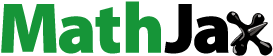
Abstract
The agricultural sector is considered to be the backbone of a countries economy. To increase the crop yield, there is an extensive use of agrochemicals in the form of insecticides, herbicides, nutrient mixture, fungicides, etc. This is necessarily involved in controlling the unwanted organisms; however, it leads to toxicity in a nearby ecosystem. Aquatic habitats being a complexed ecosystem are distressed with the increasing concentration of agrochemicals, which leads to an alteration in nontarget species at various trophic levels. Fishes are considered to be the dynamic players of this ecosystem and are the first one to be acquainted with any contaminants present in it. In this context, the present study was aimed to find the sublethal effects (1/10th of the LC50 value) of four different agrochemicals (imidacloprid—insecticide, pyrazosulfuron ethyl—herbicide, curzate—fungicide and micronutrient mixture) on the stress physiology of Oreochromis mossambicus. The hormonal titer of cortisol and gene expression of its receptor was carried out to understand the toxicity of diverse agrochemicals. The results suggested that there was a significant decrease in hormonal titer of cortisol upon the exposure of curzate and imidacloprid, with a significant downregulation of glucocorticoid receptor under the exposure of imidacloprid. Thus, the novel findings suggest that fishes were found to be devoid of stress response which may be due to hypothalamus pituitary interrenal exhaustion.
PUBLIC INTEREST STATEMENT
This article is one of the novel, depicting the negative effects of four different classes of agrochemicals on fishes. The main aim of the authors group was to check the environmental bioaccumulation and it ultimate effects in humans. Here, cortisol, one of the vital regulator of stress in an organism’s body, is being evaluated, when a toxicant enters into the body of it. Thus, this article will help the policymakers to deduce certain policies for the reduce use of agrochemicals so as to minimize its deleterious effects on the environment.
Competing Interests
The author declares that the present work has no conflict of interest.
1. Introduction
Agrochemicals (insecticide, fungicide, herbicide, etc.) have brought tremendous benefits to mankind by increasing production and controlling the vector of man and animal diseases. At the same time, the use of these agrochemicals has affected the health of aquatic organisms as it has posed potential health hazards to the life of fishes (Osman et al., Citation2011). Agrochemicals are the major cause of concern for the aquatic environment because of their toxicity, persistence and tendency to accumulate in the organisms (Joseph et al., Citation2010). The impacts of these, on aquatic organisms, are due to their movement from various diffuse or point sources and are posing a great threat to aquatic fauna, especially to fishes which constitute one of the major sources of protein-rich food for mankind (Ray, Mohan, & Sinha, Citation2015). Agrochemicals in the form of synthetic compounds are commonly referred as EDCs (Kojima, Katsura, Takeuchi, Niiyama, & Kobayashi, Citation2004), which have hormone-like activity. Of the agrochemicals proved to have endocrine disrupting property, 46% are insecticides, 21% are herbicides and 31% are fungicides (Mnif et al., Citation2011). The endocrine effects of these agrochemicals are believed to be due to their ability to: mimic the effect of endogenous hormones, antagonize the effect of endogenous hormones, disrupt the synthesis and metabolism of endogenous hormones, as well as of hormone receptors (Blair et al., Citation2000; Raun Andersen, Vinggaard, Høj Rasmussen, Gjermandsen, & Cecilie Bonefeld-Jørgensen, Citation2002; Tabb & Blumberg, Citation2006). They may also bind to these receptors without activating them; these antagonistic accomplishment blocks the receptors and inhibits their action. Finally, they may also interfere with the synthesis, transport, metabolism and elimination of hormones, thereby decreasing the concentration of natural hormones (Cocco, Citation2002).
The intricate relationship between the brain (hypothalamus and pituitary) and endocrine action allows adaptability of an organism to the environment while maintaining homeostasis. In vertebrates, the hypothalamus represents a master regulator of homeostasis and is the critical nexus between the nervous and endocrine systems. The hypothalamus mediates responses to homeostatic imbalance mainly through regulation of the pituitary gland, which, in turn, produces hormones that are able to affect systemic change, for example within the interrenal gland. The central role of the hypothalamic–pituitary axis makes it particularly susceptible and sensitive to perturbation by a variety of environmental contaminants. The response to stress in fish is characterized by the stimulation of the hypothalamus, which results in the activation of the neuroendocrine system and a subsequent cascade of metabolic and physiological changes (Lowe & Davison, Citation2005; Martínez-Porchas, Martínez-Córdova, & Ramos-Enriquez, Citation2009). These changes enhance the tolerance of an organism to face an environmental variation or an adverse situation while maintaining a homeostasis status (Martínez-Porchas et al., Citation2009). Under conditions of stress, the body of the fish emits immediate responses recognized as primary and secondary responses. The primary response is the perception of an altered state by the central nervous system and the release of the stress hormones, cortisol and catecholamines, into the bloodstream by the endocrine system (Martínez-Porchas et al., Citation2009). Secondary responses occur as a consequence of the released stress hormones (Martínez-Porchas et al., Citation2009), causing changes in the blood and tissue chemistry, e.g. an increase of plasma glucose (Begg & Pankhurst, Citation2004; Martínez-Porchas et al., Citation2009). Cortisol is a hormone secreted from the internal gland and is used in many studies as the stress indicator (Flik, Klaren, Van den Burg, Metz, & Huising, Citation2006; Gagnon, Jumarie, & Hontela, Citation2006; Sepici-Dinçel et al., Citation2009). The effects of prolonged exposures to agrochemicals on cortisol production have been examined in both the laboratory and wild populations. Many studies have indicated hypothalamic–pituitary interrenal (HPI) axis exhaustion as a common phenomenon. Prolonged cortisol elevation eventually creates negative feedback on the HPI axis, downregulating receptors and causing atrophy of cells, e.g. pituitary corticotrophs (Basu, Kennedy, Hodson, & Iwama, Citation2001; Gravel, Campbell, & Hontela, Citation2005; Laflamme, Couillard, Campbell, & Hontela, Citation2000; Levesque, Dorval, Hontela, Van Der Kraak, & Campbell, Citation2003; Marentette, Tong, & Balshine, Citation2012). Hence, the present study is focused on the alterations in the cortisol hormonal profile and its receptor as an adaptive change of the freshwater teleost, Oreochromis mossambicus exposed to the sublethal concentration of four different agrochemicals (imidacloprid—IMI, pyrazosulfuron ethyl—PE, curzate—CZ and micronutrient mixture—MN). The rationale behind selecting these agrochemicals was their widespread used in the country and its residues found in nearby aquatic ecosystem in our study (unpublished data) and their unexplored effect on interrenal axis. Similarly, O.mossambicus was selected as a model due to its wide availability in aquatic ecosystem and its use as an edible fish.
2. Materials and method
2.1. Animal maintenance
Mature Tilapia O.mossambicus (12 ± 2 cm, 25 ± 1.9 g) of similar size in length and weight were obtained from the pure brooders of the Vadodara district and transferred to the laboratory. The acclimation period was for 15 days at 27 ± 40°C, pH 7.4 ± 0.5, dissolved oxygen 8 ± 0.3 mg/L, total hardness 188 mg/L CaCO3 with a 12:12 light:dark photoperiod. Fish were supplied daily with commercial fish food during acclimation. Animal maintenance and experimental procedures were in accordance with the guideline of APHA-AWWA-WEF (Citation1998).
2.2. Experimental protocol
On the basis of the LC50 value (Patel, Pandya, et al., 2016; Sadekarpawar, Pandya, Upadhyay, & Parikh, Citation2015; Upadhyay, Pandya, & Parikh, Citation2014) of each agrochemical sublethal study, dose LC50/10 (IMI-0.74 mg/L, PE-500 mg/L, CZ-49.61 mg/L, MN-5000 mg/L) was chosen (on the basis of their effective concentration found in pond ecosystem) to find its mechanistic action on HPI axis for which, hormonal assay and gene expression studies were conducted. The experimental regime was maintained in the laboratory for 14 days with a control group having three replicates in each group. The experiment was performed semi-statically with a group of 10 fishes (5 male and 5 females) in experimental aquaria. Hormonal assays of the experiment, as well as the control fish, were carried out at day 15 of exposure. All the groups were kept under continuous observation during the experimental period. After the completion of the exposure, fish were caught very gently using a small dip net, one at a time with least disturbance. They were slowly released in the tough containing 1% clove oil to make it immobile, blotted dry and blood was collected by tail ablation.
2.3. Hormonal assays
The blood was collected and serum was separated and kept in cold storage for hormonal assays. The hormonal titer of cortisol (Cyaman Cat # 500360) was performed. Each sample was assayed in triplicates where 100 µL of ELISA buffer was added to all the wells, followed by addition of 50 µL of cortisol standard to each well. Cortisol standard was made using serial dilution from the stock solution (400 ng/mL) in eight tubes. A total of 50 µL of the sample was added to each triplicate trailed by 50 µL AChE tracer in each well except for blank and total activity (TA). Finally, 50 µl of cortisol antiserum was added to each well except for the well of TA and nonspecific binding. The plate was incubated at room temperature on an orbital shaker for 1 h and was developed using Ellman’s reagent. The standard curve and sample concentration were determined using the following formula:
2.4. Total RNA isolation and PCR amplification
On day 15, fishes were removed and washed with fresh water. Control, as well as treated groups, was euthanized by decapitation, blood was allowed to drain, organs (hypothalamus, liver, gills, kidney, thyroid, ovary) were dissected out and gene expression pattern of the glucocorticoid receptor (GR) was analyzed. Testis was not taken into account, as upon dissection it was found to be ruptured in seven fishes of two groups (CZ and IMI). Total RNA was isolated by Trizol Invitrogen according to the method of Peterson and Freeman (Citation2009) and concentration was measured spectroscopically by Perkin Elmer. cDNA was reverse transcribed from 50 ng total isolated RNA using Thermo Verso cDNA synthesis kit (AB-1453/B) and PCR for GR was performed with its specific primers (FP-5ʹ-TTTCGGTAATTGGTTGCTGATGAT-3ʹ, RP-5ʹ-AGTGCTCCTGGCTGTTTCTAAGT-3ʹ) and with standardized condition, i.e. denaturation was performed at 95°C for 1 min, annealing was carried out for 30 sec at 59°C and extension was carried out at 72°C for 7 min with 18srRNA (FP:5′-TATTGTGCCGCTAGAGGTGAA3′, RP:5′-CCTCCGACTTTCGTTCTTGA-3′) as the reference gene. A total of 35 cycles were carried out for all the genes (described in materials and method) and finally, the amplicon obtained were checked on 2% agarose gel and images were taken using ABI gel documentation system. Relative quantification analysis of the PCR products was done using Image J software.
2.5. Statistical analysis
The difference between the mean of control and the exposed fishes was determined by one-way ANOVA using Graph Pad Prism software version 6. If there was any significant difference, post hoc test was carried out where Dunnett’s multiple comparison tests were employed to recognize difference in the alterations found in between the control and the exposed groups. The significant level of the tests was set at 5% (p < 0.05).
2.6. Pathway representation
The amplification of target gene resulted in multiple action site of the agrochemicals. Thus, bioinformatic tools were used for representation of the pathways and to investigate which pathways were being affected by the tested agrochemicals. Candidate gene network maps were generated using Pathway Common and WikiPathways and were visualized in the open-source software platform Cytoscape.
3. Results
3.1. Hormonal assay
Sublethal exposure of agrochemicals resulted in an overall decrease in cortisol concentration ranging from 2.2 ± 0.15 ng/mL to 5.9 ± 0.6 ng/mL in response to agrochemical exposure. At individual level, however, the significance (*p < 0.05) was found only with exposure to CZ (−61%), a fungicide and IMI (−58%), an insecticide in comparison to the control group, while there was no significance reported in case of PE and MN exposed fishes (Figure ). There were little alterations found in sex-specific cortisol titers of male and female; however, it was nonsignificant.
3.2. Gene expression studies
The candidate gene studied for the HPI axis was GR in vital tissues (liver, brain, kidney, gills, thyroid and ovary). Of all the agrochemicals, only IMI exposure resulted in a significant (*p < 0.05) downregulation of GR in all the tissues along with a significant (*p < 0.05) downregulation in testis and ovaries of fish exposed to CZ (Figures and ). There was an alteration in the expression in other tissues also, but it was nonsignificant on exposure of PE and MN. Data of the testes have not been presented, as in seven fishes it was found to be ruptured in CZ- and IMI-exposed group; thus, we postulated that the fishes had initiated the process of undergoing sex reversal (Pandya & Parikh, Citation2016).
3.3. Pathway analysis
To know the multiple actions of the chosen agrochemicals, the bioinformatic approach was used, where 32 genes (Figure ) were found to interacting with GR and were mostly the downstream signaling molecules. Further, the analysis also conveyed the fact that it had its association with the xenobiotic metabolism enzyme cyp 450 oxygenases and can be thought to be regulated by these canonical genes.
4. Discussion
The chemical coordination by endocrine system in organisms is known to regulate a number of hormone-dependent physiological functions which are essential for the survival of the organism both in normal and in an altered environmental condition. This endocrine system is a potential target of xenobiotics, and its vulnerability resides in part in the finely tuned mechanisms through which the endocrine control system operates in animals (Hontela, Citation1997). The xenobiotics that enter into the body can have either direct adverse effects on the endocrine gland and tissues or indirect through alterations of homeostasis and damaging activities of nonendocrine organs (Pandya & Parikh, 2016).
The HPI axis is responsible for releasing corticosteroids and catecholamines in response to a stressor. Cortisol is the major corticosteroid in teleost and most mammals (Miller, Citation2006). When a fish perceives a stimulus as a stressor, the hypothalamus releases corticotropin-releasing hormone (CRH) that stimulates the pituitary gland to release adrenocorticotropic hormone (ACTH), which in turn enters the bloodstream and stimulates the steroidogenic interrenal cells in the anterior head kidney to synthesize cortisol (Hontela, Citation2005). ACTH binds to its receptor in the cell membrane, and cyclic adenosine monophosphate activates protein kinase A. Protein kinase A activates cholesterol ester hydrolase releasing free cholesterol from cholesterol esters within the cellular matrix (Stocco, Citation2000). At the outer mitochondrial membrane, steroidogenic acute regulatory (StAR) protein regulates the passage of cholesterol to the inner membrane where cytochrome P450 side-chain cleavage (cP450scc) mediates the transformation of cholesterol to pregnenolone (Stocco, Citation2000). This is a rate-limiting step in cortisol synthesis and can be disrupted by contaminants (Walsh & Stocco, Citation2000). Pregnenolone moves to the cytoplasm where cytochromes P45017α and P450C21 create 11-deoxycortisol that is shunted back to the mitochondria to be transformed into cortisol by cytochrome P45011ß (Hontela, Citation2005). Cortisol regulates its own production through a negative feedback loop by altering ATCH secretion at the pituitary and hypothalamus (Hontela, Citation2006). Cortisol affects a variety of systems that regulate homeostasis (Dorval, Leblond, & Hontela, Citation2003). High serum cortisol levels indicate that the animal is under acute or subchronic stress, while low levels indicate no stress, interrenal exhaustion or impairment of the HPI axis (Martínez-Porchas et al., Citation2009; Miller & Hontela, Citation2011). Corticosteroids can also be influenced by factors other than stressors.
Some fish are more sensitive to stress during molting and may decrease their cortisol production as they age (Barton, Citation2002; Jasmin Christal, Sumathiral, & Ramaswamy, Citation2003). Several studies have corroborated the impairment in cortisol synthesis and secretion due to the action of agrochemicals (Aluru, Jorgensen, Maule, Vijayan, 2004; Aluru, Renaud, Leatherland, Vijayan, Citation2005; Aluru & Vijayan, 2006; Hontela, Citation2006; Gravel & Vijayan, Citation2006, Citation2007). The impairment is either at the level of CRH, ACTH or at the level of StAR protein and N6, 2ʹ-dibutyl adenosine 3ʹ: 5ʹ-cyclic monophosphate (dbc-AMP) (Leblond, Bisson, & Hontela, Citation2001) which is the common target of many environmental pollutants ranging from pesticides to pharmaceuticals (Hontela, Citation2006; Martínez-Porchas et al., Citation2009). Therefore, many pollutants halt cortisol secretion and even if the fish is under stress, this will probably not be reflected in the cortisol response. In the present study, cortisol level in O. mossambicus was found to be lower than that of control level on exposure to IMI and CZ, suggesting an impairment of the interrenal exhaustion or impairment of the HPI axis (Martínez-Porchas et al., Citation2009; Miller, Citation2006). Similar results were reported in Fundulus heteroclittus where there was a decreased level of serum cortisol under toxic conditions on exposure to naphthalene as a result of severe necrosis of interrenal tissues (Swarnalatha, Citation2015).
Gagnon and coworkers (Citation2007), while investigating the impact of toxicants on fish interrenal tissues, based on the in vitro studies, also reported inhibiting effects of pollutants on interrenal function and cortisol secretion. Oreochromis mossambicus, under sublethal thiodon exposure, registered significant reductions in serum cortisol levels following 1, 6 and 12 h of exposures (Parvatham, Sumathiral, & Ramaswamy, Citation2004). Similar reductions in serum cortisol level were also reported in O.mossambicus exposed to sublethal concentration of dimecron (Karthikeyan, Sumathiral, & Ramaswamy, Citation2004), in Channa striatus exposed to lethal and sublethal concentrations of sevin (Sumathiral, Citation2006), in Labeo rohita fingerlings exposed to endosulfan and dietary pyridoxine (Akhtar et al., Citation2010), in Clarias gariepinus exposed to varying concentrations of endosulfan (Ezemonye & Ikpesu, Citation2010) and in Sarotherodon mossambicus under endosulfan toxicity (Thangavel, Sumathiral, Maheswari, Rita, & Ramaswamy, Citation2010). Wedemeyer and Yasutake (Citation1977) stated that too low levels of cortisol are indicative of interrenal exhaustion from severe stress, whereas too high levels indicated the fish to be under chronic or acute stress. Similar reductions in cortisol level as a function of interrenal exhaustion were also reported in fish exposed to various pesticides (Karthikeyan et al., Citation2004; Parvatham et al., Citation2004; Sumathiral, Citation2006; Swarnalatha, Citation2015; Thangavel et al., Citation2010) and during in vitro exposure of head and kidney tissues of fish to pesticides (Leblond et al., Citation2001) and to heavy metals (Brodeur, Sherwood, Rasmussen, & Hontela, Citation1997). The reduced cortisol level in the present study, together with similar reports of interrenal exhaustion and reduction in cortisol level in other fish under different types of toxic conditions, could be considered as an adaptive response by the fish by way of maintaining low metabolic rate under pesticide.
Genome duplication event occurs in teleost fish (Jaillon et al., Citation2004), leading to two distinct GR genes (Alsop & Vijayan, Citation2008; Bury & Sturm, Citation2007; Bury et al., Citation2003; Greenwood et al., Citation2003; Stolte et al., Citation2008). In the present work, the focus was mainly on the GR, as it has high affinity to bind its steroid cortisol (Basu, Kennedy, & Iwama, Citation2003; Cruz, Lin, Chao, & Hwang, Citation2013). Indeed, several studies have shown that the xenobiotics disrupt cortisol and its receptor response to stress by targeting multiple sites along the HPI axis, including impaired steroidogenesis and brain glucocorticoid signaling (Aluru & Vijayan, Citation2006; Aluru et al., Citation2004; Hontela, Citation2005; Vijayan, Prunet, & Boon, Citation2005). In the present study, tissue-specific receptor expression was studied under the exposure of agrochemicals, among which significant damage was encountered by IMI, which downregulated the mRNA of GR in all the organs followed by CZ in the ovary and testis only. The exposure of IMI and CZ resulted in sensitization of cortisol receptor and maybe its steroid (cortisol), substantiating the receptor downregulation probably due to its self-regulation (Sathiyaa & Vijayan, Citation2003). Thus, the mechanism that may be operative is the increase in the cortisol, which binds to its receptor leading to activation of other growth-regulated transcriptional factors and thus maintaining the physiological stress caused by the agrochemicals (Gravel & Vijayan, Citation2006).
To analyze interactions between the specific and nonspecific genes involved at the transcriptional level, an attempt was made to interconnect with the help of Cytoscape software; Pathway Common and WikiPathways were used which helped in understanding the possible role of the association of genes with the target genes. GR signaling governs many metabolic pathways through more than 100 genes. The data of the present work were applied to Cytoscape and Pathway Common, which resulted in the interaction of various genes. A total of 32 genes were found to be interacting with the GR among which major transcription factors like jun, CREBP, Smad’s may be the downstream activators which initiate the physiological response upon pesticide exposure. Moreover, CYP2C8 (cytochrome-p450) may be activated due to the toxic exposure of IMI and CZ which may lead to degradation of this xenobiotics and maintaining the adaptive response (Figure ). Further, the androgen receptor (AR) was also found to be interacting with it, which suggests that it also aids in combating the agrochemical stress and maintains its reproductive potency in its proper state.
5. Conclusion
So from the present work, it can be concluded that agrochemicals exposure invoked alterations in the expression of genes associated with HPI axis. The observed reduction in the serum cortisol level in IMI and CZ group is an indication of interrenal exhaustion and also an adaptive stress response of the fish by way of maintaining low basal metabolic rate under agrochemical toxicity (Figure ). A strong link is thus determined between the measured upregulation/downregulation of GR and indicates the potential of using gene expression in toxicological studies as markers. However, immunohistochemistry, microarray and cloning of the upstream (ACTH, StA, 17-β hydroxylase) and downstream genes (signaling molecules) will provide insights into a better understanding of the mechanisms governing the effect of agrochemicals on the canonical pathways of this axis at each level.
Abbreviations
HPI | = | Hypothalamic–pituitary interrenal axis |
GR | = | Glucocorticoid receptor |
IMI | = | Imidacloprid |
CZ | = | Curzate |
MN | = | Micronutrient mixture |
PE | = | Pyzosulphuroethyl |
CRH | = | Cortocotropin releasing hormone |
ACTH | = | Adrenocorticotropic hormone |
C | = | Cortical region |
M | = | Medullary region |
AR | = | Androgen receptor |
Figure 5. Conclusion—schematic representation of hypothalamus–pituitary interrenal axis (HPI) under the exposure of agrochemicals (PE—pyzosulphuroethyl, IMI—imidacloprid, MN—micronutrient mixture and CZ—curzate). CRH—cortocotropin releasing hormone, ACTH—adrenocorticotropic hormone, GR—glucocorticoid receptor, C—cortical region, M—medullary region.
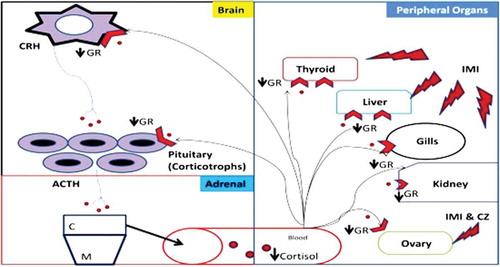
Compliance with ethical standards
The research carried out is compliance with ethical standards.
Ethical approval
All the procedures were in accordance with the guidelines of APHA-AWWA-WEF (Citation1998) for which the care and use of animals were followed.
Acknowledgments
The authors are thankful to the Head, Department of Zoology, Faculty of Science, The M.S. University of Baroda, Vadodara, for providing instrument facilities.
Additional information
Funding
Notes on contributors
Parth Pandya
Dr. Parth is immensely working the field of vertebrate endocrinology, where he has explored the HPG,HPT and HPI axis of Teleost. He has studied various markers by conducting gene and protein expression studies and has also looking into the unraveling the mechanisms behind genotoxic potential of agrochemicals in various animal models.
Ankur Upadhyay
Mr. Ankur is a researcher working on extrapolating the effect of various herbicides on fish species. He has conducted a range of studies from biochemical estimations to cell death, where in particular he gone in dept to unravel the mechanism of herbicide toxicity.
Bhumi Thakkar
Ms. Bhumi is working on different animal models, where she has proved the lethal effects of various toxic chemicals. She has investigated the cause and effect relationship of various pesticides in invertebrate and vertebrate model.

Pragna Parikh
Prof. Pragna Parikh works dynamically in the field of ecotoxicology from past 20 years and their research group has provided vital insights into the mechanism and toxicity of various groups of agrochemicals on nontarget organisms. The studies in their lab are ranging from basic biochemistry to endocrine disruption of agrochemicals, where they have shown a subchronic and subacute toxicological potential of various toxicants.
References
- Akhtar, S. M, Pal, A. K, Sahu, N. P, Alexander, C, Gupta, S. K, Choudhar, A. K, ... Rajan, M. G. (2010). Stress mitigating and immunomodulatory effect of dietary pyridoxine in Labeo rohita (Hamilton) fingerlings. Aquaculture Research, 41: 991–1002.
- Alsop, D., & Vijayan, M. M. (2008). Development of the corticosteroid stress axis and receptor expression in zebrafish. American Journal of Physiology, Regulatory, Integrative and Comparative Physiology, 294, 711–719. doi:10.1152/ajpregu.00671.2007
- Aluru, N., Jorgensen, E. H., Maule, A. G., & Vijayan, M. M. (2004). PCB disruption of the hypothalamus-pituitary-interrenal axis involves brain glucocorticoid receptor downregulation in anadromous arctic charr. American Journal of Physiology. Regulatory, Integrative and Comparative Physiology, 287, 787–793. doi:10.1152/ajpregu.00091.2004
- Aluru, N., Renaud, R., Leatherland, J. F., & Vijayan, M. M. (2005). An receptor-mediated impairment of interrenal steroidogenesis involves StAR protein and P450scc gene attenuation in rainbow trout. Toxicological Sciences, 84(2), 260–269. doi:10.1093/toxsci/kfi077
- Aluru, N., & Vijayan, M. M. (2006). Aryl hydrocarbon receptor activation impairs cortisol response to stress in rainbow trout by disrupting the rate-limiting steps in steroidogenesis. Endocrinology, 147, 1895–1903. doi:10.1210/en.2005-1143
- APHA-AWWA-WEF. (1998). Book on standard methods for the examination of water and wastewater (20th ed.). National Government Publication.
- Barton, B. A. (2002). Stress in fishes: A diversity of responses with particular reference to changes in circulating corticosteroids. Oxford Journals, 42(3), 517–525. doi:10.1093/icb/42.3.517
- Basu, N., Kennedy, C. J., Hodson, P. V., & Iwama, G. K. (2001). Altered stress responses in rainbow trout following a dietary administration of cortisol and beta-napthoflavone. Fish Physiology Biochemistry, 25, 131–140. doi:10.1023/A:1020566721026
- Basu, N., Kennedy, C. J., & Iwama, G. K. (2003). The effects of stress on the association between hsp70 and the glucocorticoid receptor in rainbow trout. Comparative Biochemistry and Physiology, 134A(3), 655–663. doi:10.1016/S1095-6433(02)00372-0
- Begg, K., & Pankhurst, N. W. (2004). Endocrine and metabolic responses to stress in a laboratory population of the tropical damselfish Acanthochromis polyacanthus. Journal of Fish Biology, 64, 133–145. doi:10.1111/j.1095-8649.2004.00290.x
- Blair, R. M., Fang, H., Branham, W. S., Hass, B. S., Dial, S. L., Moland, C. L., … Sheehan, D. M. (2000). The estrogen receptor relative binding affinities of 188 natural and xenochemicals: Structural diversity of ligands. Toxicological Sciences, 54, 138–153. doi:10.1093/toxsci/54.1.138
- Brodeur, J. C., Sherwood, G., Rasmussen, J. B., & Hontela, A. (1997). Impaired cortisol secretion in Yellow perch (Perca flavescenes) from lakes contaminated by heavy metals: In vivo and in vitro assessment. Canadian Journal of Fisheries and Aquatic Sciences, 54, 2752–2758. doi:10.1139/cjfas-54-12-2752
- Bury, N. R., & Sturm, A. (2007). Evolution of the corticosteroid receptor signalling pathway in fish. General and Comparative Endocrinology, 153, 47–56. doi:10.1016/j.ygcen.2007.03.009
- Bury, N. R., Sturm, A., Le Rouzic, P., Lethimonier, C., Ducouret, B., Guiguen, Y., … Prunet, P. (2003). Evidence for two distinct functional glucocorticoid receptors in teleost fish. Journal of Molecular Endocrinology, 31, 141–156. doi:10.1677/jme.0.0310141
- Cocco, P. (2002). On the rumors about the silent spring: Review of the scientific evidence linking occupational and environmental pesticide exposure to endocrine disruption health effects. Cadernos de Saúde. Pública, 18(2), 379–402. doi:10.1590/S0102-311X2002000200003
- Colborn, T. (2002). Clues from wildlife to create an assay for thyroid system disruption. Environmental Health Perspectives, 3, 363–367. doi:10.1289/ehp.02110s3363
- Cruz, S. A., Lin, C.-H., Chao, P.-L., & Hwang, -P.-P. (2013). Glucocorticoid receptor, but not mineralocorticoid receptor, mediates cortisol regulation of epidermal ionocyte development and ion transport in Zebrafish (Danio rerio). PLoS One, 8(10), e77997. doi:10.1371/journal.pone.0077997
- Dorval, J., Leblond, V. S., & Hontela, A. (2003). Oxidative stress and loss of cortisol secretion in adrenocortical cells of rainbow trout (Oncorhynchus mykiss) exposed in vitro to endosulfan, an organochlorine pesticide. Aquatic Toxicology, 63, 229–241. doi:10.1016/S0166-445X(02)00182-0
- Ezemonye, L. I. N., & Ikpesu, T. O. (2010). Evaluation of sublethal effects of endosulfan on cortisol secretion, glutathione s-transferase and acetycholinesterase activities in Clarias gariepinus. Food and Chemical Toxicology, 49(9), 1898–1903. doi:10.1016/j.fct.2010.10.025
- Flik, G., Klaren, P. H. M., Van den Burg, E. H., Metz, J. R., & Huising, M. O. (2006). CRF and stress in fish. General and Comparative Endocrinology, 146, 36–44. doi:10.1016/j.ygcen.2005.11.005
- Gagnon, A., Jumarie, C., & Hontela, A. (2006). Effects of Cu on plasma cortisol and cortisol secretion by adrenocortical cells of rainbow trout (Oncorhynchus mykiss). Aquatic Toxicology, 78(1), 59–65. doi:10.1016/j.aquatox.2006.02.004
- Gagnon, E., Hontela, A., & Jumarie, C. (2007). Reciprocal inhibition of Cd and Ca uptake in isolated head kidney cells of rainbow trout (Oncorhynchus mykiss). Toxicology in Vitro, 21(6), 1077–1086. doi:10.1016/j.tiv.2007.03.019
- Gravel, A., Campbell, P. G. C., & Hontela, A. (2005). Disruption of the hypothalamo-pituitaryinterrenal axis in 1+ yellow perch (Perca flavescens) chronically exposed to metals in the environment. Canadian Journal of Fisheries and Aquatic Sciences, 62, 982–990. doi:10.1139/f05-011
- Gravel, A., & Vijayan, M. M. (2006). Salicylate disrupts interrenal steroidogenesis and brain glucocorticoid receptor expression in rainbow trout. Toxicological Sciences, 93(1), 41–49. doi:10.1093/toxsci/kfj166
- Gravel, A., & Vijayan, M. M. (2007). Salicylate impacts the physiological responses to an acute handling disturbance in rainbow trout. Aquatic Toxicology, 85(2), 87–95. doi:10.1016/j.aquatox.2007.07.001
- Greenwood, A. K., Butler, P. C., White, R. B., DeMarco, U., Pearce, D., & Fernald, R. D. (2003). Multiple corticosteroid receptors in a teleost fish: Distinct sequences, expression patterns, and transcriptional activities. Endocrinology, 144, 4226–4236. doi:10.1210/en.2003-0566
- Hontela, A. (1997). Endocrine and physiological responses of fish to xenobiotics: Role of glucocorticosteroid hormones. Critical Reviews in Toxicology, 1, 1–46. doi:10.1006/taap.1999.8660
- Hontela, A. (2005). Stress and the hypothalamo-pituitary-interrenal axis: Adrenal toxicology—Effects of environmental pollutants on the structure and function of the HPI axis. Environmental Toxicology, 331–363. doi:10.1016/S1873-0140(05)80015-3
- Hontela, A. (2006). Corticosteroidogenesis and StAR protein of rainbow trout disrupted by human-use pharmaceuticals: Data for use in risk assessment. Toxicological Sciences, 93(1), 1–2. doi:10.1093/toxsci/kfl054
- Jaillon, O., Aury, J.-M., Brunet, F., Petit, J.-L., Thomann, N. S., Mauceli, E., … Roest Crollius, H. (2004). Genome duplication in the teleost fish Tetraodon nigroviridis reveals the early vertebrate proto-karyotype. Nature, 431, 946–957. doi:10.1038/nature03025
- Jasmin Christal, C., Sumathiral, K., & Ramaswamy, M. (2003). Changes in serum cortisol and electrolyte levels in a freshwater teleost, Sarotherodon mossambicus (Peters) under individual and synergistic exposures to dimecron and cuman L. In National conference on zoology (NACOZ—2003), held on 21st to 23rd December 2003 at Vivekananda College, Agastheeswaram, Kanyakumari, Tamil Nadu, India.
- Joseph, B., Raj, S. J., Edwin, B. T., Sankarganesh, P., Jeevitha, M. V., Ajisha, S. U., & Sheeja, S. R. (2010). Toxic effect of heavy metals on aquatic environment. International Journal of Biological and Chemical Sciences, 4, 939–952. doi:10.4314/ijbcs.v4i4.62976
- Karthikeyan, S., Sumathiral, K., & Ramaswamy, M. (2004). Dimecron-induced endocrine responses in a freshwater, edible, teleost fish, Oreochromis mossambicus (Peters). In National symposium on recent trends in applied biology, held on 28th & 29th January, 2004 at Avinasilingam Institute for Home Science and Higher Education for Women Deemed University, Coimbatore, Tamil Nadu, India.
- Kojima, H., Katsura, E., Takeuchi, S., Niiyama, K., & Kobayashi, K. (2004). Screening for estrogen and androgen receptor activities in 200 pesticides by in vitro reporter gene assays using Chinese hamster ovary cells. Environmental Health Perspective, 112, 524–531. doi:10.1289/ehp.6649
- Laflamme, J.-S., Couillard, Y., Campbell, P. G. C., & Hontela, A. (2000). Interrenal metallothionein and cortisol secretion in relation to Cd, Cu and Zn exposure in yellow perch, Perca flavescens, from Abitibi lakes. Canadian Journal of Fisheries and Aquatic Sciences, 57, 1692–1700. doi:10.1139/f00-118
- Leblond, V. S., Bisson, M., & Hontela, A. (2001). Inhibition of cortisol secretion in dispersed head kidney cells of rainbow trout (Oncorhynchus mykiss) by endosulfan, an organochlorine pesticide. General and Comparative Endocrinology, 121, 48–56. doi:10.1006/gcen.2000.7556
- Levesque, H. M., Dorval, J., Hontela, A., Van Der Kraak, G. J., & Campbell, P. G. C. (2003). Hormonal, morphological and physiological responses of yellow perch (Perca flavescens) to chronic environmental metal exposures. Journal of Toxicology and Environmental Health, 66, 657–676. doi:10.1080/15287390309353773
- Lowe, C. J., & Davison, W. (2005). Plasma osmolarity, glucose concentration and erythrocyte responses of two. Antarctic nototheniid fishes to acute and chronic thermal change. Journal of Fish Biology, 67, 752–766. doi:10.1111/j.0022-1112.2005.00775.x
- Marentette, J. R., Tong, S., & Balshine, S. (2012). The cortisol stress response in male round goby (Neogobius melanostomus): Effects of living in polluted environments. Environmental Biology of Fishes, 96(6), 723–733. doi:10.1007/s10641-012-0064-8
- Martínez-Porchas, M., Martínez-Córdova, L. R., & Ramos-Enriquez, R. (2009). Cortisol and glucose: Reliable indicators of fish stress. Pan-American Journal of Aquatic Sciences, 4, 158–178.
- Miller, L. (2006). The effects of selenium on the physiological stress response in fish. Thesis submitted to Department of Biological Sciences, University of Lethbridge, Lethbridge, AB.
- Miller, L. L., & Hontela, A. (2011). Species-specific sensitivity to selenium-induced impairment of cortisol secretion in adrenocortical cells of rainbow trout (Oncorhynchus mykiss) and brook trout (Salvelinus fontinalis). Toxicology and Applied Pharmacology, 253, 137–144. doi:10.1016/j.taap.2011.03.021
- Mnif, W., Hassine, A. I. H., Bouaziz, A., Bartegi, A., Thomas, O., & Roig, B. (2011). Effect of endocrine disruptor pesticides: A review. International Journal of Environmental Research and Public Health, 8(6), 2265–2303. doi:10.3390/ijerph8062265
- Osman, A. G., Abd El Reheema, A. M., Moustafa, M. A., Mahmoud, U. M., Abuel-Fadld, K. Y., & Kloas, W. (2011). In situ evaluation of the genotoxic potential of the river nile: I. Micronucleus and nuclear lesion tests of erythrocytes of Oreochromis niloticus (Linnaeus, 1758) and Clarias gariepinus (Burchell, 1822). Toxicological and Environmental Chemistry, 93, 1002–1017. doi:10.1080/02772248.2011.564496
- Pandya, P., & Parikh, P. (2016). Agrochemicals induced gene expression alterations in O. mossambicus. International Journal of Advanced Research, 4(6), 830–840. doi:10.21474/IJAR01
- Parvatham, M., Sumathiral, K., & Ramaswamy, M. (2004). Adaptive endocrine responses in a freshwater teleost, Oreochromis mossambicus (Peters) exposed to organophosphate pesticide, endosulfan. In National symposium on recent trends in applied biology held on 28th and 29th January, 2004 at Department of Life Sciences, Avinashilingam Institute for Home Science and Higher Education for Women, Deemed University, Coimbatore, Tamil Nadu, India.
- Patel, B., Pandya, P., & Parikh, P. (2016). Effects of agro-chemicals on antioxidant enzymes and lipid peroxidation in Oreochromis mossambicus and Labeo rohita. International Journal of Zoology and Applied Biosciences, 1(3), 163–172.
- Patel, B., Upadhyay, A., & Parikh, P. H. (2016). Histological changes in the tissues of Oreochromis mossambicus and Labeo rohita on exposure to imidacloprid and curzate. International Journal of Research in Applied Natural and Social Sciences, 4(5), 149–160.
- Peterson, S. M., & Freeman, J. L. (2009). RNA isolation from embryonic zebrafish and cDNA synthesis for gene expression analysis. Journal of Visualized Experiments, 30, 1470. doi:10.3791/1470
- Raun Andersen, H., Vinggaard, A. M., Høj Rasmussen, T., Gjermandsen, I. M., & Cecilie Bonefeld-Jørgensen, E. (2002). Effects of currently used pesticides in assays for estrogenicity, androgenicity, and aromatase activity in vitro. Toxicology and Applied Pharmacology, 179, 1–12. doi:10.1006/taap.2001.9347
- Ray, S. N. C., Mohan, C., & Sinha, R. C. (2015). Effect of methyl parathion on TSH, T4 and T3 in fish, Labeo rohita. International Journal of Applied Research, 5(3), 554–557.
- Sadekarpawar, S., Pandya, P., Upadhyay, A., & Parikh, P. (2015). A comparative assessment of trace metal accumulation in Oreochromis mossambicus and labeo rohita exposed to plant nutrient librel TM. International Journal of Current Advanced Research, 4(10), 441–449.
- Sathiyaa, R., & Vijayan, M. M. (2003). Autoregulation of glucocorticoid receptor by cortisol in rainbow trout hepatocytes. American Journal of Physiology-Cell Physiology, 284, 1508–1515. doi:10.1152/ajpcell.00448.2002
- Sepici-Dinçel, A., Çağlan Karasu Benli, A., Selvi, M., Sarıkaya, R., Şahin, D., Ayhanözkul, I., & Erkoç, F. (2009). Sublethal cyfluthrin toxicity to carp (Cyprinus carpio L.) fingerlings: Biochemical, hematological, histopathological alterations. Ecotoxicology and Environmental Safety, 72, 1433–1439. doi:10.1016/j.ecoenv.2009.01.008
- Stocco, D. M. (2000). The role of the StAR protein in steroidogenesis: Challenges for the future. Journal of Endocrinology, 164, 247–253. doi:10.1677/joe.0.1640247
- Stolte, E. H., de Mazon, A. F., Leon-Koosterziel, K. M., Jesiak, M., Bury, N. R., Sturm, A., … Flik, G. (2008). Corticosteroid receptors involved in stress regulation in common carp, Cyprinus carpio. Journal of Endocrinology, 198, 403–417. doi:10.1677/JOE-08-0100
- Sumathiral, K. (2006). Endocrine and immunological responsiveness in a freshwater, edible, airbreathing teleost, Channa striatus (Bloch) exposed to a carbamate pesticide, sevin ( Ph.D., thesis). Bharathiar University, Coimbatore.
- Swarnalatha, M. (2015). A comprehensive study on hormone response ionic regulation selected enzyme levels and immuneresponsiveness in a freshwater air breathing teleost mystus vittatus bloch exposed to an organophosphate pesticide quinalphos. A thesis submitted to Department of Zoology, Bharathiar University, India.
- Tabb, M. M., & Blumberg, B. (2006). New modes of action for endocrine-disrupting chemicals. Molecular Endocrinology, 20, 475–482. doi:10.1210/me.2004-0513
- Thangavel, P., Sumathiral, K., Maheswari, S., Rita, S., & Ramaswamy, M. (2010). Hormone profile of an edible, freshwater teleost, Sarotherodon mossambicus (Peters) under endosulfan toxicity. Pesticide Biochemistry and Physiology, 97, 229–234. doi:10.1016/j.pestbp.2010.03.001
- Upadhyay, A., Pandya, P., & Parikh, P. (2014). Acute exposure of pyrazosulfuron ethyl induced haematological and blood biochemical changes in the freshwater teleost fish Oreochromis mossambicus. International Journal of Advanced Research in Biological Sciences, 1(2), 79–86.
- Vijayan, M. M., Prunet, P., & Boon, A. N. (2005). Xenobiotic impact on corticosteroid signalling. In: Mommsen TP, Moon TW (eds) Environmental toxicology. Biochemistry and Molecular Biology of Fishes, 6, 365–396.
- Walsh, L. P., & Stocco, D. M. (2000). Effects of lindane on steroidogenesis and steroidogenic acute regulatory protein expression. Biology of Reproduction, 63, 1024–1033. doi:10.1095/biolreprod63.4.1024
- Wedemeyer, G. A., & Yasutake, W. T. (1977). Clinical methods for assessment of the effects of environmental stress on fish health (Vol. 9, pp. 1–19). Washington, DC: U. S. Fish and Wild Life Service.