Abstract
The ability to perceive thermal changes in the surrounding environment is critical for survival. However, sensing temperature is not the only factor among the cutaneous sensations to contribute to thermoregulatory responses in humans. Sensing skin wetness (i.e. hygrosensation) is also critical both for behavioral and autonomic adaptations. Although much has been done to define the biophysical role of skin wetness in contributing to thermal homeostasis, little is known on the neurophysiological mechanisms underpinning the ability to sense skin wetness. Humans are not provided with skin humidity receptors (i.e., hygroreceptors) and psychophysical studies have identified potential sensory cues (i.e. thermal and mechanosensory) which could contribute to sensing wetness. Recently, a neurophysiological model of human wetness sensitivity has been developed. In helping clarifying the peripheral and central neural mechanisms involved in sensing skin wetness, this model has provided evidence for the existence of a specific human hygrosensation strategy, which is underpinned by perceptual learning via sensory experience. Remarkably, this strategy seems to be shared by other hygroreceptor-lacking animals. However, questions remain on whether these sensory mechanisms are underpinned by specific neuromolecular pathways in humans. Although the first study on human wetness perception dates back to more than 100 years, it is surprising that the neurophysiological bases of such an important sensory feature have only recently started to be unveiled. Hence, to provide an overview of the current knowledge on human hygrosensation, along with potential directions for future research, this review will examine the psychophysical and neurophysiological bases of human skin wetness perception.
Abbreviations
TRP | = | Transient Receptor Potential channels |
DEG/ENaC | = | Degenerin/Epithelial sodium channels |
KCNK | = | Two-pore potassium channels |
Introduction
Since the seminal work of Pharo Gagge at the John B. Pierce Foundation Laboratory,Citation1 the measurement of skin wetness as a biophysical variable has received great attention within the context of thermal physiology, particularly for its role in the estimation of the body's heat balance under conditions of increased metabolic heat production (e.g. resulting from exercising muscles), and decreased gradient for heat loss to the environment (e.g., resulting from high ambient temperatures).Citation2-5 However, although much has been done to define the biophysical role of skin wetness in contributing to thermal homeostasis, surprisingly little is known on the neurophysiological mechanisms underpinning humans’ ability to sense skin wetness, and on how the level of “physical” wetness relates to the level of “perceived” skin wetness.
In contrast with insects, in which humidity receptors (i.e., hygroreceptors) sub-serving humidity detection (i.e. hygrosensation) have been identified and widely described,Citation6 humans’ largest sensory organ, i.e., the skin, seems not to be provided with specific receptors for the sensation of humidity and skin wetness.Citation7 It has been proposed that, as human beings, we seem to “learn” to perceive the wetness experienced when the skin is in contact with a wet surface or when sweat is producedCitation8 through a complex multisensory integrationCitation9 of thermal (i.e. heat transfer) and mechanosensory (i.e., mechanical pressure and skin friction) inputs generated by the interaction between skin, moisture and (if donned) clothing.Citation10 However, what remains unclear is how thermal and mechanosensory (i.e. tactile) cues are integrated peripherally as well as centrally by our nervous system to give rise to the perception of skin wetness. In this respect, in a recent study performed by our group,Citation11 we have shown that humans could sense and process the perception of skin wetness in a rationale fashion, according to a specific sensory information processing model, which is based on the multimodal integration of thermal and mechanical inputs occurring at the skin’ surface when wet.
In helping develop a neurophysiological model for skin wetness perception, these recent findings have provided novel behavioral and neurophysiological evidence which could explain how humans sense wetness on their skin.Citation11 However, they have also opened up questions about the potential neuromolecular mechanisms underpinning humans’ hygrosensation. Although the first study on wetness perception to be reported dates back to more than 100 years,Citation12 it is surprising that the neurophysiological bases of such an important human sensory feature have only recently started to be unveiled. Hence, in order to provide an overview of the current knowledge on human hygrosensation, along with potential directions for future research, this review will critically examine the psychophysical and neurophysiological bases of humans’ ability to perceive skin wetness.
Increasing the knowledge on how humans perceive skin wetness has both a fundamental, as well as an applied significance. On the fundamental side, this could contribute to a better understanding of how the peripheral and central nervous systems interact to generate complex somatic perceptions.Citation13 On the applied side, this knowledge could be useful for its potential clinical relevance (i.e., development of diagnostic tests for patients with somatosensory disorders, e.g. Multiple Sclerosis; replication of this unique sensory characteristic in artificial skin and neuro-prosthetics)Citation14,15 as well as for its non-clinical (e.g., industrial) implications (e.g., development of new strategies in clothing design aiming to improve thermal and clothing comfort).Citation10
Skin Wetness as a Biophysical Variable
Before examining skin wetness as a perceptual variable, a brief outline of the characteristics of skin wetness as a biophysical variable will be presented. For a more detailed overview of the biophysical role of skin wetness within the context of the body's thermal balance the reader is referred to refs.Citation1,16
As a biophysical variable, skin wetness was first introduced by GaggeCitation1 who recognized its critical role in the heat balance of the body. Whether due to increases in metabolic heat production (e.g., as a result of exercise) or exposure to hot environments, core overheating is prevented, and heat balance maintained, by means of sweating.Citation2 Evaporative heat loss trough sweating plays a critical role in cooling the skin, thus maintaining a favorable core to skin gradient for heat transport to the skin and subsequent losses from the body to the environment.Citation17 Therefore, within environmental conditions that allow full evaporation, the level of skin wetness represents an important parameter to ensure the evaporative efficiency of sweating.Citation2 As such, skin wetness is defined as the fraction of the body covered by liquid at skin temperature (e.g., sweat), and it represents a biophysical measure of the degree of wetness involved in the process of evaporation.Citation1 Skin wetness is usually expressed as a decimal fraction, with 1 representing the upper limit for a fully wet skin and 0.06 representing the minimal value due to insensible perspiration through the skin.Citation18
As well as for its biophysical role in the body's heat balance, Gagge identified the importance of skin wetness in the context of thermal comfort. The level of sweat-induced skin wetness had indeed been shown to positively correlate with thermal discomfort (i.e. the greater the skin wetness the greater the thermal discomfort), particularly during exposure to hot environments.Citation19
Since Gagge’ seminal work, the measurement of skin wetness has therefore received great attention, particularly in the context of predicting the body's heat balance during conditions of increased metabolic heat production and decreased gradient for heat loss to the environment (e.g., resulting from high ambient temperatures),Citation2-5 as well as in the context of predicting thermalCitation20 and clothing comfort.Citation10,Citation21-23 However, although much is known on the biophysical role of skin wetness in contributing to thermal homeostasis, our knowledge on the sensory integration behind humans’ ability to sense wetness on their skin is still limited.
Skin Wetness as a Perceptual Variable
The ability to sense the thermal properties (e.g., temperature and humidity) of the surrounding environment represents one the most important attributes that any animal species, including humans, requires to ensure survival.Citation24 For this reason, species such as humans are provided with specific sensory systems (e.g., among which the somatosensory one) which, by transducing distinctive physical properties of external stimuli (e.g., temperature and mechanical pressure) into biological signals (e.g., action potential generated at afferent nerve endings), provide the living organism with awareness of the characteristics of its surrounding thermal environment.Citation25 Ultimately, the ability to encode sensory information (e.g., thermal and tactile) is used by humans to initiate or adjust specific thermal behaviors (e.g., from adding or removing clothing to migrating to more thermally comfortable environments).Citation26 These behaviors are aimed at maintaining homeostasis, thermal comfort and ensuring survival.Citation27
Despite the critical role of thermosensitivity (i.e., the ability to perceive thermal changes in the surrounding environment),Citation28 sensing temperature is not the only factor among the cutaneous sensations to contribute to autonomic and behavioral thermoregulatory responses in humans. Sensing cutaneous wetness is also critical both for behavioral and autonomic adaptations. Perceiving changes in both ambient humidity and skin wetness have been shown to impact thermal comfortCitation10 and thus the thermoregulatory behavior,Citation26 both in healthy and clinical populations (e.g., individuals suffering from rheumatic pain).Citation29 From an autonomic perspective, the degree of skin wetness influences sweat gland function through a progressive suppression of the sweat output (i.e. hidromeiosis) in the presence of wet skin.Citation3 This reduction in sweat output occurs when the skin is fully saturated with sweat and the evaporative efficiency of sweating is limited by fact that any additional sweat starts to drip off the skin (instead of evaporating from it).Citation30 This reduction in sweat output in the presence of wet skin could contribute to limit the rate of dehydration in conditions of reduced evaporative efficiency of sweating. Apart for its role in thermoregulatory adaptations, sensing wetness is also important for other regulatory and behavioral mechanisms. For instance, decreases in ocular wetness seem to initiate the lacrimation reflex in order to maintain a tear film to protect the ocular surface.Citation31 Also, tactile roughness and wetness discrimination is critical for precision gripCitation32 and object manipulationCitation33 ().
Figure 1. Schematic representation of human behavioral and autonomic adaptations underpinned by the ability to sense humidity and skin wetness. With regards to behavioral responses, variations in ambient humidity and fingertip moisture influence the degree of skin and fingertip wetness; by sensing these changes, humans consciously adapt their thermal behavior and precision grip. With regards to autonomic responses, variations in sweat production and amount of teardrops influence the degree of skin and ocular wetness; by sensing these changes, the body adjust sweat glands’ output and lacrimation via reflex mechanisms.
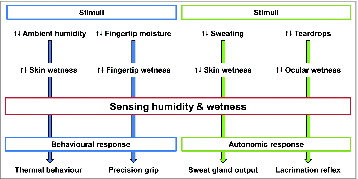
Although the ability to sense skin wetness plays an important role in several behavioral and physiological functions, the knowledge on this human sensory feature has remained scattered over the past century. Furthermore, as until recently the majority of the experimental studies investigating the perceptual bases of skin wetness have almost exclusively endorsed psychophysical procedures (which allow investigation of the relationship between physical stimuli and the sensations and perceptions evoked),Citation34 with little or no implementation of neurophysiological methods (which allow investigation of the relationship between stimuli and activity of the nervous system),Citation25 assumptions and hypotheses on the neural bases of such a complex sensory experience have remained speculative. Nevertheless, based on psychophysical evidence, hypotheses on the potential sensory mechanisms which underpin human skin wetness perception have been proposed. These have supported the recent development of neurophysiological approaches, which, by drawing upon psychophysical evidence, have started to unveil the potential neural bases of human skin wetness perception. Hence, in order to provide the reader with an overview of these different approaches and related finings, the following paragraphs will present the current evidence emerging form psychophysical investigations, followed by recent findings from neurophysiological studies.
Psychophysical Bases of Skin Wetness Perception
Investigating the psychophysical bases of human skin wetness perception represents a challenge which has attracted the interest of many scientists since the early days of the 20th century. To our knowledge, the first scientist who has attempted to explain the basis of this perception was Bentley,Citation12 who in 1900, with his famous “synthetic experiment,” tested the perception of dipping a sheath-covered finger into warm, lukewarm and cold water in blindfolded participants. The results indicated that, despite no actual contact with moisture occurred, the participants experienced a clear perception of wetness, which was more pronounced when the water was cold than when it was warm. When informed about the characteristics of the experiment (i.e., no direct contact with water), at first participants refused to believe that the finger was not actually wet.Citation12 Based on these early observations, Bentley proposed a sensory-blending hypothesis which suggests the blend of light pressure/touch and coldness as responsible for evoking the perception of wetness ().
Figure 2. (A) Overview of Bentley's hypothesisCitation12 on wetness perception (A). In his “synthetic experiment,” BentleyCitation12 tested the perception of dipping a sheath-covered finger into a liquid; the results showed that the participants at first refused to believe that the finger was not actually wet. Based on these early observations, Bentley proposed a sensory-blending hypothesis which suggests the blend of light pressure/touch and coldness as responsible for evoking the perception of wetness.Citation12 (B) Biophysical processes (i.e. variation in skin temperature and tactile interactions, with relative activations of skin thermo- and mechanoreceptors) involved in sensing skin wetness. Psychophysical evidence indicates that, in the absence of skin hygroreceptors, humans tend to associate the cold sensations evoked by the drop in skin temperature occurring during the evaporation of moisture from the skin, as a signal of the presence of moisture, and thus wetness, on the skin surface. Also, tactile inputs in the form of light touch and pressure could represent an important source of sensory information for sensing and discriminating skin wetness.
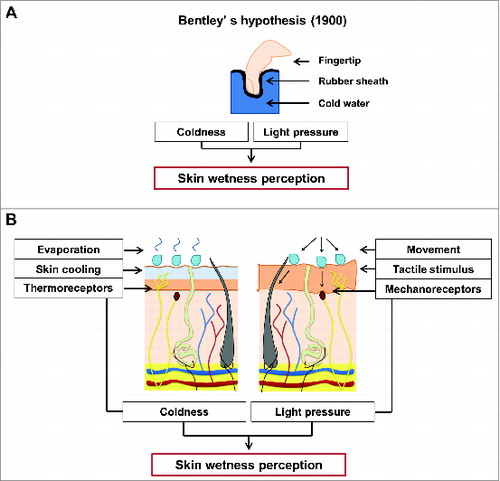
In contrast with insects, in which humidity receptors sub-serving hygrosensation have been identified and widely described,Citation6,35 human skin seems indeed not to be provided with specific humidity receptors.Citation7 Therefore, as firstly observed by Bentley, our perception of skin wetness could rely on the interaction of other somatosensory sub-modalities.Citation36 Bentley identified the role of both touch and temperature sense as determinant in characterizing this particular somatosensory experience.
Following this early work on the psychophysical bases of skin wetness perception, a number of studies have investigated the perception of skin wetness.Citation8,10,22,23,Citation37-43 By investigating the perceptual responses to either skin's contact with dry and wet stimuli,Citation8,22,23,Citation37-41 or to the active production of sweat,Citation10,42,43 these studies have provided psychophysical insights about the potential mechanisms for which skin wetness is sensed in humans. However, most of these works have tackled the investigation of skin wetness perception with an observational rather than a mechanistic approach. The lack of a mechanistic approach to the problem of skin wetness has therefore resulted in the same studies providing relatively limited conclusive evidence on which sensory sub-modality (between mechanical and temperature sense) plays the primary input in driving the perception of skin wetness, to what extent these sub-modalities interact, and how their sensory integration relates to the potentially secondary sensory inputs (e.g., vision) which overall contribute to characterize wetness as a synthetic perception (i.e. a perception that is not directly linked to the activity of one specific sensory organ, but that results from multimodal sensory integration).Citation37
The following paragraphs review the main findings of the above mentioned studies, with respect to investigating skin wetness perception as a result of the contact with an external (dry or wet) stimulus as well as during the production of sweat. Furthermore, an additional paragraph reviews the current knowledge on regional differences in skin wetness perception across the body.
Psychophysical Approach: Contact with External (dry or wet) Stimuli
Most of the literature investigating skin wetness perception as a result of the contact with an external (dry or wet) stimulus has focused on investigating the minimum amounts of wetness that individuals are able to discriminate between (i.e., discrimination of skin wetness) and whether individuals are able to characterize the level of skin wetness they experience during skin-wet stimuli contacts (i.e. magnitude estimation of skin wetness). The majority of these studies have endorsed the use of Quantitative Sensory Testing (i.e., a non-invasive sensory examination of somatosensory modalities such as light touch, vibration, thermal and pain sensation),Citation44 as the preferred methodology to assess human skin wetness perception.
In the light of this, the following paragraphs present an overview of the most representative studies which have endorsed the use of Quantitative Sensory Testing with discrimination or magnitude estimation paradigms, to investigate skin wetness perception as a result of the contact with an external (wet or a dry) stimulus.
Discrimination studies
The studies that have investigated skin wetness perception as a result of the contact with an external (dry or wet) stimulus using Quantitative Sensory Testing with a discrimination paradigm, have indicated that individuals seem to readily and accurately discriminate between higher and lower wetness levels with remarkable sensitivity.Citation45
During a discrimination experiment, Sweeney & BransonCitation22 showed that, when cotton test fabrics (25 cm2) with different water content were applied to the upper back of 13 blindfolded female participants, these discriminated between moisture content with a discrimination threshold of 1.6 μl.cm−2 against a reference stimulus of 3.6 μl.cm−2.
In line with this approach, Jeon et al.Citation46 applied 4 100 cm2 specimens of different types of fabric (i.e. cotton, regular polyester and 2 types of so-called high-performance polyester) with a range of moisture contents (1 to 21 μl.cm−2) to the right and left inner forearm of 10 blindfolded female participants (duration: 5 s). Test fabrics were applied simultaneously with one of 2 reference fabrics (with amounts of water of 5 and 15 μl.cm−2) and participants judged which stimulus caused greater wetness perception. This study found average discrimination thresholds which differed between the different materials (higher for e.g. high-performance polyester) in range of 1.9 to 2.6 μl.cm−2 against the 5 μl.cm−2 reference stimulus, and from 3.6 to 5.4 μl against the 15 μl.cm−2 reference stimulus.
Similarly, in a study in which 6 males and 6 females (blindfolded) interacted with 3 different types of wet materials (i.e. 19.6 cm2 thin and thick viscose and cotton wool), in 2 ways of exploring (i.e. the samples were either touched statically, flat on the table, in which case only thermal cues were available; or they were touched dynamically, picked up and manipulated, in which case both thermal and mechanical cues were available), Bergmann Tiest et al.Citation8 found that discrimination thresholds ranged from ∼25 to ∼400 μl.cm−2 according to the type of contact with the stimuli (dynamic vs. static).
In summary, the above mentioned studies provided evidence for the fact that individuals seem to readily discriminate between levels of wetness which differ as little as of 0.04 ml (note: to give the reader an idea of how sensitive such discriminatory performance is, one could suggest that an individual would be able to easily distinguish a 5 by 5 cm cotton handkerchief on which a very small drop of water has been applied from a similar, fully dry, cotton handkerchief; see e.g., ref.Citation21). Furthermore, these studies indicated that the noticeable difference in wetness levels seems to increase (e.g., from 0.04 to 0.54 ml; see refs.Citation21,49) with increasing magnitude of wetness (i.e., the wetter the stimuli, the greater the difference in moisture content required to perceive differences in the wetness level of the stimuli). However, although these studies endorsed the use of Quantitative Sensory Testing, by approaching the assessment of skin wetness perception with a discrimination paradigm (i.e. a forced choice between 2 wetness options, i.e., wetter or less wet), these have provided limited evidence on the potential sensory mechanisms involved in the subjects’ ability to sense and discriminate skin wetness. In this respect, the studies that have approached the assessment of skin wetness with a magnitude estimation paradigm have provided more detailed insights on the potential sensory inputs underlying human's ability to sense wetness on the skin. This, as a result of a more accurate determination of the psychophysical relationship between (1) the properties of the stimuli used; (2) the changes in skin temperature and/or mechanical properties produced by the simulation; (3) the resulting thermal and wetness sensations.
Magnitude estimation studies: thermal sense in the perception of skin wetness
The studies that have investigated what sensory inputs (e.g., thermal and tactile) contribute to skin wetness perception as a result of the contact with an external (dry or wet) stimulus using Quantitative Sensory Testing with a magnitude estimation paradigm, have indicated that the thermal sense (and specifically cold sensations) could be the key player in driving the perception of skin wetness.Citation39-41 In support of this hypothesis, it has been proposed that, as we learn to perceive skin wetness, we tend to associate the cold sensations evoked by the drop in skin temperature occurring during the evaporation of moisture from the skin, as a signal of the presence of moisture, and thus wetness, on the skin surfaceCitation39 ().
The critical role of cold sensations in influencing the perception of wetness has been observed by Yamakawa & IsajiCitation47 during a magnitude estimation experiment performed with 6 different textiles in 3 wetness conditions and at 3 different temperatures. In this study the authors found that subjects’ ratings in terms of perceived wetness correlated to the initial cooling rates occurring during the contact between the subjects’ fingers and the test fabrics: a greater initial temperature drop was linked to a greater sensation of clamminess.
The key role of experiencing coldness in the ability to sense skin wetness has also been proposed by Daanen,Citation39 who measured the temperature course of the skin (i.e. temperature’ s drop of 1 to 5°C with a 0.05 to 0.2°C.s−1 cooling rate) when this was wetted with drops of water with volumes in a range of 10 to 100 μl. The author suggested that the cold sensations experienced when such skin cooling occurs can contribute to the perception of skin wetness. Therefore, exposing the skin to a cold-dry stimulus producing such skin cooling was hypothesized to be effective in evoking an illusory perception of skin wetness.
The fact that cold stimuli able to reproduce similar skin cooling rates such as the ones occurring when the skin is physically wet, could suffice in evoking the perception of skin wetness, has been recently confirmed by Bergmann Tiest et al.,Citation40 who have shown that, when haptically manipulating dry phase-change materials which induced cool sensations, participants perceived these as being wetter than non-treated dry fabrics.
In support of the role that thermal (cold) sensations play in driving the perception of skin wetness, Niedermann and RossiCitation38 have shown that blindfolded individuals could discriminate between different drying states (i.e., 0, 5, 50, 95 and 100% dry) of different fabrics (e.g., 260 cm2 cotton and polyester samples) applied to their inner forearm, only when the different drying states (e.g., 0 and 100%) induced significantly different thermal sensations [e.g. the 0% dry fabric (i.e. fully wet) was experienced as significantly wetter than the 100% dry as the 0% dry fabric induced significantly colder thermal sensations than the 100% dry].
In line with the above, Ackerley et al.Citation41 showed that 9 blindfolded females could readily discriminate between very small amount of moisture (in the range of 1.6 μl.cm−2) applied with a tactile stimulator over different regions of the body. Although in the mentioned study no recordings of local skin temperature and thermal sensations were performed, the authors hypothesized that participants distinguished the greater from the smaller levels of moisture due to the greater evaporative cooling resulting from the residual moisture on the skin, which induced colder thermal sensations and thus wetter perceptions.
The hypothesis of coldness alone being able to drive the perception of skin wetness (regardless of skin's contact with actual moisture), was recently tested in one of our studies.Citation48 In this study, 6 cold-dry stimuli of different strength were applied in a balanced order on the bare, left forearm of 9 blindfolded female participant, while these were resting in a thermo-neutral environment. The exact temperatures of cold-dry stimuli were calculated on an individual basis and consisted of a short contact (30 s) with a cold surface set at 2, 5, 7, 10, 15 or 20°C below individual's forearm resting skin temperature. The cold-dry stimuli were delivered by a thermal probe with a contact surface of 25 cm2 and a weight of 269 g. As a result, when the application of local cold-dry stimuli on participants’ hairy skin produced a drop in skin temperature ranging between 1.4 and 4.1°C with a cooling rate of 0.14 to 0.41°C.s−1, an illusion of skin wetness perception was indeed evoked (note: 4.1°C was the highest value tested and thus this is not necessarily the upper cooling limit for skin wetness perception); whereas when cold-dry stimulations produced a drop in skin temperature of 0.2 to 0.7°C with a cooling rate of 0.02 to 0.07°C.s−1, skin wetness perception was little evoked and decreasing thermal sensations prevailed.
The hypothesis of skin wetness being a synthetic perception was further confirmed by a sub-sequent study performed by our group, in which the static application of a warm-wet stimulus (i.e. 25 cm2), with a temperature above local skin temperature, was shown not to be perceived as wet by blindfolded participants, as no skin cooling, and thus no cold sensations, occurred.Citation49
Finally, the most recent psychophysical evidence on the role of the thermal sense in the perception of skin wetness during the contact with an external stimulus has been provided by one of our latest studies.Citation11 In this study, 13 blindfolded male participants underwent a Quantitative Sensory Test, during which they actively interacted with cold-wet (i.e., 25°C), neutral-wet (i.e. 30°C) and warm-wet (i.e., 35°C) stimuli characterized by the same moisture content (i.e., 80 μl.cm−2). The stimuli were delivered by a thermal probe (i.e., 25 cm2) covered with a 100% cotton fabric, which was fully wet and brought to the required temperature before stimulation. The hairy skin of the ventral side of the left forearm and the glabrous skin of the left index finger pad were exposed to the static (i.e., only thermal cues available) and dynamic contact (i.e. thermal and tactile cues available) with the stimuli. As a result of the contact with the stimuli (and although all the stimuli presented the same wetness levels), cold-wet stimuli were perceived as significantly wetter than neutral and warm ones, particularly during the static interaction. It is therefore likely that participants relied primarily on thermal than mechanical cues to discriminate their sensation, as limited mechanosensory inputs (i.e., only in the form of static pressure) were available during the static interaction with the stimuli. In support of this hypothesis, during the subsequent dynamic interaction with the stimuli (when increased mechanosensory afferents responding to skin friction, vibration and lateral stretching were also available), wetness perception increased regardless of the thermal inputs available. Interestingly, a trend was observed with the extent of perceived wetness being higher on the hairy than on the glabrous skin. Overall, these psychophysical results indicated that participants relied primarily on their cold and tactile sensations to sense and characterize their perception of skin wetness.Citation11
In summary, the studies that have investigated what sensory inputs contribute to skin wetness perception with a magnitude estimation approach, have provided more structured evidence on the sensory inputs which could significantly contribute to drive the perception of skin wetness during the contact with an external (dry or wet) stimulus.
Overall, these results highlighted the synthetic nature of the perception of skin wetness and indicated that it is not the contact of the skin with moisture per se, but rather the integration of particular sensory inputs which could drive the perception of skin wetness.Citation48 In this respect, due to potential learning factors, thermal (cold) sensations seems to play a primary role in driving the perception of skin wetness. Finally, these studies have demonstrated that, assessing the psychophysical processes involved in the perception of skin wetness by using Quantitative Sensory Testing with a magnitude estimation paradigm can provide reliable quantitative data about the basic sensory mechanisms underlying this complex somatosensory experience.
At this point however, although perceiving coldness is likely to be determinant in humans’ ability to sense wetness in the absence of skin hygroreceptors, studies by Gerrett et al.Citation43 and everyday experience suggest that we are able to sense wetness even in the absence of coldness (e.g., during exposure to warm-humid environments or when in contact with warm water). In these particular conditions, the tactile and pressure related sensations (e.g. stickiness) resulting from the interaction between moisture and skin could therefore play a critical role in the ability to sense wetness.
Magnitude estimation studies: tactile sense in the perception of skin wetness
With regards to the potential contribution of other somatosensory modalities to the perception of skin wetness during the contact with an external (dry or wet) stimulus, the tactile sense (sub-serving the central integration of mechanosensory inputs) could represent an important source of sensory information for sensing and discriminating skin wetness ().
When the skin is exposed to external stimuli, surface's textures and properties (e.g., wetness or roughness) are usually discriminated based on the type and amount of mechanosensory inputs resulting from the skin displacement as well as the rate of movement of the stimuli across the skin.Citation50 For example, when in contact with fabrics, the level of skin wetness has been shown to increase the amount of friction within the skin-clothing system, a fact which in turn may alter the tactile sensations arising from the skin's mechanical contact with the fabric.Citation51 Gwosdow et al.Citation51 have observed that increases in physical skin wetness result in increases in the frictional force required to pull a fabric across the skin, with this being positively correlated with the level of subjective displeasure experienced. Increases in mechanical stimulation of the skin (in the form of greater skin friction) resulting from the interaction with wet materials could therefore contribute to induce and/or increase the perception skin wetness.
In this respect, Bergmann Tiest et al.Citation8 have recently provided evidence for the role of tactile inputs in the haptic perception of wetness. In their study, the authors observed that, during the interaction with wet materials (i.e. 19.6 cm2 thin and thick viscose and cotton wool), Weber fractions for wetness discrimination thresholds (i.e., psychophysical indicator of the just-noticeable difference between 2 stimuli, which is proportional to the magnitude of the stimuli)Citation25 decreased significantly when individuals were allowed dynamic as opposed to the static touching. This indicated that individuals’ skin wetness perception was increased by a higher availability of tactile information, as occurring during the dynamic exploration as opposed to the static contact with the wet materials. The authors concluded that, when thermal cues (e.g. thermal conductance of a wet material) provide insufficient sensory inputs, individuals seem to use mechanical cues (e.g., stickiness resulting from the adhesion of a wet material to the skin) to aid them in the perception of wetness.
In line with the findings of Bergmann Tiest et al.Citation8, our group has recently demonstrated that, during the contact with cold-wet (i.e. 25°C), neutral-wet (i.e. 30°C) and warm-wet (i.e. 35°C) stimuli characterized by the same moisture content (i.e., 80 μl.cm−2), cold-wet stimuli were perceived as significantly wetter than neutral and warm only during the static contact with the stimuli (i.e. when limited mechanosensory afferents were available)), and that when the participants were allowed a dynamic interaction with the stimuli (and tactile cues were therefore made available), wetness perception increased regardless of the thermal inputs available.Citation11 These results support the hypothesis that tactile cues and mechanosensory afferents are essential in aiding the perception of skin wetness.
Further psychophysical evidence in support of the potential sensory interaction occurring between thermal and mechanical cues in driving the perception of wetness was recently provided by another study performed by our group.Citation52 Our experimental design was based on the application of 6 cold-dry stimuli with different temperatures and mechanical pressures, on the bare upper and lower back of 8 blindfolded female participants. Six cold-dry stimuli, resulting from combining 3 relative temperatures (4, 8 and 15°C below the local skin temperature) and 2 mechanical pressures (7 and 10 kPa) were used in this study. As a result of the application of the stimuli, cold-dry stimulations inducing drops in skin temperature ranging between 0.6 and 4.0°C with skin cooling rates of 0.06 to 0.4°C.s−1 were shown to evoke artificial skin wetness perceptions, with colder stimuli resulting in a higher frequency and magnitude of wetness perception (note: 4°C was the highest value tested and thus this is not necessarily the upper cooling limit for skin wetness perception). However, it was observed that the application of stimuli with a higher mechanical pressure on the skin (10 vs. 7 kPa) reduced the frequency of times artificial wetness perceptions were evoked. Although thermal stimuli applied with higher and lower mechanical pressure resulted in similar drops in skin temperature, higher pressure cold-dry stimuli were indeed perceived as significantly less cold and less wet. As attenuations in thermal sensation due to an increased mechanical stimulation to the skin have been previously reported,Citation53 the outcomes of this study further strengthened the role that the level of coldness experienced plays in modulating the perception of skin wetness. Also, these findings provided evidence for the fact that cutaneous tactile afferents (as stimulated by different mechanical pressures applied to the skin) might influence the way a complex perception such as skin wetness is experienced.Citation52 Indeed, the high levels of static pressures resulting from the application of the cold-dry stimuli with a high pressure (i.e. 10 kPa), might have generated “unfamiliar” sensations which are not commonly associated to the way we learn to perceive skin wetness (e.g. when sweating or immerging a body part into a liquid, conditions which usually generate low levels of mechanical pressure on the skin). These unfamiliar sensations could have eventually resulted in the high pressure stimuli being perceived as less wet than the low pressure ones.
In summary, these psychophysical findings provided evidence for the potential role of tactile and mechanical cues in characterizing and modulating the perception of skin wetness during the skin's contact with external (dry or wet) stimuli. Light touch, low levels of mechanical pressure and increase in skin friction seem to induce an increase in the perception of skin wetness. On the other side, higher levels of static pressures (i.e. able to reduce cold sensitivity) and the related tactile sensations, seem to reduce the perception of skin wetness by generating pressure-related sensations which could be potentially different to what is experienced in natural conditions (e.g., when sweating or immerging a body part into a liquid).
Interestingly, this recent evidence (along with what has been observed in psychophysical studies investigating the role of thermal cues in the perception of wetness) appeared to be in line with what initially proposed by BentleyCitation12 on the interaction between coldness and tactile sensations as a sensory mechanism underpinning human perception of skin wetness ().
Psychophysical Approach: Sweat Production
As an alternative way to the contact with external (dry or wet) stimuli, the active production of sweat represents a mechanisms through which skin wetness can also be experienced. To our knowledge, only few studies have investigated how the level of physical skin wetness relates to the level of perceived skin wetness under conditions of sweat-induced skin wetness.
Fukazawa and HavenithCitation10 investigated thermal comfort sensitivity in relation to locally manipulated skin wetness as resulting from exercise-induced sweat production. Similarly, Gerrett et al.Citation43 investigated thermal comfort sensitivity in relation to sweat-induced skin wetness, however in a non-manipulated condition (natural sweat distribution across the torso during exercise). Finally, Lee et al.Citation42 investigated regional differences in sweat-induced perceived skin wetness during rest and moderate exercise in 25 and 32°C ambient temperature and 50% relative humidity. Overall, the results of these studies indicated that the level of perceived skin wetness strongly correlated with the level of physical skin wetness (i.e., the higher the physical wetness, the greater the perception of skin wetness) and that participants could discriminate regional differences in their perceived wetness.Citation10,42,43
Interestingly, in all these studies, skin temperature was always observed to increase significantly during the exercise protocols, a fact which indicated that participants were able to both sense and regionally discriminate sweat-induced skin wetness, despite not experiencing any cold sensations. It could be therefore suggested that in those conditions, participants relied more on tactile (i.e. stickiness of their clothing) than on thermal inputs (i.e., thermal sensations) to characterize their wetness perception.
This hypothesis could be in line with what has been previously shown for the skin's contact with an external stimulus (i.e. manual exploration of a wet material) by Bergmann Tiest et al.,Citation8 who reported that, when thermal cues (e.g. thermal conductance of a wet material) provide insufficient sensory inputs, individuals seem to use mechanical cues (e.g., stickiness resulting from the adhesion of a wet material to the skin) to aid them in the perception of wetness.
In line with the above, it could also be speculated that the greater role that tactile inputs could have played in driving the perception of skin wetness during the above mention studies, could be the result of an increased skin’ sensitivity to tactile stimuli. When sweat is produced, the internal sweat production and duct filling activates the cutaneous mechanoreceptors surrounding the sweat glands.Citation54 The increased activity of these mechanoreceptors could be responsible for the typical “tingling sensation” which is often experienced at the onset of sweating. Ultimately, this sensation of a change in the skin hydration status could contribute to an increased sensitivity to skin wetness perception at the onset of sweating under warm skin temperatures. However, as in the above mentioned studiesCitation10,42,43 the mechanical interaction at the skin as well as the skin hydration was neither manipulated nor controlled [e.g. in Fukazawa and Havenith’ s studyCitation10 only the type of garment's fabrics used to manipulate skin wetness levels was controlled in order to ensure similar tactile inputs across the whole body], these cannot provide conclusive evidence on the potential link between the thermal and mechanical changes occurring locally at the skin's surface when this was wet (due to sweating) and the resulting sensory inputs used by the participants to characterize their perception of skin wetness. Nevertheless, as the mechanisms underpinning the perception of skin wetness during the active production of sweat appear to be similar to the ones underpinning this perception during the contact with a wet stimulus (i.e., integration of thermal and tactile sensory cues), it is reasonable to hypothesize that under these conditions, common sensory processes for the perception of skin wetness could be shared. Further studies, specifically targeting these mechanisms, are therefore warranted to confirm this hypothesis.
Psychophysical Approach: Regional Differences
Due to the importance of skin wetness as a somatic perception, and due to the similarities with other bodily sensations (i.e. touch,Citation55 painCitation56 and temperature sensingCitation57,58), whose regional differences have been widely characterized across the body, an interest in investigating whether regional differences in skin wetness perception exist across the body has recently flourished.Citation10,42,43,59
This interest in mapping the perception of skin wetness across the body has been driven by olderCitation60-62 as well as more recentCitation57,58,63 investigations which examined regional differences in cutaneous thermal sensitivity. A body of literature is indeed available, indicating that the distribution of thermal sensitivity varies significantly across the human body with different regions showing different (i.e., higher/lower) sensitivity to cold and warm stimuli.Citation62 Behavioral adaptations (e.g., thermal protection of sensitive organs)Citation64 as well as anatomical (e.g., density of cutaneous thermoreceptors)Citation60 and physiological factors (e.g., central nervous integration processes)Citation65 have been shown to be responsible for such regional differences in thermal sensitivity (for a more detailed overview on the regional differences in thermosensitivity the reader is referred to ref.Citation61).
In this respect, appraising the role of cutaneous thermal sensitivity in the perception of skin wetness has led to a number of investigations attempting to examine whether regional differences in wetness perception (as induced by both sweating and contact with an external stimulus) exist across the body.
A further (and even more recent) area of interest has been the one specifically focusing on investigating the potential differences in wetness perception between glabrous (i.e. covering the palm of the hands and sole of the feet) and hairy skin (covering the rest of the body). Due to specific morphological and physiological differences between these types of skin, the question has arisen on whether glabrous skin could be more sensitive to skin wetness and vice versa.
In light of the above, the following paragraphs present a summary of the currently available psychophysical evidence on the regional differences in wetness perception across hairy skin sites, and between hairy and glabrous skin.
Hairy skin
The distribution of cutaneous sensitivity to cold (as well as to warmth, see e.g., Gerrett et al.Citation58) has been repeatedly shown to vary significantly across different hairy regions of the bodyCitation63,66,67 as well as within the same body region.Citation57 For example, the torso is suggested as among the most sensitive regions to cold.Citation63,66,67 Furthermore, the recent work of Ouzzahra et al.Citation57 has provided evidence for the presence of an uneven distribution of cold sensitivity across the front and back torso. In this respect, in line with the hypothesis that sensing skin wetness could be primarily driven by the level of coldness experienced, it would be reasonable to hypothesize that skin wetness perception could vary significantly across the body. A number of studies have investigated whether humans present regional differences in cutaneous wetness perception.Citation10,41,42
In a study in which thermal comfort sensitivity was investigated in relation to locally manipulated skin wetness (as resulting from exercise-induced sweat production), Fukazawa and HavenithCitation10 found that the torso seems to have a lower sensitivity to wetness than the limbs. Similar findings were also reported by Gerrett et al.Citation43 in a non-manipulated condition (natural sweat distribution across the torso during exercise). Lee et al.Citation42 showed that when asked, individuals reported the torso (i.e., chest and back) to be the region more often perceived as wet during rest and moderate exercise in 25 and 32°C Tair and 50% humidity. Finally, Ackerley et al.Citation41 have recently shown that when wet stimuli with different moisture contents (range: 0.8–6.6 μl.cm−2) were applied to different body regions, individuals were able to differentiate between moisture levels, with a tendency of the back as being among the most sensitive region to wetness.
The outcomes of these studies provided initial insights about the hairy regions of the body which could be more sensitive to skin wetness (e.g., limbs) and on which skin wetness might be perceived to a larger extent (e.g. the torso). However, by only measuring the physical wetness (whether due to sweat production or to contact with a wet surface) these studies had provided limited evidence on the potential link between the thermal changes occurring locally at the skin's surface when this is wet (e.g., variation in local skin temperature) and how these are perceived in terms of thermal sensations and perception of skin wetness.
In this respect, a recent study performed by our group (in which local changes in skin temperature and related thermal sensations have been linked to the perception of skin wetness),Citation59 has provided a detailed body map of cold-induced wetness perception across the human torso. In this study, 16 males underwent a quantitative sensory test during which 12 regions of the front and back of the torso were stimulated with a dry thermal probe with a temperature of 15°C below local skin temperature. Despite being dry, this cold stimulus had been previously shown to induce drops in skin temperature (and thus cold sensations) able to induce an illusion of skin wetnessCitation52 and was therefore considered appropriate to investigate the role of thermal cues in driving the perception of skin wetness.
As a result of the same cold-dry stimulation, the skin cooling response varied significantly by location with the lateral chest showing the greatest cooling and the lower back the smallest. Thermal sensations varied significantly by location and independently from regional variations in skin cooling, with colder sensations reported on the lateral abdomen and lower back. Interestingly, and similarly to what was observed for the distribution of cold sensitivity, the frequency of perceived skin wetness was significantly greater on the lateral and lower back as opposed to the medial chest ().
Figure 3. Body maps showing the regional distribution of (A) local skin cooling (°C) caused by the application (10 s) of a cold-dry stimulus previously shown to induce a perception of wetness (i.e. 15°C lower than local skin temperature), (B) absolute mean votes for resulting thermal sensation, (C) and frequency (%) of wetness perception. Regions showing greater skin cooling, colder sensations and more frequent wetness perceptions are represented in darker colors. The rating scale used by the participants to score their absolute thermal is reported next to the respective body map. Two main tendencies are shown. First, the regional differences in thermal and wetness sensation present a similar pattern across the torso (e.g., as opposed to the chest, the lateral and lower back appears more sensitive to cold and wetness). Second, these sensory patterns seem independent from the regional variations in skin cooling (i.e., regions which show greater skin cooling, such as the lateral chest, are not necessarily the ones in which the stimulus was perceived as colder and more often wet). © [The American Physiological Society]. Permission to reuse must be obtained from the rightsholder.Citation62
![Figure 3. Body maps showing the regional distribution of (A) local skin cooling (°C) caused by the application (10 s) of a cold-dry stimulus previously shown to induce a perception of wetness (i.e. 15°C lower than local skin temperature), (B) absolute mean votes for resulting thermal sensation, (C) and frequency (%) of wetness perception. Regions showing greater skin cooling, colder sensations and more frequent wetness perceptions are represented in darker colors. The rating scale used by the participants to score their absolute thermal is reported next to the respective body map. Two main tendencies are shown. First, the regional differences in thermal and wetness sensation present a similar pattern across the torso (e.g., as opposed to the chest, the lateral and lower back appears more sensitive to cold and wetness). Second, these sensory patterns seem independent from the regional variations in skin cooling (i.e., regions which show greater skin cooling, such as the lateral chest, are not necessarily the ones in which the stimulus was perceived as colder and more often wet). © [The American Physiological Society]. Permission to reuse must be obtained from the rightsholder.Citation62](/cms/asset/3b9d4daf-9125-435b-bc05-0a6cd1f33e14/ktmp_a_1008878_f0003_c.jpg)
Overall, these results confirmed the existence of regional differences in cutaneous thermosensitivity to coldCitation57,63 and indicated that these differences could translate into significant and matching regional differences in cutaneous wetness perception across the human torso.Citation59 Hence, these findings supported the hypothesis that the central integration of coldness could be critically involved in the neural processes underpinning humans’ ability to sense wetness, and that regional differences in cold sensitivity could result in matching differences in wetness perception across the torso.
In summary, the evidence provided by the above mentioned studies indicated that humans seem to regionally discriminate their wetness perception, thus suggesting potential regional differences in wetness sensitivity. These regional differences could be underpinned by regional differences in thermal (particularly cold) sensitivity, although the only evidence in support of this hypothesis is provided by a study in which wetness perceptions were induced by contact dry cooling. Hence, as regional differences in tactile sensitivityCitation56,68 could also contribute to modulate the perception of wetness across the body (particularly within the context of sweat-induced skin wetness), further studies are warranted to clarify the mechanism underlying the observed differences in regional sensitivity to skin wetness across hairy skin sites.
Hairy vs. Glabrous skin
With regards to the potential differences in skin wetness perception between hairy and glabrous skin, limited psychophysical evidence is currently available on whether wetness perception differs between these areas.
In a recent study, Ackerley et al.Citation41 found no differences between the palm of the hand (i.e. glabrous skin) and the rest of the body (i.e., hairy skin) in the ability to discriminate between externally applied wet stimuli with different moisture contents (range: 0.8–6.6 μl.cm−2). Both hairy and glabrous skin sites were indeed observed to present the same level of skin wetness sensitivity.
On the contrary, a recent study from our group has provided preliminary evidence in support of potential differences between hairy and glabrous skin sites in wetness perception.Citation11 In this study, 13 blindfolded male participants underwent a Quantitative Sensory Test, during which they actively interacted with cold-wet (i.e. 25°C), neutral-wet (i.e. 30°C) and warm-wet (i.e. 35°C) stimuli characterized by the same moisture content (i.e., 80 μl.cm−2). The stimuli were delivered by a thermal probe (i.e. 25 cm2) covered with a 100% cotton fabric, which was fully wet and brought to the required temperature before stimulation. The hairy skin of the ventral side of the left forearm and the glabrous skin of the left index finger pad were exposed to the static (i.e., only thermal cues available) and dynamic contact (i.e. thermal and tactile cues available) with the stimuli. As a result of the contact with the same stimuli, a trend was observed with the extent of perceived wetness being higher on the hairy than on the glabrous skin.
Anatomical and physiological differences between these types of skin could explain the potential differences in the ability to sense wetness across these skin sites. Indeed, hairy and glabrous skin sites differ in terms of innervation and particularly in terms of density of thermo- and mechano-sensory nerve fibers as well as in their biophysical properties. For example, the hairy skin seems to be more sensitive to thermal stimuli than the glabrous skin, which on the contrary presents higher spatial acuity.Citation69 From the receptors point of view, this could be due to the fact that, although both glabrous and hairy skin sites are innervated with slowly adapting type I mechano-sensory afferents, also known as Merkel cells (low threshold mechanoreceptors transmitting acute spatial images of tactile stimuli with remarkably high spatial resolution), glabrous skin presents a higher density of these specialized organs for tactile discrimination, a fact which could explain the higher spatial acuity to mechanical stimuli of this type of skin.Citation70 From a biophysical point of view, the presence of a thicker stratum corneum (i.e., the outermost layer of the skin) on glabrous skin, resulting in a greater thermal insulation of this type of skin, contributes to the reduced thermal conductance of the finger padCitation71 and therefore to the lower thermosensitivity of glabrous as opposed to hairy skin during short contact cooling and/or heating. This, as a result of the longer time that is needed for a given change in temperature of glabrous skin’ superficial layers to penetrate to the underlying tissues (e.g. stratum granulosum) where the thermoreceptors lay.Citation55
In this context, as thermal sensitivity seems to play the key role in sensing wetness, it is reasonable to hypothesize that, despite a larger content in highly spatially sensitive mechanoreceptive afferentsCitation70 (which could potentially contribute to an increase in the haptic perception of wetness), the lower thermal sensitivity of the glabrous skin might translate in the palm of the hands being generally less sensitive to wetness than the rest of the body.
Although the recent findings from our study seem supporting the hypothesis for which the higher thermal sensitivity of hairy skin might translate in this type of skin being generally more sensitive to wetness,Citation11 the limited number of studies specifically comparing hairy and glabrous skin’ wetness perception highlights the need for further experimental investigations.
Summary of Psychophysical Results
The evidence emerging form psychophysical investigations of human hygrosensation have provided insights on the sensory mechanisms (i.e. integration of cutaneous temperature and tactile inputs) which seems to underpin humans’ remarkable ability to sense and discriminate between moisture levels, both when these are the result of a contact with a wet stimulus as well as when resulting from actively sweating (). These psychophysical results can be summarised as follow:
Figure 4. Summary of sensory mechanisms (i.e., integration of cutaneous temperature and tactile inputs) which seems to underpin humans ability to sense and discriminate between moisture levels on the skin, both when these are the result of a contact with an external stimulus (A–C) as well as when resulting from actively sweating (D).
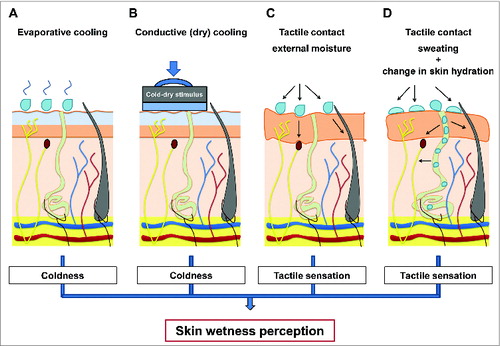
The first scientist who attempted to explain the sensory basis of the perception of skin wetness was Bentley,Citation12 who in 1900 proposed a sensory-blending hypothesis which suggests the blend of pressure and coldness as responsible for evoking the perception of wetness. Since Bentley's study, a number of studies have been performed to investigate human perceptual responses to either: a) skin's contact with external (dry or wet) stimuli; b) the active production of sweat.
It has been shown that humans seem to readily discriminate between levels of wetness which differ as little as of 0.04 ml. However, the noticeable difference in wetness levels seems to increase with increasing magnitude of wetness (i.e., the wetter the stimuli, the greater the difference in moisture content required to perceive differences in the wetness level of the stimuli).
Thermal (cold) sensory inputs have been shown to play a primary role in driving the perception of skin wetness. Indeed, it has been proposed that humans tend to associate the cold sensations evoked by the drop in skin temperature occurring during the evaporation of moisture from the skin, as a signal of the presence of moisture, and thus wetness, on the skin surface ().
Cold-dry stimuli able to reproduce skin cooling rates similar to the ones occurring when the skin is wet have been suggested and proven effective in evoking the perception of wetness (). Furthermore, warm stimuli (above skin temperature) have been shown to suppress the perception of skin wetness when statically applied to the skin. Hence, the role of cold sensations per se has been shown to be critical in driving the perception of skin wetness.
When thermal cues are however limited, individuals seems indeed to rely on tactile discrimination (e.g., stickiness) to sense skin wetness (). Psychophysical evidence has been provided on how thermal (cold) and tactile sensory cues could be integrated to aid the discrimination of skin wetness during the contact with an external (dry or wet) stimulus.
Few studies have investigated how the level of physical skin wetness relates to the level of perceived skin wetness under conditions of sweat-induced whole-body skin wetness. It appears that under conditions of sweat-induced skin wetness, individuals could rely more on mechanical (i.e. stickiness and changes in skin hydration) than on thermal inputs (i.e., thermal sensations) to characterize their skin wetness perception ().
Regional variations in wetness perception across the body have been shown and insights about the hairy regions of the body on which skin wetness might be perceived to a larger extent (e.g. the torso) have been provided. Specifically, wetness perception seems to vary significantly across the torso, with regions presenting higher cold sensitivity (i.e. lower back) also showing higher wetness perception.
Only one study has demonstrated that hairy skin could be more sensitive to skin wetness than glabrous skin (possibly due to the higher thermal sensitivity of this type of skin).
The wealth of information provided by the psychophysical findings outlined above has contributed to identify the sensory inputs (i.e., temperature and tactile) which are likely to contribute to the perception of skin wetness in the absence of specific skin hygroreceptors. However, the identification of such sensory cues has represented only the first step in the process of elucidating how peripheral (i.e. sensory receptors and nerve fibers) and central nervous structures (i.e., cortical and sub-cortical areas involved in somatosensation) interact in giving rise to the perception of skin wetness. As that, the neural mechanisms underlying humans’ ability to sense warm, neutral and cold wetness on their skin are still largely obscure.
In this respect, our group have recently attempted to elucidate the peripheral neural mechanism of human wetness perception by combining psychophysical and neurophysiological methods.Citation11 In doing so, this study has produced a neurophysiological model of human cutaneous wetness sensitivity. The findings from this recent study will be discussed in the following paragraph. As this approach represents the first to our knowledge to have specifically explored the peripheral neural mechanism of the perception of skin wetness, in the absence of similar neurophysiological studies in humans, the findings of this study will be compared with results from animal studies, in which the neuromolecular bases of humidity sensation in other hygroreceptor-lacking organisms have been recently elucidated.
Neurophysiological Bases of Skin Wetness Perception
Psychophysical evidence on the mechanisms of human hygrosensation has indicated that cutaneous thermal and mechanosensory inputs, in the form of coldness and light mechanical pressure, represent the main peripheral drivers of the perception of skin wetness. Hence, understanding the neurophysiology of skin thermal and mechanosensory afferents is critical in order to identify peripheral neural pathways which could underpin the central processing of skin wetness perception, as operated by higher order neural structures (e.g., cortical and sub-cortical regions). In light of the above, and before examining the available evidence on the neurophysiology of skin wetness perception, a brief outline of the neural mechanisms underlying cutaneous thermal and tactile sensitivity will be presented. For a more detailed overview, the reader is referred to refs.Citation55,62
Neurophysiology of cutaneous thermal and tactile afferents
Human cutaneous thermal sensations are peripherally sub-served by cold-sensitive, myelinated Aδ-nerve fibers (with conduction velocities ranging from 5–30 m.s−1) and by cold- and warm-sensitive, unmyelinated C-nerve fibers (conduction velocities ranging from 0.2–2 m.s−1),Citation65,72 transmitted through the spino-thalamic tract and the dorsal-column medial lemniscal pathway,Citation55 and centrally integrated by the primary and secondary somatosensory cortices as well as the insular cortex (a cortical region involved in cold and warm temperatures sensation, as well as pain and touch).Citation73
Myelinated Aδ-nerve fibers represent the vast majority of the so-called “cold fibers.” At steady state temperatures cold fibers have a characteristic stimulus response function which is bell-shaped, with a maximal steady-state activity between 20 and 30°C and lower activity at lower and higher temperatures.Citation65 At maintained temperatures above 40°C or below 17°C, cold fibers maintain a very low frequency discharge or become silent. Conduction velocities for these fast-responding fibers range from 5–30 m.s−174. Characterized by small receptive fields, these fibers primarily sub-serve conscious cold sensations.
C-nerve fibers (i.e. polymodal afferents responding to nociceptive, warm, cool and light mechanical stimulation with conduction velocities ranging from 0.2–2 m.s−1), represent the vast majority of the afferent warmth fibers.Citation75 These fibers have ongoing activity at static temperatures of 30°C or more. The function of their discharge rate versus steady state stimulus temperature follows a bell-shaped curve, with maximum discharge at 40–43°C and minimal activity at 50°C. Characterized by small receptive fields, these fibers primarily sub-serve conscious warmth sensations.Citation61
The recent discovery of the Transient Receptor Potential (TRP) cation channels has opened to a better understanding of the molecular logic behind peripheral temperature sensation.Citation76 TRP(s) represent a family of cation channels, which are expressed in the cell membrane of cutaneous free nerve endings (such as thermosensitive A- and C-type nerve fibers) and which are activated at specific temperature ranges. When activated, these channels induce an increase in the resting membrane potential of the specific nerve ending with which they are associated, thus generating specific temperature-dependent afferent inputs.Citation77 Cumulatively, these ion-channels cover a wide range of temperatures (i.e., ∼0 to ∼50°C).Citation78
With regards to somatosensory nerve fibers with mechano-sensitive properties, these lie in the dorsal root and trigeminal ganglia of the spinal cord, from which they extend sensory afferents to the skin. These are classified into 3 broad groups (i.e. C, Aß, and Aδ fibers) and end in the skin both in the form of free nerve endings and with specific corpuscles (i.e., specialized cells).Citation79
In general, weak, innocuous mechanical force applied to the skin activates the so-called low-threshold mechanoreceptors, namely Pacinian corpuscles, Meissner's corpuscles, Merkel's disks and Ruffini endings. These mechanoreceptors are associated to Aß nerve fibers, present conduction velocities in the range of 16–100 m.s−1, and differ between each other in terms of the stimuli they respond to as well as in terms of their receptive fields.Citation55,70
Pacinian and Meissner's corpuscles respond to the initial and final contact of a mechanical stimulus on the skin and are classified as rapidly adapting low-threshold mechanoreceptors, whereas Merkel's disks and Ruffini endings continue to fire during a constant mechanical stimulus and are classified as slowly adapting low-threshold mechanoreceptors. With regards to their receptive fields, Meissner's corpuscles and Merkel's disks possess small receptive fields, whereas Pacinian corpuscles and Ruffini have large receptive fields.
Low-threshold mechanoreceptors encode and transmit cutaneous tactile inputs which are then centrally integrated by the primary and secondary somatosensory cortices as well as the insular cortex and the posterior parietal cortex through the dorsal-column medial lemniscal pathway.Citation55
In recent years, numerous mechano-sensitive molecules and cation channels have been identified, which could contribute in gating and initiating mechanotransduction and touch sensations in mammals.Citation79 Candidate channels are Degenerin/Epithelial sodium channels (DEG/ENaC), TRP channels and 2-pore potassium (KCNK) channels. Transduction mechanisms for these cation channels could be stretch-activated, when force in the lipid bilayer cell membrane changes; alternatively, these channels could be tethered to the cytoskeleton or extracellular matrix, and could be opened by changes in the tension in the linkages between the channel and the cytoskeleton; finally, the transduction channels could be coupled to mechanically sensitive proteins through signaling intermediates.Citation80 However, as the molecular bases of tactile and mechano sensations have only recently started to be unveiled, and as the vast majority of the literature is based on in vitro and/or in vivo animal studies, these hypotheses still require further testing in humans.
Neurophysiological approach
The psychophysical evidence examined so far indicated that coldness and tactile sensations drive the perception of skin wetness () and that as humans we tend to consistently associate coldness and light touch to the idea of a typical wet stimulus. This sensory association is not entirely surprising, particularly when considering that the sensory inputs associated to the physical experience of wetness are often generated by heat transfer in the form of evaporative cooling,Citation41 and mechanical pressure in the form of friction and stickiness.Citation81 As briefly outlined, this sensory information is generally encoded by cutaneous cold-sensitive, myelinated Aδ-nerve fibers (with conduction velocities ranging from 5–30 m.s−1)Citation74 and by cutaneous mechanosensory Aß-nerve fibers (with conduction velocities ranging from 16–100 m.s−1).Citation79 Hence, it is reasonable to hypothesize that the central integration of coldness and mechanosensation, as subserved by peripheral myelinated A-nerve fibers, might be the primary neural process underpinning humans’ ability to sense wetness.
Perceptual learning and somatosensory decision making could contribute to explain why the brain processes sensory information about the perception of wetness in such fashion.Citation82 As the skin seems not to be provided with hygroreceptors,Citation7 it is reasonable to hypothesize that the perception of skin wetness is shaped by sensory experience (i.e. we learn to associate the sensations experienced when in contact with moisture to the actual perception of skin wetness). In this respect, the primary and secondary somatosensory cortices (cortical regions involved in integration somatosensory inputs among which cutaneous thermal and tactile), the insular cortex (a cortical region involved in cold and warm temperature sensation)Citation73 as well as the posterior parietal lobe (a cortical region concerned with integrating the different somatic sensory modalities necessary for perception)Citation55 could be involved in generating a neural representation of a “typical wet stimulus.” This could be based on the multimodal transformation (i.e., information from one sensory sub-modality can be transformed into a map or reference frame defined by another sub-modality) of the somatosensory inputs generated when the skin is physically wet.Citation83
The hypothesis that the central integration of cutaneous thermal and tactile A-type nerve afferents represents a specific processing mechanisms which has developed in humans to sense wetness in the absence of skin hygroreceptors, has been tested by our group.Citation11 In one of our recent studies we hypothesized that artificially dampening cutaneous cold and mechano sensitivity, by selectively reducing the activity of A-nerve fibers, would translate in significantly impairing the ability to perceive skin wetness.
In order to do so, we first characterized participants’ wetness perception with psychophysical methods. Thirteen blindfolded male participants underwent a Quantitative Sensory Test, during which the left forearm and the left index finger pad were exposed (i.e. statically and dynamically) to cold-wet (i.e., 25°C), neutral-wet (i.e. 30°C) and warm-wet (i.e., 35°C) stimuli characterized by the same moisture content (i.e. 80 μl.cm−2). As a result of the contact with stimuli (and as expected based on previous psychophysical evidence), cold-wet stimuli were perceived as significantly wetter than neutral and warm ones, particularly during the static interaction (i.e., when only low-threshold mechanoreceptors responded to the static pressure of the stimulus against the skin). Also, during a subsequent dynamic interaction with the stimuli (when additional low-threshold mechanoreceptors responding to skin friction, vibration and lateral stretching were engaged by the dynamic stimulation), wetness perception increased regardless of the thermal inputs available.
At this point, having confirmed the role that cutaneous thermal (i.e. cold sensitive) and tactile (i.e., mechanosensory afferents responding to skin friction, vibration and lateral stretching) A-type nerve afferents played in participants’ ability to sense wetness, we tested wetness perception in the same participants, after having selectively reduced the activity of their cutaneous cold- and mechanosensitive A-nerve fibers. This was accomplished by adopting a neurophysiological method based on a modified local compression-ischemia protocol. This method has been previously shown to induce a dissociated reduction in A-fibers afferent activityCitation84,85 as the compression ischemia impacts transmission in myelinated A-fibers before C-fibers (i.e. primarily sub-serving conscious warmth and pain sensitivity) are affected.Citation86 Compression-ischemia was induced by inflating a sphygmomanometer cuff on the upper arm of blindfolded participants to a suprasystolic pressure (i.e., 140 mmHg) for a maximum duration of 25 min.
As soon as the selective reduction in A-fibers afferent activity was observed effective in reducing cutaneous cold and mechano (i.e. light brush) sensitivity, the same participants performed the same Quantitative Sensory Test as performed under normal A-nerve fibers function (i.e., interaction with cold-wet, neutral-wet and warm-wet stimuli characterized by the same moisture content).
As a result (and when compared to the wetness perceptions recorded under normal A-nerve fibers function), a significant reduction in the ability to sense wetness was observed in all participants, both on hairy and glabrous skin sites. Hence, it was concluded that the central integration of conscious coldness and mechanosensation, as sub-served by peripheral myelinated A-nerve fibers, could be the primary neural process underpinning humans’ ability to sense wetness.Citation11
Based on the findings of this recent mixed approach (i.e. psychophysical and neurophysiological), a neurophysiological model of skin wetness perception was developed, in order to explain how wetness can be sensed in humans. This model is based on the hypothesis that the brain infers about the perception of wetness in rationale fashion,Citation87 and that the integration of cold and tactile sensory inputs represents the main processing pathway for sensing wetness ().
Figure 5. Neurophysiological model of cutaneous wetness sensitivity as developed by Filingeri et al. Mechano Aß, cold Aδ and warm C sensitive nerve fibers and their projections from the skin, through peripheral nerve, spinal cord (via the dorsal-column medial lemniscal pathway and the spinothalamic tract), thalamus and somatosensory cerebral cortex (including the primary and secondary somatosensory cortex cortices SI and SII, the insular cortex and the posterior parietal lobe) are shown. (A and B) Show the neural model of wetness sensitivity (consisting of Aδ and Aß afferents) under normal and under selective reduction in the activity of A-nerve fibers respectively. (C, E and G) Show the pathways for wetness sensitivity during static contact with warm, neutral and cold moisture. (D, F and H) Show the pathways for wetness sensitivity during dynamic contact with moisture. © [The American Physiological Society]. Permission to reuse must be obtained from the rightsholder.Citation11
![Figure 5. Neurophysiological model of cutaneous wetness sensitivity as developed by Filingeri et al. Mechano Aß, cold Aδ and warm C sensitive nerve fibers and their projections from the skin, through peripheral nerve, spinal cord (via the dorsal-column medial lemniscal pathway and the spinothalamic tract), thalamus and somatosensory cerebral cortex (including the primary and secondary somatosensory cortex cortices SI and SII, the insular cortex and the posterior parietal lobe) are shown. (A and B) Show the neural model of wetness sensitivity (consisting of Aδ and Aß afferents) under normal and under selective reduction in the activity of A-nerve fibers respectively. (C, E and G) Show the pathways for wetness sensitivity during static contact with warm, neutral and cold moisture. (D, F and H) Show the pathways for wetness sensitivity during dynamic contact with moisture. © [The American Physiological Society]. Permission to reuse must be obtained from the rightsholder.Citation11](/cms/asset/65f76841-887d-4eff-834a-455111e01b14/ktmp_a_1008878_f0005_c.jpg)
According to this model, thermal and mechanosensory inputs could be differently weighted in relation to the nature of stimulation (i.e., dry or wet; cold, warm or neutral; static or dynamic) to aid in the perception of wetness. For instance, in the presence of warm-wetness, tactile and mechanical inputs (e.g. skin friction and stickiness) will be more important than thermal, due to the absence of the specific thermal component (i.e. coldness) which has been shown to play a key role in contributing to the sensory pathway for wetness (). On the contrary, within conditions of cold-wetness, thermal (cold) inputs will initially prevail in driving the perception of wetness (i.e., during static contact with moisture, when only mechanosensory afferent responding to static pressure are excited by the stimulus), while additional mechanical inputs (e.g., responding to skin friction, vibration and lateral stretching) will further facilitate wetness discrimination in case these are also available (i.e. during dynamic contact with moisture) ().
The peripheral neural mechanisms for sensing wetness proposed in this model agree with previous psychophysical literature. For instance, and as previously mentioned, Bergmann Tiest et al.Citation8 have recently provided psychophysical evidence about the fact that when thermal cues (e.g. thermal conductance of a wet material) provided insufficient sensory inputs, individuals used mechanical cues (e.g., stickiness resulting from the adhesion of a wet material to the skin) to aid them in the perception of wetness during the haptic exploration of a wet material. In this particular case (and in line with the model proposed) the absence of specific cold sensations during the contact with wet materials could lead to a need for more tactile inputs (e.g. skin friction and stickiness) in order to compensate for the lack of one of the specific sensory afferent (i.e., coldness) necessary to process skin wetness at a central level.
In line with the above, the model presented could also provide insights on why, within conditions of active sweating, individuals are able to perceive skin wetness despite no drops in skin temperature are often observed and no cold sensations are usually reported.Citation10,42,43 Indeed, it could be proposed that under these conditions individuals could rely more on tactile (i.e. interactions skin-sweat-clothing) than on thermal inputs to sense wetness. Finally, this neural model agrees with psychophysical evidence on the primary role of cold sensation in driving the perception of skin wetness. Indeed, cold-dry stimuli have been shown to induce an illusion of skin wetness when the same induce cold sensations similar to the ones experienced when actual moisture evaporates from the skin. This simple but powerful illusion highlights the fact that the brain seems to rely on specific sensory inputs to give rise to the perception of wetness, and that this perception presents a synthetic nature: in the presence of the specific thermal and tactile sensory inputs, wetness will be sensed regardless of whether the inputs are produced by actual contact with moisture or by dry stimuli.Citation11
A potential explanation for the synthetic nature of skin wetness is given by the fact that the brain could process this perception in a rationale fashion, according to the Bayesian concept of perceptual inference.Citation88 According to this framework, sensory systems (such as the somatosensory one) acquire sensory knowledge of the environment and use this sensory experience to infer about the properties of specific stimuli.Citation87 As the sensory inputs received from the surrounding environment are generally multimodal (i.e., involving different sensory cues), noisy and ambiguous, perceptual systems are thought to perform on-line tasks aiming to predict the underlying causes for a sensory observation in a fashion which is considered as near optimal.Citation89 In this context, humans have been shown to integrate the different sensory cues associated with an external stimulus and to infer the most probable multimodal perception, by taking into account the reliability and variability of each sensory cue involved in the perceptual process.Citation90,91 Skin wetness seems to be one of such perceptual experiences, for which the brain infers according to a specific sensory information processing model,Citation11 which could take into account the reliability of the sensory information provided by each sensory modality (i.e. thermal and tactile) in order to determine whether wetness is / is not present and thus experienced.Citation11
At what level of the neuroaxis such sensory integration occurs and what structures are involved in the processing of thermal and tactile somatosensory inputs coded by neuronal populations?
From a neuroanatomical point of view, it could be proposed that such perceptual inference could be operated at a cortical level. The primary sensory cortex as well as the insular cortex has been shown to integrate initial tactile and thermo-sensory information which are subsequently transferred to nearby uni-modal association areas and ultimately elaborated by multimodal association areas (such as prefrontal, parietotemporal and limbic cortices) in the context of perception and decision making.Citation73,82,92 Multimodal association areas are important for the brain to construct an internal representation of the sensory stimulus to be used for successive behavior-oriented use.Citation25 It could be therefore speculated that the neural processes underpinning the Bayesian model for the perception of wetness proposedCitation11 could be ultimately elaborated at a cortical level.
However, evidence provided by studies investigating the interplay of sub-modalities (e.g., populations of different mechanosensory afferents mediating shape, texture, motion, stretch and vibration perceptions) in cutaneous tactile sensibility to object manipulations, indicate that tactile cues from different mechanoreceptor classes could be combined already in different sub-cortical rely nuclei (e.g. cuneate nucleus and ventroposterior lateral nucleus of the thalamus) before reaching the somatosensory cortex for further processing.Citation93
Hence, it cannot be excluded that convergence of different skin wetness-induced thermo- and mechanosensory inputs could already occur at a sub-cortical level and that such integration could influence further processing by higher order cortical areas.
Nevertheless, regardless of whether such perceptual integration occurs primarily at a sub-cortical or cortical level, what emerges from recent studiesCitation11 is that convergence of somatosensory inputs is clearly and critically important for the brain to infer about the perception of wetness.
This is particularly highlighted by the fact that, although cold and mechanical inputs can individually evoke the perception of wetness,Citation48,52 their dynamic combinations result in an even more powerful estimate of the perception of wetness.Citation11 In support of this observation is that evidence from Bayesian perceptual inference indicates that the combination of 2 or more sensory cues (i.e., cue combination or multisensory integration) in sensory estimation often results in more accurate percepts than would be available from either cue alone.Citation88
In summary, drawing upon psychophysical evidence, novel neurophysiological evidence for the peripheral sensory mechanisms underpinning human skin wetness perception has been recently provided. Based on the above, potential central integration mechanisms have been hypothesized, which could contribute to explain how the brain processes such a complex sensory experience. However, as the neural bases of skin wetness perception have only recently started to be unveiled, further studies are needed, in order to explore the questions opened by the development of this sensory model for human skin wetness perception. In this respect, while it is hoped that these investigations will shortly contribute to broaden and deepen our understanding of the neural mechanisms underpinning human hygrosensation, the findings of a recent study on the neuromolecular bases of humidity detection in an animal lacking hygroreceptors (i.e. the free-living roundworm Caenorhabditis elegans)Citation94 could already support the general sensory mechanisms proposed for human hygrosensation.Citation11,95 Hence, in order to provide the reader with comparative evidence on the mechanisms used for humidity detection by other hygroreceptor-lacking living organisms, due to their relevance in the context of human hygrosensation, a brief overview of these recent findings will be presented.
Human and animal hygrosensation
In their recent work, Russell et al.Citation94 have provided the first neuromolecular evidence for the existence of a specific hygrosensation strategy in an animal lacking hygroreceptors (i.e., the free-living roundworm Caenorhabditis elegans). The Caenorhabditis elegans was hypothesized to be sensitive to humidity due to its small volume and hydrostatic skeleton, a fact which makes it vulnerable to desiccation and over-hydration, and therefore potentially attracted by particular humidity levels deemed optimal for survival.
To determine whether this animal could migrate to a preferred level of ambient humidity, Russell et al.Citation94 designed a specific hygrotaxis assay which allowed testing of the directed response of a motile organism to moisture. The hygrotaxis assay was performed both with non-mutant and mutant worms lacking specific genes expressed on different sensory neurons (e.g., among which the ones required for olfaction, osmosensation, temperature- and mechano-sensation) in order to identify the neuromolecular bases of the sensory pathways used by these hygroreceptor-lacking worms to detect humidity.
Russell et al.'s resultsCitation94 indicated that the specific genes, cation channels and sensory neurons which underpin Caenorhabditis elegans’ ability to sense mechanical- and temperature-related stimuli, were functionally essential for this organism's ability to sense humidity. Indeed, when deprived of both temperature activated TRP cation channels and mechanically activated DEG/ENaC cation channels (which have been identified as molecular transducers of mammalian and non-mammalian temperature-Citation96 and mechano-sensitivityCitation79 respectively), the hygro-receptor lacking Caenorhabditis elegans showed an impaired ability to detect humidity.Citation94 It was concluded that the ability to sense humidity of this hygroreceptor-lacking organism could rely upon the central integration of sensory cues generated by changes in the mechanical (i.e. skin hydration) and thermal properties (i.e., skin temperature) of the worm’ skin when this is exposed to different humidity levels.
Remarkably, these proposed mechanisms of multimodal sensory integration of mechanical and thermal inputs, which underpin Caenorhabditis elegans’ hygrosensation strategy, are in line with the neurophysiological mechanisms proposed for human hygrosensation (i.e. integration of thermal and tactile inputs occurring at the skin’ surface when wet), thus indicating that potentially universal mechanisms of mechano- and temperature-related sensory transduction could underpin the hygrosensation strategy of hygroreceptor-lacking organisms, among which humans.Citation11,95 However, further research is warranted, as numerous questions still remain unanswered, particularly with regards to the neuromolecular bases of human hygrosensation. Indeed, our understanding of the molecular bases of peripheral temperature and mechano-transduction in humans has only recently started to be uncovered (for an extensive review see ref.Citation96) and whether temperature gated and mechanically activated cation channels (similar to the ones observed in the Caenorhabditis elegans) could also be functionally essential for human hygrosensation, remains a matter of speculation.
Conclusions and Future Directions
In conclusion, although the ability to sense skin wetness represents an important human sensory feature (both for autonomic and behavioral adaptations), the neural mechanisms which underpin this common sensory experience have only recently started to be unveiled. Historically, psychophysical studies have provided consistent evidence and directions for identifying the sensory inputs underpinning this cutaneous perception for which we are not provided with specific sensory organs, i.e., hygroreceptors. The findings of these studies have highlighted the key role played by cutaneous thermal (cold) and tactile afferents in our ability to sense wetness, both when this results from the contact with external wet stimuli as well as when actively sweating. More recently, the psychophysical evidence has been used to develop a neurophysiological approach to the study of human wetness perception. By investigating the problem of how human sense skin wetness with neurophysiological methods, evidence has been provided in support of the existence of specific sensory processing mechanisms for human wetness perception, which have been confirmed to be based on the central integration of cutaneous thermal (cold) and tactile sensory inputs. This central integration seems to rely upon specific neural pathways, whose disruption has been shown to affect the ability to sense wetness in humans.
It could therefore be proposed that, in the absence of specific skin hygroreceptors, humans have developed a specific hygrosensation strategy through biophysical (i.e. thermal and mechanical changes induced by the presence of moisture on the skin), neurophysiological (i.e., central integration of afferents inputs from thermo- and mechano-sensitive cation channels and nerve fibers) and psychophysiological mechanisms (i.e. perceptual inference operated by cortical and sub-cortical somatosensory and association areas) which are learned and shaped by sensory experience (). Remarkably, this hygrosensation strategy (i.e., multimodal integration of thermal and tactile sensory inputs occurring at the skin when wet) seems to be shared by other hygroreceptor-lacking animal species (e.g. Caenorhabditis elegans), a fact which indicates that potentially universal mechanisms of mechano- and temperature-related sensory transduction could exist and could be shared across a wide range of species, including humans. However, the neural bases of skin wetness perception have only recently started to be unveiled and still little is known on the peripheral and central neural mechanisms which allows humans to sense wetness on their skin. Furthermore, as our understanding of the molecular bases of peripheral temperature and mechano-transduction in humans is still limited, the neuromolecular mechanisms for which skin wetness is transduced (e.g., involvement of temperature gated TRP channels and mechanically activated DEG/ENaC channels) remain obscure. Future investigations should therefore deal with the question of whether pharmacological manipulation of these temperature and mechanical activated channels could influence the ability to sense humidity and wetness in humans.
Figure 6. Conceptual model of human hygrosensation. The model comprises biophysical (i.e., thermal and tactile inputs induced by the presence of moisture on the skin), neurophysiological (i.e., central integration of afferents inputs from thermo-sensitive TRP cation channels and nerve fibers and mechano-sensitive DEG/ENaC cation channels and nerve fibers) and psychophysiological mechanisms (i.e., perceptual inference operated by cortical and sub-cortical somatosensory and association areas) which allow humidity and wetness detection in humans. The skin's contact with moisture generates thermal and tactile inputs which are peripherally integrated by specific nervous structures. These inputs evoke thermal and tactile sensations which, in the absence of specific hygroreceptors, are associated to the perception of skin wetness. Repeated exposures to these stimuli (i.e., sensory experience) contribute to generate a neural representation of a typical wet stimulus via learning mechanisms. At this point, only if the learnt combination of stimuli (i.e., coldness and stickiness), as coded by the specific neural afferents (i.e., A-nerve fibers) is presented, wetness will be sensed. In the occurrence of physical wetness on the skin, the bottom-up processes (i.e., combination of thermal and mechanical sensory afferents) as well as the top-down ones (i.e., inference of the potential perception based on the neural representation of a typical wet stimulus) might therefore interact in giving rise (or not) to the perception of wetness.
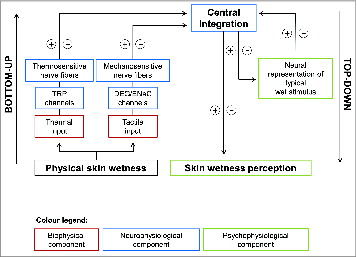
Understanding the molecular mechanisms of human hygrosensation and wetness perception could be used to develop specific treatment strategies targeting rescue and/or amelioration of sensory function in pathological conditions such as Multiple Sclerosis and polyneuropathies, conditions which are characterized by altered somatosensory function (e.g. symptoms such as spontaneous sensations of cold wetness are often experienced across the body by individuals suffering from these conditions). The fact that such an approach has already been used in other research areas (e.g., development of specific analgesic drugs targeting temperature sensitive TRP channels involved in the development of acute and chronic pain)Citation96 represents a promising avenue for future research aiming to elucidate the molecular mechanisms of human hygrosensation.
Disclosure of Potential Conflicts of Interest
No potential conflicts of interest were disclosed.
About the Authors
Davide Filingeri has recently completed a PhD in environmental physiology and somatosensory neuroscience at Loughborough University. His research has focused on the investigation of the neurophysiology of human skin wetness perception. By applying the principles of Bayesian perceptual inference, Davide's research has culminated in the development of the first neurophysiological model which explains how humans perceive skin wetness, despite having no skin humidity receptors. Davide's research interests cover the field of human and environmental physiology and somatosensory neuroscience.
George Havenith is the Director of the Environmental Ergonomics Research Center and has a Chair in Environmental Ergonomics and Physiology. His research covers two main topic areas, one being Human Thermal Physiology Environmental Ergonomics, and the other being heat and mass (vapor) transfer through clothing. Though these are quite distinct subject areas, they interact in most research application projects, and are then also supplemented with a third area: clothing ergonomics.
Acknowledgments
The present research was done in the context of an industry co-funded Doctoral degree.
Funding
Loughborough University and Oxylane Research provided financial support.
Additional information
Notes on contributors
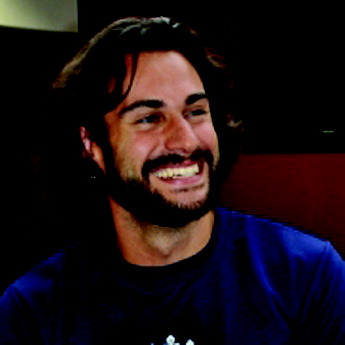
Davide Filingeri
Davide Filingeri has recently completed a PhD in environmental physiology and somatosensory neuroscience at Loughborough University. His research has focused on the investigation of the neurophysiology of human skin wetness perception. By applying the principles of Bayesian perceptual inference, Davide's research has culminated in the development of the first neurophysiological model which explains how humans perceive skin wetness, despite having no skin humidity receptors. Davide's research interests cover the field of human and environmental physiology and somatosensory neuroscience.
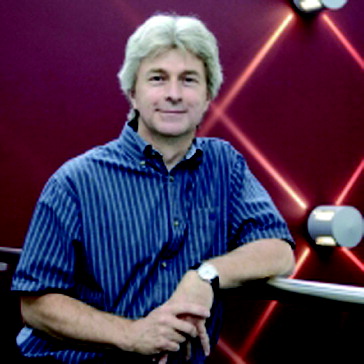
George Havenith
George Havenith is the Director of the Environmental Ergonomics Research Center and has a Chair in Environmental Ergonomics and Physiology. His research covers 2 main topic areas, one being Human Thermal Physiology / Environmental Ergonomics, and the other being heat and mass (vapor) transfer through clothing. Though these are quite distinct subject areas, they interact in most research application projects, and are then also supplemented with a third area: clothing ergonomics.
References
- Gagge AP. A new physiological variable associated with sensible and insensible perspiration. Am J Physiol 1937; 120: 277-87.
- Candas V, Libert J, Vogt J. Human skin wettedness and evaporative efficiency of sweating. J Appl Physiol Respir Environ Exerc Physiol 1979; 46: 522-8; PMID:438022
- Nadel ER, Stolwijk JAJ. Effect of skin wettedness on sweat gland response. J Appl Physiol 1973; 35: 689-94; PMID:4770352
- Havenith G, Bröde P, den Hartog E, Kuklane K, Holmer I, Rossi RM, Richards M, Farnworth B, Wang X. Evaporative cooling: effective latent heat of evaporation in relation to evaporation distance from the skin. J Appl Physiol (1985) 2013; 114: 778-85; PMID:23329814; http://dx.doi.org/10.1152/japplphysiol.01271.2012
- Havenith G. Individualized model of human thermoregulation for the simulation of heat stress response. J Appl Physiol (1985) 2001; 90: 1943-54; PMID:11299289
- Tichy H, Kallina W. Insect hygroreceptor responses to continuous changes in humidity and air pressure. J Neurophysiol 2010; 103: 3274-86; PMID:20375249; http://dx.doi.org/10.1152/jn.01043.2009
- Clark R, Edholm O. Man and his Thermal Environment. London: E. Arnold, 1985.
- Bergmann Tiest WM, Kosters ND, Kappers AML, Daanen HAM. Haptic perception of wetness. Acta Psychol (Amst) 2012; 141: 159-63; PMID:22964056; http://dx.doi.org/10.1016/j.actpsy.2012.07.014
- Driver J, Spence C. Multisensory perception: Beyond modularity and convergence. Curr Biol 2000; 10: R731-5; PMID:11069095; http://dx.doi.org/10.1016/S0960-9822(00)00740-5
- Fukazawa T, Havenith G. Differences in comfort perception in relation to local and whole body skin wettedness. Eur J Appl Physiol 2009; 106: 15-24; PMID:19159949; http://dx.doi.org/10.1007/s00421-009-0983-z
- Filingeri D, Fournet D, Hodder S, Havenith G. Why wet feels wet? A neurophysiological model of human cutaneous wetness sensitivity. J Neurophysiol 2014; 112: 1457-69; PMID:24944222; http://dx.doi.org/10.1152/jn.00120.2014
- Bentley I. The synthetic experiment. Am J Psychol 1900; 11: 405-25; http://dx.doi.org/10.2307/1412750
- Craig AD. Interoception: the sense of the physiological condition of the body. Curr Opin Neurobiol 2003; 13: 500-5; PMID:12965300; http://dx.doi.org/10.1016/S0959-4388(03)00090-4
- Gin H, Baudoin R, Raffaitin CH, Rigalleau V, Gonzalez C. Non-invasive and quantitative assessment of sudomotor function for peripheral diabetic neuropathy evaluation. Diabetes Metab 2011; 37: 527-32; PMID:21715211; http://dx.doi.org/10.1016/j.diabet.2011.05.003
- Kim J, Lee M, Shim HJ, Ghaffari R, Cho HR, Son D, Jung YH, Soh M, Choi C, Jung S, et al. Stretchable silicon nanoribbon electronics for skin prosthesis. Nat Commun 2014; 5: 5747; PMID:25490072; http://dx.doi.org/10.1038/ncomms6747
- Gagge AP, Gonzalez R. Mechanisms of heat exchange: biophysics and physiology. Compr Physiol 2011, Supplement 14: Handbook of Physiology, Environmental Physiology: 45-84. First published in print 1996; http://dx.doi.org/10.1002/cphy.cp040104
- Kondo N, Nakadome M, Zhang K, Shiojiri T, Shibasaki M, Hirata K, Iwata A. The effect of change in skin temperature due to evaporative cooling on sweating response during exercise. Int J Biometeorol 1997; 40: 99-102; PMID:9140211; http://dx.doi.org/10.1007/s004840050026
- Nishi Y, Gagge AP. Effective temperature scale useful for hypo-and hyperbaric environments. Aviat Space Environ Med 1977; 48: 97-107; PMID:871288
- Gagge AP, Stolwijk J, Hardy J. Comfort and thermal sensations and associated physiological responses at various ambient temperatures. Environ Res 1967; 1: 1-20; PMID:5614624; http://dx.doi.org/10.1016/0013-9351(67)90002-3
- Van Hoof J. Forty years of Fanger's model of thermal comfort: comfort for all? Indoor Air 2008; 18: 182-201; PMID:18363685; http://dx.doi.org/10.1111/j.1600-0668.2007.00516.x
- Havenith G. Interaction of clothing and thermoregulation. Exog Dermatol 2002; 1: 221-30; http://dx.doi.org/10.1159/000068802
- Sweeney MM, Branson DH. Sensorial comfort: part I: a psychophysical method for assessing moisture sensation in clothing. Text Res J 1990; 60: 371-7; http://dx.doi.org/10.1177/004051759006000701
- Sweeney MM, Branson DH. Sensorial comfort: part II: a magnitude estimation approach for assessing moisture sensation 1. Text Res J 1990; 60: 447-52; http://dx.doi.org/10.1177/004051759006000803
- Shelford V. A comparison of the responses of animals in gradient of environmental factors with particular reference to the method of reaction of representatives of the various groups from protozoa to mammals. Science 1918; 48: 225-30; PMID:17779482; http://dx.doi.org/10.1126/science.48.1235.225
- Kandel ER, Schwartz JH, Jessell TM. Principles of Neural Sciences. USA ET – 4th: McGraw-Hill, 2000.
- Schlader Z, Stannard S, Mündel T. Human thermoregulatory behavior during rest and exercise—a prospective review. Physiol Behav 2010; 99: 269-75; PMID:20006632; http://dx.doi.org/10.1016/j.physbeh.2009.12.003
- Parsons K. Human Thermal Environments. London, UK ET – Second: Taylor & Francis, 2003.
- Spray DC. Cutaneous temperature receptors. Annu Rev Physiol 1986; 48: 625-38; PMID:3085583; http://dx.doi.org/10.1146/annurev.ph.48.030186.003205
- Strusberg I, Mendelberg RC, Serra HA, Strusberg AM. Influence of weather conditions on rheumatic pain. J Rheumatol 2002; 29: 335-8; PMID:11838853
- Candas V, Libert J, Vogt J. Effect of hidromeiosis on sweat drippage during acclimation to humid heat. Eur J Appl Physiol Occup Physiol. 1980; 44: 123-33; PMID:7190907; http://dx.doi.org/10.1007/BF00421090
- Hirata H, Oshinsky ML. Ocular dryness excites two classes of corneal afferent neurons implicated in basal tearing in rats: involvement of transient receptor potential channels. J Neurophysiol 2012; 107: 1199-209; PMID:22114162; http://dx.doi.org/10.1152/jn.00657.2011
- Augurelle AS, Smith AM, Lejeune T, Thonnard JL. Importance of cutaneous feedback in maintaining a secure grip during manipulation of hand-held objects. J Neurophysiol 2003; 89: 665-71; PMID:12574444; http://dx.doi.org/10.1152/jn.00249.2002
- André T, Lefevre P, Thonnard J. Fingertip moisture is optimally modulated during object manipulation. J Neurophysiol 2010; 103: 402-8; http://dx.doi.org/10.1152/jn.00901.2009
- Marks L. Sensory Processes: The new Psychophysics. New York: Academic Press Inc., 1974.
- Yokohari F, Tateda H. Moist and dry hygroreceptors for relative humidity of the cockroach, Periplaneta americana L. J Comp Physiol A 1976; 106: 137-52; http://dx.doi.org/10.1007/BF00620495
- Bolanowski SJ, Gescheider GA, Fontana AM, Niemec JL, Troblay J. The effects of heat-induced pain on the detectability, discriminability, and sensation magnitude of vibrotactile stimuli. Somatosens Mot Res 2001; 18: 5-9; PMID:11327570; http://dx.doi.org/10.1080/08990220020002015
- Li Y. Perceptions of temperature, moisture and comfort in clothing during environmental transients. Ergonomics 2005; 48: 234-48; PMID:15764324; http://dx.doi.org/10.1080/0014013042000327715
- Niedermann R, Rossi R. Objective and subjective evaluation of the human thermal sensation of wet fabrics. Text Res J 2012; 82: 374-84; http://dx.doi.org/10.1177/0040517511418559
- Daanen HAM. Method and system for alerting the occurrence of wetness. EP Pat 2,110,108, 2009
- Bergmann Tiest WM, Kosters ND, Kappers AML, Daanen HAM. Phase change materials and the perception of wetness. Ergonomics 2012; 55: 508-12; PMID:22423680; http://dx.doi.org/10.1080/00140139.2011.645886
- Ackerley R, Olausson H, Wessberg J, McGlone F. Wetness perception across body sites. Neurosci Lett 2012; 522: 73-7; PMID:22710006; http://dx.doi.org/10.1016/j.neulet.2012.06.020
- Lee JY, Nakao K, Tochihara Y. Validity of perceived skin wettedness mapping to evaluate heat strain. Eur J Appl Physiol 2011; 111: 2581-91; PMID:21373868; http://dx.doi.org/10.1007/s00421-011-1882-7
- Gerrett N, Redortier B, Voelcker T, Havenith G. A comparison of galvanic skin conductance and skin wettedness as indicators of thermal discomfort during moderate and high metabolic rates. J Therm Biol 2013; 38: 530-8; http://dx.doi.org/10.1016/j.jtherbio.2013.09.003
- Chong PST, Cros DP. Technology literature review: quantitative sensory testing. Muscle Nerve 2004; 29: 734-47; PMID:15116380; http://dx.doi.org/10.1002/mus.20053
- Bergmann Tiest WM. Tactual perception of liquid material properties. Vision Res 2014; pii: S0042-6989:00179-5; PMID:25128819; http://dx.doi.org/10.1016/j.visres.2014.08.002
- Jeon E, Yoo S, Kim E. Psychophysical determination of moisture perception in high-performance shirt fabrics in relationtosweating level. Ergonomics 2011; 54: 576-86; http://dx.doi.org/10.1080/00140139.2011.582958
- Yamakawa M, Isaji S. Factors affecting the clamminess. J Text Mach Soc Japan 1987; 33: 9-15.
- Filingeri D, Redortier B, Hodder S, Havenith G. The role of decreasing contact temperatures and skin cooling in the perception of skin wetness. Neurosci Lett 2013; 551: 65-9; PMID:23886487; http://dx.doi.org/10.1016/j.neulet.2013.07.015
- Filingeri D, Redortier B, Hodder S, Havenith G. Warm temperature stimulus suppresses the perception of skin wetness during initial contact with a wet surface. Skin Res Technol 2015; 21:9-14; PMID:24612108; http://dx.doi.org/10.1111/srt.12148
- Yoshioka T, Craig JC, Beck GC, Hsiao SS. Perceptual constancy of texture roughness in the tactile system. J Neurosci 2011; 31: 17603-11; PMID:22131421; http://dx.doi.org/10.1523/JNEUROSCI.3907-11.2011
- Gwosdow A R, Stevens JC, Berglund LG, Stolwijk JAJ. Skin friction and fabric sensations in neutral and warm environments. Text Res J 1986; 56: 574-80; http://dx.doi.org/10.1177/004051758605600909
- Filingeri D, Redortier B, Hodder S, Havenith G. Thermal and tactile interactions in the perception of local skin wetness at rest and during exercise in thermo-neutral and warm environments. Neuroscience 2014; 258: 121-30; PMID:24269934; http://dx.doi.org/10.1016/j.neuroscience.2013.11.019
- Green BG, Schoen KL. Evidence that tactile stimulation inhibits nociceptive sensations produced by innocuous contact cooling. Behav Brain Res 2005; 162: 90-8; PMID:15922069; http://dx.doi.org/10.1016/j.bbr.2005.03.015
- Shibasaki M, Sakai M, Oda M, Crandall CG. Muscle mechanoreceptor modulation of sweat rate during recovery from moderate exercise. J Appl Physiol (1985) 2004; 96: 2115-9; PMID:14766775; http://dx.doi.org/10.1152/japplphysiol.01370.2003
- McGlone F, Reilly D. The cutaneous sensory system. Neurosci Biobehav Rev 2010; 34: 148-59; PMID:19712693; http://dx.doi.org/10.1016/j.neubiorev.2009.08.004
- Mancini F, Bauleo A, Cole J, Lui F, Porro CA, Haggard P, Iannetti GD. Whole-body mapping of spatial acuity for pain and touch. Ann Neurol 2014; 75: 917-24; PMID:24816757; http://dx.doi.org/10.1002/ana.24179
- Ouzzahra Y, Havenith G, Redortier B. Regional distribution of thermal sensitivity to cold at rest and during mild exercise in males. J Therm Biol 2012; 37: 517-23; http://dx.doi.org/10.1016/j.jtherbio.2012.06.003
- Gerrett N, Ouzzahra Y, Coleby S, Hobbs S, Redortier B, Voelcker T, Havenith G. Thermal sensitivity to warmth during rest and exercise: a sex comparison. Eur J Appl Physiol 2014; 14: 1451-62; http://dx.doi.org/10.1007/s00421-014-2875-0
- Filingeri D, Fournet D, Hodder S, Havenith G. Body mapping of cutaneous wetness perception across the human torso during thermo-neutral and warm environmental exposures. J Appl Physiol 2014; 117:887-97; PMID:25103965; http://dx.doi.org/10.1152/japplphysiol.00535.2014
- Zotterman Y. Thermal sensation. In: Handbook of Physiology, vol. 1, Neurophysiology. Washington, D.C.: American Physiological Society, 1959: 431-58.
- Hensel H. Thermoreception and Temperature Regulation. London: Academic Press Inc. Ltd, 1981.
- Darian-Smith I. Thermal Sensibility. Compr Physiol 2011, Supplement 3: Handbook of Physiology, The Nervous System, Sensory Processes: 879-913. First published in print 1984; http://dx.doi.org/10.1002/cphy.cp010319
- Nakamura M, Yoda T, Crawshaw L, Yasuhara S, Saito Y, Kasuga M, Nagashima K, Kanosue K. Regional differences in temperature sensation and thermal comfort in humans. J Appl Physiol (1985) 2008; 105: 1897-906; PMID:18845785; http://dx.doi.org/10.1152/japplphysiol.90466.2008
- Nakamura M, Yoda T, Crawshaw LI, Kasuga M, Uchida Y, Tokizawa K, Nagashima K, Kanosue K. Relative importance of different surface regions for thermal comfort in humans. Eur J Appl Physiol 2013; 113: 63-76; PMID:22569893; http://dx.doi.org/10.1007/s00421-012-2406-9
- Schepers R, Ringkamp M. Thermoreceptors and thermosensitive afferents. Neurosci Biobehav Rev 2010; 34: 177-84; PMID:19822171; http://dx.doi.org/10.1016/j.neubiorev.2009.10.003
- Burke W, Mekjavic I. Estimation of regional cutaneous cold sensitivity by analysis of the gasping response. J Appl Physiol (1985) 1991; 71: 1933-40; PMID:1761494
- Keatinge W, Nadel J. Immediate respiratory response to sudden cooling of the skin. J Appl Physiol 1965; 20: 65-9; PMID:14257563
- Ackerley R, Carlsson I, Wester H, Olausson H, Backlund Wasling H. Touch perceptions across skin sites: differences between sensitivity, direction discrimination and pleasantness. Front Behav Neurosc 2014; 8: 54.
- Norrsell U, Finger S, Lajonchere C. Cutaneous sensory spots and the “law of specific nerve energies”: history and development of ideas. Brain Res Bull 1999; 48: 457-65; PMID:10372506; http://dx.doi.org/10.1016/S0361-9230(98)00067-7
- Abraira VE, Ginty DD. The sensory neurons of touch. Neuron 2013; 79: 618-39; PMID:23972592; http://dx.doi.org/10.1016/j.neuron.2013.07.051
- Rushmer RF, Buettner KJK, Short JM, Odland GF. The skin. Science 1966; 154: 343-8; PMID:5917085; http://dx.doi.org/10.1126/science.154.3747.343
- Campero M, Bostock H. Unmyelinated afferents in human skin and their responsiveness to low temperature. Neurosci Lett 2010; 470: 188-92; PMID:19576956; http://dx.doi.org/10.1016/j.neulet.2009.06.089
- Craig AD, Chen K, Bandy D, Reiman EM. Thermosensory activation of insular cortex. Nat Neurosci 2000; 3: 184-90; PMID:10649575; http://dx.doi.org/10.1038/72131
- Campero M, Serra J, Bostock H, Ochoa JL. Slowly conducting afferents activated by innocuous low temperature in human skin. J Physiol 2001; 535: 855-65; PMID:11559780; http://dx.doi.org/10.1111/j.1469-7793.2001.t01-1-00855.x
- McGlone F, Wessberg J, Olausson H. Discriminative and affective touch: sensing and feeling. Neuron 2014; 82: 737-55; PMID:24853935; http://dx.doi.org/10.1016/j.neuron.2014.05.001
- Reid G. ThermoTRP channels and cold sensing: what are they really up to? Pflugers Arch 2005; 451: 250-63; PMID:16075243; http://dx.doi.org/10.1007/s00424-005-1437-z
- Tominaga M, Caterina M. Thermosensation and pain. J Neurobiol 2004; 61: 3-12; PMID:15362149; http://dx.doi.org/10.1002/neu.20079
- Romanovsky AA. Thermoregulation: some concepts have changed. Functional architecture of the thermoregulatory system. Am J Physiol Regul Integr Comp Physiol 2007; 292: R37-46; PMID:17008453; http://dx.doi.org/10.1152/ajpregu.00668.2006
- Tsunozaki M, Bautista DM. Mammalian somatosensory mechanotransduction. Curr Opin Neurobiol 2009; 19: 362-9; PMID:19683913; http://dx.doi.org/10.1016/j.conb.2009.07.008
- Lumpkin EA, Caterina MJ. Mechanisms of sensory transduction in the skin. Nature 2007; 445: 858-65; PMID:17314972; http://dx.doi.org/10.1038/nature05662
- Adams MJ, Johnson SA, Lefèvre P, Lévesque V, Hayward V, André T, Thonnard JL. Finger pad friction and its role in grip and touch. J R Soc Interface 2013; 10(80):20120467
- Pleger B, Villringer A. The human somatosensory system: from perception to decision making. Prog Neurobiol 2013; 103: 76-97; PMID:23123624; http://dx.doi.org/10.1016/j.pneurobio.2012.10.002
- Haggard P, Iannetti GD, Longo MR. Spatial sensory organization and body representation in pain perception. Curr Biol 2013; 23: R164-76; PMID:23428330; http://dx.doi.org/10.1016/j.cub.2013.01.047
- Yarnitsky D, Ochoa JL. Release of cold-induced burning pain by block of cold-specific afferent input. Brain 1990; 113: 893-902; PMID:2397391; http://dx.doi.org/10.1093/brain/113.4.893
- Davis KD. Cold-induced pain and prickle in the glabrous and hairy skin. Pain 1998; 75: 47-57; PMID:9539673; http://dx.doi.org/10.1016/S0304-3959(97)00203-0
- Torebjörk HE, Hallin RG. Perceptual changes accompanying controlled preferential blocking of A and C fibre responses in intact human skin nerves. Exp Brain Res 1973; 16: 321-32; PMID:4686614
- Geisler WS, Kersten D. Illusions, perception and Bayes. Nat Neurosci 2002; 5: 508-10; PMID:12037517; http://dx.doi.org/10.1038/nn0602-508
- Knill D, Richards W. Perception as Bayesian inference. New York: Cambridge Univ. Press, 1996.
- Lochmann T, Deneve S. Neural processing as causal inference. Curr Opin Neurobiol 2011; 21: 774-81; PMID:21742484; http://dx.doi.org/10.1016/j.conb.2011.05.018
- Weiss Y, Simoncelli EP, Adelson EH. Motion illusions as optimal percepts. Nat Neurosci 2002; 5: 598-604; PMID:12021763; http://dx.doi.org/10.1038/nn0602-858
- Ernst MO, Banks MS. Humans integrate visual and haptic information in a statistically optimal fashion. Nature 2002; 415: 429-33; PMID:11807554; http://dx.doi.org/10.1038/415429a
- Tommerdahl M, Favorov OV, Whitsel BL. Dynamic representations of the somatosensory cortex. Neurosci Biobehav Rev 2010; 34: 160-70; PMID:19732790; http://dx.doi.org/10.1016/j.neubiorev.2009.08.009
- Saal HP, Bensmaia SJ. Touch is a team effort: interplay of submodalities in cutaneous sensibility. Trends Neurosci 2014; 37: 689-97; PMID:25257208; http://dx.doi.org/10.1016/j.tins.2014.08.012
- Russell J, Vidal-Gadea AG, Makay A, Lanam C, Pierce-Shimomura JT. Humidity sensation requires both mechanosensory and thermosensory pathways in Caenorhabditis elegans. Proc Natl Acad Sci USA 2014; 111: 8269-74; PMID:24843133; http://dx.doi.org/10.1073/pnas.1322512111
- Filingeri D. Humidity sensation, cockroaches, worms and humans: are common sensory mechanisms for hygrosensation shared across species? J Neurophysiol 2014; http://dx.doi.org/10.1152/jn.00730.2014
- Vriens J, Nilius B, Voets T. Peripheral thermosensation in mammals. Nat Rev Neurosci 2014; 15: 573-89; PMID:25053448; http://dx.doi.org/10.1038/nrn3784