Abstract
Effects of reducing body mass on body core temperature and locomotor activity of mice originally kept on conventional rodent diet (Group-1) were compared to those made obese by feeding them a high-fat diet (Group-2), both groups being kept at a cool ambient temperature. Based on earlier experience, threshold torpor core temperature of 31° was chosen as the endpoint to decreasing body mass. It was hypothesized that the onset of this hypothermia develops in obese mice only when their body mass approaches a similar low body mass as in lean mice. Mice in Group-1 maintained nocturnal core temperature but developed marked daytime hypothermia of 30–31°C with their body mass approaching 20 g by this time. Mice in Group-2 could maintain normal circadian temperature rhythm for 3 weeks before similar daytime hypothermia started to develop while their body mass dropped also to about 20 g. Mice belonging to Group-1 or Group-2 could regain original body mass after re-feeding with the original diet within 2 days or 5 weeks, respectively. In the course of the development of daily torpor, nighttime normothermia was accompanied by progressive increases in locomotor activity in both groups of mice. It is concluded that in mice a marked fall of daytime body core temperature is only induced when a similar low critical body mass is reached, irrespective of the initial body mass. In other words, in both groups of mice the nutritional state determines the threshold for the thermoregulatory change during torpor.
Abbreviations
ANOVA | = | analysis of variance, AU, arbitrary unit, f, fasting days or weeks, rf, refeeding days or weeks, I.P, intraperitoneal, SEM, standard error of mean, Tc, core temperature, Tc max, maximal core temperature, Tc min, minimal core temperature, Tc mean, average core temperature |
Introduction
Obesity has an ever increasing prevalence worldwide and is known to result from a combination of increased energy intake and decreased energy utilization. As a special nutritional example, free availability of high-fat diet can characteristically speed up accumulation of energy reserves (fat tissue) both in humansCitation1 and animals.Citation2 Conversely, in extreme cases of morbid obesity restriction of food intake or even fasting may be the only method of choice for achieving rapid fall of body mass, a practice obviously full of health risks.Citation3,4,5 Signs and symptoms of imminent functional breakdown or even fatal outcome in the course of severe food restriction have been sought both in human clinical practiceCitation6 and in animal studies on obesity to make body mass reducing procedures safe.Citation7
The literature abounds with information about effects of fasting on factors of energetics other than body mass such as body core temperature and spontaneous locomotor activity in normal mice.Citation8,9,10,11 In several other homeotherm species, such as pigeons and rats, it has been observed that body temperature of food-deprived animals becomes progressively lower on the consecutive days in the inactive phase of the day, whereas body core temperature remains normal in the active phase.Citation12,13 During fasting, small endotherms may employ daily torpor, a suppression of metabolic rate to as low as 30% of basal metabolic rate together with a fall of core temperature lasting for a period of several hours.Citation14 These animals also called daily heterotherms tend to be small, typically 5–50 g, and include hamsters, mice, shrews, gerbils, hummingbirds, numerous marsupials and many others.Citation15
As opposed to the wealth of information on thermoregulatory effects of fasting (not longer than 3 days) in mice fed a conventional rodent diet and having normal body mass, similar studies in mice made obese either by an energy-rich diet or by genetic manipulation have not been reported so far. In a recent paper regular development of daily torpor has been described in ob/ob mice exposed either to a stable cool ambient temperature or to short-lasting fasting.Citation15 As far as the long-term effects of fasting in obese mice are concerned, only one study has been published several decades agoCitation16 in which detailed metabolic analysis of ob/ob mice subjected to 2 weeks of total fasting was carried out, but body temperature was not recorded.
It appeared therefore worthwhile to study thermoregulatory effects of fasting in mice made obese by a fat-rich diet and to compare the effects to those of lean control mice. Our main goal was to find out whether obese mice also respond to fasting with a similar change of body core temperature as was observed in lean control mice. Another aim was to find a simple non-invasive parameter, which can safely indicate the necessity to terminate fasting and initiate refeeding. It was hypothesized that marked daytime hypothermia develops in obese mice only when body mass approaches a low value similar to that observed in lean mice.
Materials and Methods
We studied 2 groups (n = 6/group) of C57BL/6 male mice (Charles River Hungary) with an age range of 5- to 6 months at the start of fasting held individually in plastic cages with wood shavings at a subtermoneutral temperature of 24–25°C, that is, below thermoneutrality allowing the development of torpor in mice.Citation15 A 12/12 hour light/darkness schedule was used, light starting at 6 a.m.
In one group (Group-1) normal laboratory chow (CRLT/N, Charles River Kft, Budapest, Hungary) was supplied ad libitum followed by a period of 3 days without food when only tap water was provided until re-feeding occurred with the same normal chow. Mice of the other group (Group-2) were fed ad libitum with a fat-rich diet containing 60 per cent energy from fat (TestDiet 58Y1, IPS Product Supplies Ltd., London, UK) until body mass increased above 50 g after 3 months. These mice were also exposed to lack of food with free access to water until daytime body core temperature gradually approached 31°C, when re-feeding was started with the same fat-rich diet, since earlier experienceCitation17,18 suggested that lack of food can be well tolerated by non-obese mice as long as daytime body core temperature approaches a value of 30–31°C. As observed in the same experiments, below that core temperature even the night core temperature maxima would markedly decline. In other words, by choosing an hourly average core temperature threshold of 31°C, hypothermia was well within the safe range allowing effective rewarming in both groups of mice during refeeding. Additionally, this value represents the upper threshold of the torpor body temperature range (17–31°C) for mice.Citation19 All mice were habituated to daily body mass measurements.
One week before the experiments, each of the mice was intra-abdominally implanted with a biotelemetry transmitter (Minimitter-VMFH, Series 4000, Sunriver, OR) under intraperitoneal (I.P.) ketamine/xylazine anesthesia (78 mg/13 mg kg-1) for continuous monitoring core temperature and locomotor activity allowing 3 days for recovery (following which, normal circadian rhythm appears) and a 4-day baseline monitoring. After the implantation of the biotelemetry transmitter 0.02 mg gentamycin was given I.P. for preventing infection. Sampling abdominal temperature and activity data was set to 5 minutes throughout the experiments. For further data sampling and analysis the Vital View software supplied by the manufacturer was used. Daily excursions of core temperature were determined from differences between night maxima and day minima using 1-hour averages of core temperature. Daily mean core temperature was determined after 24-hour averaging.
The experiments were done according to the general rules set in the Hungarian law on animals and the experimental protocols used were approved by the Ethical Committee of Pécs University (BA02/2000-13/2006) with the implicit limitation that as a result of food restriction nadir body mass should not be lower than that developing after 2 to 3 days of fasting in mice kept on normal laboratory chow. During this period, all mice were closely monitored regarding their body weight, core body temperature, activity levels and behavioral changes. As soon as the mean hourly core temperature reached 31°C, food was immediately returned. Water was freely available and water consumption was regularly checked. The 3-R principle was carefully followed.
For statistical analysis one-way analysis of variance (ANOVA) test with Scheffe's post hoc test, repeated measures ANOVA tests and regression analyses (with piecewise linear regression) were used. SPSS Statistics for Windows 11.0 and SigmaPlot for Windows 11.0 were applied. The differences were regarded statistically significant at the level of P < 0.05.
Results
In Group-1 mice fasting resulted in a fall of body mass from 24.3 ± 0.2 to 19.7 ± 0.3 g within 3 days with a return to the pre-fasting body mass after the first re-feeding day (23.7 ± 0.5) (left panel of ). As shown in the right panel of , fasting led to a linear fall of body mass Group-2 mice starting from 54.7 ± 1.8 g and reaching 20.0 ± 0.4 g (by the 27th day, on the average) together with a decrease of daytime core temperature to about 30–31°C. In other words, these animals lost 64 per cent of their original body mass. Re-feeding reversed body mass of Group-2 with a more rapid increase during the first week and by the end of the second re-feeding week the body mass doubled (40.3 ± 2.6) followed by a less rapid rise leading to the original obese values by the end of the fifth week (53.0 ± 4.6) (rf1 to rf5 in ).
Figure 1. Body mass of control mice (Group-1, left panel) and obese mice (Group-2, right panel) before, during fasting (f1-3 or f1-5) and after (rf1-5) fasting lasting for 3 d or 5 weeks, respectively(f – days or weeks of fasting, rf – days or weeks of refeeding). Mean ± SEM are shown.
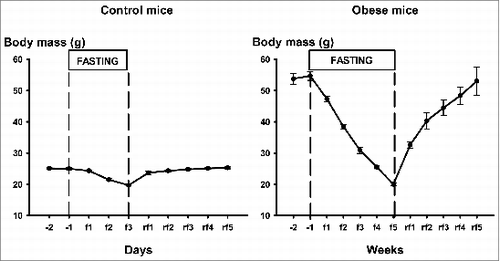
In Group-2 mice, over the first 3 weeks of fasting, body core temperature was modified only slightly with decrease during the day, while at night core temperature was maintained at 38°C (see typical experimental recording in the ). It was only during the last week of fasting when daytime core temperature started to decline more progressively on consecutive days reaching one-hour average values lower than 31°C. Re-feeding led to a rapid rise in core temperature together with a decrease of daily body temperature oscillations. In contrast to body mass requiring some 4 weeks for normalization to the previous obese levels (right panel of ), the normalization of daily rhythm of the core temperature occurred within the first 5 days of re-feeding (right panel of ).
Figure 2. Daily body core temperature (Tc) of control mice (Group-1, left panel) and obese mice (Group-2, right panel) before, during and after fasting lasting for 3 days or for 26 days, respectively. Single mouse experiments (one-hour averages) are shown.
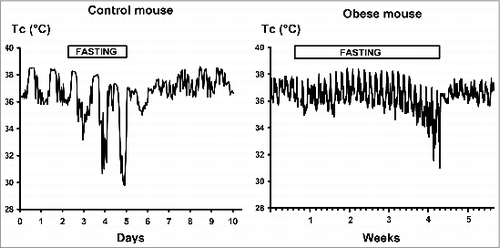
In Group-2 mice average daily excursion of core temperature (mean ± standard error of mean, SEM) was 1.5 ± 0.1°C before fasting and remained similar (day minima, night maxima and mean core temperature values measured on days 2, 5 and 10 of fasting are shown in upper panel) by the 20th day of fasting on the average, i.e. up to 7 days before refeeding. From this time on (day -7 in , upper panel), progressive fall of core temperature [day minima (P < 0.001) and mean core temperature (p = 0.001) started (one-way ANOVA). Therefore, daily excursions of core temperature started to rise significantly and reached a value of 4.1 ± 0.4°C on the last day of fasting (day -1 in lower panel). On the last day of fasting even the night core temperature maxima started to decline (similarly as in controls, ). Re-feeding led to a rapid increase of day minima resulting in significant decrease of daily body temperature excursions to 0.4 ± 0.1°C and 0.5 ± 0.1°C (day 1 and day 2 of re-feeding, respectively) with a return to the control value by day 5 of re-feeding (1.5 ± 0.3°C).
Figure 3. Upper panel: Night-time maximal (Tc max), daytime minimal (Tc min) and daily average core temperature (Tc mean) in obese mice (Group-2) before fasting, during the first days (2, 5, 10) of fasting, during the last week of fasting (7 and 1 day before re-feeding) and on 1st and 5th days of re-feeding. Lower panel: Daily excursions of core temperature were determined from differences between night maxima and day minima using one-hour averages of core temperature. Asterisks show significant differences between the data sets indicated (**P < 0.01, ***P < 0.001, one-way ANOVA). All mice were fed on a fat-rich diet before and after fasting. Mean ± SEM are shown.
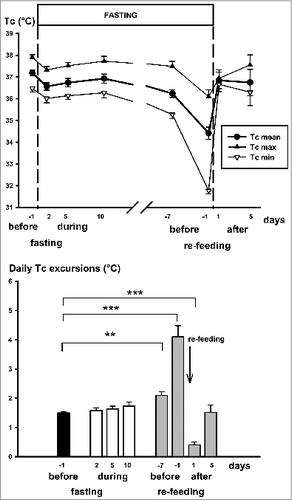
As shown in , the change in daytime minimum core temperature as a function of body mass in Group-1 mice was nearly linear (y = 0.81x + 15.14; coefficient of correlation: 0.94, for the regression line: t = 8.85 P < 0.001). However, in Group-2 mice the regression line has a break and a transition point at 36.0 ± 0.2°C and 29.5 ± 0.7 g (piecewise linear regression). Over the body mass of 29.5 g (i.e., during the first 2 to 3 weeks) there is no relation between daytime minimum core temperature and body mass, but under that body mass level a linear correlation has been found between these parameters (y = 0.50x + 21.15; coefficient of correlation: 0.89, for the regression line: t = 13.75 P < 0.001).
Figure 4. Daytime minimum core temperature (2-hour averages) (Tc min) as a function of body mass in obese (Group-2, filled symbols) and control (Group-1) mice (open symbols) during fasting. Measurements on the same animals were performed on different fasting days. Regression lines for control group (thin) and obese animals (thick) are indicated. For details of statistics see Results.
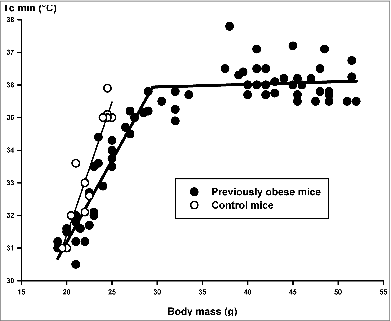
Concerning the slopes of the regression lines of the 2 animal groups over a body weight range of 29.5–19.0 g, we found a statistically significant difference (95% confidence interval for Group-1: 0.611 and 1.045 and for Group-2: 0.427 and 0.576). In other words, both in normal and previously obese mice the fall of daytime core temperature to 30–31°C corresponded to a body mass of about 20 g.
Recordings of locomotor activity from 2 individual experiments are shown in (3-day-long fasting of Group-1, in left panel and 27-day-long fasting of Group-2, in right panel). Nighttime locomotor activity of the Group-1 mice showed a gradual rise within the 3 days of fasting followed by a sudden fall on re-feeding. At the same time, daytime activity values did not change. Mean daytime and nighttime activity values of Group-1 mice were similar to those previously reported earlier by the same laboratory.Citation17 In Group-2 mice, daytime activity values did not change, while a gradual rise in nighttime activity could be seen, again with a reduction in activity after re-feeding. Because of the individual differences in their raw initial activity values, percentage changes of nighttime locomotor activity values were evaluated for each Group-1 mouse (). Rise in their locomotor activity was significant in the course of fasting [repeated measures ANOVA, within subject test: F (4,24) = 8.170; P < 0.001]. On the first day after re-feeding the mean value of nighttime activity returned to the pre-fasting level (103.9 ± 2.4% of the pre-fasting control value, not different from the control day).
Figure 5. Locomotor activity in a lean mouse (left panel) and in an obese mouse (right panel). See progressive rises of activity during fasting and rapid decrease on re-feeding both in the obese mouse and in the control mouse. AU = arbitrary units. Two-hour averages are shown. For details of statistics see Results.
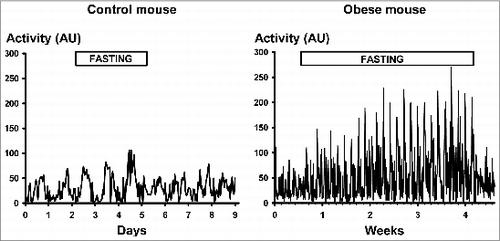
Table 1. Values of spontaneous night-time locomotor activity (12-h averages) in obese mice (Group-2) during the first days (2, 5, 10) of fasting, 7 days before re-feeding and on the first day after re-feeding (values are shown as percentage of the last pre-fasting control day values). For details of statistics see Results
Discussion
Fasting as a way of reducing grossly obese body mass of humans has been applied since the 60s of the past centuryCitation5,20 and was also tested in ratsCitation21 and in miceCitation16 to measure changes of body composition. In earlier studies carried out either in humans or animals, no clear threshold symptom or physiological parameter to stop fasting before irreversible pathological changes occurred had been defined, although limitations such as the size of remaining protein pool for gluconeogenesis or adverse changes in different plasma electrolytes affecting cardiac rhythmicity have long been known.Citation22 As an alternative sign of severe depletion of energy stores for basic life processes, hypothermia has also been observed in our previous studies carried out during fasting in mice previously fed with normal chow.Citation17,18 It was therefore worthwhile to apply biotelemetry also in obese mice to follow the time-course of changes in body core temperature during fasting to see if there was any major difference in the appearance of hypothermia.
Under natural conditions, small rodents have been known to enter torpidity or daily hypothermia when food sources are severely limited and/or ambient temperature decreases.Citation14,23,24 Metabolic response to short-term (24 hours) fasting of mice with diet-inducedCitation25 has been published without data on body temperature. Information on body temperature response to short-term fasting appeared only in mice with genetic obesity.Citation26 Still, no relevant data have been published so far to compare the effects of fasting on body mass, core temperature and locomotor activity in obese mice to those in normal mice. The use of biotelemetry in the present study furnished some evidence for the close link between the loss of body mass and daytime hypothermia, the latter being utilized as an indicator. In fact, body core temperature just a couple of °C higher than ambient temperature (30–31°C vs. 24–25°C) is still around the safe low level, below which spontaneous re-warming seems to be difficult during severe loss of body mass.Citation27,28
As demonstrated by the present data, Group-2 mice previously kept on a fat-rich diet, could tolerate fasting with their daytime body temperature reaching 31°C, a value also observed in mice previously kept on conventional chow and exposed to fasting. It should be emphasized that no attempt has been made in the present study to investigate the survival of mice, in other words, not even one mouse was lost as a result of fasting. In fact, experience gained from earlier studies served as a safeguard to ensure complete recovery of the animals in the present study by carefully monitoring core temperature with biotelemetry and hence interrupting fasting if daytime core temperature approached a threshold value of 31°C.Citation17,18
In our present study, in both groups of mice, locomotor activity increased at night with day values remaining about the same during fasting, a widely known phenomenon characteristic of both rats and mice kept on normal dietCitation16,29,30 and obese rats.Citation31 As to the mechanism of nighttime normothermia induced by increased locomotor activity, a myokine factor, irisin can be mentioned that has been shown to be produced by high skeletal muscle activity and may lead to increased release of energy supplying substrates from fat tissue.Citation32
As opposed to the Group-1 mice able to withstand only 2 to 3 days of fasting with daily core temperature oscillations increasing from the first day onwards, Group-2 mice showed normal daily body temperature oscillation for 2 to 3 weeks of fasting in the present study. It was only during the last week of fasting, when daytime body temperature approached values just below 31°C, while nighttime core temperature was still maintained around normothermia.
The authors of the present study are not aware of any study carried out so far in mice with diet-induced or any other types of obesity, in which body mass decreased as much as in the present study (by 64 per cent) and the animals survived after 4 weeks of fasting and re-feeding with the final body mass ending up the original obese value measured at the beginning of the experiments. It is most likely that a hormonal signal such as leptin in fed state and its fall in fasting could be involved in the alterations of daily body temperature oscillations.Citation15,33 In fact, if leptin level decreased in the present studies only by about the third week of fasting, it possibly could explain the development of gradual daytime metabolic depression, hypothermia and torpor. In contrast to the probably hyperleptinemic obese mice of the present study, in leptin-deficient ob/ob mice food deprivation can induce daily torpor shortly after the start of fasting; moreover, obese mice become more hypothermic than the lean sex-matched controls.Citation8 It has not been clear so far if the fall of the whole body mass or the fall of fat tissue mass could be the regulatory factor serving as a signal to allow progressive hypothermia. Conversely, metabolically active tissue mass decreasing to some critically low level could limit the availability of substrate(s) needed as fuel for brain energy metabolisms which finally may lead to severe hypothermia.
It is concluded that during fasting, a fall in daytime body core temperature is only induced when a low critical body mass is reached, irrespective of the time of fasting and of the initial pre-fasting body weight. Fall in daytime minimum body core temperature is probably determined by the remaining body mass. The results of the present study suggest that in mice not fasting itself, but its effect on body mass may induce torpor or daytime hypothermia. In other words, although diet-induced obesity in mice changed the course and prolonged the duration of our experimental intervention, it does not seem to influence the body mass for the critical thermoregulatory change that is similar to that observed in the control group. The gradual and progressive fall of daytime core temperature during the late phases of fasting both in control and obese mice in the present study may be utilized in experiments where cold exposure and/or fasting presents a challenge to thermal homeostasis and could even present a threat to survival. The relative ease of continuous biotelemetric monitoring of body core temperature of mice possessing a small body mass is also the method of choice when pharmacological actions of substances affecting body energetics are tested.Citation34
Perspectives
The presented data suggest that in the course of fasting, the falling body mass and possibly the decreasing fat content are responsible for the initiation of progressive daytime hypothermia. Determination of body composition and fat ratio at different phases of fasting seems to be of utmost importance. In the regulation of energy balance (including metabolic rate and body temperature) the most significant known signals of body mass and composition are leptin and insulin. Therefore, the plasma levels of these peptides and their metabolic responsiveness to exogenous administration should be monitored both during fasting in control mice and in diet-induced obese animals. Plasma fatty acids, glucose, and ketone bodies, as well as respiratory exchange ratio are also to be measured in the course of fasting, together with indicators of gluconeogenesis.
Disclosure of Potential Conflicts of Interest
No potential conflicts of interest were disclosed.
Acknowledgments
The expert technical help by Ms. A. Jech-Mihálffy and Ms M. Koncsecskó-Gáspár and statistical analysis by Ms. K. Farkas-Borbás is gratefully acknowledged. We thank Prof. M. Székely and Prof. G. Rébék-Nagy for critically reading this manuscript.
Funding
This work was supported by Hungarian national grants: Hungarian Ministry of Health (ETT 6003/1/2001), Ministry of Education (OM PhD-School), Hungarian Scientific Research Funds (OTKA T62598 and PD84241).
References
- Ravussin E, Tataranni PA. Dietary Fat and human obesity. J Amer Diet Assoc 1997; 97:S42-6; PMID: 9216566; http://dx.doi.org/10.1016/S0002-8223(97)00728-1 potential conflicts of interest were disclosed.
- Surwit RS, Feinglos MN, Rodin J, Sutherland A, Petro AE, Opara EC, Kuhn CM, Rebuffe-Scrivé M. Differential effects of fat and sucrose on the development of obesity and diabetes in C57BL/6J and A/J mice. Metabolism 1995; 44:645-51; PMID:7752914; http://dx.doi.org/10.1016/0026-0495(95)90123-Xn
- Thomson TJ, Runcie J, Miller V. Treatment of obesity by total fasting for up to 249 days. Lancet 1966; 288:992-6; PMID:4162688; http://dx.doi.org/10.1016/S0140-6736(66)92925-4
- Michalsen A. Prolonged fasting as a method of mood enhancement in chronic pain syndromes: a review of clinical evidence and mechanisms. Curr Pain Headache Rep 2010; 14:80-7; PMID:20425196; http://dx.doi.org/10.1007/s11916-010-0104-z
- Johnston AM. Fasting - the ultimate diet? Obes Rev 2007; 8:211-22; PMID:17444963; DOI: 10.1111/j.1467-789X.2006.00266.x
- Gilliand IC. Total fasting in the treatment of obesity. Postgrad Med 1968; 44:58-61; PMID:5639230; http://dx.doi.org/10.1136/pgmj.44.507.58
- Garruti G, De Pergola G, Cignarelli M, Marangelli V, Santoro G, Triggiani V, Ciampolillo A, Giorgino R. 34-day total fast in adult man. Int J Obes Relat Metab Disord 1995; 19:46-9; PMID:7719390
- Dubuc PU, Wilden NJ, Carlislea HJ. Fed and fasting thermoregulation in ob/ob mice. Ann Nutr Metab 1985; 29:358-65; PMID:4062246; http://dx.doi.org/10.1159/000176992
- Williams TD, Chambers JB, Henderson RP, Rashoutte ME, Overton JM. Cardiovascular responses to caloric restriction and thermoregulation in C57BL66J mice. Am J Physiol 2002; 282:R1459-67; PMID:15234180; http://dx.doi.org/10.1152/ajpregu.00612.2001
- Overton JM, Williams TD. Behavioral and physiologic responses to caloric restriction in mice. Physiol Behav 2004; 81:749-54; PMID:15234180; http://dx.doi.org/10.1016/j.physbeh.2004.04.025
- Swoap JS, Gutilla MJ. Cardiovascular changes during daily torpor in the laboratory mouse. Am J Physiol 2009; 297:R769-74; PMID:19587115; http://dx.doi.org/10.1152/ajpregu.00131.2009
- Graf R. Regulated nocturnal hypothermia induced in pigeons by food-deprivation. Am J Physiol 1989; 256:R733-8; PMID:2923260
- Yoda T, Crawshaw LI, Yoshida K, Su L, Hosono T, Shido O, Sakurada S, Fukuda Y, Kanosue K. Effects of food deprivation on daily changes in body temperature and behavioral thermoregulation in rats. Am J Physiol 2000; 278:R134-9; PMID:10644631
- Geiser F. Metabolic and body temperature reduction during hibernation and daily torpor. Annu Rev Physiol 2004; 66:239-27; PMID:14977403; http://dx.doi.org/10.1146/annurev.physiol.66.032102.115105
- Swoap JS. The pharmacology and molecular mechanisms underlying temperature regulation and torpor. Biochem Pharmacol 2008; 76:817-24; PMID:18644349; http://dx.doi.org/10.1016/j.bcp.2008.06.017
- Cuendet GS, Loten EG, Cameron DP, Renold AE, Marliss EB. Hormone-substrate responses to total fasting in lean and obese mice. Am J Physiol 1975; 228: 276-83; PMID:1096642
- Kanizsai P, Garami A, Solymár M, Szolcsányi J, Szelényi Z. Energetics of fasting heterothermia in TRPV1-KO and wild type mice. Physiol Behav 2009; 96:149-54; PMID:18938188; http://dx.doi.org/10.1016/j.physbeh.2008.09.023
- Solymár M, Kanizsai P, Pétervári E, Garami A, Szelényi Z. Mechanism of fasting heterothermia and re-feeding normothermia in mice. J Therm Biol 2010; 35:280-3; http://dx.doi.org/10.1016/j.jtherbio.2010.06.003
- Swoap SJ, Gutilla MJ, Liles LC, Smith RO, Weinshenker D. The full expression of fasting-induced torpor requires β3-adrenergic receptor signaling. J Neurosci 2006; 26:241-5; PMID:16399693; doi: 10.1523/JNEUROSCI.3721-05.2006
- Bray GA, Davidson MB, Drenick EJ. Obesity: a serious symptom. Ann Int Med 1972; 77:779-95; PMID:4562970; http://dx.doi.org/10.7326/0003-4819-77-5-779
- Babirak S, Dowell RT, Oscai LB. Total fasting and total fasting plus exercise effects on body composition of the rat. J Nutr 1974; 104:452-7; PMID:4816930;
- Cahill GF Jr, Owen OE. Starvation and survival. Trans Am Climatol Assoc 1968; 79:13-20; PMID:5667163
- Hudson JW, Scott JM. Daily torpor in the laboratory mouse, mus musculus, var. albino. Physiol Zool 1979; 52:205-18; http://www.jstor.org/stable/30152564
- Himms-Hagen J. Food restriction increases torpor and improves brown adipose tissue thermogenesis in ob/ob mice. Am J Physiol 1985; 248:E531-9; PMID:4039539
- Ueno N, Asakawa A, Inui A. Blunted metabolic response to fasting in obese mice. Endocrine 2007; 32:192-6; PMID:1797546; http://dx.doi.org/10.1007/s12020-007-9016-z
- Gavrilova O, Leon LR, Marcus-Samuels B, Mason MM, Castle AL, Vinson C, Reitman ML. Torpor in mice is induced by both leptin-dependent and – independent mechanisms. Proc Natl Acad Sci USA 1999; 96:14623-8; PMID:10588755; http://dx.doi.org/10.1073/pnas.96.25.14623
- Webb GP, Jagot SA, Jakobson ME. Fasting-induced torpor in mus musculus and its implications in the use of murine models for human obesity studies. Comp Biochem Physiol A Comp Physiol 1982; 72:211-9; PMID:6124358
- Swoap SJ, Rathvon M, Gutilla M. AMP does not induce torpor. Am J Physiol 2007; 293:R468-73; PMID:17409259; http://dx.doi.org/10.1152/ajpregu.00888.2006
- Koubi HE, Robin JP, Dewasmes G, Le Maho Y, Frutoso J, Minaire Y. Fasting-induced rise in locomotor activity in rats coincides with increased protein utilization. Physiol Behav 1991; 50:337-43; PMID:1745678
- Sakurada S, Shido O, Sugimoto N, Hiratsuka Y, Yoda T, Kanosue K. Autonomic and behavioural thermoregulation in starved rats. J Physiol (Lond) 2000; 526:417-24; PMID:10896730; http://dx.doi.org/10.1111/j.1469-7793.2000.00417.x
- Overton JM, Williams TD, Chambers JB, Rashotte ME. Cardiovascular and metabolic responses to fasting and thermoneutrality are conserved in obese Zucker rats. Am J Physiol 2001; 280:R1007-15; PMID:11247881
- Boström P, Wu J, Jedrychowski MP, Korde A, Ye L, Lo JC, Rasbach KA, Boström EA, Choi JH, Long JZ, et al. A PGC1α-dependent myokine that drives browning of white fat and thermogenesis. Nature 2012; 481:469-8; PMID:22237023; http://dx.doi.org/10.1038/nature10777
- Stehling O, Doring H, Ertl J, Preibisch G, Schmidt I. Leptin reduces juvenile fat stores by altering the circadian cycle of energy expenditure. Am J Physiol 1996; 271:R1770-4; PMID:8997381
- Metzger JM, Gagen K, Raustad KA, Yang L, White A, Wang S-P, Craw S, Liu P, Lanza T, Lin LS, et al. Body temperature as a mouse pharmacodynamic response to bombesin receptor subtype-3 agonists and other potential obesity treatments. Am J Physiol 2010; 299:E816-24; PMID:20807840; http://dx.doi.org/10.1152/ajpendo.00404.2010