Abstract
When skin temperature falls below a set-point, mammals experience “cold in the skin” and exhibit heat-seeking behaviors for error correction. Physiological thermostats should perform the behavioral thermoregulation, and it is important to identify the thermostats. A classical model of the sensory system states that thermoreceptors (e.g., thermoTRPs) in skin nerve endings are sensors that transform temperature into the firing rate codes that are sent to the brain, where the codes are decoded as “cold” by a labeled line theory. However, the view that the temperature code is transformed into “cold” (not temperature) is conflicting. Another model states that a thermostat exists in the brain based on the view that a skin thermo-receptor is a sensor. However, because animals have no knowledge of the principle of temperature measurement, the brain is unable to measure skin temperature with a thermometer calibrated based on a code table of each sensor in the skin. Thus, these old models cannot identify the thermostats. We have proposed a new model in which temperature receptors in a nerve ending are molecules of the thermostats. When skin temperature falls below a set-point, these molecules as a whole induce impulses as command signals sent to the brain, where these impulses activate their target neurons for “cold” and heat-seeking behaviors for error correction. Our study challenges the famous models that sensory receptor is a sensor and the brain is a code processor.
Abbreviations
TRP channel | = | transient receptor potential channel |
Introduction
In artificial systems, a thermostat is a thermoregulator that automatically regulates temperature by starting a heater (or cooler) against thermal load. A key component of a thermostat is a comparator that compares whether a system's controlled temperature is lower (or higher) than a set-point and generates an error-dependent command signal to start the heater (or cooler) so that the error may vanish.Citation1,2 In biological systems, when skin temperature falls below a set-point, mammals experience “cold” and exhibit heat-seeking behaviors so that “cold” may vanish.Citation3-6 Physiological thermostats should perform the behavioral thermoregulation against thermal load, and it is very important to identify the thermostats in temperature physiology.
A classical model of the sensory systemCitation7,8 states that low temperature-sensitive receptors (e.g., TRPM8 channelsCitation3-6) in cutaneous nerve endingsCitation9,10 are sensors (i.e., transducers) that transform skin temperature into the firing rate code that is sent to the brain, where the code is decoded as “cold” by a labeled line theory.Citation11,12 However, because temperature and “cold” are entirely different phenomena, the model in which the temperature code is decoded as “cold” (not temperature) is very inconsistent. Thus, the model cannot explain what the physiological thermostats are. Another model states that a thermostat exists in the brainCitation13,14 based on the view that a skin thermoreceptor is a sensor for monitoring skin temperature. However, animals (except humans) have no knowledge of the principle of temperature measurement. In the brain of animals, there is no one who would measure temperature by observing a thermometer previously calibrated based on a code table of each sensor in the skin. Thus, the model also cannot identify what the thermostats are.
In contrast, we have proposed a new model in which thermoreceptors (e.g., TRPM8 channels) in a cutaneous nerve endingCitation9,10 are thermostat molecules,Citation1,15-17; when skin temperature falls below a set-point, these molecules as a whole induce nerve impulses as command signals (not codes), which are sent to the brain to activate the target neurons for “cold in the skin” and start heat-seeking behaviorsCitation3-6 so that “cold” may vanish.
The present article describes (1) problems inherent in old models of thermostats and (2) a new model of thermostats. We primarily describe low temperature-sensitive receptors.Citation16,17
Problems Inherent in Old Models of the Physiological Thermostats
A classical model of the sensory system
AdrianCitation7 proposed a model that explains “sensation” using the sensory system. In the thermosensory system (), for example, it has been assumed that thermoreceptors (e.g., thermoTRP channels, such as TRPM8Citation3-6 and TRPV1Citation18) in skin nerve endings are sensors that transform temperatures into the firing rate codes, which are sent to the brain, where these codes are decoded as “cold” (or “hot”) by a labeled line theory.Citation11,12 Such a sensory mechanism is also reported in flies.Citation19-21 The model assuming that the sensory system is apparently similar to the coding-decoding system in technology has generally been used to explain various types of “sensation”Citation8,22 and thermoregulatory behaviorCitation4-6 as well as the famous view that the brain is a code processor like a digital computer based on a binary code system.
Figure 1. Classical models of the thermoregulatory system. (A) The thermosensory system. It is assumed that thermoreceptor in cutaneous nerve ending is a thermosensor that sends nerve impulses as a temperature code to the brain, where the code is somehow decoded as “cold sensation.” (B) A thermostat in the brain. It is assumed that a thermostat (interneuron) exists in the brain based on the view that a skin thermoreceptor is a sensor to monitor skin temperature. This model states that the thermostat compares skin temperature with a set-point, and the error dependent output induces thermoregulatory responses. A circle with 2 inputs (+ and −) shows a comparator of the thermostat.
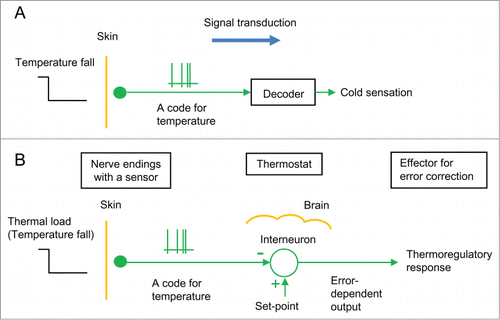
However, temperature is an objective physical quantity and “cold” is a subjective cognitive function. Because temperature and “cold” are entirely different phenomena, the model in which the temperature code is decoded as “cold sensation” (not temperature) is inconsistent. Thus, the model is unable to explain what the physiological thermostats are.
A thermostat in the brain
Based on the model that skin thermoreceptors are sensors (), temperature physiologistsCitation13,14 have proposed a model in which a thermostat exists in the brain (). This model states that the thermostat (interneuron) compares skin temperature sent from a cutaneous sensor with a set-point at the code level and the error-dependent output induces thermoregulatory responses so that the error may vanish. In regulation of core temperature, it has been assumed that thermosensitive neurons in the brain are thermosensors for monitoring local brain temperatures and that a thermostat in the brain similarly processes thermal codes to induce thermoregulatory responses.Citation13,14,23-25
Temperature is a physical quantity defined by thermodynamics. Only humans have the knowledge of the definition of temperature and the principle of temperature measurement. We measure temperature by observing a thermometer, which is calibrated previously based on a code table showing a relation between temperature and code (e.g., a relation between temperature and an electric resistance (code) of a thermistor). It should be emphasized that animals (except humans) have no knowledge of the thermodynamic definition of temperature or the principle of temperature measurement. In the brain of animals, there is no one who would measure skin temperature by observing a thermometer calibrated based on a code table of each thermosensor in the skin. The model that the thermosensory system is similar to the artificial temperature measurement system is doubtful.
Because animals do not know the definition of temperature, it is impossible for the brain of animals to generate a set-point at the code level. Thus, the presence of the cerebral thermostat is unlikely. Interneurons that receive impulses sent from skin thermoreceptors and nearby neurons are incapable of processing or integrating temperature codes; they are only activated or inhibited by pre-synaptic impulses.
Judging from their responses to temperature, thermoreceptors are unlikely to act as thermosensors. If thermoreceptors are sensors, they should respond to a wide range of temperatures () as described by Adrian.Citation7 However, these thermoreceptors show threshold responses to a temperature fall (or rise) (). TRPM8Citation3,11,26 and TRPV1Citation18 stop generating output at normal temperatures ().
Figure 2. A new model of a physiological thermostat. (A, B) A typical patch-clamp recording from sensory neurons responding to low temperatures (drawn referring to Okazawa et al.Citation16). (A) Whole-cell recordings. When the temperature falls below its whole-cell set-point (˜28 °C, threshold temperature), receptor potential occurs. When the receptor potential is above a threshold potential, action potentials occur. However, action potentials are inhibited when the receptor potential continues. Inset: time scale is expanded. (B) Single-channel recordings from an isolated patch membrane. When the temperature falls below its single-channel set-point (˜18°C, threshold temperature), single-channel activity appears.
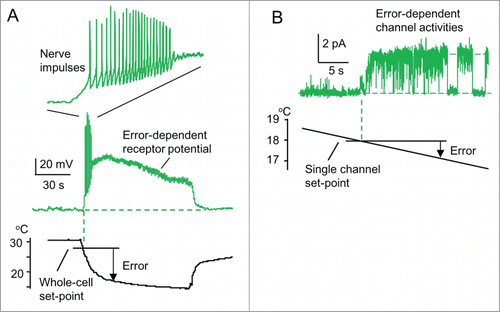
Figure 3. ThermoTRP channels as thermostat molecules. Error-dependent receptor potential of typical thermoTRP channels (Patapoutian et al.Citation11). The numbers in parentheses represent whole-cell set-points. TRPM8 and TRPA1 are thermostat molecules against low temperatures. TRPV1-4 are thermostat molecules against high temperatures.
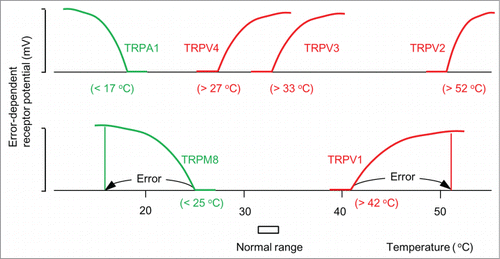
ThermoTRP channels are multimodal receptors that respond to both temperature and chemical compounds.Citation11 For example, TRPM8 channels are sensitive to low temperatures, menthol, and icilin.Citation3 These multimodal responses show that thermoTRP channels are unusable as a thermosensor. Thus, these old models, based on the view that thermoreceptors are sensors,Citation7 cannot explain the physiological thermostats.
Problems inherent in the old models of the thermostats are summarized as follows:
1. | A classical model of the sensory system () states that the temperature code sent from a sensor is decoded as “cold” by a labeled line theory. However, because temperature and “cold” are different phenomena, the view that a temperature code is decoded as “cold” (not temperature) is inconsistent. The model cannot explain what physiological thermostats are. | ||||
2. | Another model () states that a thermostat exists in the brain based on the view that a temperature receptor is a thermosensor. However, because animals (except humans) have no knowledge of the principle of temperature measurement, the brain is impossible to measure skin temperature by observing a thermometer calibrated based on a code table of each sensor in the skin. Thus, the presence of the brain thermostat is doubtful. | ||||
3. | These old models based on the view that a temperature receptor is a sensor cannot explain what physiological thermostats are. |
A New Model of the Physiological Thermostats
Thermoreceptors act as the thermostat molecules
Extracellular unit recordings from slices of the rat hypothalamus have shown that there are low- and high-temperature sensitive neurons inactive at normal core temperature.Citation15 These low-temperature sensitive neurons start firing only when the slice temperature is decreased below their threshold temperatures lower than normal. Because of the threshold responses, we have proposed that these neurons act as thermostats (not thermosensors), which have their threshold temperatures as set-points for regulation of the core temperature.Citation1,15
To study a mechanism of temperature comparison in low temperature-sensitive thermostat neurons, we have performed patch-clamp recordings from peripheral sensory neurons isolated from the dorsal root ganglia of rats.Citation16 From their threshold responses, we have proposed that thermoreceptors are thermostat molecules () and the mechanism of temperature comparison is phase transition at the set-point.Citation16,17 These receptors are non-selective cation channelsCitation16 and appear to correspond to TRPM8Citation3,17 and TRPA1.Citation27,28
shows a typical example of the patch-clamp recordings. These neurons were used as a model of nerve terminals in the skin. In whole-cell recordings of membrane potential (), the temperature is decreased from 30 to 15°C and maintained at 15°C for 90 s. When the temperature falls below its set-point (threshold temperature, ˜28°C), depolarization occurs as a receptor potential. The receptor potential depends on the thermal error from the set-point to the temperature, but it decreases slightly with time (time-dependent desensitization of receptors).
When the depolarization at a rising phase is above a threshold potential, repetitive nerve impulses (a conductive signal used for stimulating the target cells in vivo) appear. However, these impulses are rather inhibited despite a continued receptor potential and last only for a short period (˜6 s). This indicates a depolarization-induced inhibition of reactivation of voltage gated Na channel.Citation29 Thus, a moderate size of a receptor potential seems to lengthen the duration of firing (cf. ). The brief impulse activities (phasic response) at the start of temperature fall correspond to a differential control in an artificial thermostat. However, the phasic response is a feature of this thermostat neuron using a feedback control (), and is not a feedforward control. Whether a feedforward controlCitation2,30 is true should be examined. Thus, the duration (˜6 s) of the firing is much shorter than that (90 s) of the thermal stimulation. This indicates that these nerve impulses are not used as a code for the low temperature.
Figure 4. A new model of the thermoregulatory system (Tajino et al.Citation17). Thermoregulatory system is composed of neural connection from a cutaneous nerve ending with thermostat molecules (TRPM8) to its target cerebral neurons for “cold” and heat-seeking behavior. A circle with 2 inputs (+ and −) shows a thermostat neuron. When skin temperature falls below a set-point, thermostat molecules in a nerve ending together generate error-dependent receptor potential for nerve impulses. These impulses run to the brain to activate its target neurons for “cold” and heat-seeking behaviors for error correction.
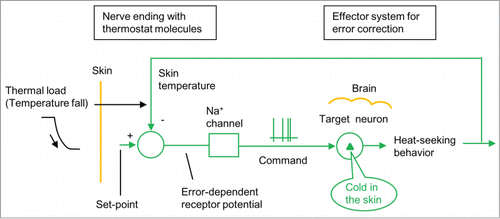
To analyze the comparator mechanism of thermoreceptors, we recorded single-channel currents underlying the whole-cell receptor potential in a membrane patch excised from a neuron (). Temperature was decreased slowly. When temperature falls below a set-point (18°C), a surge in channel current occurs. The threshold response indicates that these receptor channels are thermostat molecules that compare whether temperature is lower than the set-point without using a code, and induce error-dependent channel activities as output signals. The mechanism of the temperature comparison in this channel is its phase transition between “on” and “off” states at the set-point (). A phase transition at a set-point is common among ion channels, such as voltage-gated Na channelsCitation29,31 and sensory receptors.Citation32
ThermoTRP channels act as physiological thermostats
Molecular studies have clarified that excitatory thermoTRP channels in the transient receptor potential (TRP) non-selective cation channel family act as thermoreceptors.Citation11 schematically shows thermal responses of receptor potential in a cell expressing typical TRP channels.Citation11 These responses indicate that TRP channels as a whole compare cell temperature with a whole-cell set-point and generate error-dependent receptor potential without using a code. These channels are divided into 2 groups. TRPM8Citation3,26 and TRPA1Citation27,28 are activated when the temperature is below the set-point (25°C in TRPM8 and 17 °C in TRPA1), which shows that they are thermostats functioning against low ambient temperatures. TRPV1-4Citation11,18,33,34 channels are activated when the temperature is above the set-point (42°C in TRPV1, 52°C in TRPV2, 33°C in TRPV3, and 27°C in TRPV4), which shows that they are thermostats against high ambient temperatures.
TRPM8 knockout studies have shown that when the ambient temperature falls, TRPM8 induces “cold” and heat-seeking behaviors in wild-type mice.Citation4-6,17 This indicates that cutaneous nerve endings with TRPM8 channelsCitation9,10 are connected to the cerebral target neurons for “cold” ().Citation17 Thus, TRPM8 channels act as thermostat molecules and induce “cold in the skin” and heat-seeking behaviors in response to low ambient temperatures. Skin nerve endings expressing TRPM8Citation9,10 are present in the whole body. This indicates that skin temperatures are regulated by a large number of nerve endings with thermostat molecules.Citation4–6,17
As described above, objective temperature and subjective “cold” are entirely different phenomena. The old model stating that temperature is equivalently transformed into “cold” () is inconsistent. The new model consistently explains that the sensory system functions to replace a low temperature applied to skin nerve endings with “cold in the skin” ().
Electric stimuli of the somatosensory cortex of the brain evoke “touch sensations in the skin.”Citation35 When the face is touched, the sensation of “phantom fingers” is reported by amputees.Citation36 These results suggest that the mechanisms that induce “sensations” are present in brain neurons and are consistent with the above model.
ThermoTRP channels are multimodal receptors that respond to chemical compounds as well as temperature.Citation11 For example, TRPM8 responds to menthol (>20 μMCitation3), TRPA1 responds to allyl isothiocyanate (>5 μMCitation37,38), and TRPV1 responds to capsaicin (>0.2 μMCitation18). These threshold responses show that thermoTRP channels are also comparators that compare whether agonist concentrations are above their threshold concentrations. Menthol application on the skin induces “cold in the skin.”Citation39 This observation demonstrates that both low temperature and menthol induce “cold in the skin,” which supports the hypothesis that cutaneous TRPM8 is connected to brain neurons responsible for “cold in the skin.”
The important contribution of the present study is summarized as follows:
1. | Temperature receptors (e.g., thermoTRP channels) in skin nerve endings of a whole body are molecules of the physiological thermostats. | ||||
2. | The mechanism of the temperature comparison in the thermostats' molecules is a phase transition of these ion channels at a set-point. | ||||
3. | When skin temperature falls below a set-point, cutaneous nerve endings with TRPM8 thermostats generate nerve impulses sent to the brain, where these impulses activate their target neurons for “cold in the skin” and heat-seeking behaviors for maintaining skin temperature and resultantly core temperature. | ||||
4. | The new model consistently explains that the sensory system functions to replace a low temperature applied to the skin with “cold in the skin” (). |
Conclusion
When ambient temperature falls below a set-point, animals experience “cold in the skin” and exhibit heat-seeking behaviors so that “cold” may vanish. Physiological thermostats should induce these heat-seeking behaviors for thermoregulation against thermal load. However, it is not well understood what physiological thermostats are. The important contribution of this study is to show that low temperature-sensitive receptors (e.g., TRPM8) in a cutaneous nerve ending are molecules of the physiological thermostat.Citation17 When skin temperature falls below a set-point, these thermostat molecules in nerve endings induce error-dependent receptor potential, which induces nerve impulses sent to the brain, where these impulses activate the target neurons for “cold” and heat-seeking behaviors for error correction. The new model consistently explains that the sensory system functions to replace a low skin temperature with “cold in the skin.” This study challenges the famous models that sensory receptors are sensors and that the brain is a code processor.
The new thermostat model requires connections from nerve endings with thermostat molecules to their target neurons for “cold.”Citation17 Determining the molecular basis that underlies “cold sensation” of our consciousness will be important for understanding genes for cognitive functions.
The intraperitoneal injection of 5′-AMP induces a decrease in core temperature to an ambient temperature in mice,Citation40,41 indicating that homeostasis of the core temperature is broken by the 5′-AMP treatment. Meanwhile, thermoTRP channels are involved in the modulation of core temperature.Citation17,42-44 More studies may be necessary to understand the thermoregulatory system for homeostasis of core temperature in mammals.
Disclosure of Potential Conflicts of Interest
No potential conflicts of interest were disclosed.
References
- Kobayashi S. Temperature-sensitive neurons in the hypothalamus: a new hypothesis that they act as thermostats, not as transducers. Prog Neurobiol 1989; 32:103–35; PMID:2645618; http://dx.doi.org/10.1016/0301-0082(89)90012-9
- Romanovsky A. Skin temperature: its role in thermoregulation. Acta Physiol 2014; 210:498–507; PMID:24716231; http://dx.doi.org/10.1111/apha.12231
- McKemy DD, Neuhausser WM, Julius D. Identification of a cold receptor reveals a general role for TRP channels in thermosensation. Nature 2002; 416:52–8; PMID:11882888; http://dx.doi.org/10.1038/nature719
- Bautista DM, Siemens J, Glazer JM, Tsuruda PR, Basbaum AI, Stucky CL, Jordt SE, Julius D. The menthol receptor TRPM8 is the principal detector of environmental cold. Nature 2007; 448:204–8; PMID:17538622; http://dx.doi.org/10.1038/nature05910
- Dhaka A, Murray AN, Mathur J, Earley TJ, Petrus MJ, Patapoutian A. TRPM8 is required for cold sensation in mice. Neuron 2007; 54:371–8; PMID:17481391; http://dx.doi.org/10.1016/j.neuron.2007.02.024
- Colburn RW, Lubin ML, Stone DJ, Jr., Wang Y, Lawrence D, D'Andrea MR, Brandt MR, Liu Y, Flores CM, Qin N. Attenuated cold sensitivity in TRPM8 null mice. Neuron 2007; 54:379–86; PMID:17481392; http://dx.doi.org/10.1016/j.neuron.2007.04.017
- Adrian ED. The Basis of Sensation: The Action of the Sence Organs. London: Christophers, 1928.
- Kandel ER, Schwartz JH, Jessell TM, eds. Principles of Neural Science. New York: McGraw-Hill, 2000.
- Takashima Y, Daniels RL, Knowlton W, Teng J, Liman ER, McKemy DD. Diversity in the neural circuitry of cold sensing revealed by genetic axonal labeling of transient receptor potential melastatin 8 neurons. J Neurosci 2007; 27:14147–57; PMID:18094254; http://dx.doi.org/10.1523/JNEUROSCI.4578-07.2007
- Dhaka A, Earley TJ, Watson J, Patapoutian A. Visualizing cold spots: TRPM8-expressing sensory neurons and their projections. J Neurosci 2008; 28:566–75; PMID:18199758; http://dx.doi.org/10.1523/JNEUROSCI.3976-07.2008
- Patapoutian A, Peier AM, Story GM, Viswanath V. ThermoTRP channels and beyond: mechanisms of temperature sensation. Nat Rev Neurosci 2003; 4:529–39; PMID:12838328; http://dx.doi.org/10.1038/nrn1141
- Ma Q. Labeled lines meet and talk: population coding of somatic sensations. J Clin Invest 2010; 120:3773–8; PMID:21041959; http://dx.doi.org/10.1172/JCI43426
- Bligh J. Temperature Regulation in mammals and other vertebrates. Amsterdam: North-Holland Publishing, 1973.
- Hensel H. Neural processes in thermoregulation. Physiol Rev 1973; 53:948–1017; PMID:4355518
- Kobayashi S. Warm- and cold-sensitive neurons inactive at normal core temperature in rat hypothalamic slices. Brain Res 1986; 362:132–9; PMID:3942861; http://dx.doi.org/10.1016/0006-8993(86)91406-X
- Okazawa M, Takao K, Hori A, Shiraki T, Matsumura K, Kobayashi S. Ionic basis of cold receptors acting as thermostats. J Neurosci 2002; 22:3994–4001; PMID:12019319
- Tajino K, Hosokawa H, Maegawa S, Matsumura K, Dhaka A, Kobayashi S. Cooling-sensitive TRPM8 is thermostat of skin temperature against cooling. PLoS One 2011; 6:e17504; PMID:21407809
- Caterina MJ, Schumacher MA, Tominaga M, Rosen TA, Levine JD, Julius D. The capsaicin receptor: a heat-activated ion channel in the pain pathway. Nature 1997; 389:816–24; PMID:9349813; http://dx.doi.org/10.1038/39807
- Gallio M, Ofstad TA, Macpherson LJ, Wang JW, Zuker CS. The coding of temperature in the Drosophila brain. Cell 2011; 144:614–24; PMID:21335241; http://dx.doi.org/10.1016/j.cell.2011.01.028
- Liu WW, Mazor O, Wilson RI. Thermosensory processing in the Drosophila brain. Nature 2015; 519:353–7; PMID:25739502; http://dx.doi.org/10.1038/nature14170
- Frank DD, Jouandet GC, Kearney PJ, Macpherson LJ, Gallio M. Temperature representation in the Drosophila brain. Nature 2015; 519:358–61; PMID:25739506; http://dx.doi.org/10.1038/nature14284
- Ranade SS, Woo SH, Dubin AE, Moshourab RA, Wetzel C, Petrus M, Mathur J, Begay V, Coste B, Mainquist J, et al. Piezo2 is the major transducer of mechanical forces for touch sensation in mice. Nature 2014; 516:121–5; PMID:25471886; http://dx.doi.org/10.1038/nature13980
- Nakayama T, Hammel HT, Hardy JD, Eisenman JS. Thermal stimulation of electrical activity of single units of the preoptic region. Am J Physiol 1963; 204:1122–6.
- Hammel HT, DC J, Stolwijk JAJ, Hardy JD, Stromme SB. Temperature regulation by hypothalamic proportional control with an adjustable set-points. J Appl Physiol 1963; 18:1146–54; PMID:14080734
- Mitchell D, Snellen JW, Atkins AR. Thermoregulation during fever: change of set-point or change of gain. Pflugers Arch 1970; 321:293–302; PMID:5531238; http://dx.doi.org/10.1007/BF00588644
- Peier AM, Moqrich A, Hergarden AC, Reeve AJ, Andersson DA, Story GM, Earley TJ, Dragoni I, McIntyre P, Bevan S, et al. A TRP channel that senses cold stimuli and menthol. Cell 2002; 108:705–15; PMID:11893340; http://dx.doi.org/10.1016/S0092-8674(02)00652-9
- Story GM, Peier AM, Reeve AJ, Eid SR, Mosbacher J, Hricik TR, Earley TJ, Hergarden AC, Andersson DA, Hwang SW, et al. ANKTM1, a TRP-like channel expressed in nociceptive neurons, is activated by cold temperatures. Cell 2003; 112:819–29; PMID:12654248; http://dx.doi.org/10.1016/S0092-8674(03)00158-2
- Sawada Y, Hosokawa H, Hori A, Matsumura K, Kobayashi S. Cold sensitivity of recombinant TRPA1 channels. Brain Res 2007; 1160:39–46; PMID:17588549; http://dx.doi.org/10.1016/j.brainres.2007.05.047
- Ulbricht W. Sodium channel inactivation: molecular determinants and modulation. Physiol Rev 2005; 85:1271–301; PMID:16183913; http://dx.doi.org/10.1152/physrev.00024.2004
- Nakamura K, Morrison SF. A thermosensory pathway that controls body temperature. Nat Neurosci 2008; 11:62–71; PMID:18084288; http://dx.doi.org/10.1038/nn2027
- Hodgkin AL, Huxley AF. A quantitative description of membrane current and its application to conduction and excitation in nerve. J Physiol 1952; 117:500–44; PMID:12991237; http://dx.doi.org/10.1113/jphysiol.1952.sp004764
- Hille B. Ionic channels of excitable membranes. Sunderland: Sinauer, 2001.
- Caterina MJ, Rosen TA, Tominaga M, Brake AJ, Julius D. A capsaicin-receptor homologue with a high threshold for noxious heat. Nature 1999; 398:436–41; PMID:10201375; http://dx.doi.org/10.1038/18906
- Smith GD, Gunthorpe MJ, Kelsell RE, Hayes PD, Reilly P, Facer P, Wright JE, Jerman JC, Walhin JP, Ooi L, et al. TRPV3 is a temperature-sensitive vanilloid receptor-like protein. Nature 2002; 418:186–90; PMID:12077606; http://dx.doi.org/10.1038/nature00894
- Penfield W, Rasmussen T. The cerebral cortex of man. A clinical study of localization of function. New York: Macmillan, 1950.
- Ramachandran VS, Hirstein W. The perception of phantom limbs. The D. O. Hebb lecture. Brain 1998; 121:1603–30; PMID:9762952; http://dx.doi.org/10.1093/brain/121.9.1603
- Bandell M, Story GM, Hwang SW, Viswanath V, Eid SR, Petrus MJ, Earley TJ, Patapoutian A. Noxious cold ion channel TRPA1 is activated by pungent compounds and bradykinin. Neuron 2004; 41:849–57; PMID:15046718; http://dx.doi.org/10.1016/S0896-6273(04)00150-3
- Jordt SE, Bautista DM, Chuang HH, McKemy DD, Zygmunt PM, Hogestatt ED, Meng ID, Julius D. Mustard oils and cannabinoids excite sensory nerve fibres through the TRP channel ANKTM1. Nature 2004; 427:260–5; PMID:14712238; http://dx.doi.org/10.1038/nature02282
- Green BG. The sensory effects of l-menthol on human skin. Somatosens Mot Res 1992; 9:235–44; PMID:1414120; http://dx.doi.org/10.3109/08990229209144774
- Zhang J, Kaasik K, Blackburn MR, Lee CC. Constant darkness is a circadian metabolic signal in mammals. Nature 2006; 439:340–3; PMID:16421573; http://dx.doi.org/10.1038/nature04368
- Swoap SJ, Rathvon M, Gutilla M. AMP does not induce torpor. Am J Physiol Regul Integr Comp Physiol 2007; 293:R468–73; PMID:17409259; http://dx.doi.org/10.1152/ajpregu.00888.2006
- Gavva NR, Bannon AW, Surapaneni S, Hovland DN, Jr., Lehto SG, Gore A, Juan T, Deng H, Han B, Klionsky L, et al. The vanilloid receptor TRPV1 is tonically activated in vivo and involved in body temperature regulation. J Neurosci 2007; 27:3366–74; PMID:17392452; http://dx.doi.org/10.1523/JNEUROSCI.4833-06.2007
- Gavva NR, Treanor JJ, Garami A, Fang L, Surapaneni S, Akrami A, Alvarez F, Bak A, Darling M, Gore A, et al. Pharmacological blockade of the vanilloid receptor TRPV1 elicits marked hyperthermia in humans. Pain 2008; 136:202–10; PMID:18337008; http://dx.doi.org/10.1016/j.pain.2008.01.024
- Garami A, Pakai E, Oliveira DL, Steiner AA, Wanner SP, Almeida MC, Lesnikov VA, Gavva NR, Romanovsky AA. Thermoregulatory phenotype of the Trpv1 knockout mouse: thermoeffector dysbalance with hyperkinesis. J Neurosci 2011; 31:1721–33; PMID:21289181; http://dx.doi.org/10.1523/JNEUROSCI.4671-10.2011