Abstract
In humans, the TRP superfamily of cation channels includes 27 related molecules that respond to a remarkable variety of chemical and physical stimuli. While physiological roles for many TRP channels remain unknown, over the past years several have been shown to function as molecular sensors in organisms ranging from yeast to humans. In particular, TRP channels are now known to constitute important components of sensory systems, where they participate in the detection or transduction of osmotic, mechanical, thermal, or chemosensory stimuli. We here summarize our current understanding of the role individual members of this versatile receptor family play in thermosensation and thermoregulation, and also touch upon their immerging role in metabolic control.
Introduction
The founding member of the Transient Receptor Potential ion channel family was first discovered by Craig Montell and colleagues in Drosophila, where it constitutes a central component of the visual transduction cascade in fly photoreceptors.Citation1
There are 6 TRP subfamilies: “canonical” TRPCs (TRPC1–7), “vanilloid” TRPVs (TRPV1–6), “melastatin-like” TRPMs (TRPM1–8), “polycystin” TRPPs (TRPP2, TRPP3, TRPP5), “mucolipin” TRPMLs (TRPML1–3), and “ankyrin-rich” TRPA (TRPA1). Hallmark features of all TRPs are a similar transmembrane topology that is comprised of 6 (S1-S6) transmembrane domains and a cation-permeable pore region between S5 and S6. Sequence and length of the intracellular N- and C-termini vary significantly among different TRP members.Citation2
Gating of several TRP ion channels has been found to display steep temperature dependence in the physiological temperature range. The Q10 value, defined here as the change in current amplitude upon a temperature increase of 10 degrees, has been widely used to characterize the degree of temperature sensitivity of ion channels. Most ion channels exhibit a Q10 value between 1 and 3.Citation3 On the other hand, 11 mammalian TRP ion channels -when heterologously expressed- have Q10 values that are either larger than 5 (warm-sensitive) or lower than 0.2 (cold sensitive). By these criteria, TRPV1–4 and TRPM2–5 are warm-sensitive,Citation4–13 while TRPM8, TRPA1 and TRPC5 have been found to be cold sensitive ion channels ().Citation14,15
Figure 1. Temperature Sensitive (TRP) Ion Channels. (A) The cartoon illustrates the different ion-selectivity and current directionality of temperature-sensitive ion channels that reside in primary afferent sensory neurons detecting warm and cold temperatures, respectively. Modified with permission from ref. 51. (B) The graphs schematically depict temperature activation curves of warm/hot sensitive ion channels (upper panel) and cold sensitive channels (lower panel) that are found in primary afferent temperature-sensitive sensory neurons as depicted in (A).
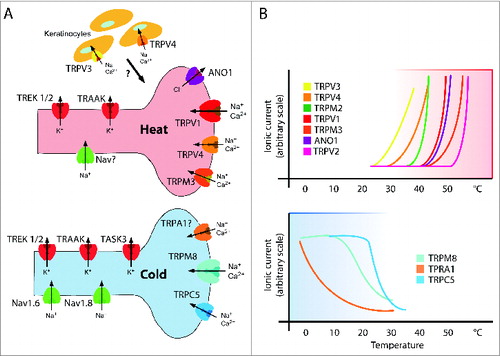
Temperature sensitivity alone does by itself not infer that these ion channels are functionally relevant temperature detectors. Two additional criteria have been used as filters to single out relevant molecular temperature detectors: (i) expression of TRP channels in tissues and at sites that are relevant to detect temperature change and (ii) thermosensory (or thermoregulatory) phenotypes in genetically tractable organisms such as knock-out mice or mutant flies and worms.
Both criteria are not as straight forward to apply as it might seem. Temperature receptors residing in the skin (or in neuronal processes that innervate the skin) are likely to experience the largest temperature changes to be physiologically relevant. However, smaller temperature changes within the body cavity, intestine, central nervous system and other tissues that occur as part of metabolic changes, increased (neuronal) activity or inflammatory responses (such as fever) are clearly physiologically -and pathologically- relevant and are very likely monitored by sensory mechanisms. These types of interoceptive temperature receptors have largely remained elusive. One reason for the lack of knowledge is that exact whereabouts and molecular markers for these temperature sensitive structures are not known. Thus it is currently not clear if TRP channels have a role in interoceptive temperature detection.
Along similar lines, TRP channel knockout models have been extensively studied in the context of environmental temperature stimuli such as heat- and cold-pain assays and preference tests.Citation16 However, it is possible that putative temperature phenotypes have not yet been identified, simply because these models have not been tested in the context of more tissue specific (or internal) temperature changes.
TRP Channels in Thermosensation
When applying the aforementioned criteria and when focusing on environmental temperature detection, only a few “Thermo-TRPs” pass the test and have thus far been implicated in thermosensation ().
Table 1. Thermosensation in vivo
The only TRP channel for which there is broad agreement about its central role in temperature detection is TRPM8. Three research groups independently identified a crucial function for TRPM8 in detecting cool to cold temperatures (between ∼15°C and ∼30°C) in a number of different physiological and behavior tests.Citation17–20 TRPM8 is expressed in a subset of small to medium diameter dorsal root ganglia (DRG) sensory neurons that innervate the skin. Additionally, TRPM8 is also found in trigeminal ganglion (TG) sensory neurons that innervate (among other areas in the head and neck region) the eye and cornea. Interestingly, cooling of the cornea as a consequence of evaporation has been shown to trigger tearing in a TRPM8-dependent manner.Citation21 Thus TRPM8, likely via detecting temperature changes, contributes to proper moistening of the cornea.
Interestingly, tuning of sensory neurons to a range of different (cold) temperatures appears not to be regulated by the mere presence (or expression level) of the TRPM8 receptor, but is governed by combinatorial expression of other (potassium) ion channels such as Task-3 (KCNK9), Kv1 (KCNA) and Kv7 (KCNQ) ion channels, that control and modulate cold-induced excitability ().Citation22–24
Cold-induced gating of another TRP ion channel, TRPC5, has recently been reported.Citation25 However, ablation of the TRPC5 gene did not result in detectable cold-sensitivity defects in standard mouse behavior tests. Future studies will tell whether this ion channel is required for (more restricted) tissue specific or context-specific cold detection.
TRPV1 knock-out mice appear to have a subtle phenotype in acute heat sensation, exemplified by their difficulties in detecting noxious temperatures in tail immersion assays.Citation26 Pharmacological inhibition of TRPV1 in human subjects also reduces their ability to detect painful heat stimuli.Citation27,28 Generally, however, TRPV1 knockout mouse models appear to have only minor difficulties in detecting hot temperatures,Citation29 suggesting that other mechanisms participate in heat detection.
In this regard it is interesting to note that an alternatively spliced variant of TRPV1, that is present in vampire bat sensory neurons, is tuned to a lower temperature threshold of around 30°C which has been correlated with exquisite heat sensitivity allowing the animal to target warm-blooded prey.Citation30
Contrary to the mild thermosensory phenotype in TRPV1 knock-out mice under basal physiological conditions, it is well established that TRPV1 plays a dominant role in heat detection when the receptor is sensitized in the context of inflammation and tissue injury: A diverse group of disparate inflammatory mediators dramatically decrease the temperature threshold for TRPV1 and increase the response magnitude of the ion channel, thereby producing heat hyperalgesia and inflammatory pain.Citation4,26,29,31,32 Sensitization of the capsaicin receptor emphasizes its pathological property. This feature has promoted the receptor to become a prime target for analgesic drug development. However, blocking TRPV1 activity triggers severe advers effects such as hyperthermia,Citation33,34 demonstrating that TRPV1 -at least acutely- affects core body temperature, as discussed below.
For analegsic therapy it would thus be more desirable to specifically inhibit sensitization of the receptor while leaving normal homeostactic activity of the ion channel intact. A recent study demonstrates that this might be feasable. By engaging a non canonical GABA/GABAB pathway, TRPV1 sensitzation is specifically counteracted while basal activity of the receptor is left intact.Citation35
Recently, TRPM3 has been found to constitute a heat-sensitive ion channel expressed in DRG and TG sensory neurons that contributes to heat nociception. The TRPM3 knockout phenotype bears some similiarity to the TRPV1 knockout phenotype.Citation13 It will be interesting to test whether TRPM3 and TRPV1 synergistically participate in heat nociception, potentially even in combinations with other (non-TRP) warm/heat-sensitive ion channels and proteins. In this regard it is very interesting to note that the chloride channel Anoctamin 1 has been demonstrated to be also activated by noxious heat and to also participate in heat nociception.Citation36,37
Albeit TRPM2 and TRPM4 are temperature-sensitive ion channels, no thermosensory phenotype has yet been reported for either receptor knock-out mouse model. The temperature sensitivity of TRPM5 has been reported to modulate taste perception,Citation11 but whether this property of TRPM5 is relevant in any other sensory or homeostatic context is currently unknown.
For other members of the TRPV family, notably TRPV2, 3 and 4, variable results have been reported. Initial findings suggested that TRPV3 and TRPV4 knockout mice have defects in detecting warm tempertures in behavior paradigms.Citation38,39 However, more recent data refute these results and suggest that these phenotypes are highly dependent on the genetic background. In these studies, even TRPV3 - TRPV4 double knock-out mice only have very subtle deficits in temperature preference and heat avoidance assays.Citation40,41 Similarly, TRPV2 deficient mice do not display any aberrant thermosensation.Citation42
Arguably the most contentious member of the TRP channel family in regards to thermosensation in mice is TRPA1. While some studys find TRPA1 to contribute to cold sensation,Citation43,44 others find no evidence for TRPA1's involvement in cold thermosensation in vivo.Citation45,46
Interestingly, in other species, such as insects and reptiles there is evidence that TRPA1 is required for detecting warm/hot (but not cold) temperatures.Citation47–50 Thus, albeit doubts remain about the temperture sensitivity of human and mouse TRPA1, there is accumulating evidence that TRPA1 in other species functions as temperature sensor.
Collectively these studies show that for none of the TRP channels (maybe with the exception of TRPM8) a dominant -or universal- function in environmental temperature detection has been found. Rather, these studies suggest that particular TRP channels are recruited (and are exquisitely tuned) for thermosensation in a (i) species-specific, (ii) tissue-specific and (iii) context-specific manner. This feature is perhaps best exemplified by TRPV1 and TRPA1, as discussed above.
One possible reason for the lack of a universal thermosensory phenotype is that many temperature-sensitive TRP (and non-TRP-) receptors detect overlapping temperatures in the warm to hot range ().Citation3,51,52 Therefore, individual TRP channel knockout models (and even double knock-out mice) might not display strong temperature phenotypes as extensive compensation by redundant temperature detectors takes place. In the context of heat nociception this is exemplified by the overlapping expression and temperature sensitivities of TRPV1, TRPM3 and Anoctamin 1 ().
It is important to note that other (non-TRP) ion channels are also intrinsically temperature sensitive and can modulate temperature activation of sensory neurons. In particular, it has been found that, similar to TRP channels, the 2-pore potassium channels TRAAK (KCNK4), TREK1 (KCNK2), and TREK2 (KCNK10) cover a broad range of the physiological relevant temperature range (). It has been demonstrated that these 3 2-pore channels are important for inhibiting temperature sensitive neurons, as mice deficient for these ion channels are hypersensitive to temperature stimuli.Citation22,53,54
Additionally, it is very well possible, that other, non-ion channel proteins play a role in thermosensation. For example it has been shown that a temperature stimulus (>35°C) triggers stim1 clustering with Orai ion channels to mediate store operated Ca2+ entry.Citation55 If and in what context this temperature-sensitive phenomenon plays a physiological role awaits future studies.
Peripheral DRG and TG neurons constitute the principle sensory system that relays environmental temperature information to spinal and supra-spinal CNS areas (). This, for one, allows the initiation of protective reflexes that prevent tissue damage. Additionally, this system is also required for our conscious perception of thermal stimuli that allows us to qualify these stimuli as painful (noxious), innocuous or pleasant.Citation20,56
Figure 2. Simplified cartoon depicting thermoregulatory pathways. Peripheral thermoreceptors detect environmental and visceral temperatures and report these to the hypothalamus. Hypothalamic temperature receptors detect internal temperature. The thermoregulatory center initiates heat-loss or heat-gain responses in peripheral organs.
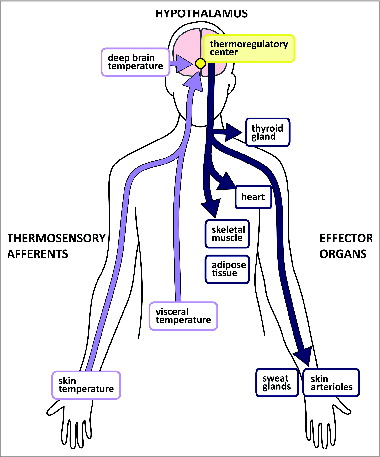
Temperature pathways branch off from the spinothalamic tract and reach (via passage of parabrachial structures) the hypothalamus -the key center for thermoregulation.Citation57 While it has been shown that peripheral temperature sensors innervating the skin contribute to thermoregulation and maintenance of temperature homeostasis,Citation58 it is less clear if other types of (interoceptive) peripheral temperature sensors provide information for thermoregulation.
Thermoregulatory Functions of TRPs
In the context of thermoregulation, we here distinguish 2 different types of mechanisms: (1) peripheral TRP channels that are activated and affect body temperature by modulating the autonomic system and (2) TRP channels in the central nervous system that directly influence the detection of CNS temperature and/or the integration of the thermoregulatory afferent signals. Since both peripheral and central pathways interact at multiple -and often unknown- levels, careful experimentation and controls are necessary to establish the roles of specific TRP channels and their actions in different parts of the nervous system.
To name one example, global TRPV1 knockout mice reveal the involvement of the ion channel in acute thermoregulation.Citation26,59 However, besides its robust and specific expression in the DRG sensory neurons, limited TRPV1 expression has been found in some brain structures and smooth muscle cells,Citation60 as well as peripheral tissues including adipocytes,Citation61 the vascular systemCitation62 and the gastrointestinal tract.Citation63 It is therefore currently unclear at what sites TRPV1 contributes to thermoregulation. Furthermore, the thermoregulatory role of TRPV1 is further confounded by its function in regulating metabolism.Citation64 Therefore, tissue-specific genetic, pharmacogenetic and/or optogenetic manipulations constitute potential useful future approaches to dissect and define the molecular mechanisms underlying thermoregulation.
We will now first focus on peripherally mediated thermoregulation.
Peripherally mediated thermoregulation
Several thermosensitive TRP ion channels do not only play an important role in detecting ambient temperature change but also in regulating core body temperature ().Citation65
Table 2. Thermoregulation
Major efforts have been made to elucidate the mechanisms by which TRPV1 and TRPM8 channels modulate Tb in the past decades.
Long before the TRPV1 channel was cloned, systemic capsaicin administration has been found to decrease the core body temperature, an effect largely due to pronounced peripheral vasodilation.Citation66 Additionally it was found that capsaicin-induced ablation of sensory fibers rendered animals sensitive to heat challenges.Citation67 After the discovery of the TRPV1 ion channel,Citation4 we obtained a deeper understanding of its role in thermoregulation only subsequent to the identification of selective antagonists. In opposite to TRPV1 activation, inhibition of the ion channel leads to hyperthermia in animal models as well as humans.Citation33 When peripherally restricted TRPV1 antagonists are administered via intragastric application, an increase of Tb is also observed in mice, dogs and humans,Citation33,68 demonstrating that TRPV1 expressed at peripheral sites contributes to thermoregulation. Several lines of evidence support the notion that tonically active TRPV1 in abdominal viscera influences core body temperature and that antagonizing TRPV1 promotes vasoconstriction, thermogenesis and modulation of thermoregulatory neurons in the hypothalamus,Citation69,70 TRPV1 antagonist-induced hyperthermia is mainly associated with autonomic cold-defense effectors without triggering the heat-seeking behavior of the animal.Citation69 Among the antagonists studied, it appeared that preferentially those inhibitors of TRPV1 trigger hyperthermia in vivo that block proton-induced activation of TRPV1.Citation71 For example, the compound JYL1421 blocks proton activation of dog and monkey TRPV1 but not of rat TRPV1.Citation68 Concomitantly, the TRPV1 inhibitor produced hyperthermia in dogs and monkeys but failed to increase body temperature in rats. Although the initial exposure to an antagonist leads to hyperthermia, the hypothermic response is blunted by repetitive dosing of the same antagonist.Citation33
These exciting pharmacological experiments implicating TRPV1 in thermoregulation were subsequently humbled by the phenotype of the TRPV1 knock-out mice:Citation26,29 TRPV1 knockout mice have a normal core body temperature compared to controls. However, it was found that the TRPV1 mutant animals display increased vasoconstriction and locomotion, which suggests that different thermoeffector organs are engaged in the knock-out animals compared to wild-type controls. When aged, the knockout mice also become obese,Citation59,72 revealing a connection of TRP ion channels to metabolic regulation.
These data are consistent with an acute and transient effect of TRPV1 on core body temperature but argue for a minor role of the ion channel in regulating core temperature at steady state or -alternatively- for robust compensatory pathways that substitute for loss of the receptor in the knock-out animals.
It is interesting to note that perception of heat pain is also not abolished in TRPV1 knockout mice and that other mechanisms and pathways are contributing to this sensory modality.Citation29 As mentioned above, heat-gated ion channels such as Anoctamin 1,Citation36 TRPM3Citation13 as well as 2-pore potassium channels contribute to the detection of painfully hot stimuli and it will be interesting to decipher whether they also influence core body temperature.
In line with capsaicin-induced hypothermia, activation of the cold receptor TRPM8, has the opposite effect to TRPV1 activation: the topical application of the TRPM8 agonist menthol triggers autonomic responses such as hyperthermia, oxygen consumption, shivering-like muscle activity and tail skin vasoconstriction as well as heat-seeking behavior.Citation73 Several selective TRPM8 antagonists have been tested in vivo. These substances induce dose-dependent hypothermia in mice and rats. The hypothermic effect is entirely TRPM8 dependent and thus is absent in TRPM8 knock-out mice.Citation74 Repetitive stimulation with a TRPM8 antagonist also results in a tachyphylactic response pattern and subsequent exposures produce a diminished hypothermic effect.Citation74 This desensitizing effect is similar to that observed for antagonizing TRPV1, albeit the net effect on Tb is oppositely directed for the 2 TRP ion channels.
Likely, this observed thermoregulatory role of TRPM8 is mediated by activation of the ion channel expressed in skin afferents.Citation73,74 Support for this notion comes from the observation that intravenous infusion of a TRPM8 antagonist decreases environmental cold-induced c-fos expression in the lateral parabrachial nucleus (LPB) —a nucleus in the CNS which receives peripheral thermosensory input and is believed to relay this information to hypothalamic thermoregulatory sites.Citation57 Possibly TRPM8 expressed at other (peripheral) sites is also contributing to acute thermoregulation. In this regard it is noteworthy that intravenous application, in opposite to intrathecal or intracerebroventricular application of the TRPM8 antagonist, is the most effective way to elicit hypothermia.Citation74 Moreover, TRPM8 is expressed in the vasculature and can modulate vasculature tone and thus may also contribute to the regulation of body temperature by modulating cardiovascular functions.Citation75 In addition, TRPM8 positive nerve fibers innervate the vasculature, such as the hepatic portal vein.Citation76 Further studies are necessary to dissect the thermoregulatory function of TRPM8 in neuronal and non-neuronal tissues.
Besides TRPM8, TRPA1 is another cold sensor candidate in the thermoregulatory pathway. Although activators (allylisothiocyanate and cinnamonaldehyde) of TRPA1 can induce changes in body temperature,Citation77 these chemicals are reactive compounds and thus not strictly TRPA1-specific and the effect might be TRPA1-independent. In addition, 2 studies based on antagonist administration and employing TRPA1 knockout mice as controls have shown that TRPA1 is not involved in mediating cold defense responses.Citation78,79
Given that TRPV3 and TRPV4 are activated by warmth, they were hypothesized to participate in thermoregulation. However, intragastric delivery of natural TRPV3 activators (thymol and ethyl vanillin) has no detectable impact on Tb or on thermal effectors such as adipose tissue.Citation80 TRPV4 knock-out mice display indistinguishable basal body temperature compared to control mice and no response to acute heat change.Citation38 So far, there is no clear evidence supporting their role in maintaining body temperature.
Up to now, our knowledge about thermoregulation is largely based on research in mammals. Little is known about other endotherms, such as birds, let alone ectotherms. However, one study has linked TRP channels to thermoregulatory behavior in reptiles, which move between sun-exposed places and cooler, shady places to maintain temperature homeostasis. This shuttling behavior of crocodiles can be blocked by capsazepine, an antagonist of TRPV1 and TRPM8 ion channels.Citation81
Central thermoregulation
The central nervous system regulates body temperature homeostasis by providing neural and hormonal controls at multiple levels. The skin, viscera and CNS provide temperature information indirectly or directly to the thermoregulatory center residing in the preoptic area and anterior part of the hypothalamus (POA/AH). This area is believed to compute the temperature information and to send signals either directly or via relay stations in more caudal parts of the hypothalamus, to multiple effector organs. A change of body temperature may occur either via neural control of the autonomic system and/or hormonal responses (). Several reviews have summarized neural circuits underlying thermoregulation in the context of physiological and inflammatory (fever) conditions.Citation82,83 Among the best-mapped pathways is the neural modulation of non-shivering thermogenesis: primary somatosensory neurons, whose somata are located in the DRGs, detect a change in ambient temperature. Cutaneous thermal information is relayed via the lateral parabrachial nucleus (LPB) to the preoptic area. In the hypothalamic thermoregulatory center output signals are generated and delivered to the rostral raphe pallidus (rRPa) via multisynaptic relays. The rRPa neurons further extend projections to the sympathetic preganglionic neurons in the spinal cord. Preganglionic neurons modulate sympathetic outflow to regulate and maintain temperature homeostasis. However, little is known about the detailed circuitry in the hypothalamus and the mechanisms that compute temperature information to generate appropriate outputs.
Among all the areas involved in thermoregulation, the preoptic area/anterior hypothalamus (POA/AH) is believed to be a key site for maintaining temperature homeostasis. It does not only integrate temperature information from peripheral sites but also harbors multiple types of temperature-sensitive neurons. The findings demonstrating a regulatory role for POA/AH in temperature homeostasis, have been covered in excellent reviews by others.Citation57,84,85 Most importantly, modulation of the activity of warm sensitive neurons (WSNs), by directly cooling or heating of the POA elicits heat conservation/production or heat loss phenomena, respectively.Citation85 Warm-sensitivity of the WSNs appears to be an intrinsic, cell autonomous property: temperature-triggered electrophysiological responses remain even after blockage of synaptic inputs.Citation86
It is tempting to speculate that certain thermo-sensitive TRP ion channels mediate temperature responses in POA/AH WSNs in a similar fashion as they have been shown to mediate thermosensitivity in DRG sensory neurons. While Ionic currents have been measured in WSNs,Citation87,88 we currently don't know if (or which) TRP ion channels are expressed in WSNs and whether any TRP ion channel mediates (or modulates) temperature responses in hypothalamic neurons. Currently, the absence of suitable molecular markers for hypothalamic WSNs renders this type of neuronal population difficult to study.
It is possible that other ion channels, such as ATP-sensitive potassium channels,Citation89 temperature-sensitive 2-pore channelsCitation90 or the interplay of different temperature-sensitive ion channels are mediating temperature responses in WSNs.Citation85
Once we are able to molecularly identify WSNs, we will be able to (i) address their mechanism of temperature sensitivity and (ii) examine whether their temperature sensitivity is physiological relevant for thermoregulation.
Even if TRP channels turn out not to be direct temperature sensors in WSNs, some interesting observations correlating TRP channel expression with hypothalamic thermoregulatory function have been obtained. In LPS-induced fever, heat shock factor 1 (HSF1) was shown to bind to the 5' promoter region of TRPV1, thereby up-regulating TRPV1 expression in the preoptic area.Citation91 TRPV1 expression was also found to be induced in the processes of astrocytes in the circumventricularorgans (CVO) where the blood brain barrier (BBB) is ‘leaky’. This possibility allows TRPV1-generated signals to be relayed into the CNS. Indeed, activation of these TRPV1 channels by intravenous or intracerebroventricular infusion of agonists leads to increased c-fos expression in the preopic area, indicating its potential role in thermoregulation.Citation92 Additionally, expression of a TRPV1 variant insensitive to capsaicin stimulation has been implicated in temperature responses of hypothalamic magnocellular neurons that are crucial for osmoregulation.Citation93–95 However, it is presently unclear whether these findings have any implication for thermoregulation. Instead it has been suggested that this TRPV1 variant might play a role in osmosensing.Citation96,97
Punctuated staining of TRPV4 is observed in the neuropil of the preoptic area and anterior hypothalamus.Citation98 Although the molecular identity of WSNs remain unknown, a recent study reveals that about 2 thirds of parvalbumin (PV) positive neurons in the anterior hypothalamus area can be activated by heat and the other remaining neurons are inhibited by heat. Strikingly, more than half of the PV cells express TRPV4 and the majority of them also express TRPM8.Citation99 Currently this data is correlative and it is unclear whether TRPV4 and/or TRPM8 mediate temperature sensitivity of the identified hypothalamic neuron population.
Additionally, the transcripts of thermosensitive TRP channels (TRPA1, TRPM8, TRPV1, TRPV2, TRPV3 and TRPV4) can be found in the hypothalamus.Citation100 The precise expression pattern and function in thermoregulation await further study.
Metabolic Functions of TRPs
Temperature regulation and metabolic control are intimately related. Any metabolic reaction is accelerated at higher temperature. A growing body of evidence suggests that sensory systems are intricately involved in regulation metabolic homeostasis.Citation101 Since many TRP channels are functionally implicated in sensory systems, it is thus not surprising to discover their direct (or indirect) modulatory role in the context of metabolic regulation (). Moreover, TRP channels are expressed in thermoregulatory effector organs, such as adipose tissue, muscle, vasculature and gastrointestinal tract, thereby potentially modulating the metabolic status.
Table 3. Metabolism
TRPV1
TRPV1 has been associated with both beneficial and detrimental effects on metabolism.
Administering the TRPV1 agonist capsaicin as dietary supplement, prevents mice from diet-induced obesity (DIO).Citation61 Adipose tissue isolated from obese mice and humans displays reduced TRPV1 expression.Citation61 TRPV1 knockout mice develop age-associated obesity and hypometabolism.Citation59 Many of these beneficial effects of TRPV1 in antagonizing obesity have been reviewed by Zsombok.Citation102 However, recent reviews have pointed out that the beneficial effects are likely to be subtle and would probably require long-term consumption of TRPV1 agonists.Citation103,104
Somewhat counter intuitive based on the aforementioned results, another study finds TRPV1 knock-out mice to be protected from DIO.Citation105 This study details an interesting feature of TRPV1 knockout mice: they exhibit an increased life span which correlates with the maintenance of a juvenile metabolic profile. It turns out that TRPV1 knock-out mice maintain low CGRP release in the sensory afferents innervating the pancreas which promotes insulin secretion. This neuroendocrine regulation is achieved by preventing the CREBregulated transcription coactivator 1 from entering the nucleus, resulting in reduced CREB levels in the DRG neurons and reduced CGRP secretion.Citation64
Besides the pancreas, TRPV1 channels are believed to exert their multifaceted metabolic effects in various tissues, such as hypothalamus, adipose tissue and gastrointestinal tract.Citation63,106 However, putative molecular mechanisms underlying protective functions in these tissues remain unclear. Collectively, depending on the tissue type –and likely also depending on the metabolic state– both activating and inhibiting TRPV1 appear to be useful to positively influence metabolic homeostasis. These somewhat contradictory results of TRPV1's effect on metabolism maybe reconciled by a potential switch in TRPV1 function from the beneficial effects the receptor has in young animals to a more pathological role it adopts in aged animals.Citation107 An alternative explanation how these different results might be reconciled is that dietary activation of TRPV1 might mediate its positive effects by desensitizing the receptor, thereby inhibiting excessive TRPV1 activity. This type of beneficial effect would be similar to the desensitization potential of TRPV1 that is exploited in locally restricted and surface-accessible forms of inflammatory pain: local application of Capsaicin lotions or patches desensitizes (and even de-innervates) TRPV1-positive primary afferent nociceptors to produce analgesia.Citation108,109 Future work is required to elucidate how the balance of TRPV1 activity in different organs affects metabolic and cardiovascular parameters to bring about health benefits.
TRPM8
TRPM8 positive afferents innervate the hepatic portal vein. This may serve as a mechanism by which TRPM8 regulates the insulin clearance rate in mice.Citation76 Similar to TRPV1, the TRPM8 channel is expressed in adipocytes.Citation110,111 Menthol induces up-regulation of UCP-1 in brown adipose tissue (BAT) in a dose-dependent manner in WT but not in TRPM8 KO mice. Moreover, prolonged dietary menthol supplements protect mice from DIO. The effect is dependent on TRPM8 as the effect is absent in TRPM8 KO mice.Citation111 In humans, TRPM8 activation has been associated with ‘browning’ of white adipose tissue thereby possibly promoting energy utilization.Citation110
TRPV4
TRPV4 KO mice are protected from DIO. Loss of TRPV4 initiates a compensatory regulatory mechanism in skeletal muscles and results in the increase of oxidative potential in the tissue.Citation112 Another team of researchers also observes the beneficial effects of TRPV4 ablation.Citation113 However, they find that TRPV4 is expressed in adipose tissue and promotes the expression of proinflammatory cytokines. Antagonizing TRPV4 by pharmacologic blockade improves glucose homeostasis in mice.Citation113
TRPM2
TRPM2 KO mice are also protected from DIO. The TRPM2 knockout mice are more sensitive to insulin, partially due to higher glucose turnover rate in peripheral tissues.Citation114 In line with its specific expression in the pancreatic β cells,Citation12 TRPM2-deficient mice also have impaired insulin production under both normal chow and high-fat diet conditions.Citation115 TRPM2 is widely expressed in multiple organs, including brain, heart, kidney and the immune system, where it has been suggested to act as oxidative stress sensor.Citation116–120 Defining its functional role in these diverse tissues awaits further studies.
Disclosure of Potential Conflicts of Interest
No potential conflicts of interest were disclosed.
Funding
J.S. is supported by the European Research Council (ERC-2011-StG-280565) and the Alexander von Humboldt Foundation/BMBF (Sofja Kovalevskaja Award).
References
- Montell C, Rubin G. Molecular characterization of the Drosophila trp locus: a putative integral membrane protein required for phototransduction. Neuron 1989; 2:1313-23; PMID:2516726; http://dx.doi.org/10.1016/0896-6273(89)90069-X
- Owsianik G, D'hoedt D, Voets T, Nilius B. Structure-function relationship of the TRP channel superfamily. Rev Physiol Biochem Pharmacol 2006; 156:61-90; PMID:16634147
- Vriens J, Nilius, B, Voets T. Peripheral thermosensation in mammals. Nat Rev Neuroscience 2014; 15:573-89; PMID:25053448
- Caterina M, Schumacher MA, Tominaga M, Rosen TA, Levine JD, Julius D. The capsaicin receptor: a heat-activated ion channel in the pain pathway. Nature 1997; 389:816-24; PMID:9349813; http://dx.doi.org/10.1038/39807
- Caterina M, Rosen T, Tominaga M, Brake A, Julius D. A capsaicin-receptor homologue with a high threshold for noxious heat. Nature 1999; 398:436-41; PMID:10201375; http://dx.doi.org/10.1038/18906
- Güler A, Lee H, Iida T, Shimizu I, Tominaga M, Caterina M. Heat-evoked activation of the ion channel, TRPV4. J Neurosci 2002; 22:6408-14; PMID:12151520
- Peier A, Reeve AJ, Andersson DA, Moqrich A, Earley TJ, Hergarden AC, Story GM, Colley S, Hogenesch JB, McIntyre P, et al. A heat-sensitive TRP channel expressed in keratinocytes. Science 2002; 296:2046-9; PMID:12016205; http://dx.doi.org/10.1126/science.1073140
- López B. Beyond modularisation: the need of a socio-neuro-constructionist model of autism. J Autism Dev Disord 2015; 45:31-41; PMID:24154760; http://dx.doi.org/10.1007/s10803-013-1966-9
- Smith G, Gunthorpe MJ, Kelsell RE, Hayes PD, Reilly P, Facer P, Wright JE, Jerman JC, Walhin JP, Ooi L, et al. TRPV3 is a temperature-sensitive vanilloid receptor-like protein. Nature 2002; 418:186-90; PMID:12077606; http://dx.doi.org/10.1038/nature00894
- Xu H, Ramsey IS, Kotecha SA, Moran MM, Chong JA, Lawson D, Ge P, Lilly J, Silos-Santiago I, Xie Y, et al. TRPV3 is a calcium-permeable temperature-sensitive cation channel. Nature 2002; 418:181-6; PMID:12077604; http://dx.doi.org/10.1038/nature00882
- Talavera K, Yasumatsu K, Voets T, Droogmans G, Shigemura N, Ninomiya Y, Margolskee RF, Nilius B. Heat activation of TRPM5 underlies thermal sensitivity of sweet taste. Nature 2005; 438:1022-5; PMID:16355226
- Togashi K, Hara Y, Tominaga T, Higashi T, Konishi Y, Mori Y, Tominaga M. TRPM2 activation by cyclic ADP-ribose at body temperature is involved in insulin secretion. EMBO J 2006; 25:1804-15; PMID:16601673; http://dx.doi.org/10.1038/sj.emboj.7601083
- Vriens J, Owsianik G, Hofmann T, Philipp SE, Stab J, Chen X, Benoit M, Xue F, Janssens A, Kerselaers S, et al. TRPM3 is a nociceptor channel involved in the detection of noxious heat. Neuron 2011; 70:482-94; PMID:21555074; http://dx.doi.org/10.1016/j.neuron.2011.02.051
- McKemy D, Neuhausser W, Julius D. Identification of a cold receptor reveals a general role for TRP channels in thermosensation. Nature 2002; 416:52-8; PMID:11882888; http://dx.doi.org/10.1038/nature719
- Peier A, Moqrich A, Hergarden AC, Reeve AJ, Andersson DA, Story GM, Earley TJ, Dragoni I, McIntyre P, Bevan S, et al. A TRP channel that senses cold stimuli and menthol. Cell 2002; 108:705-15; PMID:11893340; http://dx.doi.org/10.1016/S0092-8674(02)00652-9
- Basbaum A, Bautista D, Scherrer G, Julius D. Cellular and molecular mechanisms of pain. Cell 2009; 139:267-84; PMID:19837031; http://dx.doi.org/10.1016/j.cell.2009.09.028
- Bautista D, Siemens J, Glazer JM, Tsuruda PR, Basbaum AI, Stucky CL, Jordt SE, Julius D. The menthol receptor TRPM8 is the principal detector of environmental cold. Nature 2007; 448:204-8; PMID:17538622; http://dx.doi.org/10.1038/nature05910
- Colburn R, Lubin ML, Stone DJ Jr, Wang Y, Lawrence D, D'Andrea MR, Brandt MR, Liu Y, Flores CM, Qin N. Attenuated cold sensitivity in TRPM8 null mice. Neuron 2007; 54:379-86; PMID:17481392; http://dx.doi.org/10.1016/j.neuron.2007.04.017
- Dhaka A, Murray AN, Mathur J, Earley TJ, Petrus MJ, Patapoutian A. TRPM8 is required for cold sensation in mice. Neuron 2007; 54:371-8; PMID:17481391; http://dx.doi.org/10.1016/j.neuron.2007.02.024
- Milenkovic N, Zhao WJ, Walcher J, Albert T, Siemens J, Lewin GR, Poulet JF. A somatosensory circuit for cooling perception in mice. Nat Neurosci 2014; 17(11):1560-6; PMID:25262494; http://dx.doi.org/10.1038/nn.3828
- Parra A, Madrid R, Echevarria D, del Olmo S, Morenilla-Palao C, Acosta MC, Gallar J, Dhaka A, Viana F, Belmonte C. Ocular surface wetness is regulated by TRPM8-dependent cold thermoreceptors of the cornea. Nat Med 2010; 16:1396-9; PMID:21076394; http://dx.doi.org/10.1038/nm.2264
- Morenilla-Palao C, Luis E, Fernández-Peña C, Quintero E, Weaver JL, Bayliss DA, Viana F. Ion channel profile of TRPM8 cold receptors reveals a role of TASK-3 potassium channels in thermosensation. Cell reports 2014; 8:1571-82; PMID:25199828; http://dx.doi.org/10.1016/j.celrep.2014.08.003
- Madrid R, Peña E, Donovan-Rodriguez T, Belmonte C, Viana F. Variable threshold of trigeminal cold-thermosensitive neurons is determined by a balance between TRPM8 and Kv1 potassium channels. J Neurosci 2009; 29:3120-31; PMID:19279249; http://dx.doi.org/10.1523/JNEUROSCI.4778-08.2009
- Vetter I, Hein A, Sattler S, Hessler S, Touska F, Bressan E, Parra A, Hager U, Leffler A, Boukalova S, et al. Amplified cold transduction in native nociceptors by M-channel inhibition. J Neurosci 2013; 33:16627-41; PMID:24133266; http://dx.doi.org/10.1523/JNEUROSCI.1473-13.2013
- Zimmermann K, Lennerz JK, Hein A, Link AS, Kaczmarek JS, Delling M, Uysal S, Pfeifer JD, Riccio A, Clapham DE. Transient receptor potential cation channel, subfamily C, member 5 (TRPC5) is a cold-transducer in the peripheral nervous system. Proc Natl Acad Sci U S A 2011; 108:18114-9; PMID:22025699; http://dx.doi.org/10.1073/pnas.1115387108
- Caterina M, Leffler A, Malmberg AB, Martin WJ, Trafton J, Petersen-Zeitz KR, Koltzenburg M, Basbaum AI, Julius D. Impaired nociception and pain sensation in mice lacking the capsaicin receptor. Science 2000; 288, 306-13; PMID:10764638; http://dx.doi.org/10.1126/science.288.5464.306
- Rowbotham M, Nothaft W, Duan WR, Wang Y, Faltynek C, McGaraughty S, Chu KL, Svensson P. Oral and cutaneous thermosensory profile of selective TRPV1 inhibition by ABT-102 in a randomized healthy volunteer trial. Pain 2011; 152:1192-200; PMID:21377273; http://dx.doi.org/10.1016/j.pain.2011.01.051
- Szallasi A, Sheta M. Targeting TRPV1 for pain relief: limits, losers and laurels. Expert Opin Investig Drugs 2012; 21:1351-69; PMID:22780443; http://dx.doi.org/10.1517/13543784.2012.704021
- Davis J, Gray J, Gunthorpe MJ, Hatcher JP, Davey PT, Overend P, Harries MH, Latcham J, Clapham C, Atkinson K, et al. Vanilloid receptor-1 is essential for inflammatory thermal hyperalgesia. Nature 2000; 405:183-7; PMID:10821274; http://dx.doi.org/10.1038/35012076
- Gracheva E, Cordero-Morales JF, González-Carcacía JA, Ingolia NT, Manno C, Aranguren CI, Weissman JS, Julius D. Ganglion-specific splicing of TRPV1 underlies infrared sensation in vampire bats. Nature 2011; 476:88-91; PMID:21814281; http://dx.doi.org/10.1038/nature10245
- Huang J, Zhang X, McNaughton, P. Inflammatory pain: the cellular basis of heat hyperalgesia. Curr Neuropharmacol 2006; 4:197-206; PMID:18615146; http://dx.doi.org/10.2174/157015906778019554
- Tominaga M, Caterina MJ, Malmberg AB, Rosen TA, Gilbert H, Skinner K, Raumann BE, Basbaum AI, Julius D. The cloned capsaicin receptor integrates multiple pain-producing stimuli. Neuron 1998; 21:531-43; PMID:9768840; http://dx.doi.org/10.1016/S0896-6273(00)80564-4
- Gavva N, Treanor JJ, Garami A, Fang L, Surapaneni S, Akrami A, Alvarez F, Bak A, Darling M, Gore A, et al. Pharmacological blockade of the vanilloid receptor TRPV1 elicits marked hyperthermia in humans. Pain 2008; 136:202-10; PMID:18337008; http://dx.doi.org/10.1016/j.pain.2008.01.024
- Wong G, Gavva N. Therapeutic potential of vanilloid receptor TRPV1 agonists and antagonists as analgesics: recent advances and setbacks. Brain Res Rev 2009; 60:267-77; PMID:19150372; http://dx.doi.org/10.1016/j.brainresrev.2008.12.006
- Hanack C, Moroni M, Lima WC, Wende H, Kirchner M, Adelfinger L, Schrenk-Siemens K, Tappe-Theodor A, Wetzel C, Kuich PH, et al. GABA blocks pathological but not acute TRPV1 pain signals. Cell 2015; 160:759-70; PMID:25679765; http://dx.doi.org/10.1016/j.cell.2015.01.022
- Cho H, Yang YD, Lee J, Lee B, Kim T, Jang Y, Back SK, Na HS, Harfe BD, Wang F, et al. The calcium-activated chloride channel anoctamin 1 acts as a heat sensor in nociceptive neurons. Nat Neurosci 2012; 15:1015-21; PMID:22634729; http://dx.doi.org/10.1038/nn.3111
- Lee B, Cho H, Jung J, Yang YD, Yang DJ, Oh U. Anoctamin 1 contributes to inflammatory and nerve-injury induced hypersensitivity. Mol Pain 2014; 10:5; PMID:24450308; http://dx.doi.org/10.1186/1744-8069-10-5
- Lee H, Iida T, Mizuno A, Suzuki M, Caterina M. Altered thermal selection behavior in mice lacking transient receptor potential vanilloid 4. J Neurosci 2005 25:1304-10; PMID:15689568; http://dx.doi.org/10.1523/JNEUROSCI.4745.04.2005
- Moqrich A, Hwang SW, Earley TJ, Petrus MJ, Murray AN, Spencer KS, Andahazy M, Story GM, Patapoutian A. Impaired thermosensation in mice lacking TRPV3, a heat and camphor sensor in the skin. Science 2005; 307:1468-72; PMID:15746429; http://dx.doi.org/10.1126/science.1108609
- Huang S, Li X, Yu Y, Wang J, Caterina M. TRPV3 and TRPV4 ion channels are not major contributors to mouse heat sensation. Mol Pain 2011; 7:37; PMID:21586160; http://dx.doi.org/10.1186/1744-8069-7-37
- Miyamoto T, Petrus M, Dubin A, Patapoutian A. TRPV3 regulates nitric oxide synthase-independent nitric oxide synthesis in the skin. Nat Commun 2011; 2:369; PMID:21712817; http://dx.doi.org/10.1038/ncomms1371
- Park U, Vastani N, Guan Y, Raja SN, Koltzenburg M, Caterina MJ. TRP vanilloid 2 knock-out mice are susceptible to perinatal lethality but display normal thermal and mechanical nociception. J Neurosci 2011; 31:11425-36; PMID:21832173; http://dx.doi.org/10.1523/JNEUROSCI.1384-09.2011
- Kwan K, Allchorne AJ, Vollrath MA, Christensen AP, Zhang DS, Woolf CJ, Corey DP. TRPA1 contributes to cold, mechanical, and chemical nociception but is not essential for hair-cell transduction. Neuron 2006; 50:277-89; PMID:16630838; http://dx.doi.org/10.1016/j.neuron.2006.03.042
- Karashima Y, Talavera K, Everaerts W, Janssens A, Kwan KY, Vennekens R, Nilius B, Voets T. TRPA1 acts as a cold sensor in vitro and in vivo. Proc Natl Acad Sci U S A 2009; 106:1273-8; PMID:19144922; http://dx.doi.org/10.1073/pnas.0808487106
- Bautista D, Jordt SE, Nikai T, Tsuruda PR, Read AJ, Poblete J, Yamoah EN, Basbaum AI, Julius D. TRPA1 mediates the inflammatory actions of environmental irritants and proalgesic agents. Cell 2006; 124:1269-82; PMID:16564016; http://dx.doi.org/10.1016/j.cell.2006.02.023
- Knowlton W, Bifolck-Fisher A, Bautista D, McKemy D. TRPM8, but not TRPA1, is required for neural and behavioral responses to acute noxious cold temperatures and cold-mimetics in vivo. Pain 2010; 150:340-50; PMID:20542379; http://dx.doi.org/10.1016/j.pain.2010.05.021
- Wang H, Schupp M, Zurborg S, Heppenstall P. Residues in the pore region of Drosophila TRPA1 dictate sensitivity to thermal stimuli. J Physiol 2012; 591:185-201; http://dx.doi.org/10.1113/jphysiol.2012.242842
- Rosenzweig M, Brennan KM, Tayler TD, Phelps PO, Patapoutian A, Garrity PA. The Drosophila ortholog of vertebrate TRPA1 regulates thermotaxis. Genes Dev 2005; 19:419-24; PMID:15681611; http://dx.doi.org/10.1101/gad.1278205
- Viswanath V, Story GM, Peier AM, Petrus MJ, Lee VM, Hwang SW, Patapoutian A, Jegla T. Opposite thermosensor in fruitfly and mouse. Nature 2003; 423:822-3; PMID:12815418; http://dx.doi.org/10.1038/423822a
- Gracheva E, Ingolia NT, Kelly YM, Cordero-Morales JF, Hollopeter G, Chesler AT, Sánchez EE, Perez JC, Weissman JS, Julius D. Molecular basis of infrared detection by snakes. Nature 2010; 464:1006-11; PMID:20228791; http://dx.doi.org/10.1038/nature08943
- Palkar R, Lippoldt E, McKemy D. The molecular and cellular basis of thermosensation in mammals. Curr Opin Neurobiol 2015; 34C:14-19; PMID:25622298; http://dx.doi.org/10.1016/j.conb.2015.01.010
- Vay L, Gu C, McNaughton P. The thermo-TRP ion channel family: properties and therapeutic implications. Br J Pharmacol 2012; 165:787-801; PMID:21797839; http://dx.doi.org/10.1111/j.1476-5381.2011.01601.x
- Noël J, Zimmermann K, Busserolles J, Deval E, Alloui A, Diochot S, Guy N, Borsotto M, Reeh P, Eschalier A, et al. The mechano-activated K+ channels TRAAK and TREK-1 control both warm and cold perception. EMBO J 2009; 28:1308-18; PMID:19279663; http://dx.doi.org/10.1038/emboj.2009.57
- Pereira V, Busserolles J, Christin M, Devilliers M, Poupon L, Legha W, Alloui A, Aissouni Y, Bourinet E, Lesage F, et al. Role of the TREK2 potassium channel in cold and warm thermosensation and in pain perception. Pain 2014; 155:2534-44; PMID:25239074; http://dx.doi.org/10.1016/j.pain.2014.09.013
- Xiao B, Coste B, Mathur J, Patapoutian A. Temperature-dependent STIM1 activation induces Ca2+ influx and modulates gene expression. Nat Chem Biol 2011; 7:351-8; PMID:21499266; http://dx.doi.org/10.1038/nchembio.558
- Craig A. How do you feel? Interoception: the sense of the physiological condition of the body. Nat Rev Neurosci 2002; 3:655-66; PMID:12154366; http://dx.doi.org/10.1038/nrn894
- Morrison SF, Nakamura K. Central neural pathways for thermoregulation. Front Biosci (Landmark Ed) 2011; 16:74-104; PMID:21196160; http://dx.doi.org/10.2741/3677
- Romanovsky AA. Skin temperature: its role in thermoregulation. Acta Physiol 2014; 210(3):498-507; PMID:24716231; http://dx.doi.org/10.1111/apha.12231
- Garami A, Pakai E, Oliveira DL, Steiner AA, Wanner SP, Almeida MC, Lesnikov VA, Gavva NR, Romanovsky AA. Thermoregulatory phenotype of the Trpv1 knockout mouse: thermoeffector dysbalance with hyperkinesis. J Neurosci 2011; 31:1721-33; PMID:21289181; http://dx.doi.org/10.1523/JNEUROSCI.4671-10.2011
- Cavanaugh D, Chesler AT, Jackson AC, Sigal YM, Yamanaka H, Grant R, O'Donnell D, Nicoll RA, Shah NM, Julius D, et al. Trpv1 reporter mice reveal highly restricted brain distribution and functional expression in arteriolar smooth muscle cells. J Neurosci 2011; 31:5067-77; PMID:21451044; http://dx.doi.org/10.1523/JNEUROSCI.6451-10.2011
- Zhang LL, Yan Liu D, Ma LQ, Luo ZD, Cao TB, Zhong J, Yan ZC, Wang LJ, Zhao ZG, Zhu SJ, et al. Activation of transient receptor potential vanilloid type-1 channel prevents adipogenesis and obesity. Circ Res 2007; 100:1063-70; PMID:17347480; http://dx.doi.org/10.1161/01.RES.0000262653.84850.8b
- Tóth A, Czikora A, Pásztor ET, Dienes B, Bai P, Csernoch L, Rutkai I, Csató V, Mányiné IS, Pórszász R, et al. Vanilloid receptor-1 (TRPV1) expression and function in the vasculature of the rat. J Histochem Cytochem 2014; 62:129-44; PMID:24217926; http://dx.doi.org/10.1369/0022155413513589
- Wang P, Yan Z, Zhong J, Chen J, Ni Y, Li L, Ma L, Zhao Z, Liu D, Zhu Z. Transient receptor potential vanilloid 1 activation enhances gut glucagon-like peptide-1 secretion and improves glucose homeostasis. Diabetes 2012; 61:2155-65; PMID:22664955; http://dx.doi.org/10.2337/db11-1503
- Riera CE, Huising MO, Follett P, Leblanc M, Halloran J, Van Andel R, de Magalhaes Filho CD, Merkwirth C, Dillin A. TRPV1 pain receptors regulate longevity and metabolism by neuropeptide signaling. Cell 2014; 157:1023-36; PMID:24855942; http://dx.doi.org/10.1016/j.cell.2014.03.051
- Caterina M. Transient receptor potential ion channels as participants in thermosensation and thermoregulation. Am J Physiol Regul Integr Comp Physiol 2007; 292:R64-76; PMID:16973931; http://dx.doi.org/10.1152/ajpregu.00446.2006
- Jancsó-Gábor A, Szolcsányi J, Jancsó N. Irreversible impairment of thermoregulation induced by capsaicin and similar pungent substances in rats and guinea-pigs. J Physiol 1970; 206:495-507; PMID:5498502; http://dx.doi.org/10.1113/jphysiol.1970.sp009027
- Szelényi Z, Hummel Z, Szolcsányi J, Davis J. Daily body temperature rhythm and heat tolerance in TRPV1 knockout and capsaicin pretreated mice. Eur J Neurosci 2004; 19:1421-4; PMID:15016100; http://dx.doi.org/10.1111/j.1460-9568.2004.03221.x
- Gavva N, Bannon AW, Surapaneni S, Hovland DN Jr, Lehto SG, Gore A, Juan T, Deng H, Han B, Klionsky L, et al. The vanilloid receptor TRPV1 is tonically activated in vivo and involved in body temperature regulation. J Neurosci 2007; 27:3366-74; PMID:17392452; http://dx.doi.org/10.1523/JNEUROSCI.4833-06.2007
- Steiner A, Turek VF, Almeida MC, Burmeister JJ, Oliveira DL, Roberts JL, Bannon AW, Norman MH, Louis JC, Treanor JJ, et al. Nonthermal activation of transient receptor potential vanilloid-1 channels in abdominal viscera tonically inhibits autonomic cold-defense effectors. J Neurosci 2007; 27:7459-68; PMID:17626206; http://dx.doi.org/10.1523/JNEUROSCI.1483-07.2007
- McGaraughty S, Segreti J, Fryer R, Brown B. Antagonism of TRPV1 receptors indirectly modulates activity of thermoregulatory neurons in the medial preoptic area of rats. Brain Res 2009; 1268:58-67; PMID:19236852.
- Garami A, Shimansky YP, Pakai E, Oliveira DL, Gavva NR, Romanovsky AA. Contributions of different modes of TRPV1 activation to TRPV1 antagonist-induced hyperthermia. J Neurosci 2010; 30:1435-40; PMID:20107070; http://dx.doi.org/10.1523/JNEUROSCI.5150-09.2010
- Iida T, Shimizu I, Nealen ML, Campbell A, Caterina M. Attenuated fever response in mice lacking TRPV1. Neurosci Lett 2005; 378:28-33; PMID:15763167; http://dx.doi.org/10.1016/j.neulet.2004.12.007
- Tajino K, Matsumura K, Kosada K, Shibakusa T, Inoue K, Fushiki T, Hosokawa H, Kobayashi S. Application of menthol to the skin of whole trunk in mice induces autonomic and behavioral heat-gain responses. Am J Physiol Regul Integr Comp Physiol 2007; 293:R2128-35; PMID:17761510; http://dx.doi.org/10.1152/ajpregu.00377.2007
- Almeida MC, Hew-Butler T, Soriano RN, Rao S, Wang W, Wang J, Tamayo N, Oliveira DL, Nucci TB, Aryal P, et al. Pharmacological blockade of the cold receptor TRPM8 attenuates autonomic and behavioral cold defenses and decreases deep body temperature. J Neurosci 2012; 32:2086-99; PMID:22323721; http://dx.doi.org/10.1523/JNEUROSCI.5606-11.2012
- Johnson CD, Melanaphy D, Purse A, Stokesberry SA, Dickson P, Zholos AV. Transient receptor potential melastatin 8 channel involvement in the regulation of vascular tone. Am J Physiol Heart Circ Physiol 2009; 296:H1868-77; PMID:19363131; http://dx.doi.org/10.1152/ajpheart.01112.2008
- McCoy DD, Zhou L, Nguyen AK, Watts AG, Donovan CM, McKemy DD. Enhanced insulin clearance in mice lacking TRPM8 channels. Am J Physiol Endocrinol Metab 2013; 305:E78-88; PMID:23651844; http://dx.doi.org/10.1152/ajpendo.00542.2012
- Mori N, Kawabata F, Matsumura S, Hosokawa H, Kobayashi S, Inoue K, Fushiki T. Intragastric administration of allyl isothiocyanate increases carbohydrate oxidation via TRPV1 but not TRPA1 in mice. Am J Physiol Regul Integr Comp Physiol 2011; 300:R1494-505; PMID:21430076; http://dx.doi.org/10.1152/ajpregu.00645.2009
- Chen J, Joshi SK, DiDomenico S, Perner RJ, Mikusa JP, Gauvin DM, Segreti JA, Han P, Zhang XF, Niforatos W, et al. Selective blockade of TRPA1 channel attenuates pathological pain without altering noxious cold sensation or body temperature regulation. Pain 2011; 152:1165-72; PMID:21402443; http://dx.doi.org/10.1016/j.pain.2011.01.049
- Oliveira C, Garami A, Lehto SG, Pakai E, Tekus V, Pohoczky K, Youngblood BD, Wang W, Kort ME, Kym PR, et al. Transient receptor potential channel ankyrin-1 is not a cold sensor for autonomic thermoregulation in rodents. J Neurosci 2014; 34:4445-52; PMID:24671991; http://dx.doi.org/10.1523/JNEUROSCI.5387-13.2014
- Masamoto Y, Kawabata F, Fushiki T. Intragastric administration of TRPV1, TRPV3, TRPM8, and TRPA1 agonists modulates autonomic thermoregulation in different manners in mice. Biosci Biotechnol Biochem 2009; 73:1021-7; PMID:19420725; http://dx.doi.org/10.1271/bbb.80796
- Seebacher F, Murray SA. Transient receptor potential ion channels control thermoregulatory behaviour in reptiles. PLoS One 2007; 2:e281; PMID:17356692; http://dx.doi.org/10.1371/journal.pone.0000281
- Romanovsky A, Almeida MC, Garami A, Steiner AA, Norman MH, Morrison SF, Nakamura K, Burmeister JJ, Nucci TB. The transient receptor potential vanilloid-1 channel in thermoregulation: a thermosensor it is not. Pharmacol Rev 2009; 61:228-61; PMID:19749171; http://dx.doi.org/10.1124/pr.109.001263
- Nakamura K. Central circuitries for body temperature regulation and fever. Am J Physiol Regul Integr Comp Physiol 2011; 301:R1207-28; PMID:21900642; http://dx.doi.org/10.1152/ajpregu.00109.2011
- Morrison SF, Nakamura K, Madden CJ. Central control of thermogenesis in mammals. Exp Physiol 2008; 93:773-97; PMID:18469069; http://dx.doi.org/10.1113/expphysiol.2007.041848
- Boulant J. Role of the preoptic-anterior hypothalamus in thermoregulation and fever. Clin Infect Dis 2000; 5:S157-61; PMID:11113018; http://dx.doi.org/10.1086/317521
- Kelso S, Boulant J. Effect of synaptic blockade on thermosensitive neurons in hypothalamic tissue slices. Am J Physiol 1982; 243:R480-90; PMID:7137377
- Griffin J, Boulant J. Temperature effects on membrane potential and input resistance in rat hypothalamic neurones. J Physiol 1995; 488:407-18; PMID:8568679; http://dx.doi.org/10.1113/jphysiol.1995.sp020975
- Zhao Y, Boulant J. Temperature effects on neuronal membrane potentials and inward currents in rat hypothalamic tissue slices. J Physiol 2005; 564:245-57; PMID:15695248; http://dx.doi.org/10.1113/jphysiol.2004.075473
- Parsons M, Belanger-Willoughby N, Linehan V, Hirasawa M. ATP-sensitive potassium channels mediate the thermosensory response of orexin neurons. J Physiol 2012; 590:4707-15; PMID:22802589; http://dx.doi.org/10.1113/jphysiol.2012.236497
- Patel A, Honoré E. Properties and modulation of mammalian 2P domain K+ channels. Trends Neurosci 2001; 24:339-46; PMID:11356506; http://dx.doi.org/10.1016/S0166-2236(00)01810-5
- Fan-xin M, Li-mei S, Bei S, Xin Q, Yu Y, Yu C. Heat shock factor 1 regulates the expression of the TRPV1 gene in the rat preoptic-anterior hypothalamus area during lipopolysaccharide-induced fever. Exp Physiol 2012; 97:730-40; PMID:22427437; http://dx.doi.org/10.1113/expphysiol.2011.064204
- Mannari T, Morita S, Furube E, Tominaga M, Miyata S. Astrocytic TRPV1 ion channels detect blood-borne signals in the sensory circumventricular organs of adult mouse brains. Glia 2013; 61:957-71; PMID:23468425; http://dx.doi.org/10.1002/glia.22488
- Sudbury J, Ciura S, Sharif-Naeini R, Bourque C. Osmotic and thermal control of magnocellular neurosecretory neurons-role of an N-terminal variant of trpv1. Eur J Neurosci 2010; 32:2022-30; PMID:21143657; http://dx.doi.org/10.1111/j.1460-9568.2010.07512.x
- Sudbury J, Bourque C. Dynamic and permissive roles of TRPV1 and TRPV4 channels for thermosensation in mouse supraoptic magnocellular neurosecretory neurons. J Neurosci 2013; 33:17160-5; PMID:24155319; http://dx.doi.org/10.1523/JNEUROSCI.1048-13.2013
- Naeini R, Witty M-F, Séguéla P, Bourque C. An N-terminal variant of Trpv1 channel is required for osmosensory transduction. Nat Neurosci 2006; 9:93-98; PMID:16327782; http://dx.doi.org/10.1038/nn1614
- Prager-Khoutorsky M, Khoutorsky A, Bourque CW. Unique interweaved microtubule scaffold mediates osmosensory transduction via physical interaction with TRPV1. Neuron 2014; 83:866-78; PMID:25123313; http://dx.doi.org/10.1016/j.neuron.2014.07.023
- Sharif-Naeini R, Ciura S, Bourque CW. TRPV1 gene required for thermosensory transduction and anticipatory secretion from vasopressin neurons during hyperthermia. Neuron 2008; 58:179-85; PMID:18439403; http://dx.doi.org/10.1016/j.neuron.2008.02.013
- Wechselberger M, Wright C, Bishop G, Boulant, J. Ionic channels and conductance-based models for hypothalamic neuronal thermosensitivity. Am J Physiol Regul Integr Comp Physiol 2006; 291:R518-29; PMID:16690776; http://dx.doi.org/10.1152/ajpregu.00039.2006
- Mittag J, Lyons DJ, Sällström J, Vujovic M, Dudazy-Gralla S, Warner A, Wallis K, Alkemade A, Nordström K, Monyer H, et al. Thyroid hormone is required for hypothalamic neurons regulating cardiovascular functions. J Clin Invest 2013; 123:509-16; PMID:23257356; http://dx.doi.org/10.1172/JCI65252
- Voronova I, Tuzhikova A, Kozyreva T. Expression of genes for temperature-sensitive TRP channels in the rat hypothalamus in normal conditions and on adaptation to cold. Neurosci Behav Physiol 2014; 44:565-70; http://dx.doi.org/10.1007/s11055-014-9952-z
- Alcedo J, Flatt T, Pasyukova E. Neuronal inputs and outputs of aging and longevity. Front Genet 2013; 4:71; PMID:23653632; http://dx.doi.org/10.3389/fgene.2013.00071
- Zsombok A. Vanilloid receptors-do they have a role in whole body metabolism? Evidence from TRPV1. J Diabetes Complications 2013; 27:287-92; PMID:23332888; http://dx.doi.org/10.1016/j.jdiacomp.2012.11.006
- Saito M, Yoneshiro T. Capsinoids and related food ingredients activating brown fat thermogenesis and reducing body fat in humans. Curr Opin Lipidol 2013; 24:71-7; PMID:23298960; http://dx.doi.org/10.1097/MOL.0b013e32835a4f40
- Whiting S, Derbyshire E, Tiwari B. Capsaicinoids and capsinoids. A potential role for weight management? A systematic review of the evidence. Appetite 2012; 59:341-8; PMID:22634197; http://dx.doi.org/10.1016/j.appet.2012.05.015
- Motter A, Ahern G. TRPV1-null mice are protected from diet-induced obesity. FEBS Lett 2008; 582:2257-62; PMID:18503767; http://dx.doi.org/10.1016/j.febslet.2008.05.021
- Baboota R, Murtaza N, Jagtap S, Singh DP, Karmase A, Kaur J, Bhutani KK, Boparai RK, Premkumar LS, Kondepudi KK, et al. Capsaicin-induced transcriptional changes in hypothalamus and alterations in gut microbial count in high fat diet fed mice. J Nutr Biochem 2014; 25:893-902; PMID:24917046; http://dx.doi.org/10.1016/j.jnutbio.2014.04.004
- Wanner S, Garami A, Pakai E, Oliveira DL, Gavva NR, Coimbra CC, Romanovsky AA. Aging reverses the role of the transient receptor potential vanilloid-1 channel in systemic inflammation from anti-inflammatory to proinflammatory. Cell Cycle 2012; 11:343-9; PMID:22214765; http://dx.doi.org/10.4161/cc.11.2.18772
- Anand P, Bley K. Topical capsaicin for pain management: therapeutic potential and mechanisms of action of the new high-concentration capsaicin 8% patch. Br J Anaesth 2011; 107:490-502; PMID:21852280; http://dx.doi.org/10.1093/bja/aer260
- Peppin JF, Pappagallo M. Capsaicinoids in the treatment of neuropathic pain: a review. Ther Adv Neurol Disord 2014; 7:22-32; PMID:24409200; http://dx.doi.org/10.1177/1756285613501576
- Rossato M, Granzotto M, Macchi V, Porzionato A, Petrelli L, Calcagno A, Vencato J, De Stefani D, Silvestrin V, Rizzuto R, et al. Human white adipocytes express the cold receptor TRPM8 which activation induces UCP1 expression, mitochondrial activation and heat production. Mol Cell Endocrinol 2014; 383:137-46; PMID:24342393; http://dx.doi.org/10.1016/j.mce.2013.12.005
- Ma S, Yu H, Zhao Z, Luo Z, Chen J, Ni Y, Jin R, Ma L, Wang P, Zhu Z, et al. Activation of the cold-sensing TRPM8 channel triggers UCP1-dependent thermogenesis and prevents obesity. J Mol Cell Biol 2012; 4:88-96; PMID:22241835; http://dx.doi.org/10.1093/jmcb/mjs001
- Kusudo T, Wang Z, Mizuno A, Suzuki M, Yamashita H. TRPV4 deficiency increases skeletal muscle metabolic capacity and resistance against diet-induced obesity. J Appl Physiol (1985) 2012; 112:1223-32; PMID:22207724
- Ye L, Kleiner S, Wu J, Sah R, Gupta RK, Banks AS, Cohen P, Khandekar MJ, Boström P, Mepani RJ, et al. TRPV4 is a regulator of adipose oxidative metabolism, inflammation, and energy homeostasis. Cell 2012; 151:96-110; PMID:23021218; http://dx.doi.org/10.1016/j.cell.2012.08.034
- Zhang Z, Zhang W, Jung DY, Ko HJ, Lee Y, Friedline RH, Lee E, Jun J, Ma Z, Kim F, et al. TRPM2 Ca2+ channel regulates energy balance and glucose metabolism. Am J Physiol Endocrinol Metab 2012; 302:E807-16; PMID:22275755
- Uchida K, Dezaki K, Damdindorj B, Inada H, Shiuchi T, Mori Y, Yada T, Minokoshi Y, Tominaga M. Lack of TRPM2 impaired insulin secretion and glucose metabolisms in mice. Diabetes 2011; 60:119-26; PMID:20921208; http://dx.doi.org/10.2337/db10-0276
- Jang Y, Lee MH, Lee J, Jung J, Lee SH, Yang DJ, Kim BW, Son H, Lee B, Chang S, et al. TRPM2 mediates the lysophosphatidic acid-induced neurite retraction in the developing brain. Pflügers Arch 2014; 466:1987-98; PMID:24413888; http://dx.doi.org/10.1007/s00424-013-1436-4
- Park L, Wang G, Moore J, Girouard H, Zhou P, Anrather J, Iadecola C. The key role of transient receptor potential melastatin-2 channels in amyloid-β-induced neurovascular dysfunction. Nat Commun 2014; 5:5318; PMID:25351853; http://dx.doi.org/10.1038/ncomms6318
- Hoffman N, Miller BA, Wang J, Elrod JW, Rajan S, Gao E, Song J, Zhang XQ, Hirschler-Laszkiewicz I, Shanmughapriya S, et al. Ca2+ entry via Trpm2 is essential for cardiac myocyte bioenergetics maintenance. Am J Physiol Heart Circ Physiol 2015; 308:H637-50; PMID:25576627; http://dx.doi.org/10.1152/ajpheart.00720.2014
- Gao G, Wang W, Tadagavadi RK, Briley NE, Love MI, Miller BA, Reeves WB. TRPM2 mediates ischemic kidney injury and oxidant stress through RAC1. J Clin Invest 2014; 124:4989-5001; PMID:25295536; http://dx.doi.org/10.1172/JCI76042
- Knowles H, Li Y, Perraud A-L. L. The TRPM2 ion channel, an oxidative stress and metabolic sensor regulating innate immunity and inflammation. Immunol Res 2013; 55:241-8; PMID:22975787; http://dx.doi.org/10.1007/s12026-012-8373-8
- Gavva N, Bannon AW, Hovland DN Jr, Lehto SG, Klionsky L, Surapaneni S, Immke DC, Henley C, Arik L, Bak A, et al. Repeated administration of vanilloid receptor TRPV1 antagonists attenuates hyperthermia elicited by TRPV1 blockade. J Pharmacol Exp Thera 2007; 323:128-37; PMID:17652633; http://dx.doi.org/10.1124/jpet.107.125674