoretti, N., Verdier, D., Fournier, C., Pillet, O., … Hauger, O. (2014). Bisphosphonate therapy for unresectable symptomatic benign bone tumors: A long-term prospective study of tolerance and efficacy. Bone, 58, 11–16. doi:10.1016/j.bone.2013.10.004
- Cote, G. M. (2015). Rank ligand as a target in musculoskeletal neoplasms. Current Reviews in Musculoskeletal Medicine, 8(4), 339–343. doi:10.1007/s12178-015-9310-y
- Dieli, F., Gebbia, N., Poccia, F., Caccamo, N., Montesano, C., Fulfaro, F., … Sireci, G. (2003). Induction of γδ T-lymphocyte effector functions by bisphosphonate zoledronic acid in cancer patients in vivo. Blood, 102(6), 2310–2311. doi:10.1182/blood-2003-05-1655
- Fotakis, G., & Timbrell, J. A. (2006). In vitro cytotoxicity assays: Comparison of LDH, neutral red, MTT and protein assay in hepatoma cell lines following exposure to cadmium chloride. Toxicology Letters, 160(2), 171–177. doi:10.1016/j.toxlet.2005.07.001
- Fournier, P. G., Chirgwin, J. M., & Guise, T. A. (2006). New insights into the role of T cells in the vicious cycle of bone metastases. Current Opinion in Rheumatology, 18(4), 396–404. doi:10.1097/01.bor.0000231909.35043.da
- Gao, Y., Yang, W., Pan, M., Scully, E., Girardi, M., Augenlicht, L. H., … Yin, Z. (2003). γδ T cells provide an early source of interferon γ in tumor immunity. Journal of Experimental Medicine, 198(3), 433–442. doi:10.1084/jem.20030584
- Giger, E. V., Castagner, B., & Leroux, J. C. (2013). Biomedical applications of bisphosphonates. Journal of Controlled Release, 167(2), 175–188. doi:10.1016/j.jconrel.2013.01.032
- Goblirsch, M. J., Zwolak, P., & Clohisy, D. R. (2005). Advances in understanding bone cancer pain. Journal of Cellular Biochemistry, 96(4), 682–688. doi:10.1002/jcb.20589
- Goldsby, R. E., Fan, T. M., Villaluna, D., Wagner, L. M., Isakoff, M. S., Meyer, J., … Gorlick, R. (2013). Feasibility and dose discovery analysis of zoledronic acid with concurrent chemotherapy in the treatment of newly diagnosed metastatic osteosarcoma: A report from the Children’s Oncology Group. European Journal of Cancer, 49(10), 2384–2391. doi:10.1016/j.ejca.2013.03.018
- Hofstaetter, J. G., Roschger, A., Puchner, S. E., Dominkus, M., Sulzbacher, I., Windhager, R., … Roschger, P. (2013). Altered matrix mineralization in a case of a sclerosing osteosarcoma. Bone, 53(2), 409–413. doi:10.1016/j.bone.2012.12.043
- Huang, K. C., Cheng, C. C., Chuang, P. Y., & Yang, T. Y. (2015). The effects of zoledronate on the survival and function of human osteoblast-like cells. BMC Musculoskeletal Disorders, 16(1), 355. doi:10.1186/s12891-015-0818-5
- Im, G. I., Qureshi, S. A., Kenney, J., Rubash, H. E., & Shanbhag, A. S. (2004). Osteoblast proliferation and maturation by bisphosphonates. Biomaterials, 25(18), 4105–4115. doi:10.1016/j.biomaterials.2003.11.024
- Kabelitz, D., Wesch, D., & He, W. (2007). Perspectives of γδ T cells in tumor immunology. Cancer Research, 67(1), 5–8. doi:10.1158/0008-5472.CAN-06-3069
- Kansara, M., Teng, M. W., Smyth, M. J., & Thomas, D. M. (2014). Translational biology of osteosarcoma. Nature reviews. Cancer, 14(11), 722. doi:10.1038/nrc3838
- Kobayashi, A., Hirano, F., & Makino, I. (2005). The inhibitory effect of bisphosphonates on glucocorticoid-induced RANKL expression in human cells. Scandinavian Journal of Rheumatology, 34(6), 480–484. doi:10.1080/03009740510026788
- Kotake, S., Nanke, Y., Mogi, M., Kawamoto, M., Furuya, T., Yago, T., … Kamatani, N. (2005). IFN-γ-producing human T cells directly induce osteoclastogenesis from human monocytes via the expression of RANKL. European Journal of Immunology, 35(11), 3353–3363. doi:10.1002/eji.200526141
- Kunzmann, V., Bauer, E., Feurle, J., Weißinger, F., Tony, H. P., & Wilhelm, M. (2000). Stimulation of γδ T cells by aminobisphosphonates and induction of antiplasma cell activity in multiple myeloma. Blood, 96(2), 384–392. Retrieved from http://www.bloodjournal.org/content/96/2/384
- Lee, J. A., Jung, J. S., Kim, D. H., Lim, J. S., Kim, M. S., Kong, C. B., … Koh, J. S. (2011). RANKL expression is related to treatment outcome of patients with localized, high-grade osteosarcoma. Pediatric Blood & Cancer, 56(5), 738–743. doi:10.1002/pbc.22720
- Li, Z., Peng, H., Xu, Q., & Ye, Z. (2012). Sensitization of human osteosarcoma cells to Vγ9 Vδ2 T-cell-mediated cytotoxicity by zoledronate. Journal of Orthopaedic Research, 30(5), 824–830. doi:10.1002/jor.21579
- Lopes-Júnior, L. C., Silveira, D. S. C., Vulczak, A., dos Santos, J. C., Veronez, L. C., Fisch, A., … Pereira-da-Silva, G. (2016). Emerging cytokine networks in osteosarcoma. Oncology Communications, 2. doi:10.14800/oc.1167
- Lu, Y., Cai, Z., Xiao, G., Keller, E. T., Mizokami, A., Yao, Z., … Zhang, J. (2007). Monocyte chemotactic protein-1 mediates prostate cancer–induced bone resorption. Cancer Research, 67(8), 3646–3653. doi:10.1158/0008-5472.CAN-06-1210
- Mariani, S., Muraro, M., Pantaleoni, F., Fiore, F., Nuschak, B., Peola, S., … Bruno, B. (2005). Effector γδ T cells and tumor cells as immune targets of zoledronic acid in multiple myeloma. Leukemia, 19(4), 664–670. doi:10.1038/sj.leu.2403693
- Mirabello, L., Troisi, R. J., & Savage, S. A. (2009). Osteosarcoma incidence and survival rates from 1973 to 2004. Cancer, 115(7), 1531–1543. doi:10.1002/cncr.24121
- Nannuru, K. C., & Singh, R. K. (2010). Tumor-stromal interactions in bone metastasis. Current Osteoporosis Reports, 8(2), 105–113. doi:10.1007/s11914-010-0011-6
- Ohba, T., Cole, H. A., Cates, J. M., Slosky, D. A., Haro, H., Ando, T., … Schoenecker, J. G. (2014). Bisphosphonates inhibit osteosarcoma-mediated osteolysis via attenuation of tumor expression of MCP-1 and RANKL. Journal of Bone and Mineral Research, 29(6), 1431–1445. doi:10.1002/jbmr.2182
- Pan, B., Farrugia, A. N., To, L. B., Findlay, D. M., Green, J., Lynch, K., & Zannettino, A. C. (2004). The nitrogen-containing bisphosphonate, zoledronic acid, influences RANKL expression in human osteoblast-like cells by activating TNF-α converting enzyme (TACE). Journal of Bone and Mineral Research, 19(1), 147–154. doi:10.1359/jbmr.2004.19.1.147
- Sato, K., Kimura, S., Segawa, H., Yokota, A., Matsumoto, S., Kuroda, J., … Maekawa, T. (2005). Cytotoxic effects of γδ T cells expanded ex vivo by a third generation bisphosphonate for cancer immunotherapy. International Journal of Cancer, 116(1), 94–99. doi:10.1002/ijc.20987
- Sato, K., Suematsu, A., Okamoto, K., Yamaguchi, A., Morishita, Y., Kadono, Y., … Cua, D. J. (2006). Th17 functions as an osteoclastogenic helper T cell subset that links T cell activation and bone destruction. Journal of Experimental Medicine, 203(12), 2673–2682. doi:10.1084/jem.20061775
- Takayanagi, H. (2000). T-cell-mediated regulation of osteoclastogenesis by signaling cross-talk between RANKL and IFN-γ. Nature, 408, 535–600. doi:10.1038/35046102
- Van Poznak, C. H., Temin, S., Yee, G. C., Janjan, N. A., Barlow, W. E., Biermann, J. S., … Zuckerman, D. S. (2011). American society of clinical oncology executive summary of the clinical ancepractice guideline update on the role of bone-modifying agents in metastatic breast cr. Journal of Clinical Oncology, 29(9), 1221–1227. doi:10.1200/JCO.2010.32.5209
- Zhu, Z. Q., Tang, J. S., Gang, D., Wang, M. X., Wang, J. Q., Lei, Z., … Yan, L. (2015). Antibody microarray profiling of osteosarcoma cell serum for identifying potential biomarkers. Molecular Medicine Reports, 12(1), 1157–1162. doi:10.3892/mmr.2015.3535