Abstract
Hairless skin acts as a heat exchanger between body and environment, and thus greatly contributes to body temperature regulation by changing blood flow to the skin (cutaneous) vascular bed during physiological responses such as cold- or warm-defense and fever. Cutaneous blood flow is also affected by alerting state; we ‘go pale with fright’. The rabbit ear pinna and the rat tail have hairless skin, and thus provide animal models for investigating central pathway regulating blood flow to cutaneous vascular beds. Cutaneous blood flow is controlled by the centrally regulated sympathetic nervous system. Sympathetic premotor neurons in the medullary raphé in the lower brain stem are labeled at early stage after injection of trans-synaptic viral tracer into skin wall of the rat tail. Inactivation of these neurons abolishes cutaneous vasomotor changes evoked as part of thermoregulatory, febrile or psychological responses, indicating that the medullary raphé is a common final pathway to cutaneous sympathetic outflow, receiving neural inputs from upstream nuclei such as the preoptic area, hypothalamic nuclei and the midbrain. Summarizing evidences from rats and rabbits studies in the last 2 decades, we will review our current understanding of the central pathways mediating cutaneous vasomotor control.
Abbreviations
AVAs | = | arteriovenous anastomoses |
CLPO | = | caudolateral preoptic region |
GABA | = | γ-aminobutyric acid |
PGE2 | = | prostaglandin E2 |
RMPO | = | rostromedial preoptic region |
RVLM | = | rostral ventrolateral medulla oblongata |
5-HT | = | 5-hydroxytryptamine. |
Introduction
The skin acts as a protective barrier between the body and the external environment. The skin, especially hairless (glabrous) skin, also functions as a variable capacity heat exchanger. Regulating blood flow to the glabrous skin is an important mechanism determining heat exchanges between the body and the environment, and thus contributes to thermoregulation, while the primary function of the cutaneous vascular bed is to supply substances to the skin itself. Increasing blood flow to the skin by cutaneous vasodilatation enhances heat dissipation from the skin surface, a part of the heat-defense response. Decreasing skin blood flow by cutaneous vasoconstriction greatly contributes to accumulation of central core heat, as a part of the cold-defense response or of the fever response. Cutaneous vasoconstriction also occurs, when the individual is aroused, especially under aversive situation.
Thermo-receptors in the skin are part of the thermoregulatory system. Nakamaura and Morrison recently discovered thermal afferent pathways that convey temperature signals from the periphery to the thermoregulatory center in the preoptic areaCitation1 (For a review see refs.Citation2-4), focusing on thermogenesis as an index of thermoregulatory response.Citation1 The same afferent pathways are involved in thermoregulatory cutaneous vasomotor responses.Citation5
The cutaneous vascular bed is dilated and constricted by hormonal and neural control. In response to acute thermoregulatory or aversive events such as cold exposure or sudden exposure to alerting stimuli, neural influence is predominant. Arteriovenous anastomoses (AVAs) play an important role in cutaneous blood flow regulation. Dilating AVAs provides low-resistance bypasses, which increase cutaneous vascular volume and thus deliver more blood to the skin.Citation6 The AVAs are abundant in the glabrous skin, and are densely innervated by sympathetic nerve fibers.Citation7,8 The sympathetic nerve terminals release noradrenaline, and the noradrenaline binds α-adrenergic receptors on cutaneous vascular smooth muscle resulting in cutaneous vasoconstriction.Citation9-11 Functional studies show that cutaneous blood flow is regulated by sympathetic vasoconstrictor nerves.Citation12,13 There is no consensus about the presence of a sympathetic vasodilator innervation in laboratory animals. Thus this review focuses only on cutaneous vasoconstrictor sympathetic outflow.
The rabbit ear pinna and the rat tail have hairless skin that can act as a heat exchanger,Citation14,15 and thus these animals have provided important animal models for investigating central pathway regulating blood flow to thermoregulatory cutaneous vascular beds. In the last 2 decades, extensive investigations have identified central nuclei and neural pathways that are involved in cutaneous vasomotor control (). The first approach was to find out possible nuclei for cutaneous vasomotor control by investigating the effect of stimulating various brain regions on basal cutaneous vasomotor activity.Citation16-20 Then, the involvement of each nucleus in cutaneous vasomotor changes elicited physiologically was investigated. Cutaneous vasomotor responses are elicited by cold/heat exposure (thermoregulatory response), pyrogenic substance (fever response) or by salient/alerting stimuli (psychological response). With these experimental approaches, the most well investigated nuclei are 1) the raphé/parapyramidal region of the rostral medulla oblongata (the medullary raphé) that contains sympathetic premotor neurons controlling cutaneous vasomotor activity, and 2) the preoptic area that plays a key role for the thermoregulatory and fever responses (). Therefore, in the first 2 sections, we will summarize a series of physiological studies focusing on the medullary raphé, and then studies regarding the preoptic areas. We will focus on other hypothalamic and midbrain nuclei that are associated with cutaneous vasomotor control during thermoregulatory and fever response. A summary of the studies focusing on serotonergic system will be presented, since the medullary raphé region contains the serotonin synthesising B1-B3 bulbospinal cells. The last section highlights several studies focusing on cutaneous vasoconstriction that occurs during aversive/alerting situations, and on possible nuclei that are involved in the psychologically-elicited cutaneous vasoconstriction.
Figure 1. Schematic model for neuronal pathways controlling cutaneous vasomotor activity. Cutaneous vasoconstrictor sympathetic premotor neurons in the medullary raphé excite cutaneous vasoconstrictor sympathetic preganglionic neurons in the spinal intermediolateral nucleus at least in part by serotonergic (5-HT) activation of 5-HT2A receptors and by glutamatergic (GLU) activation. Excitatory drive from the rostral ventrolateral medulla (RVLM) contributes to maintaining cutaneous sympathetic tone. Temperature-responsive neurons in the caudolateral preoptic region (CLPO) and rostromedial preoptic region (RMPO) provide thermoregulatory control of cutaneous vasomotor response to cold or warm stimuli. Warm-responsive preoptic neurons send direct inhibitory (GABAergic) projections to the medullary raphé. The warm-responsive neurons inhibit cutaneous sympathetic premotor neurons under warm-condition and contribute to cutaneous vasodilator response. Some of warm-responsive neurons exert inhibitory influence on cutaneous sympathetic outflow by inhibiting cutaneous vasoconstrictor neurons in the ventral tegmental area (VTA) that may provide excitatory drive to the medullary raphé neurons, or by activating cutaneous vasodilatative neurons in the rostral ventrolateral periaqueductal gray (rvlPAG) that may provide inhibitory drive the medullary raphé neurons. Cold-responsive RMPO neurons send direct excitatory (glutamatergic) projections to the medullary raphé. The cold-responsive neurons excite cutaneous sympathetic premotor neurons in the cold, and contribute to cutaneous vasoconstriction. The cold-responsive RMPO neurons receive tonic GABAergic inputs under warm-condition. PGE2 possibly inhibits GABAergic interneurons in the RMPO that send direct projection to cold-responsive neurons in the RMPO, and elicit cutaneous vasoconstriction by reducing inhibitory influence on the medullary raphé neurons. The GABAergic interneurons may also exert inhibitory influence on the medullary raphé neurons via other indirect pathway. During aversive psychological events, cutaneous vasoconstrictor sympathetic premotor neurons in the medullary raphé are activated at least in part by excitatory drive from neurons in the amygdala, the dorsomedial hypothalamus (DMH), and orexinergic neurons in the hypothalamic area (lateral hypothalamus (LH), perifornical area (PeF) and DMH). Noradrenergic neurons in the locus coeruleus (LC) contribute to the excitatory drive via the amygdala. Activation of the neurons in the habenula elicits cutaneous vasoconstriction possibly by activation of the medullary raphé neurons. Solid black line with a question mark indicates that the pathway is not established. Solid black with dashed lines indicates that it is not known whether pathway is direct or indirect.
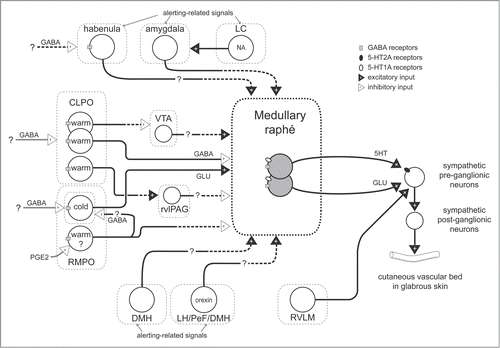
Sympathetic Premotor Neurons Regulating Thermoregulatory Cutaneous Vascular Bed
The medullary raphé
The importance of the medullary raphé in controlling cutaneous vasomotor activity was suggested originally by a report that chemical activation of neurons in the medullary raphé at the rostrocaudal level of the caudal third of the facial nucleus increases tail sympathetic nerve activity in rats.Citation16 Following this report, Blessing and colleagues showed that disinhibition of neurons in the medullary raphé with bicuculline ((γ-aminobutyric acid (GABA)A receptor antagonist)) causes strong cutaneous vasoconstriction in the rat tail and the rabbit ear, measured with Doppler flow probes.Citation17,18 Inhibition of neurons in the medullary raphé causes cutaneous vasodilatation in the rat tail and the rabbit ear.Citation19-21
Cold exposure decreases tail blood flow (cutaneous vasoconstriction). Inhibiting neurons in the medullary raphé reverses cold-evoked cutaneous vasoconstriction in anaesthetized rats by reducing the activation of cutaneous sympathetic nerves ().Citation18,22 Heat exposure or warming the preoptic area causes cutaneous vasodilatation and increases tail skin temperature.Citation23-25 Blocking GABAergic inhibitory inputs to the medullary raphé neurons with bicuculline suppresses the tail cutaneous vasodilatory response ()Citation23
Figure 2. Cutaneous sympathetic nerve is activated by truncal skin cooling in anesthetized rabbits (A) and rats (B). These responses are abolished after microinjection of muscimol (1 nmol in 100 nl) or glycine (100 nmol in 200 nl) into the medullary raphé. The circled numbers (1-3) in each graph correspond to the circled numbers on the bottom X-axis, indicating the experimental period during which the nerve recording was made. Insets show transverse sections of rostral medulla oblongata showing injection sites in rabbit (A) and in rat (B). MVe, medial vestibular nucleus; py, pyramidal tract; VII, facial nucleus. Modified from Ootsuka et al.Citation22 © Elsevier. Permission to reuse must be obtained from the rightsholder.
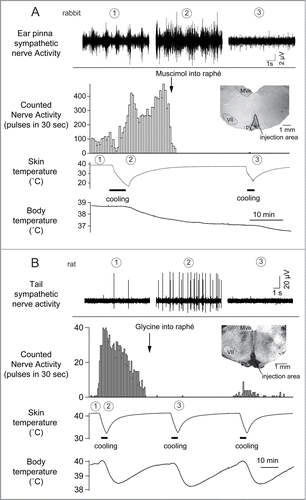
Figure 3. The increase in tail skin temperature elicited by warming the preoptic area (POA) is suppressed by blocking inhibitory input to neurons in the medullary raphé, not in the rostral ventrolateral medulla (RVLM) with bicuculline (150 pmol, in 300 nl per site, arrows) in anesthetized rats. Modified from Tanaka et al.Citation23 © Wiley. Permission to reuse must be obtained from the rightsholder.
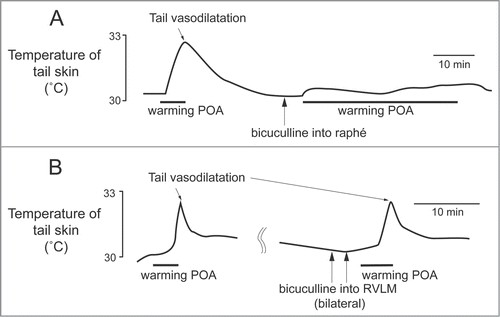
Cutaneous vasoconstriction also occurs in fever, and contributes to an increase in body temperature by reducing heat dissipation from the skin surface. Injection of pyrogenic substances such as lipopolysaccharide and prostaglandin E2 (PGE2), a final humoral mediator that elicits fever,Citation26,27 increases cutaneous sympathetic nerve dischargeCitation28,29,Citation30,Citation31 and elicits cutaneous vasoconstriction.Citation32 Inhibition of the medullary raphé neurons reverses the PGE-elicited cutaneous vasoconstriction.Citation28,33,34
Cold exposure and fever increases fos immunoreactivity in neurons of the medullary raphé/parapyramidal region.Citation35-37 The medullary raphé contains a population of spinally projecting neurons that respond to cooling truncal skin.Citation38 Anatomical studies with transneuronal viral tracing approaches show that the medullary raphé is among the earliest infected cell groups after injection of pseudorabies virus into the rat tail.Citation36,39,40
These functional and anatomical evidences strongly indicate that the medullary raphé contains sympathetic premotor neuron linking to the central neural pathway mediating thermoregulatory signals to sympathetic preganglionic neurons controlling cutaneous sympathetic vasoconstrictor nerves. Functional magnetic resonance imaging suggests involvement of neurons in the medullary raphé in cold-defense response in humans.Citation41
It should be added that disinhibition of neurons in the caudal portion of the medullary raphé nuclei at the level of the rostral part of the inferior olivary nucleus causes cutaneous vasodilatation rather than vasoconstriction.Citation21 The caudal medullary raphé region also contains neurons labeled transneuronally after pseudorabies virus injection in the rat tail.Citation36,39,40 The inhibitory transmitter GABA is present in spinally-projecting medullary raphé neurons,Citation42,43 although their peripheral targets and physiological roles have are not identified. GABA modulates activity of sympathetic preganglionic neurons.Citation44-46 Thus, sympathetic premotor neurons may exist in the caudal medullary raphé that inhibit cutaneous vasoconstrictor sympathetic outflow.
Rostral ventrolateral medulla oblongata (RVLM)
The rostral ventrolateral medulla oblongata (RVLM) contains sympathetic premotor neurons for the cardiovascular system, controlling vasoconstriction, heart rate and arterial pressure.Citation47,48 Neurons in the RVLM, like the medullary raphé, are infected at the same early stage after injection of pseudorabies virus into the rat tail.Citation39 In rats, electrical stimulation of the RVLM reduces tail temperature, indicating cutaneous vasoconstriction.Citation49 In rabbits, inhibition of neurons in the RVLM increases ear pinna blood flow.Citation50 Ootsuka and McAllen demonstrated that chemical inactivation of neurons in the RVLM inhibits ongoing tail cutaneous sympathetic fiber discharges and abolishes its normal excitatory response elicited by cooling truncal skinCitation51 (). These studies suggest that neurons in the RVLM can also influence the thermoregulatory control of cutaneous vasomotor activity. However, further strong skin cooling still increases tail sympathetic nerve discharges after inactivation of neurons in the RVLM (), but not in the medullary raphé (). Thus tonic background drive from the RVLM as well as the medullary raphé is necessary to maintain cutaneous sympathetic activity, while the medullary raphé neurons play a major role in thermoregulatory drive to the cutaneous vascular bed at least during cold- and heat-defense responses. The predominant role of the medullary raphé in cutaneous vasomotor control during thermoregulatory response is partly supported by a report that blockade of inhibitory signal inputs to the medullary raphé but not to the RVLM suppresses cutaneous vasodilatation during heat-defense responseCitation23 ().
Figure 4. Tail sympathetic fiber response to bilateral inhibition of RVLM neurons with muscimol (336 pmol in 160 nl per side, 2 arrows) or to inhibition of the medullary raphé neurons with muscimol (360 pmol in 120nl) in anesthetized rats. (A) After the inhibition of the RVLM neurons, either of L-glutamate (L-Glu, 6 nmol in 120 nl) injection into the medullary raphé or strong cooling was still able to activate tail sympathetic fibers. (B) After neuronal inhibition in the medullary raphé, either of L-Glu injection into the RVLM or strong cooling fails to reactivate the tail fibers. Horizontal bars on skin temperature traces show periods when cooling was performed. Modified from Ootsuka and McAllen.Citation51 © American Physiological Society. Permission to reuse must be obtained from the rightsholder.
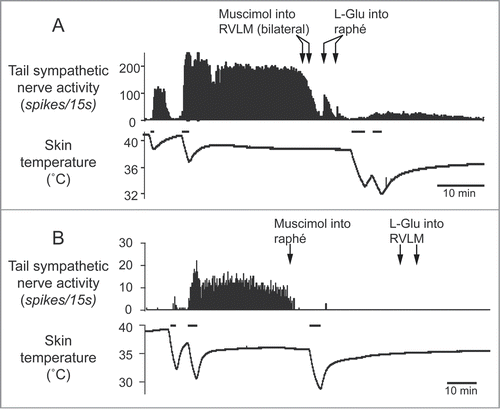
Hypothalamic and Midbrain Nuclei Associated with Cutaneous Vasomotor Control
Preoptic area
The preoptic area plays a key role in controlling body temperature, integrating temperature information from shell and core of the body and then sending efferent signals to thermoregulatory effector organs.Citation52 The preoptic area contains neurons, which respond to local brain, core and skin temperature.Citation53-58 Warming the preoptic area elicits cutaneous vasodilatation in the rat tail (), and inhibits cold-induced activation of cutaneous sympathetic fibers supplying the rat tail ().Citation59
Figure 5. Warming the preoptic area (POA) significantly reduces cold-elicited increase in tail sympathetic nerve activity in anesthetized rats. The left record shows a control cutaneous sympathetic excitatory response to cooling via the water jacket. The right record shows the cold-elicited response was reversed by preoptic warming to 45°C. The inset shows thermode tip locations in 4 experiments (black dots) on a coronal section of the preoptic area. An arrow marks the site warmed in the record. ac, anterior commissure nucleus; CPu, caudate-putamen; LV, lateral ventricle; OX, optic chiasm. Modified from Owens et al.Citation59 © Wiley. Permission to reuse must be obtained from the rightsholder.
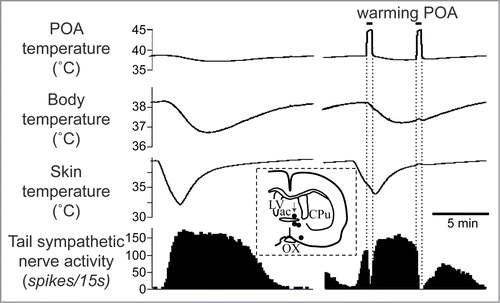
Tanaka and colleagues performed detailed mapping in the preoptic area with nano-injections of GABA. They found 2 distinct preoptic regions providing an inhibitory drive to the tail cutaneous sympathetic fibers; a rostromedial preoptic region (RMPO) surrounding the organum vasaculosum of the lamina terminalis and the ventral part of the median preoptic nucleus, and a preoptic region centered 1mm caudolaterally (CLPO).Citation31 Inhibition of neurons with GABA in both the RMPO and the CLPO activate tail cutaneous sympathetic nerves under warm condition when their activity is low, suggesting that neurons in the 2 preoptic regions are active in the warm (warm-responsive), and that the warm-responsive preoptic neurons provide tonic inhibitory influence on the cutaneous sympathetic outflow.Citation31
Several studies indicate that the preoptic area provides major descending outputs to the medullary raphé for controlling cutaneous vasomotor activity in the rat tail.Citation23,30,34 As already mentioned, blockade of an inhibitory input to the medullary raphé by bicuculline reverses cutaneous vasodilatory response to warming the preoptic area (). Furthermore, warm-responsive neurons projecting directly to the medullary raphé are found in the preoptic area, mainly in the CLPO (). These findings suggest the importance of an inhibitory input from the preoptic area (possibly from the warm-responsive neurons) to the medullary raphé for thermoregulatory cutaneous vasomotor control. Since few warm-responsive neurons which project directly to the medullary raphé are found in the RMPO,Citation30 an inhibitory drive from the RMPO could be indirect.
Figure 6. Thermo-responsive raphé-projecting neurons in the preoptic area in anesthetized rats. (A) Cold-responsive that are activated by skin cooling and inhibited by skin warming. (B) Warm-responsive neurons that are activated by skin warming and inhibited by skin cooling. (C, D) Drawing of rostral and caudal coronal sections in the preoptic area showing some of identified cold-responsive (gray circle) and warm-responsive (white circle) neurons that are antidromically activated by electrical stimulation in the medullary raphé. (E) Distribution of all identified warm- /cold-responsive raphé-projecting neurons in the horizontal plane reconstructed from sequential coronal planes. Crosses show thermo-insensitive raphé-projecting neurons. Shaded areas show the RMPO and CLPO.Citation30 3V, Third ventricle; ac, anterior commissure; AVPV, anteroventral periventricular nucleus; f, fornix; HDB, horizontal limb of the diagonal band of Broca; MnPO, median preoptic area; MPN, medial preoptic nucleus; MPO, medial preoptic area; LPO, lateral preoptic area; LV, lateral ventricle; OVLT, organum vasculosum of the lamina terminalis; ox, optic chiasm. Modified from Tanaka et al.Citation30 © Society for Neuroscience. Permission to reuse must be obtained from the rightsholder.
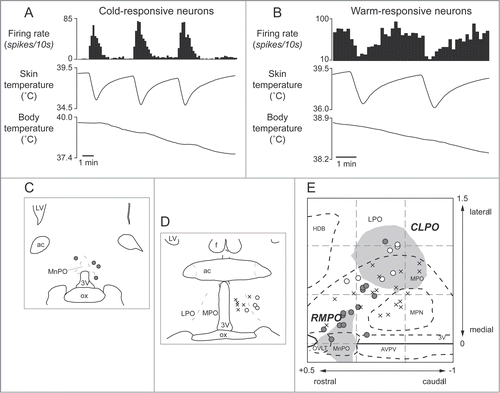
Interestingly, some of the raphé-projecting preoptic neurons are activated by skin cooling (cold-responsive). Most of the cold-responsive raphé-projecting neurons are in the RMPO ().Citation30 Under warm conditions, disinhibition of neurons in the RMPO, but not in the CLPO, with bicuculline increases tail cutaneous sympathetic nerve discharges followed by an increase in body temperatureCitation30 (). Moreover, blockade of excitatory inputs to the medullary raphé with glutamate receptor antagonist kynurenate abolishes the cutaneous sympatho-excitation in response to skin cooling or to bicuculline microinjected into the RMPO ().Citation30 These findings suggest that an excitatory pathway from the cold-responsive RMPO neurons to the medullary raphé mediate cutaneous vasoconstriction response during cold-defense,Citation30 and that the cold-responsive RMPO neurons receive tonic inhibitory GABAergic inputs under warm condition, though the origin of the GABAergic input is unknown (). Thus, the medullary raphé may receive excitatory as well as inhibitory signaling from the preoptic area to regulate cutaneous vasomotor activity.
Figure 7. Microinjection of bicuculline (7.5 pmol in 15 nl) into the RMPO (A) but not into the CLPO (B) activate tail sympathetic fibers in anesthetized rats. Modified from Tanaka et al.Citation30 © Society for Neuroscience. Permission to reuse must be obtained from the rightsholder.
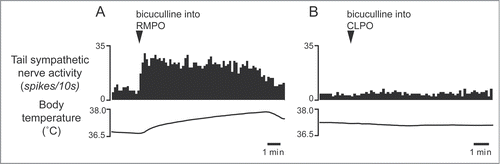
Figure 8. Blocking excitatory inputs to the medullary raphé with kynurenate (6 nmol in 120 nl) inhibits tail sympathetic excitatory response to skin cooling. Modified from Tanaka et al.Citation30 © Society for Neuroscience. Permission to reuse must be obtained from the rightsholder.
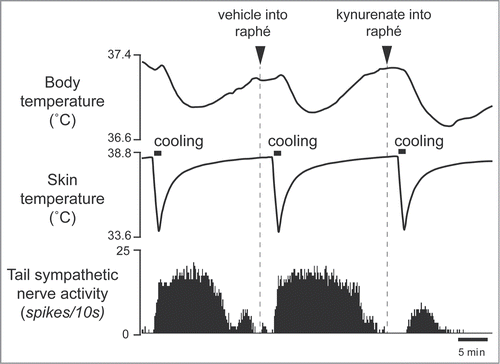
Figure 9. Blocking excitatory inputs to the medullary raphé with kynurenate inhibits tail sympathetic nerve activity elicited by microinjection of bicuculline (7.5 pmol in 15 nl) into the RMPO in anesthetized rats. Vehicle (artificial cerebrospinal fluid, 120nl) (A) or kynurenate (6 nmol in 120 nl) (B) was injected into the medullary raphé. Modified from Tanaka et al.Citation30 © Society for Neuroscience. Permission to reuse must be obtained from the rightsholder.
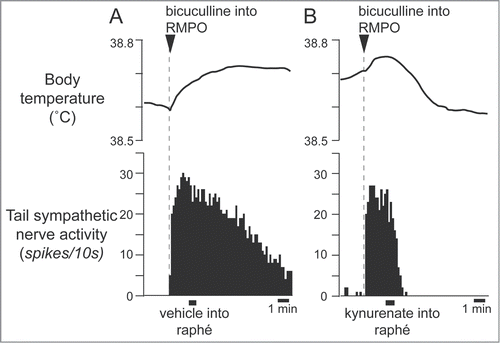
The preoptic area is also the key brain structure for febrile action of PGE2. Several studies have indicated that the RMPO is the most PGE2 sensitive region to cause febrile responses including cutaneous vasoconstriction.Citation31,60,61 The prostaglandin E receptor 3 (EP3 receptor) is considered to be the critical receptor responding to PGE2 to mediate febrile response, and is strongly expressed in the RMPO.Citation62-64,Citation65 More than 85% of EP3-expressing neurons in the preoptic area are GABAergic,Citation35 and PGE2 exerts inhibitory action on neurons via EP3 receptors.Citation66,67,Citation68 The data support the simplest hypothesis that PGE2 inhibits those preoptic neurons (probably warm-responsive) through EP3 receptors,Citation35,69 and then withdraws tonic inhibitory drive to the medullary raphé, causing a febrile response.
Tanaka and colleagues have recently suggested that the excitatory pathway from the RMPO to the medullary raphé might be also involved in the fever response. Microinjection of PGE2 into the RMPO causes a rapid increase in tail cutaneous sympathetic discharges, and subsequent microinjection of glutamate receptor antagonists into the medullary raphé reverses the response ().Citation34 Furthermore, inhibition of neurons in the RMPO with glycine or muscimol injection substantially reduces cutaneous sympatho-excitation elicited by PGE2 injected into the RMPO.Citation34 These results reveal that the cutaneous vasoconstrictor response during experimental fever depends upon an excitatory synaptic relay in the medullary raphé, and that an excitatory drive from the RMPO contributes to the cutaneous vasoconstrictor responses during fever.
Figure 10. Blocking excitatory inputs to the medullary raphé with kynurenate inhibits tail sympathetic excitatory response to PGE2 (0.2 ng in 60 nl) injected into the RMPO in anesthetized rats. Vehicle (artificial cerebrospinal fluid, 120nl) (A) or kynurenate (6nmol in 120 nl) (B) was injected into the medullary raphé. Modified from Tanaka et al.Citation34 © American Physiological Society. Permission to reuse must be obtained from the rightsholder.
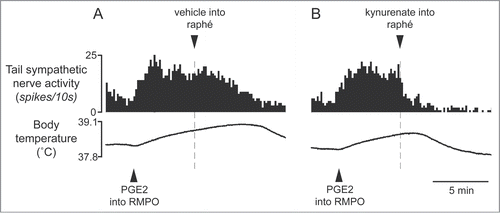
It is not clear how PGE2 activates preoptic neurons. One possibility is that PGE2 activates the cold-responsive RMPO neurons, indirectly by inhibiting interneurons in the RMPO Citation35(), but it remains to be tested. It also remains to be determined whether the fever-driven excitation of cutaneous vasoconstrictor premotor neurons in the medullary raphé comes directly from the RMPO, as does the mechanism through which PGE2 might activate RMPO neurons.
Bilateral injections of bicuculline in the dorsal preoptic area that corresponds to the CLPO region inhibit fever responses elicited by systemic administration of lipopolysaccharide, and elicit cutaneous vasodilatation in the rat tail Citation70(). GABAergic drive to warm-responsive neurons in the CLPO may be promoted during fever responses, and greatly contribute to cutaneous vasoconstriction responses.
Figure 11. Bilateral injections of bicuculline into the dorsal preoptic area (POA) increases tail skin temperature during fever response elicited by intravenous injection of lipopolysaccharide (LPS) (1 µg/kg) in anesthetized rats. Solid arrows show the times when bicuculline (500 pmol in 100 nl) or saline (100 nl) was microinjected into the dorsal POA at intervals of 40 min. Bicuculline-injection group was shown by filled circles (n = 4 ). Saline-injection group was shown by open circles (n = 4 ). Modified from Osaka.Citation70 © Elsevier. Permission to reuse must be obtained from the rightsholder.
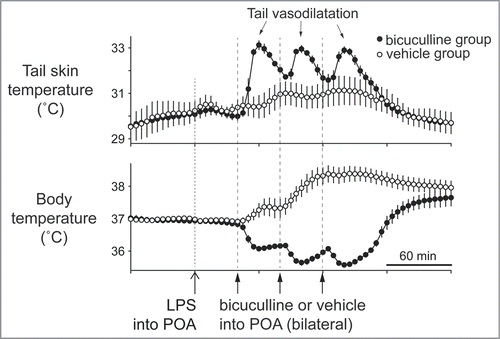
The RMPO and CLPO neurons probably act synergistically to control cutaneous vasomotor activity. This view is supported by a report that combined lesioning of both region, but not independent lesioning of either one, elicits an increase in body temperature.Citation71
Dorsomedial hypothalamus
It has been proposed that the dorsomedial hypothalamus (DMH) integrates thermoregulatory responses to cold and fever.Citation72 Electrical stimulation of the DMH elicits cutaneous vasoconstriction in the rabbit ear pinna.Citation73,Citation74 Pharmacological activation of neurons in the DMH increases cutaneous sympathetic vasoconstrictor activity in rats.Citation28,75 However, the inhibition of the DMH neurons fails to suppress the cutaneous vasoconstriction elicited by PGE2 injected into the preoptic area or by skin cooling, suggesting that the DMH is not involved in cutaneous vasoconstriction during cold-defense and fever responses.Citation28
The DMH has been implicated as mediating behavioral and autonomic physiological response to aversive or psychological stimuli.Citation76,Citation77 Cutaneous vasoconstriction is also elicited by aversive environmental events (see ‘Cutaneous vasoconstriction elicited by psychological stimuli’ section in below). The DMH may be important in this response.
Ventral tegmental area and periaqueductal gray matter
Apart from direct projections from the preoptic area to the medullary raphé, the midbrain area seems to participate in cutaneous vasomotor control. Zhang and colleagues found cutaneous vasoconstrictor neurons in the ventral tegmental area (VTA), and cutaneous vasodilatative neurons in the rostral ventrolateral periaqueductal gray matter (rvl PAG).Citation24 Cutaneous vasodilatory response to warming the preoptic area is inhibited by electrical and pharmacological activation of neurons in the VTA (). Blocking downstream signaling from the VTA by transection of area caudal to the VTA elicits cutaneous vasodilatation. Blocking signal inputs from upstream to the VTA by transection of area rostral to the VTA suppresses the cutaneous vasodilatory response to warming the preoptic area.Citation24
Figure 12. Electrical (0.2 mA, 200 ms, 30 Hz) and pharmacological (D,L-homocysteic acid, 300 pmol in 300 nl ) stimulation in the ventral tegmental area (VTA) reverse increases in tail skin temperature and tail blood flow elicited by warming the preoptic area (POA) in anesthetized rats. A stimulation site (black dot) in the VTA is shown in the inset. ML, medial lemniscus; MP, mammillary nucleus; PAG, periaqueductalgrey. Modified from Zhang et al.Citation24 © Wiley. Permission to reuse must be obtained from the rightsholder.
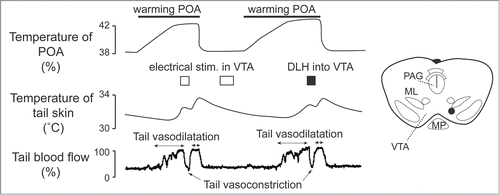
Stimulation of neurons in the rvl PAG elicits cutaneous vasodilatation. Furthermore, blocking signal outputs from the rvl PAG to downstream by the transection of regions in the caudal PAG suppresses the cutaneous vasodilatory response to warming the preoptic area.Citation24 Environmental heat exposure increases fos immunoreactivity in the rvl PAG.Citation78
Considering that there are direct projections from the preoptic area to the rvl PAG and the VTA,Citation79,80 it is possible that these regions contribute to thermoregulatory cutaneous vasomotor control by receiving excitatory inputs in the rvl PAG from the warm-responsive neurons in the preoptic area, and by receiving inhibitory inputs in the VTA from the warm-responsive neurons. In addition, there are direct projections to the medullary raphé from the rvl PAG, but not from the VTA.Citation81 The medullary raphé is likely to mediate the thermoregulatory signals via these areas by receiving excitatory drive from the VTA and inhibitory drive from the rvl PAG.
Contribution of Serotonin in the Regulation of Cutaneous Vasomotor Activity
Neurons in the medullary raphé region include bulbospinal neurons that synthesize 5-hydroxytryptamine (5-HT) Citation82,Citation40,Citation36,Citation83,Citation84,Citation85, known to affect body temperature. Activation of 5-HT1A receptors decreases body temperature,Citation86-90 while activation of 5-HT2A receptors increases body temperature.Citation87,89,91 Acute and chronic inhibition of 5-HT synthesising neurons in the brain causes hypothermia.Citation92,93 Transneuronal viral tracing studies in rats have shown that 5-HT neurons in the medullary raphé are infected at the earliest stage after injection of virus into the rat tail.Citation39 In the study by Toth and colleagues, 90% of virus-infected neurons in the medullary raphé, the parapyramidal region bordering and lateral to the pyramid are found to be 5-HT positive,Citation40 suggesting that 5-HT is one of the candidate neurotransmitter of bulbospinal neurons controlling tail cutaneous vascular bed. Serotonergic terminals are found in the intermediolateral cell column in the spinal cord.Citation83 Serotonergic-immunoreactive terminals are juxtaposed to sympathetic preganglionic cells.Citation94 Pharmacological studies show that 5-HT agonists activate functionally-unspecified sympathetic preganglionic neurons.Citation95,Citation96,Citation97 5-HT antagonists inhibit excitation of sympathetic preganglionic neurons elicited by stimulation of the medullary raphé.Citation98 These studies suggest that some of the 5-HT neurons in the medullary raphé are likely to function as cutaneous sympathetic premotor neurons.
5-HT1A receptors
Ootsuka and Blessing conducted a series of studies to investigate contribution of 5-HT system to cutaneous vasomotor control. The 5-HT1A receptors are considered to be inhibitory somatodendric autoreceptors, that are expressed principally on 5-HT cells,Citation99 although the receptors are also present on non-5-HT neurons.Citation100 Systemic administration of a 5-HT1A agonist, 8-hydroxy-2-(di-n-propylamino)tetralin (8-OH-DPAT) inhibits sympathetically-mediated cutaneous vasoconstriction during cold-defense and fever responses.Citation32,90,Citation101 The systemic administration of 8-OH-DPAT does not affect ear pinna cutaneous post-ganglionic sympathetic nerve discharge evoked by electrical stimulation of preganglionic sympathetic fibers.Citation90 Thus the cutaneous sympatho-inhibitory action of the 8-OH-DPAT is not in the periphery, but in central nervous system, possibly in the medullary raphé. This view is confirmed by further studies in rabbits. Focal injection of 8-OH-DPAT into the medullary raphé inhibits cutaneous vasoconstriction and sympatho-excitation during cold exposure.Citation101 Furthermore, focal injection of a 5-HT1A antagonists, WAY100635 (N-[2-[4-(2-methoxyphenyl)-1-piperazinyl]ethyl]- N-(2-pyridyl)cyclohexanecarboxamide) reverses sympathetically-mediated cutaneous vasomotor changes elicited by intravenous injection of 8-OH-DPAT ().Citation101 Neurons in the medullary raphé inhibited by systemic administration of 8-OH-DPAT increase their activity in response to cold exposure.Citation102 Thus it is likely that inhibitory 5-HT1A receptors are present in the medullary raphé that mediates cutaneous vasoconstriction in response to cold exposure.
Figure 13. Intravenous administration of 5-hydroxytryptamine (5HT) 1A receptor agonist (8-OH-DPAT) inhibits cold-induced ear pinna cutaneous vasoconstriction in conscious rabbits. Microinjection of 5-HT1A receptor antagonist (WAY-100635), into the medullary raphé/parapyramidal region reverses the ear pinna cutaneous vasomotor response to intravenous administration of 8-OH-DPAT. Records of ultrasonic Doppler signal measuring phasic ear pinna blood flow in conscious freely moving rabbits. Modified from Ootsuka and Blessing.Citation101 © Elsevier. Permission to reuse must be obtained from the rightsholder. The second intravenous injection of 8-OH-DPAT after the WAY-100635 did not cause significant effect on cutaneous blood flow, confirming antagonize action of WAY-100635 on 8-OH-DPAT.
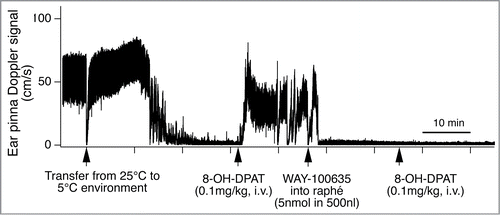
5-HT1A antagonists themselves do not affect resting cutaneous blood flow, suggesting no tonic action through 5-HT1A receptors in the signaling process of cutaneous vasomotor control.Citation90 The physiological role of intrinsic 5-HT1A receptor agonists in cutaneous vasomotor control remains to be established. 5-HT1A receptors might be related to menstrual-related temperature increase.Citation103 5-HT1A receptor agonists like buspirone are used as an anxiolytic agent.Citation104 The combination of anxiolytic and cutaneous symaptoinhibitory properties might therefore be therapeutically useful.Citation32,105
5-HT2A receptors
Cutaneous vasomotor activity is also affected by 5-HT2A receptor agonists and antagonists. Activation of 5-HT2A receptors by systemic administration of 5-HT2A agonist, DOI (R(−)-1-(2,5-dimethoxy-4-iodophenyl)-2-aminopropane hydrochloride) elicits sympathetically-mediated cutaneous vasoconstriction ().Citation89,106,Citation107 This cutaneous sympatho-excitatory effect of 5-HT2A agonists occurs even after the blockade of signaling from the medullary raphé to the spinal cord (), strongly suggesting the involvement of spinal 5-HT2A receptors in the excitation of sympathetic preganglionic cutaneous vasomotor neurons. Indeed, cutaneous sympathetic vasomotor discharges elicited by the stimulation of the medullary raphé is reduced by the blockade of spinal 5HT2A receptors after focal application of the 5HT2A antagonist, SR46349B (trans-4-((3 Z)3-[(2-dimethylaminoethyl)oxyimino]-3-(2-fluorophenyl) propen-1-yl)-phenol, hemifumarate) to the cerebrospinal fluid in the thoracic spinal cord where ear pinna sympathetic vasomotor preganglionic neurons are locatedCitation108 Citation106 (). These studies support a view that 5-HT neurons in the medullary raphé contribute to the regulation of sympathetic cutaneous vasomotor discharges.
Figure 14. Intravenous administration of 5-hydroxytryptamine (5HT) 2A agonist (±)-1-(2,5-dimethoxy-4-iodophenyl)-2-aminopropane (DOI) elicits ear pinna cutaneous vasoconstriction. (A) The 5HT2A agonist DOI (100 µg/kg i.v.) decreases Doppler blood flow signal selectively in ear pinna not in mesenteric artery, and increases body temperature in a conscious rabbit. Modified from Blessing and Seaman.Citation107 © Elsevier. Permission to reuse must be obtained from the rightsholder. (B) Microinjection of muscimol into the medullary raphé (1 nmol in 100 nl) inhibits spontaneous cutaneous sympathetic activity in ear pinna in an anesthetized rabbit. Subsequent DOI (0.1 mg/kg, i.v.) administration activates ear pinna sympathetic fiber. The circled numbers (1-3) on the nerve discharge traces correspond to the circled numbers on the X axis in the integrated nerve activity traces, indicating the experimental period during which the nerve recording was made. Modified Ootsuka et al.Citation106© Elsevier. Permission to reuse must be obtained from the rightsholder.
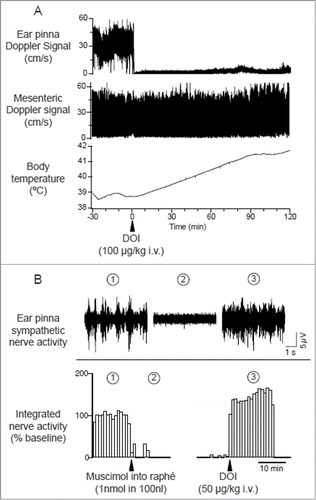
Figure 15. Raphé-elicited ear pinna sympathetic nerve discharge is reduced by 5-hydroxytryptamine (5HT) 2A receptor antagonist, SR-46349B to an isolated cerebrospinal fluid pool (CSF), between T1-T7 thoracic spinal segments in anesthetized rabbits. The remaining response is substantially reduced by subsequent glutamate receptor antagonist, kynurenate. Peri-stimulus average (16 sweeps) of ear pinna sympathetic nerve discharge evoked by triple-pulse electrical stimulation (25 µA, 0.5 ms, 3 pulses at 100 Hz) of the medullary raphé after application to the isolated CSF pool of vehicle (A), SR-46349B (80 µg/kg, 0.8 ml; B), and kynurenate (25 µmol in 0.5 ml; C). Modified from Ootsuka and Blessing.Citation108 © American Physiological Society. Permission to reuse must be obtained from the rightsholder.
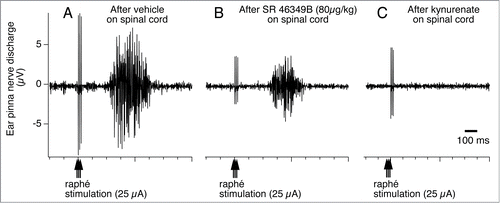
It must be noted that the blockade of 5-HT2A receptors does not completely suppress cutaneous vasomotor sympathetic excitation elicited by electrical stimulation of the medullary raphé (). Subsequent additional blockade of glutamate receptors abolishes the raphé-elicited cutaneous sympatho-excitation (). A transneuronal tracing study demonstrates that the majority of virus-positive medullary raphé neurons express vesicular glutamate transporter 3 after injection of pseudorabies virus into the rat tail.Citation36 Thus, this suggests that the glutamate is as important as 5-HT as a neurotransmitter in the medullary raphé-spinal neurons regulating cutaneous sympathetic outflow. It remains to be investigated whether glutamate co-localizes with 5-HT in the medullary raphé-spinal neurons that regulate cutaneous sympathetic outflow.
To identify neurochemical properties of raphé-spinal sympathetic cutaneous vasomotor neurons, single neural recordings from a neuron labeled transneuronally from cutaneous vascular bed and its immunohistochemical identification are required. So far there are no such studies. Nevertheless, studies with orthodromic activation of descending axons of the raphé-spinal neurons show that their conduction velocity is about 1 m/s in rabbitsCitation108 and in rats (unpublished data, Ootsuka and McAllen). This conduction velocity is within the range of unmyelinated fibers, to which serotonergic axons in the spinal cord belong.Citation109
Cutaneous Vasoconstriction Elicited by Salient/Alerting Stimuli
Majority of the studies on central neural mechanisms of cutaneous vasomotor control have been performed from the thermoregulatory point of view, since cutaneous blood flow are closely associated with changes in body temperature. Cutaneous blood flow is also affected by salient or aversive environmental event. Thus, changes in cutaneous blood flow are actually a reliable index to assess alerting state condition as well as thermoregulatory state in experimental animals.
Cutaneous vasoconstriction is also part of the patterned cardiovascular response to aversive stimuli or conditioned fear as well as thermoregulatory stimuli,Citation110-112,Citation20,113-116 a response known as ‘pale with fright’. The sympathetically-mediated cutaneous vasoconstrictor response to salient stimuli is associated with occurrence of hippocampal theta rhythm, a marker of alertness.Citation110,112 The physiological role of the psychologically-elicited cutaneous vasoconstriction is not properly established yet, but it partly contributes to an increase in body temperature, a response referred to as ‘emotional hyperthermia or ‘psychological fever’.Citation117-120 The cutaneous vasoconstriction may also contribute to equalizing skin surface temperature to surrounding environmental temperature, so that individuals may have more chance to slip through thermal detection by predators such as rattlesnakes and vampire bats.Citation121-123 The cutaneous vasoconstriction may provide some protection to the loss of blood in case of a cut or break in the skin during attacking from predators.Citation116,124
Blessing and colleagues have established an animal model of cutaneous vasoconstriction elicited by salient/alerting events, and have investigated brain mechanisms for the response. Cutaneous blood flow falls without changing intestinal, renal or skeletal muscle blood flow, when individuals confront salient or aversive situations ().Citation20,110 Similarly to thermoregulatory-elicited response, the psychologically-elicited cutaneous vasoconstriction is reversed by inhibition of neurons in the medullary raphé (),Citation20,125 and by 5-HT1A agonist and 5-HT2A antagonists.Citation126,127 It should be noted that microinjection of glutamate receptor antagonists in the medullary raphé does not affect cutaneous vasoconstriction elicited by conditioned fear, suggesting that the vasoconstriction response during the fear response is not under local glutamatergic control unlike the febrile response.Citation125 Dopamine D2 agonists, by its central action, suppress the cutaneous vasoconstriction elicited by alerting stimuli,Citation115 suggesting the involvement of central dopamine system in cutaneous vasomotor control.
Figure 16. Ear pinna Doppler blood flow signal demonstrating cutaneous vasoconstriction elicited by salient stimuli before (A) and after (B) muscimol (3 nmol in 300 nl) injection into the medullary raphé in conscious rabbits. Salient stimuli (a brief sound, cage tap, sudden 1 cm drop of cage, sideways movement of the cage and touching of the animals fur. See details in refsCitation129,139) were applied at the time point indicated by arrows. Modified from Ootsuka and Blessing.Citation20 © Elsevier. Permission to reuse must be obtained from the rightsholder.
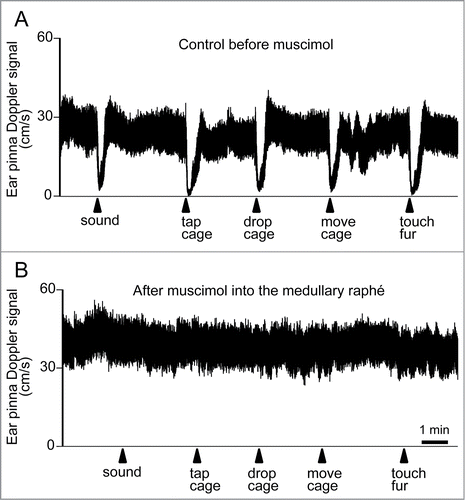
Alerting-related signals are processed in the forebrain nuclei. Stimulation of the amygdala complex, which has been suggested to have an important role in vigilance and arousal, elicits a robust selective fall in cutaneous blood flow.Citation73 Inactivation of the amygdala complex inhibits the psychologically-elicited cutaneous vasoconstriction.Citation111,128,Citation129 Interestingly, removing noradrenergic axons from the locus coeruleus to the amygdala substantially inhibits the psychologically-elicited cutaneous vasoconstriction,Citation130 suggesting that noradrenergic innervation into the amygdala facilitates the forebrain process driving cutaneous vasoconstriction during aversive psychological events. The psychologically-elicited cutaneous vasoconstriction is also attenuated by ablation of orexin neuronsCitation114 in the lateral hypothalamus, which has an important role in regulating wakefulness, motivation and appetite.Citation131,132
Neurons in the habenula complex are activated by aversive environmental events or when animals fail to obtain a reward.Citation133-135 Recently, Ootsuka and Mohammed discovered that disinhibition of neurons in the habenula complex, a phylogenetically ancient nucleus in the dorsal diencephalon, elicits strong vasoconstriction selectively in the thermoregulatory cutaneous vascular bed followed by an increase in body temperature in anesthetized rats.Citation136
Signals from these higher brain centers driving the psychologically-elicited cutaneous vasomotor changes may be integrated in the lower brain stem thermoregulatory pathway including the medullary raphé. The amygdala-induced cutaneous vasoconstriction is reversed by inhibition of neurons in the medullary raphé.Citation73
Conclusion
Many investigations have suggested possible brain nuclei that contribute to cutaneous vasomotor control. So far only 2 nuclei have been thoroughly investigated; the medullary raphé and the preoptic area. Some other nuclei such as the VTA, PAG, hypothalamic and other forebrain nuclei are likely involved in the pathways controlling cutaneous vasomotor activity. Further studies are required to characterize these nuclei and their anatomical and functional connections, including connection from cutaneous vasodilatative neurons in the rvl PAG, and cutaneous vasoconstrictor neurons in the VTA to the medullary raphé. The local circuitry in the preoptic area also remains to be delineated.
Central mechanisms controlling blood flow to cutaneous vascular bed is essentially integrated into the circulatory system that functions to distribute blood flow to tissues proportional to their activity. Under certain circumstances, central mechanisms of cutaneous blood flow control are overridden by signals from other system such as thermoregulatory and alerting systems. The medullary raphé seems to be a common nucleus controlling cutaneous vasomotor outflow for all purposes.
This review does not address thermogenesis, but it is important to note that the medullary raphé is also an important relay for thermogenesis. Significant research progress has enhanced our understanding of central mechanisms controlling thermogenesis focusing on brown adipose tissue (For a review see refs.Citation2-4, 137). Although both cutaneous vasomotor and brown adipose tissue thermogenesis control depend on synaptic relays in the medullary raphé, these 2 thermo effectors targets are controlled by independent neural pathways.Citation28,138 From the thermoregulatory point of view, changing cutaneous vasomotor activity is the most cost effective way to cope with thermoregulatory events, and thus the first-choice during a thermoregulatory challenge, because it requires less water resources compared to panting and sweating, and less bodily fuel compered to shivering and non-shivering thermogenesis in brown adipose tissue. This consideration may explain the necessity of the independent pathways.
Disclosure of Potential Conflicts of Interest
No potential conflicts of interest are disclosed.
About the Authors
Dr Youichirou Ootsuka (Yoichiro Otsuka), also known as YoYo Ootsuka, completed his Bachelor of Biology in 1992, his Master of Medical Science in 1994, and then his PhD in Physiology in 1998 at the Department of Physiology, University of Tsukuba, Japan. During his academic appointment as an assistant lecturer at Institute of Basic Medical Sciences, University of Tsukuba, he was invited to Howard Florey Institute of Experimental and Medicine, as an International Hypertension Society Research Fellow in 2000, and then to the Neurology Laboratory of Flinders University in 2002. In 2009, he was appointed Associate Professor for Department of Physiology, Kagoshima University, Japan. In 2013, he returned to Flinders University as the Mary Overton Neuroscience Research Fellow. He promotes understanding of the brain mechanisms controlling autonomic functions.
Dr Mutsumi Tanaka completed her Bachelor of Health Science in 1998, her Master of Medical Science in 2000 and then her PhD in Physiology in 2003 at Osaka University, Japan. After she undertook her PhD, she joined the Systems Neurobiology group at Florey Neuroscience Institutes as a post-doctoral research officer. She then returned to Japan to take a research position at Health Effect Research Group in Japan Automobile Research Institute in 2013. She is engaged in investigating effects of air pollutants including automobile exhaust, to human health including respiratory and cardiovascular systems.
1069437_SUPPLEMENTAL_FILES.zip
Download Zip (425.4 KB)Funding
Our series of studies was supported by Australian Research Council, Japan Grants-in-Aid for Scientific Research, High Blood Pressure Research Council of Australia, National Health and Medical Research Council, and Flinders Medical Center Foundation.
References
- Nakamura K, Morrison SF. A thermosensory pathway that controls body temperature. Nat Neurosci 2008; 11:62-71; PMID:18084288; http://dx.doi.org/10.1038/nn2027
- Morrison SF, Nakamura K. Central neural pathways for thermoregulation. Front Biosci (Landmark Ed) 2011; 16:74-104; PMID:21196160; http://dx.doi.org/10.2741/3677
- Morrison SF, Madden CJ. Central nervous system regulation of brown adipose tissue. Compr Physiol 2014; 4:1677-713; PMID:25428857; http://dx.doi.org/10.1002/cphy.c140013
- Nakamura K. Central circuitries for body temperature regulation and fever. Am J Physiol Regul Integr Comp Physiol 2011; 301:R1207-28; PMID:21900642; http://dx.doi.org/10.1152/ajpregu.00109.2011
- Nakamura K, Morrison SF. A thermosensory pathway mediating heat-defense responses. Proc Natl Acad Sci U S A 2010; 107:8848-53; PMID:20421477; http://dx.doi.org/10.1073/pnas.0913358107
- Grayson J. Cold and warmth vasoconstrictor responses in the skin of man. Br Heart J 1951; 13:167-72; PMID:14821199; http://dx.doi.org/10.1136/hrt.13.2.167
- Grant RT, Bland EF. Observation on arteriovenous anastomoses in human skin and in the bird's foot with special reference to the reaction to cold. Heart 1931; 15:385-411.
- Grant RT, Bland EF, Camp PD. Observation of the vessles and nerves of the rabbits ear with special refference to the reaction. Heart 1932; 16:69-101.
- Green HD, Howard WB, Kenan LF. Autonomic control of blood flow in hind paw of the dog. Am J Physiol 1956; 187:469-72; PMID:13402908
- Koss MC. Characterization of adrenoceptor subtypes in cat cutaneous vasculature. J Pharmacol Exp Ther 1990; 254:221-7; PMID:2366182
- Ruffolo RR, Jr. Distribution and function of peripheral α-adrenoceptors in the cardiovascular system. Pharmacol Biochem Behav 1985; 22:827-33; PMID:2989947; http://dx.doi.org/10.1016/0091-3057(85)90535-0
- Krogstad AL, Elam M, Karlsson T, Wallin BG. Arteriovenous anastomoses and the thermoregulatory shift between cutaneous vasoconstrictor and vasodilator reflexes. J Auton Nerv Syst 1995; 53:215-22; PMID:7560758; http://dx.doi.org/10.1016/0165-1838(94)00178-M
- O'Leary DS, Johnson JM, Taylor WF. Mode of neural control mediating rat tail vasodilation during heating. J Appl Physiol 1985; 59:1533-8; PMID:4066584
- Wathen P, Mitchell JW, Porter WP. Theoretical and experimental studies of energy exchange from jackrabbit ears and cylindrically shaped appedages. Biophys J 1971; 11:1030-47; PMID:5134209; http://dx.doi.org/10.1016/S0006-3495(71)86276-8
- Rand RP, Burton AC, Ing T. The tail of the rat, in temperature regulation and acclimatization. Can J Physiol Pharmacol 1965; 43:257-67; PMID:14329334; http://dx.doi.org/10.1139/y65-025
- Rathner JA, McAllen RM. Differential control of sympathetic drive to the rat tail artery and kidney by medullary premotor cell groups. Brain Res 1999; 834:196-9; PMID:10407115; http://dx.doi.org/10.1016/S0006-8993(99)01568-1
- Blessing WW, Yu YH, Nalivaiko E. Raphe pallidus and parapyramidal neurons regulate ear pinna vascular conductance in the rabbit. Neurosci Lett 1999; 270:33-6; PMID:10454139; http://dx.doi.org/10.1016/S0304-3940(99)00459-0
- Blessing WW, Nalivaiko E. Raphe magnus/pallidus neurons regulate tail but not mesenteric arterial blood flow in rats. Neuroscience 2001; 105:923-9; PMID:11530230; http://dx.doi.org/10.1016/S0306-4522(01)00251-2
- Osaka T. Hypoxia-induced hypothermia mediated by GABA in the rostral parapyramidal area of the medulla oblongata. Neuroscience 2014; 267:46-56; PMID:24607346; http://dx.doi.org/10.1016/j.neuroscience.2014.02.035
- Ootsuka Y, Blessing WW. Inhibition of medullary raphe/parapyramidal neurons prevents cutaneous vasoconstriction elicited by alerting stimuli and by cold exposure in conscious rabbits. Brain Res 2005; 1051:189-93; PMID:15993863; http://dx.doi.org/10.1016/j.brainres.2005.05.062
- Cerri M, Zamboni G, Tupone D, Dentico D, Luppi M, Martelli D, Perez E, Amici R. Cutaneous vasodilation elicited by disinhibition of the caudal portion of the rostral ventromedial medulla of the free-behaving rat. Neuroscience 2010; 165:984-95; PMID:19895871; http://dx.doi.org/10.1016/j.neuroscience.2009.10.068
- Ootsuka Y, Blessing WW, McAllen RM. Inhibition of rostral medullary raphé neurons prevents cold-induced activity in sympathetic nerves to rat tail and rabbit ear arteries. Neurosci Lett 2004; 357:58-62; PMID:15036613; http://dx.doi.org/10.1016/j.neulet.2003.11.067
- Tanaka M, Nagashima K, McAllen RM, Kanosue K. Role of the medullary raphé in thermoregulatory vasomotor control in rats. J Physiol 2002; 540:657-64; PMID:11956351; http://dx.doi.org/10.1113/jphysiol.2001.012989
- Zhang YH, Hosono T, Yanase-Fujiwara M, Chen XM, Kanosue K. Effect of midbrain stimulations on thermoregulatory vasomotor responses in rats. J Physiol 1997; 503 (Pt 1):177-86; PMID:9288685; http://dx.doi.org/10.1111/j.1469-7793.1997.177bi.x
- Almeida MC, Steiner AA, Branco LG, Romanovsky AA. Cold-seeking behavior as a thermoregulatory strategy in systemic inflammation. Eur J Neurosci 2006; 23:3359-67; PMID:16820025; http://dx.doi.org/10.1111/j.1460-9568.2006.04854.x
- Romanovsky AA, Almeida MC, Aronoff DM, Ivanov AI, Konsman JP, Steiner AA, Turek VF. Fever and hypothermia in systemic inflammation: recent discoveries and revisions. Front Biosci 2005; 10:2193-216; PMID:15970487; http://dx.doi.org/10.2741/1690
- Nakamura K, Morrison SF. Central efferent pathways for cold-defensive and febrile shivering. J Physiol 2011; 589:3641-58; PMID:21610139; http://dx.doi.org/10.1113/jphysiol.2011.210047
- Rathner JA, Madden CJ, Morrison SF. Central pathway for spontaneous and prostaglandin E2-evoked cutaneous vasoconstriction. Am J Physiol Regul Integr Comp Physiol 2008; 295:R343-54; PMID:18463193; http://dx.doi.org/10.1152/ajpregu.00115.2008
- Tanaka M, McAllen RM. A subsidiary fever center in the medullary raphe? Am J Physiol Regul Integr Comp Physiol 2005; 289:R1592-8; PMID:15976309; http://dx.doi.org/10.1152/ajpregu.00141.2005
- Tanaka M, McKinley MJ, McAllen RM. Preoptic-raphe connections for thermoregulatory vasomotor control. J Neurosci 2011; 31:5078-88; PMID:21451045; http://dx.doi.org/10.1523/JNEUROSCI.6433-10.2011
- Tanaka M, McKinley MJ, McAllen RM. Roles of two preoptic cell groups in tonic and febrile control of rat tail sympathetic fibers. Am J Physiol Regul Integr Comp Physiol 2009; 296:R1248-57; PMID:19211726; http://dx.doi.org/10.1152/ajpregu.91010.2008
- Blessing WW. Five-hydroxytryptamine 1A receptor activation reduces cutaneous vasoconstriction and fever associated with the acute inflammatory response in rabbits. Neuroscience 2004; 123:1-4; PMID:14667435; http://dx.doi.org/10.1016/j.neuroscience.2003.09.021
- Korsak A, Gilbey MP. Rostral ventromedial medulla and the control of cutaneous vasoconstrictor activity following i.c.v. prostaglandin E1. Neuroscience 2004; 124:709-17; PMID:14980740; http://dx.doi.org/10.1016/j.neuroscience.2003.12.019
- Tanaka M, McKinley MJ, McAllen RM. Role of an excitatory preoptic-raphe pathway in febrile vasoconstriction of the rat's tail. Am J Physiol Regul Integr Comp Physiol 2013; 305:R1479-89; PMID:24133101; http://dx.doi.org/10.1152/ajpregu.00401.2013
- Nakamura K, Matsumura K, Kaneko T, Kobayashi S, Katoh H, Negishi M. The rostral raphe pallidus nucleus mediates pyrogenic transmission from the preoptic area. J Neurosci 2002; 22:4600-10; PMID:12040067
- Nakamura K, Matsumura K, Hubschle T, Nakamura Y, Hioki H, Fujiyama F, Boldogkoi Z, Konig M, Thiel H-J, Gerstberger R, et al. Identification of Sympathetic Premotor Neurons in Medullary Raphe Regions Mediating Fever and Other Thermoregulatory Functions. J Neurosci 2004; 24:5370-80; PMID:15190110; http://dx.doi.org/10.1523/JNEUROSCI.1219-04.2004
- Morrison SF, Sved AF, Passerin AM. GABA-mediated inhibition of raphe pallidus neurons regulates sympathetic outflow to brown adipose tissue. Am J Physiol 1999; 276:R290-7; PMID:9950904
- Rathner JA, Owens NC, McAllen RM. Cold-activated raphe-spinal neurons in rats. J Physiol (Lond) 2001; 535:841-54; PMID:11559779; http://dx.doi.org/10.1111/j.1469-7793.2001.t01-1-00841.x
- Smith JE, Jansen AS, Gilbey MP, Loewy AD. CNS cell groups projecting to sympathetic outflow of tail artery: neural circuits involved in heat loss in the rat. Brain Res 1998; 786:153-64; PMID:9554992; http://dx.doi.org/10.1016/S0006-8993(97)01437-6
- Toth IE, Toth DE, Boldogkoi Z, Hornyak A, Palkovits M, Blessing WW. Serotonin-synthesizing neurons in the rostral medullary raphe/parapyramidal region transneuronally labelled after injection of pseudorabies virus into the rat tail. Neurochem Res 2006; 31:277-86; PMID:16570210; http://dx.doi.org/10.1007/s11064-005-9018-2
- McAllen RM, Farrell M, Johnson JM, Trevaks D, Cole L, McKinley MJ, Jackson G, Denton DA, Egan GF. Human medullary responses to cooling and rewarming the skin: a functional MRI study. Proc Natl Acad Sci U S A 2006; 103:809-13; PMID:16407125; http://dx.doi.org/10.1073/pnas.0509862103
- Jones BE, Holmes CJ, Rodriguez-Veiga E, Mainville L. GABA-synthesizing neurons in the medulla: their relationship to serotonin-containing and spinally projecting neurons in the rat. J Comp Neurol 1991; 313:349-67; PMID:1722490; http://dx.doi.org/10.1002/cne.903130210
- Stornetta RL, Guyenet PG. Distribution of glutamic acid decarboxylase mRNA-containing neurons in rat medulla projecting to thoracic spinal cord in relation to monoaminergic brainstem neurons. J Comp Neurol 1999; 407:367-80; PMID:10320217; http://dx.doi.org/10.1002/(SICI)1096-9861(19990510)407:3%3c367::AID-CNE5%3e3.0.CO;2-6
- Dun NJ, Karczmar AG, Wu SY, Shen E. Putative transmitter systems of mammalian sympathetic preganglionic neurons. Acta Neurobiol Exp (Wars) 1993; 53:53-63; PMID:8100378
- Krupp J, Feltz P. Synaptic- and agonist-induced chloride currents in neonatal rat sympathetic preganglionic neurones in vitro. J Physiol 1993; 471:729-48; PMID:8120831; http://dx.doi.org/10.1113/jphysiol.1993.sp019925
- Wang L, Spary E, Deuchars J, Deuchars SA. Tonic GABAergic inhibition of sympathetic preganglionic neurons: a novel substrate for sympathetic control. J Neurosci 2008; 28:12445-52; PMID:19020037; http://dx.doi.org/10.1523/JNEUROSCI.2951-08.2008
- Guyenet PG, Haselton JR, Sun MK. Sympathoexcitatory neurons of the rostroventrolateral medulla and the origin of the sympathetic vasomotor tone. Prog Brain Res 1989; 81:105-16; PMID:2616776; http://dx.doi.org/10.1016/S0079-6123(08)62002-6
- Guyenet PG. The sympathetic control of blood pressure. Nat Rev Neurosci 2006; 7:335-46; PMID:16760914; http://dx.doi.org/10.1038/nrn1902
- Key BJ, Wigfield CC. The influence of the ventrolateral medulla on thermoregulatory circulations in the rat. J Auton Nerv Syst 1994; 48:79-89; PMID:8027521; http://dx.doi.org/10.1016/0165-1838(94)90162-7
- Ootsuka Y, Terui N. Functionally different neurons are organized topographically in the rostral ventrolateral medulla of rabbits. J Auton Nerv Syst 1997; 67:67-78; PMID:9470146; http://dx.doi.org/10.1016/S0165-1838(97)00094-5
- Ootsuka Y, McAllen RM. Interactive drives from two brain stem premotor nuclei are essential to support rat tail sympathetic activity. Am J Physiol Regul Integr Comp Physiol 2005; 289:R1107-R15; PMID:15961539; http://dx.doi.org/10.1152/ajpregu.00005.2005
- Romanovsky AA. Thermoregulation: some concepts have changed. Functional architecture of the thermoregulatory system. Am J Physiol Regul Integr Comp Physiol 2007; 292:R37-46; PMID:17008453; http://dx.doi.org/10.1152/ajpregu.00668.2006
- Nakayama T, Hammel HT, Hardy JD, Eisenman JS. Thermal stimulation of electrcial activity of single units of the preopitc region. Am J Physiol 1963; 204:1122-6.
- Hayward JN, Baker MA. Diuretic and thermoregulatory responses to preoptic cooling in the monkey. Am J Physiol 1968; 214:843-50; PMID:4966814
- McEwen GN, Jr., Heath JE. Thermoregulatory responses to preoptic cooling in unrestrained rabbits. Am J Physiol 1974; 227:954-7; PMID:4429145
- Nagashima K, Nakai S, Tanaka M, Kanosue K. Neuronal circuitries involved in thermoregulation. Auton Neurosci 2000; 85:18-25; PMID:11189023; http://dx.doi.org/10.1016/S1566-0702(00)00216-2
- Zhang YH, Yanase-Fujiwara M, Hosono T, Kanosue K. Warm and cold signals from the preoptic area: which contribute more to the control of shivering in rats? J Physiol(Lond) 1995; 485:195-202; PMID:7658373; http://dx.doi.org/10.1113/jphysiol.1995.sp020723
- Kanosue K, Hosono T, Zhang YH, Chen XM. Neuronal networks controlling thermoregulatory effectors. Prog Brain Res 1998; 115:49-62; PMID:9632929; http://dx.doi.org/10.1016/S0079-6123(08)62029-4
- Owens NC, Ootsuka Y, Kanosue K, McAllen RM. Thermoregulatory control of sympathetic fibres supplying the rat's tail. J Physiol (Lond) 2002; 543:849-58; PMID:12231643; http://dx.doi.org/10.1113/jphysiol.2002.023770
- Stitt JT. Differential sensitivity in the sites of fever production by prostaglandin E1 within the hypothalamus of the rat. J Physiol 1991; 432:99-110; PMID:1886074; http://dx.doi.org/10.1113/jphysiol.1991.sp018378
- Scammell TE, Elmquist JK, Griffin JD, Saper CB. Ventromedial preoptic prostaglandin E2 activates fever-producing autonomic pathways. J Neurosci 1996; 16:6246-54; PMID:8815905
- Ek M, Arias C, Sawchenko P, Ericsson-Dahlstrand A. Distribution of the EP3 prostaglandin E(2) receptor subtype in the rat brain: relationship to sites of interleukin-1-induced cellular responsiveness. J Comp Neurol 2000; 428:5-20; PMID:11058221; http://dx.doi.org/10.1002/1096-9861(20001204)428:1%3c5::AID-CNE2%3e3.0.CO;2-M
- Nakamura K, Kaneko T, Yamashita Y, Hasegawa H, Katoh H, Negishi M. Immunohistochemical localization of prostaglandin EP3 receptor in the rat nervous system. J Comp Neurol 2000; 421:543-69; PMID:10842213; http://dx.doi.org/10.1002/(SICI)1096-9861(20000612)421:4%3c543::AID-CNE6%3e3.0.CO;2-3
- Lazarus M, Yoshida K, Coppari R, Bass CE, Mochizuki T, Lowell BB, Saper CB. EP3 prostaglandin receptors in the median preoptic nucleus are critical for fever responses. Nat Neurosci 2007; 10:1131-3; PMID:17676060; http://dx.doi.org/10.1038/nn1949
- Oka T, Oka K, Scammell TE, Lee C, Kelly JF, Nantel F, Elmquist JK, Saper CB. Relationship of EP(1-4) prostaglandin receptors with rat hypothalamic cell groups involved in lipopolysaccharide fever responses. J Comp Neurol 2000; 428:20-32; PMID:11058222; http://dx.doi.org/10.1002/1096-9861(20001204)428:1%3c20::AID-CNE3%3e3.0.CO;2-X
- Negishi M, Sugimoto Y, Ichikawa A. Molecular mechanisms of diverse actions of prostanoid receptors. Biochim Biophys Acta 1995; 1259:109-19; PMID:7492609; http://dx.doi.org/10.1016/0005-2760(95)00146-4
- Narumiya S, Sugimoto Y, Ushikubi F. Prostanoid receptors: structures, properties, and functions. Physiol Rev 1999; 79:1193-226; PMID:10508233
- Exner HJ, Schlicker E. Prostanoid receptors of the EP3 subtype mediate the inhibitory effect of prostaglandin E2 on noradrenaline release in the mouse brain cortex. Naunyn Schmiedebergs Arch Pharmacol 1995; 351:46-52; PMID:7715741; http://dx.doi.org/10.1007/BF00169063
- Nakamura Y, Nakamura K, Morrison SF. Different populations of prostaglandin EP3 receptor-expressing preoptic neurons project to two fever-mediating sympathoexcitatory brain regions. Neuroscience 2009; 161:614-20; PMID:19327390; http://dx.doi.org/10.1016/j.neuroscience.2009.03.041
- Osaka T. Lipopolysaccharide-induced thermogenesis mediated by GABA in the preoptic area of anesthetized rats. J Thermal Biol 2006; 31:229-34; http://dx.doi.org/10.1016/j.jtherbio.2005.12.003
- Yoshida K, Li X, Cano G, Lazarus M, Saper CB. Parallel preoptic pathways for thermoregulation. J Neurosci 2009; 29:11954-64; PMID:19776281; http://dx.doi.org/10.1523/JNEUROSCI.2643-09.2009
- Dimicco JA, Zaretsky DV. The dorsomedial hypothalamus: a new player in thermoregulation. Am J Physiol Regul Integr Comp Physiol 2007; 292:R47-63; PMID:16959861; http://dx.doi.org/10.1152/ajpregu.00498.2006
- Nalivaiko E, Blessing WW. Raphe region mediates changes in cutaneous vascular tone elicited by stimulation of amygdala and hypothalamus in rabbits. Brain Res 2001; 891:130-7; PMID:11164816; http://dx.doi.org/10.1016/S0006-8993(00)03210-8
- Kishi E, Ootsuka Y, Terui N. Different cardiovascular neuron groups in the ventral reticular formation of the rostral medulla in rabbits: single neurone studies. J Auton Nerv Syst 2000; 79:74-83; PMID:10699637; http://dx.doi.org/10.1016/S0165-1838(99)00079-X
- Tanaka M, McAllen RM. Functional topography of the dorsomedial hypothalamus. Am J Physiol Regul Integr Comp Physiol 2008; 294:R477-86; PMID:18077509; http://dx.doi.org/10.1152/ajpregu.00633.2007
- DiMicco JA, Samuels BC, Zaretskaia MV, Zaretsky DV. The dorsomedial hypothalamus and the response to stress: Part renaissance, part revolution. Pharmacol Biochem Behav 2002; 71:469-80; PMID:11830181; http://dx.doi.org/10.1016/S0091-3057(01)00689-X
- DiMicco JA, Sarkar S, Zaretskaia MV, Zaretsky DV. Stress-induced cardiac stimulation and fever: common hypothalamic origins and brainstem mechanisms. Auton Neurosci 2006; 126-127:106-19; PMID:16580890
- Yoshida K, Maruyama M, Hosono T, Nagashima K, Fukuda Y, Gerstberger R, Kanosue K. Fos expression induced by warming the preoptic area in rats. Brain Res 2002; 933:109-17; PMID:11931855; http://dx.doi.org/10.1016/S0006-8993(02)02287-4
- Yoshida K, Konishi M, Nagashima K, Saper CB, Kanosue K. Fos activation in hypothalamic neurons during cold or warm exposure: Projections to periaqueductal gray matter. Neuroscience 2005; 133:1039; PMID:15927405; http://dx.doi.org/10.1016/j.neuroscience.2005.03.044
- Simerly RB, Swanson LW. Projections of the medial preoptic nucleus: a Phaseolus vulgaris leucoagglutinin anterograde tract-tracing study in the rat. J Comp Neurol 1988; 270:209-42; PMID:3259955; http://dx.doi.org/10.1002/cne.902700205
- Hermann DM, Luppi PH, Peyron C, Hinckel P, Jouvet M. Afferent projections to the rat nuclei raphe magnus, raphe pallidus and reticularis gigantocellularis pars α demonstrated by iontophoretic application of choleratoxin (subunit b). J Chem Neuroanat 1997; 13:1-21; PMID:9271192; http://dx.doi.org/10.1016/S0891-0618(97)00019-7
- Loewy AD. Raphe pallidus and raphe obscurus projections to the intermediolateral cell column in the rat. Brain Res 1981; 222:129-33; PMID:6170384; http://dx.doi.org/10.1016/0006-8993(81)90946-X
- Steinbusch HWM. Distribution of serotonin-immunoreactivity in the central nervous system of the rat–Cell bodies and terminals. Neuroscience 1981; 6:557-618; PMID:7017455; http://dx.doi.org/10.1016/0306-4522(81)90146-9
- Skagerberg G, Bjorklund A. Topographic principles in the spinal projections of serotonergic and non-serotonergic brainstem neurons in the rat. Neuroscience 1985; 15:445-80; PMID:4022334; http://dx.doi.org/10.1016/0306-4522(85)90225-8
- Nicholas AP, Pieribone VA, Arvidsson U, Hökfelt T. Serotonin-, substance P- and glutamate/aspartate-like immunoreactivities in medullo-spinal pathways of rat and primate. Neurosci 1992; 48:545-59; http://dx.doi.org/10.1016/0306-4522(92)90401-M
- Hjorth S. Hypothermia in the rat induced by the potent serotoninergic agent 8-OH-DPAT. J Neural Transm 1985; 61:131-5; PMID:3156965; http://dx.doi.org/10.1007/BF01253058
- Gudelsky GA, Koenig JI, Meltzer HY. Thermoregulatory responses to serotonin (5-HT) receptor stimulation in the rat. Evidence for opposing roles of 5-HT2 and 5-HT1A receptors. Neuropharmacology 1986; 25:1307-13; PMID:2951611; http://dx.doi.org/10.1016/0028-3908(86)90101-2
- Cryan JF, Kelliher P, Kelly JP, Leonard BE. Comparative effects of serotonergic agonists with varying efficacy at the 5-HT(1A) receptor on core body temperature: modification by the selective 5-HT(1A) receptor antagonist WAY 100635. J Psychopharmacol (Oxf) 1999; 13:278-83; http://dx.doi.org/10.1177/026988119901300310
- Ootsuka Y, Blessing WW. Thermogenesis in brown adipose tissue: Increase by 5-HT2A receptor activation and decrease by 5-HT1A receptor activation in conscious rats. Neurosci Lett 2006; 395:170-4; PMID:16293365; http://dx.doi.org/10.1016/j.neulet.2005.10.062
- Ootsuka Y, Blessing WW. Five-Hydroxytryptamine 1A receptors inhibit cold-induced sympathetically mediated cutaneous vasoconstriction in rabbits. J Physiol (Lond) 2003; 552:303-14; PMID:12909675; http://dx.doi.org/10.1113/jphysiol.2003.048041
- Loscher W, Witte U, Fredow G, Ganter M, Bickhardt K. Pharmacodynamic effects of serotonin (5-HT) receptor ligands in pigs: stimulation of 5-HT2 receptors induces malignant hyperthermia. Naunyn Schmiedebergs Arch Pharmacol 1990; 341:483-93; PMID:2118235
- Cerpa V, Gonzalez A, Richerson GB. Diphtheria toxin treatment of Pet-1-Cre floxed diphtheria toxin receptor mice disrupts thermoregulation without affecting respiratory chemoreception. Neuroscience 2014; 279:65-76; PMID:25171790; http://dx.doi.org/10.1016/j.neuroscience.2014.08.018
- Loffler S, Korber J, Nubbemeyer U, Fehsel K. Comment on “Impaired respiratory and body temperature control upon acute serotonergic neuron inhibition.” Science 2012; 337:646; author reply; PMID:22879486; http://dx.doi.org/10.1126/science.1221810
- Jensen I, Llewellyn-Smith IJ, Pilowsky P, Minson JB, Chalmers J. Serotonin inputs to rabbit sympathetic preganglionic neurons projecting to the superior cervical ganglion or adrenal medulla. J CompNeurol 1995; 353:427-38.
- McCall RB. Serotonergic excitation of sympathetic preganglionic neurons: a microiontophoretic study. Brain Res 1983; 289:121-7; PMID:6318892; http://dx.doi.org/10.1016/0006-8993(83)90012-4
- Coote JH, Macleod VH, Fleetwood-Walker S, Gilbey MP. The response of individual sympathetic preganglionic neurones to microelectrophoretically applied endogenous monoamines. Brain Res 1981; 215:135-45; PMID:7260583; http://dx.doi.org/10.1016/0006-8993(81)90497-2
- Lewis DI, Coote JH. The influence of 5-hydroxytryptamine agonists and antagonists on identified sympathetic preganglionic neurones in the rat, in vivo. Br J Pharmacol 1990; 99:667-72; PMID:2361167; http://dx.doi.org/10.1111/j.1476-5381.1990.tb12987.x
- McCall RB. Evidence for a serotonergically mediated sympathoexcitatory response to stimulation of medullary raphe nuclei. Brain Res 1984; 311:131-9; PMID:6488035; http://dx.doi.org/10.1016/0006-8993(84)91405-7
- Sprouse JS, Aghajanian GK. Electrophysiological responses of serotoninergic dorsal raphe neurons to 5-HT1A and 5-HT1B agonists. Synapse 1987; 1:3-9; PMID:3505364; http://dx.doi.org/10.1002/syn.890010103
- Kirby LG, Pernar L, Valentino RJ, Beck SG. Distinguishing characteristics of serotonin and non-serotonin-containing cells in the dorsal raphe nucleus: electrophysiological and immunohistochemical studies. Neuroscience 2003; 116:669-83; PMID:12573710; http://dx.doi.org/10.1016/S0306-4522(02)00584-5
- Ootsuka Y, Blessing WW. Activation of 5-HT1A receptors in rostral medullary raphe inhibits cutaneous vasoconstriction elicited by cold exposure in rabbits. Brain Res 2006; 1073-1074:252-61; PMID:16455061; http://dx.doi.org/10.1016/j.brainres.2005.12.031
- Martin-Cora FJ, Fornal CA, Metzler CW, Jacobs BL. Single-unit responses of serotonergic medullary and pontine raphe neurons to environmental cooling in freely moving cats. Neuroscience 2000; 98:301-9; PMID:10854761; http://dx.doi.org/10.1016/S0306-4522(00)00133-0
- Jiang YC, Wu HM, Cheng KH, Sunny Sun H. Menstrual cycle-dependent febrile episode mediated by sequence-specific repression of poly(ADP-ribose) polymerase-1 on the transcription of the human serotonin receptor 1A gene. Hum Mutat 2012; 33:209-17; PMID:21990073; http://dx.doi.org/10.1002/humu.21622
- Cohn JB, Rickels K. A pooled, double-blind comparison of the effects of buspirone, diazepam and placebo in women with chronic anxiety. Curr Med Res Opin 1989; 11:304-20; PMID:2649317; http://dx.doi.org/10.1185/03007998909115213
- Sessler DI. Thermoregulatory defense mechanisms. Crit Care Med 2009; 37:S203-10; PMID:19535948; http://dx.doi.org/10.1097/CCM.0b013e3181aa5568
- Ootsuka Y, Nalivaiko E, Blessing WW. Spinal 5-HT2A receptors regulate cutaneous sympathetic vasomotor outflow in rabbits and rats; relevance for cutaneous vasoconstriction elicited by MDMA (3,4-methylenedioxymethamphetamine, “Ecstasy”) and its reversal by clozapine. Brain Res 2004; 1014:34-44; PMID:15212989; http://dx.doi.org/10.1016/j.brainres.2004.03.058
- Blessing WW, Seaman B. Five-hydroxytryptamine(2A) receptors regulate sympathetic nerves constricting the cutaneous vascular bed in rabbits and rats. Neuroscience 2003; 117:939-48; PMID:12654345; http://dx.doi.org/10.1016/S0306-4522(02)00810-2
- Ootsuka Y, Blessing WW. Activation of slowly conducting medullary raphe-spinal neurons, including serotonergic neurons, increases cutaneous sympathetic vasomotor discharge in rabbit. Am J Physiol Regul Integr Comp Physiol 2005; 288:R909-18; PMID:15550616; http://dx.doi.org/10.1152/ajpregu.00564.2004
- Basbaum AI, Zahs K, Lord B, Lakos S. The fiber caliber of 5-HT immunoreactive axons in the dorsolateral funiculus of the spinal cord of the rat and cat. Somatosens Res 1988; 5:177-85; PMID:3358043; http://dx.doi.org/10.3109/07367228809144625
- Yu YH, Blessing WW. Cutaneous vasoconstriction in conscious rabbits during alerting responses detected by hippocampal theta-rhythm. Am J Physiol 1997; 272:R208-16; PMID:9039011
- Yu YH, Blessing WW. Neurons in amygdala mediate ear pinna vasoconstriction elicited by unconditioned salient stimuli in conscious rabbits. Auton Neurosci 2001; 87:236-42; PMID:11476284; http://dx.doi.org/10.1016/S1566-0702(00)00278-2
- de Menezes RC, Ootsuka Y, Blessing WW. Sympathetic cutaneous vasomotor alerting responses (SCVARs) are associated with hippocampal theta rhythm in non-moving conscious rats. Brain Res 2009; 1298:123-30; PMID:19699727; http://dx.doi.org/10.1016/j.brainres.2009.08.042
- Mohammed M, Ootsuka Y, Blessing W. Brown adipose tissue thermogenesis contributes to emotional hyperthermia in a resident rat suddenly confronted with an intruder rat. Am J Physiol Regul Integr Comp Physiol 2014; 306:R394-400; PMID:24452545; http://dx.doi.org/10.1152/ajpregu.00475.2013
- Mohammed M, Ootsuka Y, Yanagisawa M, Blessing W. Reduced brown adipose tissue thermogenesis during environmental interactions in transgenic rats with ataxin-3-mediated ablation of hypothalamic orexin neurons. Am J Physiol Regul Integr Comp Physiol 2014; 307:R978-89; PMID:25324552; http://dx.doi.org/10.1152/ajpregu.00260.2014
- Blessing WW, Ootsuka Y. Activation of dopamine D2 receptors in the CNS inhibits sympathetic cutaneous vasomotor alerting responses (SCVARs), contributing to clozapine's SCVAR-inhibiting action. Prog Neuropsychopharmacol Biol Psychiatry 2007; 31:328-36; PMID:17055139; http://dx.doi.org/10.1016/j.pnpbp.2006.09.005
- Vianna DM, Carrive P. Changes in cutaneous and body temperature during and after conditioned fear to context in the rat. Eur J Neurosci 2005; 21:2505-12; PMID:15932607; http://dx.doi.org/10.1111/j.1460-9568.2005.04073.x
- Briese E, Cabanac M. Stress hyperthermia: physiological arguments that it is a fever. Physiol Behav 1991; 49:1153-7; PMID:1896496; http://dx.doi.org/10.1016/0031-9384(91)90343-M
- Cabanac M, Gosselin F. Emotional fever in the lizard Callopistes maculatus (Teiidæ). Anim Behav 1993; 46:200-2; http://dx.doi.org/10.1006/anbe.1993.1178
- Lkhagvasuren B, Nakamura Y, Oka T, Sudo N, Nakamura K. Social defeat stress induces hyperthermia through activation of thermoregulatory sympathetic premotor neurons in the medullary raphe region. Eur J Neurosci 2011; 34:1442-52; PMID:21978215; http://dx.doi.org/10.1111/j.1460-9568.2011.07863.x
- Oka T, Oka K, Hori T. Mechanisms and mediators of psychological stress-induced rise in core temperature. Psychosom Med 2001; 63:476-86; PMID:11382276; http://dx.doi.org/10.1097/00006842-200105000-00018
- Gracheva EO, Cordero-Morales JF, Gonzalez-Carcacia JA, Ingolia NT, Manno C, Aranguren CI, Weissman JS, Julius D. Ganglion-specific splicing of TRPV1 underlies infrared sensation in vampire bats. Nature 2011; 476:88-91; PMID:21814281; http://dx.doi.org/10.1038/nature10245
- Campbell AL, Naik RR, Sowards L, Stone MO. Biological infrared imaging and sensing. Micron 2002; 33:211-25; PMID:11567889; http://dx.doi.org/10.1016/S0968-4328(01)00010-5
- Kardong KV, Mackessy SP. The strike behavior of a congenitally blind rattlesnake. J Herpetol 1991; 25:208-11; http://dx.doi.org/10.2307/1564650
- Blessing WW. Lower brainstem pathways regulating sympathetically mediated changes in cutaneous blood flow. Cell Mol Neurobiol 2003; 23:527-38; PMID:14514013; http://dx.doi.org/10.1023/A:1025020029037
- Vianna DM, Allen C, Carrive P. Cardiovascular and behavioral responses to conditioned fear after medullary raphe neuronal blockade. Neuroscience 2008; 153:1344-53; PMID:18440153; http://dx.doi.org/10.1016/j.neuroscience.2008.03.033
- Blessing WW. Clozapine increases cutaneous blood flow and reduces sympathetic cutaneous vasomotor alerting responses (SCVARs) in rats: comparison with effects of haloperidol. Psychopharmacology (Berl) 2005; 181:518-28; PMID:15986198; http://dx.doi.org/10.1007/s00213-005-0012-9
- Ootsuka Y, Blessing WW, Nalivaiko E. Selective blockade of 5-HT2A receptors attenuates the increased temperature response in brown adipose tissue to restraint stress in rats. Stress 2008; 11:125-33; PMID:18311601; http://dx.doi.org/10.1080/10253890701638303
- Mohammed M, Kulasekara K, De Menezes RC, Ootsuka Y, Blessing WW. Inactivation of neuronal function in the amygdaloid region reduces tail artery blood flow alerting responses in conscious rats. Neuroscience 2013; 228:13-22; PMID:23069750; http://dx.doi.org/10.1016/j.neuroscience.2012.10.008
- Yu YH, Blessing WW. Amygdala co-ordinates sudden falls in ear pinna blood flow in response to unconditioned salient stimuli in conscious rabbits. Neuroscience 1999; 93:135-41; PMID:10430478; http://dx.doi.org/10.1016/S0306-4522(99)00097-4
- Mohammed M, Ootsuka Y, Blessing WW. Bilateral amygdala injections of anti-DBH saporin conjugate substantially impar sympathetic cutaneous alerting vasomotor responses (SCVARS). Australian Neuroscience Society Annual Meeting. Gold Coast, 2012:109.
- Sakurai T. The neural circuit of orexin (hypocretin): maintaining sleep and wakefulness. Nat Rev Neurosci 2007; 8:171-81; PMID:17299454; http://dx.doi.org/10.1038/nrn2092
- Kuwaki T, Zhang W. Orexin neurons and emotional stress. Vitam Horm 2012; 89:135-58; PMID:22640612; http://dx.doi.org/10.1016/B978-0-12-394623-2.00008-1
- Hikosaka O. The habenula: from stress evasion to value-based decision-making. Nat Rev Neurosci 2010; 11:503-13; PMID:20559337; http://dx.doi.org/10.1038/nrn2866
- Stamatakis AM, Stuber GD. Activation of lateral habenula inputs to the ventral midbrain promotes behavioral avoidance. Nat Neurosci 2012; 15:1105-7; PMID:22729176; http://dx.doi.org/10.1038/nn.3145
- Lawson RP, Seymour B, Loh E, Lutti A, Dolan RJ, Dayan P, Weiskopf N, Roiser JP. The habenula encodes negative motivational value associated with primary punishment in humans. Proc Natl Acad Sci U S A 2014; 111:11858-63; PMID:25071182; http://dx.doi.org/10.1073/pnas.1323586111
- Ootsuka Y, Mohammed M. Activation of the habenula complex evokes autonomic physiological responses similar to those associated with emotional stress. Physiol Rep 2015; 3:e12297; PMID:25677551; http://dx.doi.org/10.14814/phy2.12297
- Morrison SF, Madden CJ, Tupone D. Central neural regulation of brown adipose tissue thermogenesis and energy expenditure. Cell metabolism 2014; 19:741-56; PMID:24630813; http://dx.doi.org/10.1016/j.cmet.2014.02.007
- Ootsuka Y, McAllen RM. Comparison between two rat sympathetic pathways activated in cold defense. Am J Physiol Regul Integr Comp Physiol 2006; 291:R589-95; PMID:16601257; http://dx.doi.org/10.1152/ajpregu.00850.2005
- Nalivaiko E, Ootsuka Y, Blessing WW. Activation of 5-HT1A receptors in the medullary raphe reduces cardiovascular changes elicited by acute psychological and inflammatory stresses in rabbits. Am J Physiol Regul Integr Comp Physiol 2005; 289:R596-604; PMID:15802554; http://dx.doi.org/10.1152/ajpregu.00845.2004