Abstract
We recently reported that provocative motion (rotation in a home cage) causes hypothermic responses in rats, similar to the hypothermic responses associated with motion sickness in humans. Many stimuli inducing emesis in species with an emetic reflex also provoke hypothermia in the rat, therefore we hypothesized that a fall in body temperature may reflect a “nausea-like” state in these animals. As rats do not possess an emetic reflex, we employed a pharmacological approach to test this hypothesis. In humans, motion- and chemically-induced nausea have differential sensitivity to anti-emetics. We thus tested whether the hypothermia induced in rats by provocative motion (rotation at 0.7 Hz) and by the emetic LiCl (63 mg/kg i.p.) have a similar differential pharmacological sensitivity. Both provocations caused a comparable robust fall in core body temperature (−1.9 ± 0.3°C and −2.0 ± 0.2°C for chemical and motion provocations, respectively). LiCl−induced hypothermia was completely prevented by ondansetron (2mg/kg, i.p., a 5-HT3 receptor antagonist that reduces cancer chemotherapy-induced nausea and vomiting), but was insensitive to promethazine (10 mg/kg, i.p., a predominantly histamine-H1 and muscarinic receptor antagonist that is commonly used to treat motion sickness). Conversely, motion-induced hypothermia was unaffected by ondansetron but promethazine reduced the rate of temperature decline from 0.20 ± 0.02 to 0.11 ± 0.03°C/min (P < 0.05) with a trend to decrease the magnitude. We conclude that this differential pharmacological sensitivity of the hypothermic responses of vestibular vs. chemical etiology in rats mirrors the observations in other pre-clinical models and humans, and thus supports the idea that a “nausea-like” state in rodents is associated with disturbances in thermoregulation.
Abbreviations
AP | = | area postrema |
CTA | = | conditioned taste aversion |
H | = | histamine |
5-HT | = | 5-hydroxytryptamine (serotonin) |
LiCl | = | lithium chloride |
NMDA | = | N-methyl-D-asparate |
NTS | = | nucleus tractus solitarii |
POAH | = | preoptic anterior hypothalamus |
Introduction
The relationship between the unpleasant sensation of nausea and thermoregulation has until recently been largely overlooked.Citation1 Studies of motion sickness revealed that the response integrates physiological (cutaneous vasodilatation, regional sweating, reduction of basal metabolic rate), cognitive (altered perception of ambient temperature) and behavioral (preference for a cooler environment) components (for review, see Ref Citation1). Of note, in rodents hypothermia develops during toxic insults produced by substances (e.g. bacterial toxins, toxic heavy metals, organophosphates etc.) which elicit nausea in humans.Citation2 In view of this relationship we previously investigated whether thermoregulation-related symptoms could be used to identify and quantify “nausea-like” states in real-time in experimental animals.Citation3,4 Current methods for assessing the presence of “nausea-like” states (assuming they occur) in animals rely on behavioral indices with relatively low temporal resolution (e.g., picaCitation5), which require training paradigms (e.g. conditioned taste aversion (CTA),Citation6 conditioned gaping or retchingCitation7,8) or utilize complex analysis of behavioral clusters.Citation9 Although plasma vasopressin is considered a biomarker of nausea in humans and animal species with an emetic reflex (for review, see Ref. Citation10), in rodents lacking an emetic reflexCitation11 it is oxytocin that increases in response to emetic stimuli.Citation12 However, measurement of neither plasma vasopressin nor oxytocin with high temporal resolution is possible in rodents, precluding it from being a potential real-time marker of a “nausea-like” state.
If thermoregulatory disturbances indeed reflect a “nausea-like” state in animals, the changes would be predicted to occur prior to, or coincident with, the onset of emesis (retching or vomiting) in species with an emetic reflex (e.g., Suncus murinus, house musk shrew) and should be sensitive to the effects of anti-emetic drugs with demonstrated efficacy in emetic species and in species lacking an emetic reflex (e.g. rats) using indices of emetic pathway activation such as pica or CTA. Indeed, we found that in house musk shrews provocative motion caused tail vasodilation and reduced temperature in the interscapular region (reflecting reduced thermogenesis in the brown adipose tissue); this happened prior to the onset of emetic episodes.Citation3
The initial aim of this study was to characterize the temporal pattern of the thermoregulatory effects of provocative motion and lithium chloride in rats; these stimuli have been shown to induce picaCitation13-15 or CTACitation16,17 in the rat. Both motion and lithium chloride can induce retching and vomiting in species with an emetic reflex.Citation18-20 In contrast to anti-cancer cytotoxic drugs (e.g., cisplatin), both stimuli can potentially be re-tested in the same animal reducing the use of animals and enabling a counter-balanced design in pharmacological studies. Having characterized the hypothermic response to motion and lithium chloride, we hypothesized that as motion and lithium chloride induce their effects by different pathways (vestibular systemCitation21,22 vs. area postrema/abdominal visceral afferents,Citation23-26 with differing pharmacology, the hypothermic effects should reflect these differences when investigated using agents with well-defined anti-emetic effects. Based on the pharmacological profile in emesis studies, we anticipated that: i) ondansetron (a 5-hydroxytryptamine3 [5-HT3] receptor antagonistCitation10) would prevent or reduce the hypothermia elicited by LiCl as in the rat the unconditioned “lying-on belly” response to lithium chloride (i.p.) was reduced by ondansetronCitation27 but it would have little or no effect on motion-induced hypothermia as it has no effect against motion-induced pica in ratsCitation28 or motion sickness in humansCitation29; ii) promethazine (a histamine1 [H1] receptor antagonist with significant muscarinic antagonist propertiesCitation21,30,31 would have the opposite effects as this class of agent has demonstrated efficacy against motion sickness in humansCitation21 and motion-induced pica in rats.Citation14,32,33
Material and Methods
Animals and surgery
Experiments were conducted in adult male Wistar rats weighing 250 – 300 g, obtained from the Federal University of Paraiba Animal House. Experimental protocols were approved by the Animal Ethics Committee of the Federal University of Paraiba (CEUA # 305/2013). Animals were kept in a temperature-controlled room at 21-22°C, with 12/12h light/dark cycle (lights on at 7 am). Food (standard rat pellets) and water were supplied ad libitum. At least one week before the onset of the experiments, rats were anesthetized (Isoflurane 2% in 100% O2) and miniature programmable temperature data loggers (Thermochron TC, OnSolution Sydney Australia) were implanted intraperitoneally through a small midline incision in the abdominal wall. The surgical technique and validation of the method in rats have been published previously.Citation34 Immediately after surgery, rats were given a single dose of antibiotic - Baytril (Enrofloxacin, 5 mg/kg s.c.) and analgesic - Rimadyl (Carprofen, 5 mg/kg s.c.). The animals were housed individually during the recovery period of 8-10 days; during this time they ate and drank normally, and their body weight increased. At the end of the study, animals were euthanized with an overdose of anesthetic (Lethabarb, 1 ml/kg, i.p.).
Experimental protocol
Experiment 1: This experiment determined the dose of LiCl for the subsequent experiments. In two groups of rats (n = 6 each) LiCl was administered i.p. at either 63 or 126 mg/kg as 0.15 M solution in distilled water, based upon doses in the literature 8; control data (isotonic saline injection, 0.6 ml, i.p.) were taken from Experiment. 3. Throughout the experiment animals remained in their home cages.
Experiment 2: To determine whether a counterbalanced design could be employed in subsequent experiments, we tested whether either LiCl−or rotation-induced hypothermic responses habituated. For this purpose, in one group of rats (n = 6) we administered saline (0.6 ml, i.p.) followed by LiCl (63 mg/kg i.p.) 30 minutes later; the same procedure was repeated one week later. Another group or rats (n = 5) were subjected to 3 × 40-min sessions of rotation (48 h apart) on a turntable (0.75 Hz) in their home cages. This provocation reliably caused hypothermic responses and reduced food consumption in our previous studies 3, 4.
Experiment 3: This experiment determined whether ondansetron alone (2 mg/kg i.p.) or promethazine alone (50, 20 and 10 mg/kg i.p.) affected core body temperature; this was compared to saline (0.6ml, i.p.). In one group of rats (n = 6) either the drugs or vehicle were administered 3 days apart in a rotational design. Comparisons were made for drug effects at 70 and 110 min post-injection as these time points corresponded to the nadir of the hypothermic response after rotation- and LiCl respectively.
Experiment 4: This experiment determined whether either ondansetron (2 mg/kg i.p.) or promethazine (10mg/kg i.p.) affected LiCl−induced hypothermia. In three groups of rats (n = 6 each) LiCl injection was preceded by saline (group 1), ondansetron (group 2) or promethazine (group 3); treatments preceded LiCl by 30 min.
Experiment 5: The purpose of this experiment was to determine whether either ondansetron (2 mg/kg i.p.) or promethazine (10 mg/kg i.p.) pretreatment affected motion-induced hypothermia. One group of rats (n = 6) was tested 3 times, 72 h apart. In a rotational design, animals were pre-treated with saline (0.6 ml, i.p.), ondansetron (2 mg/kg, i.p.) or promethazine (10 mg/kg, i.p.).
Drugs
Lithium chloride hydrate, promethazine hydrochloride and ondansetron hydrochloride were from Sigma (USA). The drugs were dissolved either in distilled water (LiCl) or in isotonic sodium chloride (saline) that was also used for control injections. Since solution of LiCl and corresponding vehicle were injected in the volume of 10 ml/kg, both they were warmed to 36°C prior to injections.
Data collection and analysis
All experiments were conducted in the morning, between 9 am and noon. Data loggers were programmed to start acquisition one week after implantation. Sampling rate was set at one data point every 3 min. For all experiments, baseline measurements were taken from 10 min before the first injection. Baseline values for the core body temperature were compared to maximal changes induced by a drug, by rotation or by combination of both. In addition, using IGOR Pro software (WaveMetrics, Lake Oswego, USA), we determined the maximal slope of temperature fall (in °C/min), the minimal time during which temperature fall reached a value exceeding 3 standard deviations was computed from the baseline period and the time to the nadir of temperature fall. Statistical significance was determined by one-way ANOVA followed by Dunnett's Multiple Comparison post-hoc test, with significance level set at P < 0.05.
Results
Selecting the minimal hypothermic dose of LiCl
Baseline values for core body temperature for the 2 groups of rats that received LiCl were 37.5 ± 0.2°C and 37.3 ± 0.12°C, and did not differ from that for saline (37.3 ± 0.1°C; F = 0.8, p>0.05). In animals that were habituated to injections, administration of saline had minimal effects on body temperature (). In contrast, a few minutes after LiCl injection, body temperature started to fall, reached a nadir approximately one hour post-injection and returned to the baseline 3-3.5 hours later (). Temperature fell by 1.9 ± 0.3°C and 2.2 ± 0.2°C after LiCl doses of 63 and 126 mg/kg, respectively, without between-group differences (p > 0.05). We thus chose to use the smaller dose (10 mg/kg) for subsequent experiments.
Figure 1. Hypothermic responses provoked by LiCl at the dose of 63 mg/kg (A) and 126 mg/kg (B) of 150 mM solution. For consistency with subsequent experiments, i.p. injection of LiCl was preceded by i.p injection of saline. Gray traces - individual recordings; black traces - averaged data from 6 rats.
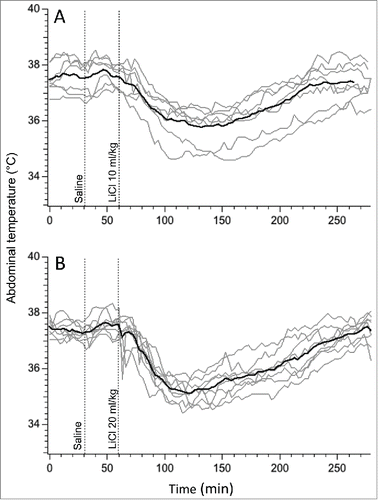
Habituation of hypothermic responses
The baseline values of core body temperature in the LiCl group did not differ on day 1 (37.0 ± 0.2°C) vs. day 8 (39.0 ± 0.1°C, p > 0.05). These animals were not habituated to injections, and it is most likely that for this reason vehicle (saline) caused a noticeable transient hyperthermic response on day 1. Subsequent hypothermic responses to LiCl on day 1 were similar to those observed in experiment 1, with a fall of 1.6 ± 0.3°C (measured from pre-saline baseline) or 2.8 ± 0.3°C (measured from post-saline peak). LiCl−induced hypothermia was substantially attenuated on day 8, being reduced by 0.4 ± 0.2°C (measured from pre-saline baseline, P < 0.05) or 1.3 ± 0.2°C (measured from post-saline peak, P < 0.01). Original and averaged traces are shown in .
Figure 2. Habituation of LiCl−induced (63 mg/kg) hypothermic responses (A) and lack of habituation of rotation-induced hypothermic responses. The latter were induced by a rotation of a home cage with an animal on a turntable at 0.75 rpm. In (A), interval between experiments was 7 days; in (B) - 2 days. Gray traces - individual recordings; black traces - averaged data from 6 rats (A) or 5 rats (B). Rot. - rotations session (40 min).
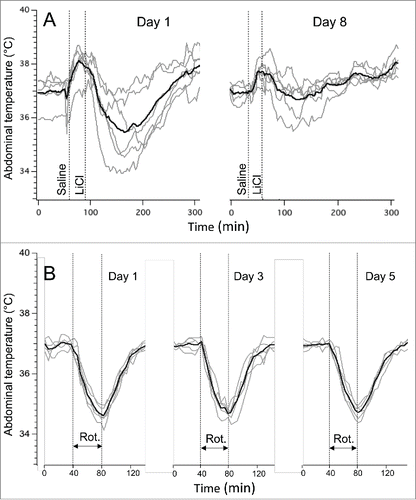
In rats subjected to repetitive rotation sessions, baseline values of core body temperature did not differ between the 3 testing days, being 37.2 ± 0.2°C, 37.0 ± 0.1°C and 37.1 ± 0.2°C (p > 0.05). The temperature started to fall within 2-3 min after the onset of the provocative motion, and kept falling until the end of the rotation, and started to recover soon after its termination. In contrast to the prominent habituation of LiCl−induced responses, rotation-induced hypothermic responses were similar during testing on 3 separate days (−2.3 ± 0.1°C, −2.1 ± 0.2°C and −2.2 ± 0.2°C for day 1, 3 and 5, respectively; p > 0.05). Original and averaged traces from this experiment are shown in .
Effects of ondansetron and promethazine on the core body temperature
Basal values of core body temperature were 37.3 ± 0.1°C; 37.2 ± 0.1°C; 37.2 ± 0.1°C; 37.1 ± 0.1°C saline and 37.3 ± 0.2°C for saline, ondansetron 2 mg/kg (i.p.) and promethazine 50 / 20 / 10 mg/kg (i.p.) conditions, respectively, with no significant difference between them (F = 0.56, p > 0.05). Saline and ondansetron provoked small (about 1°C) and short-lasting temperature rises (), so that at the time points used for comparison (70 and 110 min post-injection), there was no difference compared to the basal values (F = 1.38, p > 0.05 and F = 1.77, p > 0.05 for saline and ondansetron, respectively). Promethazine at doses of 50 and 20 mg/kg (i.p.) provoked substantial (2.0-2.2°C) and prolonged (>3h) hypothermia (); consequently at 70 and 110 min post-injection core body temperature was still significantly lower compared to the baseline values (F = 13.36, P < 0.01 for the dose of 50 mg/kg and F = 35.76, P < 0.01 for the doses of 50 and 20 mg/kg, i.p., respectively). In contrast, hypothermia after the lowest dose (10 mg/kg) of promethazine was of small magnitude and short-duration (), so that at 70 and 100 min post-injection body temperature did not differ significantly from the pre-injection values (F = 1.96, p>0.05). Consequently, this lowest dose of 10 mg/kg promethazine was chosen for subsequent studies.
Experiment 4: Effects of Ondansetron and Promethazine on LICl−induced hypothermia
Because of prominent habituation of the hypothermic response to LiCl found in experiment 2, in this experiment each treatment was administered to a separate group of rats. Data for control (saline) group were from Exp. 1. Basal values of core body temperature were similar in these 3 groups (37.5 ± 0.1°C, 37.4 ± 0.1°C and 37.2 ± 0.2°C for saline, ondansetron and promethazine groups, respectively; p>0.05). In the saline group, LiCl caused fall in body temperature of 1.9 ± 0.3°C (). Pre-treatment with ondansetron completely prevented LiCl−induced hypothermia: at the end of rotation, core body temperature did not change (+0.2 ± 0.1°C, P < 0.0001 compared to saline, ). In contrast, pre-treatment with promethazine was without effect, with a temperature fall of 2.2 ± 0.3°C (p > 0.05) compared to saline (). Ondansetron also substantially and significantly reduced the maximal slope of temperature fall post-LiCl () and substantially and significantly increased the time during which temperature fall exceeded 3 SD computed from the baseline period (), but did not affect time to nadir of hypothermic responses (). Promethazine did not have effect on any of these indices ().
Figure 4. Ondansetron (2 mg/kg i.p.) prevents hypothermic responses provoked by i.p. administration of LiCl (63 mg/kg i.p.) whereas promethazine (10 mg/kg i.p.) has no effect. In three separate groups of rats, LiCl was preceded by saline (A), ondansetron (B) or promethazine (C). Gray traces - individual recordings; black traces - averaged data.
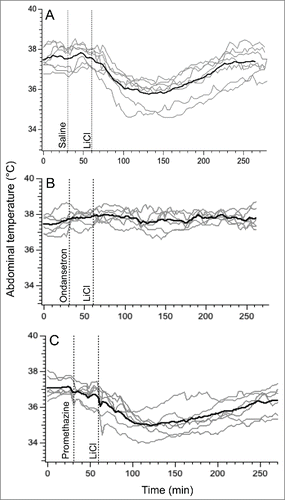
Figure 5. Effects of ondansetron (2 mg/kg i.p.) and promethazine (2 mg/kg i.p.) on hypothermic responses elicited by i.p. administration of LiCl. Bar graphs represent results of statistical analysis of the raw data shown in ; data for comparison were selected from 75 to 85 min post-LiCl injection. (A) Effects on changes in the core body temperature; (B) effects on the maximal slope of the fall in core body temperature; (C) effects on the minimal time during which temperature fall reached values exceeding 3 SD computed from the baseline period; (D) effects on the time to the nadir of the temperature fall. White bars - control (saline, Sal); black bars - ondansetron (Onda); gray bars - promethazine (Prom). Data presented as Mean ± SEM; N = 6 for each group. *,** and *** - P < 0.05, P < 0.01 and P < 0.005, respectively.
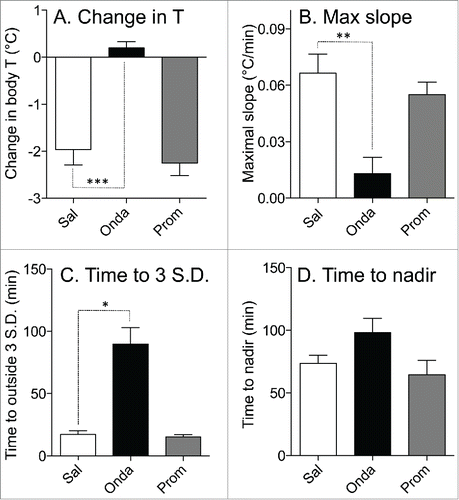
Effects of ondansetron and promethazine on rotation-induced hypothermia
In a group of rats subjected to 3 repetitive sessions of rotation, baseline values of core body temperature did not differ between days 1, 3 and 5 (37.3 ± 0.2°C, 37.1 ± 0.1°C and 37.5 ± 0.2°C, respectively; p > 0.05). Following pre-treatment with saline, provocative motion consistently caused hypothermic responses similar to those described above; core body temperature fell by 2.0 ± 0.2°C (). Pre-treatment with ondansetron had no effect on motion-induced hypothermia (, fall of 2.3 ± 0.1°C, p > 0.05 compared to saline). Administration of promethazine (10 mg/kg, i.p.) caused a small reduction in core temperature; however data from Exp. 3 demonstrated that at the time of the maximal effect of rotation (i.e. 70 min post-promethazine, the time point that we used for comparison) the hypothermic effect of the drug was negligible. While statistical analysis revealed only a trend for reducing the magnitude of hypothermia (−1.4 ± 0.3°C, p > 0.05 vs. saline ), there was substantial inter-individual variability in the effects of promethazine (): in one rat the hypothermic response was virtually abolished, in 3 others - reduced and/or shortened (saline/promethazine: −2.1/−1.3; −1.9/−1.0; −3.0/−2.4°C), and in one - unchanged. Of note, in the animal with the response abolished, promethazine was the first treatment, and thus lack of hypothermia was not a result of habituation. Promethazine, but not ondansetron, also reduced the slope of the temperature fall during rotation (). Neither of the 2 drugs affected the time during which temperature fall exceeded 3 SD computed from the baseline period or the time to nadir of the hypothermic responses ().
Figure 6. Ondansetron (2 mg/kg i.p.) has no effect on hypothermic responses induced by provocative motion whereas promethazine (10 mg/kg i.p.) tended to reduce them. Using rotational design, one group of rats (N = 6) was subjected to provocative motion preceded by saline (A), ondansetron (B) or promethazine (C). Gray traces - individual recordings; black traces - averaged data.
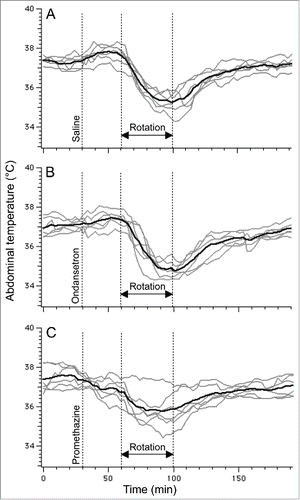
Figure 7. Effects of ondansetron (2 mg/kg i.p.) and promethazine (10 mg/kg i.p.) on hypothermic responses elicited by provocative motion. Bar graphs represent results of statistical analysis of the raw data shown in ; data for comparison were collected from the last 5 min of rotation. (A) Effects on changes in the core body temperature; (B) effects on the maximal slope of the fall in core body temperature; (C) effects on the minimal time during which temperature fall reached values exceeding 3 SD computed from the baseline period; (D) effects on the time to the nadir of the temperature fall. White bars: control (saline, Sal); black bars: ondansetron (Onda); gray bars: promethazine (Prom). Data presented as Mean ± SEM; N = 6 * - P < 0.05.
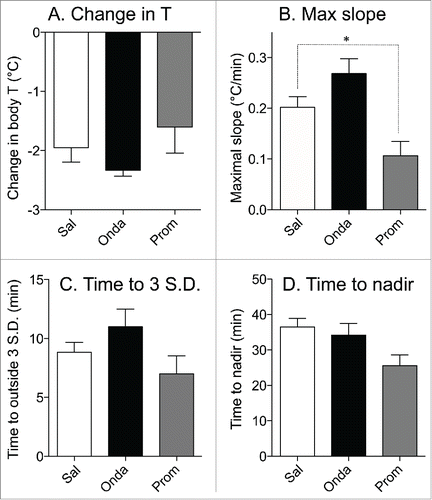
Although we did not formally quantify animals' behavior during provocative motion or after LiCl administration, our general observation was that following short-term (several minutes) behavioral activation either post-injection or after motion onset, rats were quiescent.
Discussion
The principal new finding of the current study is that the commonly used anti-emetic drugs ondansetron and promethazine differentially suppressed, in a predictable way, the hypothermic responses elicited in rats by the chemical emetic agent LiCl and by provocative motion respectively. This differential pharmacological effect mirrors the anti-emetic effects of 5-HT3 and H1 receptor antagonists in humans and animal models and is consistent with the hypothesis that hypothermic responses to emetic stimuli reflect a “nausea-like” state in rodents.
Choice of provocative stimuli and drugs
Passive motion (rotation) and LiCl were used previously in studies of “nausea-like” states in rats (for review, see Ref. Citation35). Motion and LiCl induce CTA and pica (see Introduction) which are accepted indices of activation of pathways (e.g. vestibular system, area postrema, abdominal vagal afferents) implicated in the induction of nausea and/or vomiting (in species capable of reporting nausea and/or of vomiting) and “nausea-like” states in rodents. Our principal hypothesis was that if the hypothermia that accompanies provocative motion or develops following LiCl administration also reflects a “nausea-like” state in rats, it should be differentially sensitive to anti-emetic agents. Ondansetron, a 5-HT3 receptor antagonist, is one of the principal drugs for preventing chemotherapy-induced nausea and vomiting in both humansCitation36 and companion animals,Citation37 while promethazine is among the most efficacious treatments for motion sickness and vertigo in humans, dogs and cats.Citation38 The pharmacological profile of promethazine is complex, and although its anti-emetic effects in motion sickness are ascribed primarily to antagonism of H1 and muscarinic receptorsCitation21,22,30,38 it also has activity at NMDA,Citation39 α-adrenergic,Citation40 5-HT 2A/C,Citation41 dopamine D2Citation42 and a number of ligand- and voltage-gated ion channelsCitation43,44 the extent to which activity at these other receptors contributed to its effects at the doses used in this study is not possible to assess. Anti-emetic effects of promethazine in experimental animals are well documented, with doses used in different species ranging from 2 to 50 mg/kg.Citation19,45,46 However, there appears to be no data on the potency of promethazine in rats to prevent CTA or pica, but there is data utilizing compounds with H1 and muscarinic receptor antagonist actions to support the involvement of histamine and acetylcholine in central mechanism of motion sickness in ratsCitation33,47 as is also the case in humans.Citation21 As our principal measure was body temperature, our experimental constraint was that the drugs used in the study should not affect thermoregulation when given alone. Since high doses of promethazine provoke hypothermia in rodents,Citation48 in the preliminary experiments we attempted to determine the dose of the drug that had no effect on the body temperature. In accord with the previous study,Citation48 we found that at the doses of 50 and 20 mg/kg promethazine provoked significant and prolonged hypothermic effects; an effect that is difficult to explain, since a block of muscarinic receptors at higher doses should produce hyperthermia.Citation49 One possible explanation is that promethazine at higher doses produces hypothermia by blocking NMDA receptors,Citation39 and interestingly NMDA receptor antagonist also have anti-emetic properties against motion-induced emesis in cats.Citation38 For this reason we chose a promethazine dose of 10 mg/kg that had no effect on temperature during the time points selected for comparison (70 min post-injection for rotation and 110 min post-injection for LiCl). Ondansetron alone had no effect on body temperature, in agreement with previous studies.Citation50,51
We found that motion-induced hypothermic responses do not diminish, at least when provocations are presented at 48 h intervals. This allowed a rotational design in the subsequent pharmacological studies. In contrast, substantial blunting of LiCl−induced responses necessitated conducting the pharmacological studies in separate groups of animals. Substantial reduction of the hypothermic response to the second presentation of LiCl is probably not surprising. LiCl has been extensively used as an unconditioned stimulus in numerous studies of conditioned taste aversion (e.g.,Citation16), and attenuation of conditioned responses by a pre-exposure to LiCl has been reported.Citation52
Pathways and pharmacology of the hypothermic responses to motion and lithium chloride
Vestibular stimulation (rotation in a home cage) is associated with both hypothermia and conditioned taste aversion in rats, with both effects abolished by bilateral vestibular lesion.Citation53,54 The presence of both H1 and muscarinic receptors in the medullary vestibular nuclei is well documented (e.g.Citation55,56). In species capable of vomiting, projections from the vestibular nuclei (including via the cerebellum) to the brainstem nucleus tractus solitarius would evoke vomiting while rostral projections to structures including the hypothalamus and limbic system would evoke nausea.Citation22 It is the latter pathways that are most likely responsible for the thermoregulatory changes. In humans and cats the emetic effect of motion is unaffected by the 5-HT3 receptor antagonistsCitation18,29 and this is consistent with the finding in the present study. Promethazine slowed the rate of fall in core temperature induced by motion but the effects on the magnitude of the fall were variable with only a trend toward a reduction. The control studies () showed that the hypothermic response to motion itself is consistent within a group and this suggests that the variability in the effect of promethazine may reflect inter-individual differences in sensitivity to the drug itself. In humans, promethazine is effective against motion but is given at doses where in addition to the central H1 receptor antagonism it also exerts anti-muscarinic effects.Citation31 In the present study the hypothermic effect of promethazine itself was a confounding factor necessitating the selection of a non-hypothermic dose. However, the efficacy in the rat of this dose against the central H1 and muscarinic receptors involved in motion sickness is not known and the lack of a fully efficacious dose combined with inter-individual variability in the motion response may account for the variable effects in the hypothermic response.
Systemic lithium chloride activates neurons in the area postrema as demonstrated by c-fos immunohistochemistry in both emetic (e.g., ferretCitation57) and non-emetic (e.g. ratCitation24) species. In the rat its topical application can produce either an increase or a decrease in discharge of area postrema neurons,Citation26 but when given intraperitoneally lithium chloride increased discharge in both vagal and splanchnic visceral afferents.Citation23 These observations indicate that lithium chloride could activate the central emetic pathways within the NTS in at least 3 ways which are not mutually exclusive: i) directly in the AP which projects to the NTS; ii) through stimulation of visceral afferent (particularly vagal) projections to the NTS; iii) indirectly via activation of vagal afferents projecting to the AP. Although lesion of the area postrema abolishes both the hypothermic and CTA effects of LiCl in the rat,Citation25,58 this does not allow differentiation of the pathway options by which lithium chloride induced its effects as lesion of the AP may also damage the visceral afferent projections into the subjacent NTS. In addition, LiCl could also stimulate the secretion of an endocrine agent(s), for example from the gut (e.g., cholecystokinin), which could act on the area postrema known to be sensitive to a wide range of endogenous substances.Citation35 The pathway from the AP to the NTS does not appear sensitive to blockade by 5-HT3 receptor antagonists including ondansetron as the emetic response to apomorphine and loperamide, both of which act via the AP, are unaffected,Citation28,59,60 and in addition ondansetron was without effect on the CTA produced by lithium chloride, ipecacuanha or cisplatin.Citation61,62 More recent studies showed that while ondansetron did not affect taste avoidance induced by lithium chloride, it did affect the conditioned rejection response implicating 5-HT3 receptors in the genesis of the latter, but the site of action is likely to be outside the brainstem, most likely in the insular cortex.Citation8 Our working hypothesis for the action of lithium chloride in the present study is that it affected 5-HT3 receptors in the dorsal vagal complexCitation63 and/or on the abdominal vagal afferents,Citation10 with subsequent activation of the NTS. However, as the 5-HT3 receptor is permeable to lithium (and other ionsCitation64) an effect of lithium via area postrema 5-HT3 receptors that could be affected by ondansetron cannot be excluded.
For both lithium chloride and motion it is proposed that the hypothermic response is the result of signaling from the NTS to the hypothalamus and limbic systems via direct and indirect (parabrachial nucleus) pathways. This proposal leads to 2 questions discussed below; i) what is the mechanism responsible for the fall in temperature and; ii) why should core temperature fall when emetic pathways are activated?
Potential mechanisms mediating hypothermic response to emetic stimuli and its biological significance
Neural pathways that control thermoregulation are relatively well understood, at least in ratsCitation65,66; they include peripheral and central thermoreceptors, central integrative network and efferent pathways to thermoeffectors - brown adipose tissue (non-shivering thermogenesis), skeletal muscles (shivering thermogenesis) and cutaneous vasculature (heat dissipation). It is unlikely that emetic stimuli or the drugs employed in this study have any direct effects on the peripheral thermosensors, so they can be excluded as potential targets. The central site that integrates thermoregulatory control is located in the preoptic anterior hypothalamus (POAH)Citation65,66; from there, efferent pathways that regulate non-shivering thermogenesis and cutaneous vascular tone sequentially relay in the dorsomedial hypothalamus, the medullary raphe, the intermedio-lateral column of the spinal cord and in the sympathetic ganglia.Citation65,66 For nausea-related hypothermic responses to occur, it is necessary that one or more of these thermoregulatory nodes is affected by either ascending projection from the NTS or by the output from brain areas responsible for processing, at higher levels, neural signals produced by emetic stimuli.Citation67-69 Providing that nausea-related hypothermia represents a highly coordinated integrative response (see below), it is not unreasonable to suggest that the interaction occurs at the highest level the thermoregulatory hierarchy - in the POAH; this hypothalamic region receives direct projections from the NTS.Citation70
In rodents - species that do not possess emetic reflex - hypothermia is a well documented response to toxic substances, including LiCl, morphine, apomorphine, nicotine, alcohol, resiniferatoxinCitation71-75 and copper sulfate (unpublished observation). The adaptive value of this response has been directly confirmed in an animal model of toxic shock where it appeared to be critical for survival,Citation76,77 and it is thus currently accepted that toxin-induced hypothermia is protective in its nature.Citation78 Indeed, since the final effect of many toxins is reduced ATP supply, it is advantageous to reduce body metabolism until a toxin is eliminated. It is more difficult to explain why hypothermia develops in response to provocative motion. Human studies demonstrated that the hypothermic response to visually-induced motion sickness is the result of the coordinated action of thermoeffectors (reduced metabolic rate, increased heat loss), cognition (altered perception of the ambient temperature) and behavior (preference for a cooler environment) (for review, see Ref. Citation1), and thus appears to be a specific coordinated response. Based on ideas initially proposed by TreismanCitation79 and Ossenkopp,Citation53 we have suggested the following interpretationCitation1: static and dynamic postural stability requires exquisite coordination between visual and vestibular systems; any disruption of this coordination is potentially fatal. During evolution, a defensive response against natural toxins affecting visual/vestibular coordination has developed; it comprises a highly aversive experience (nausea) and protective hypothermia. It now appears that this same response could be triggered if visual/vestibular coordination is disturbed by unusual vestibular or visual stimuli, or their combination. In other words, the brain interprets these provocations as a sign of intoxication, and activates pre-programmed protective hypothermic response.
An alternative possible explanation - that nausea-related hypothermia may be associated with drowsiness/reduced arousal is unlikely, at least for provocative motion. In a study that specifically addressed this question we have demonstrated that during this provocation animals remained awake most of the time.Citation4
Conclusions and perspectives
These results are relevant to the neurobiology of nausea in 2 ways. Firstly, our findings further support the proposal that measuring thermal (hypothermic) responses to emetic stimuli might be a fruitful novel approach to detect and quantify a “nausea-like” state in experimental animals. With the exception of a relatively limited number of chemical agents that selectively and directly affect the thermoregulatory pathways, there are only 2 situations when the core body temperature falls: toxic shock and exposure to an excessively cold environment. Those aside, hypothermia appears to be a relatively specific biomarker of a “nausea-like” state. Indeed, it has been shown to be provoked by well established emetic stimuli; it is associated with vomiting in the house musk shrew and with nausea in humans; and, as we show here, the differential pharmacological sensitivity of hypothermia of different origin mirrors similar pharmacological dependence on chemically- vs. motion-induced nausea in humans.
Secondly, our study advances identification of the neural substrate of nausea. It now becomes evident that neural pathways responsible for the sensation of nausea (or “nausea-like” states in animals) must either include or directly project to one of only a few brain regions that control thermoeffectors, namely preoptic anterior and dorsomedial hypothalamus or, less likely, the medullary raphe.
Disclosure of Potential Conflicts of Interest
No potential conflicts of interest were disclosed.
References
- Nalivaiko E, Rudd J, So R. Motion sickness, nausea and thermoregulation: a ‘toxic’ hypothesis. Temperature 2014; 1:164-71; http://dx.doi.org/10.4161/23328940.2014.982047
- Gordon CJ. Temperature and toxicology: an integrative, comparative, and environmental approach. CRC Press: Boca Raton, FL; 2005.
- Ngampramuan S, Cerri M, Del Vecchio F, Corrigan JJ, Kamphee A, Dragic AS, Rudd JA, Romanovsky AA, Nalivaiko E. Thermoregulatory correlates of nausea in rats and musk shrews. Oncotarget 2014; 5:1565-75; PMID:24728971
- Del Vecchio F, Nalivaiko E, Cerri M, Luppi M, Amici R. Provocative motion causes fall in brain temperature and affects sleep in rats. Exp Brain Res 2014; 232:2591-99; PMID:24658633; http://dx.doi.org/10.1007/s00221-014-3899-8
- Yamamoto K, Asano K, Matsukawa N, Imaizumi M, Yamatodani A. Time-course analysis of pica in rats using an automatic feeding monitoring system. J Pharmacol Tox Met 2011; 63:30-4; PMID:20451632; http://dx.doi.org/10.1016/j.vascn.2010.04.011
- Garcia J, Hankins WG. On the origin of food aversion paradigms. In: Learning mechanisms in food selection. Ed. Barker LM. Baylor University Press: Waco, TX; 1977, pp. 3-22.
- Parker LA, Kemp SWP. Tetrahydrocannabinol (THC) interferes with conditioned retching in Suncus murinus: An animal model of anticipatory nausea and vomiting (ANV). Neuroreport 2001; 12:749-51; PMID:11277577; http://dx.doi.org/10.1097/00001756-200103260-00027
- Tuerke KJ, Winters BD, Parker LA. Ondansetron interferes with unconditioned lying-on belly and acquisition of conditioned gaping induced by LiCl as models of nausea-induced behaviors in rats. Physiol Behav 2012; 105:856-60; PMID:22056540; http://dx.doi.org/10.1016/j.physbeh.2011.10.017
- Horn CC, Henry S, Meyers K, Magnusson MS. Behavioral patterns associated with chemotherapy-induced emesis: a potential signature for nausea in musk shrews. Front Neurosci 2011; 5:88; PMID:21808604; http://dx.doi.org/10.3389/fnins.2011.00088
- Andrews PLR, Sanger GJ. Nausea and the quest for the perfect anti-emetic. Eur J Pharmacol 2014; 722:108-21; PMID:24157981; http://dx.doi.org/10.1016/j.ejphar.2013.09.072
- Horn CC, Kimball BA, Wang H, Kaus J, Dienel S, Nagy A, Gathright GR, Yates BJ, Andrews PLR. Why can't rodents vomit? A comparative behavioral, anatomical, and physiological study. Plos One 2013; 8:e60537; PMID:23593236; http://dx.doi.org/10.1371/annotation/1c75cd5d-9dde-4ace-8524-a4980745e804
- Stricker EM, McCann MJ, Flanagan LM, Verbalis JG. Neurohypophyseal secretion and gastric function: biological correlates of nausea. In: Biowarning system in the brain. Ed. Takagi H. University of Tokyo Press: Tokyo, Japan; 1988.
- Mitchell D, Krusemark ML, Hafner D. Pica: a species relevant behavioural assay of motion sickness in the rat. Physiol Behav. 1977; 18:125-30; PMID:561970; http://dx.doi.org/10.1016/0031-9384(77)90103-2
- Morita M, Takeda N, Kubo T, Matsunaga T. Pica as an index of motion sickness in rats. Orl J 1988; 50:188-92; PMID:3261413; http://dx.doi.org/10.1159/000275989
- Yamamoto K, Ngan MP, Takeda N, Yamatodani A, Rudd JA. Differential activity of drugs to induce emesis and pica behavior in Suncus murinus (house musk shrew) and rats. Physiol Behav 2004; 83:151-6; PMID:15501502; http://dx.doi.org/10.1016/S0031-9384(04)00332-4
- Coil JD, Hankins WG, Jenden DJ, Garcia J. Attenuation of a specific cue-to-consequence association by anti-emetic agents. Psychopharmacol 1978; 56:21-5; PMID:415322; http://dx.doi.org/10.1007/BF00571403
- Hutchison S. Taste Aversion in Albino-Rats Using Centrifugal Spin as an Unconditioned Stimulus. Psychol Rep 1973; 33:467-70; PMID:4760830; http://dx.doi.org/10.2466/pr0.1973.33.2.467
- Lucot JB. Blockade of 5-hydroxytryptamine3 receptors prevents cisplatin-induced but not motion- or xylazine-induced emesis in the cat. Pharm Biochem Behav 1989; 32:207-10; PMID:2734331; http://dx.doi.org/10.1016/0091-3057(89)90235-9
- Ueno S, Matsuki N, Saito H. Suncus-murinus as a new experimental-model for motion sickness. Life Sci 1988; 43:413-20; PMID:2899827; http://dx.doi.org/10.1016/0024-3205(88)90520-6
- Rabin BM, Hunt WA. Relationship between vomiting and taste aversion learning in the ferret: studies with ionizing radiation, lithium chloride, and amphetamine. Behav Neural Biol 1992; 58:83-93; PMID:1333765; http://dx.doi.org/10.1016/0163-1047(92)90291-B
- Golding JF, Gresty MA. Pathophysiology and treatment of motion sickness. Curr Opin Neurol 2015; 28:83-8; PMID:25502048; http://dx.doi.org/10.1097/WCO.0000000000000163
- Yates BJ, Catanzaro MF, Miller DJ, McCall AA. Integration of vestibular and emetic gastrointestinal signals that produce nausea and vomiting: potential contributions to motion sickness. Exp Brain Res 2014; 232:2455-69; PMID:24736862; http://dx.doi.org/10.1007/s00221-014-3937-6
- Niijima A, Yamamoto T. The effects of litium chloride on the activity of the afferent nerve fibres from the abdominal visceral organs in the rat. Brain Res 1994; 35:141-5; PMID:7953770
- Olson BR, Freilino M, Hoffman GE, Stricker EM, Sved AF, Verbalis JG. C-Fos expression in rat-brain and brain-stem nuclei in response to treatments that alter food-intake and gastric-motility. Mol Cell Neurosci 1993; 4:93-106; PMID:19912912; http://dx.doi.org/10.1006/mcne.1993.1011
- Ritter S, Mcglone JJ, Kelley KW. Absence of lithium-induced taste-aversion after area postrema lesion. Brain Res 1980; 201:501-6; PMID:7417860; http://dx.doi.org/10.1016/0006-8993(80)91061-6
- Tsukamoto G, Adachi A. Neural responses of rat area postrema to stimuli producing nausea. J Autonom Nerv Syst 1994; 49:55-60; PMID:7963265; http://dx.doi.org/10.1016/0165-1838(94)90020-5
- Tuerke KJ, Limebeer CL, Fletcher PJ, Parker LA. Double dissociation between regulation of conditioned disgust and taste avoidance by serotonin availability at the 5-HT3 receptor in the posterior and anterior insular cortex. J Neurosci 2012; 32:13709-17; PMID:23035083; http://dx.doi.org/10.1523/JNEUROSCI.2042-12.2012
- Takeda N, Hasegawa S, Morita M, Horii A, Uno A, Yamatodani A, Matsunaga T. Neuropharmacological mechanisms of emesis. 1. Effects of antiemetic drugs on motion- and apomorphine-induced pica in rats. Method Find Exp Clin 1995; 17:589-96; PMID:8786672
- Stott JRR, Barnes GR, Wright RJ, Ruddock CJS. The effect on motion sickness and oculomotor function of Gr-38032f, a 5-Ht3-receptor antagonist with anti-emetic properties. Brit J Clin Pharmaco 1989; 27:147-57; PMID:2523720; http://dx.doi.org/10.1111/j.1365-2125.1989.tb05345.x
- Kubo N, Shirakawa O, Kuno T, Tanaka C. Antimuscarinic effects of antihistamines: quantitative evaluation by receptor-binding assay. Jap J Pharmacol 1987; 43:277-82; PMID:2884340; http://dx.doi.org/10.1254/jjp.43.277
- Wood CD, Graybiel A. Evaluation of sixteen antimotion scikenss drugs under controlled laboratory conditions. Aerospace Med 1986; 39:1341-55; PMID:4881887
- Takeda N, Morita M, Hasegawa S, Horii A, Kubo T, Matsunaga T. Neuropharmacology of motion sickness and emesis - a review. Acta Oto-Laryngol 1993; 10-5; PMID:8447218; http://dx.doi.org/10.3109/00016489309126205
- Takeda N, Morita M, Kubo T, Yamatodani A, Watanabe T, Wada H, Matsunaga T. Histaminergic mechanism of motion sickness - neurochemical and neuropharmacological studies in rats. Acta Oto-Laryngol 1986; 101:416-21; PMID:3727976; http://dx.doi.org/10.3109/00016488609108626
- Dallmann R, Steinlechner S, von Horsten S, Karl T. Stress-induced hyperthermia in the rat: comparison of classical and novel recording methods. Lab Anim-Uk 2006; 40:186-93; PMID:16600078; http://dx.doi.org/10.1258/002367706776319015
- Stern R, Koch K, Andrews P. Nausea; Oxford University Press: New York, NY; 2011.
- Roila F, Herrstedt J, Aapro M, Gralla RJ, Einhorn LH, Ballatori E, Bria E, Clark-Snow RA, Espersen BT, Feyer P, et al. Guideline update for MASCC and ESMO in the prevention of chemotherapy- and radiotherapy-induced nausea and vomiting: results of the Perugia consensus conference. Ann Oncol 2010; 21:v232-43; PMID:20555089; http://dx.doi.org/10.1093/annonc/mdq194
- Elwood C, Devauchelle P, Elliott J, Freiche V, German AJ, Gualtieri M, Hall E, den Hertog E, Neiger R, Peeters D, et al. Emesis in dogs: a review. J Small Anim Pract 2010; 51:4-22; PMID:20137004; http://dx.doi.org/10.1111/j.1748-5827.2009.00820.x
- Lucot JB. Neurochemistry and pharmacology of motion sickness in nonhuman species. Chapter 5. In: Motion and space sickness. Ed. Crampton GH. CRC Press: Boca Raton, FL; 1990.
- Adolph O, Koster S, Georgieff M, Georgieff EM, Moulig W, Fohr KJ. Promethazine inhibits NMDA-induced currents - new pharmacological aspects of an old drug. Neuropharmacol 2012; 63:280-91; PMID:22507664; http://dx.doi.org/10.1016/j.neuropharm.2012.03.006
- Zupko I, Janossy K, Maul K, Marki A, Falkay G. Alpha-adrenergic blockade: a possible mechanism of tocolytic action of certain benzodiazepines in a postpartum rat model in vivo. Life Sci 2003; 72:1093-102; PMID:12505540; http://dx.doi.org/10.1016/S0024-3205(02)02306-8
- Fiorella D, Rabin RA, Winter JC. Role of 5-HT2A and 5-HT2C receptors in the stimulus effects of hallucinogenic drugs. II: Reassessment of LSD false positives. Psychopharmacol 1995; 121:357-63; PMID:8584618; http://dx.doi.org/10.1007/BF02246075
- Monsma FJ, Jr., Barton AC, Sibley DR. Expression of functional D2 dopamine receptors following differentiation of Y-79 human retinoblastoma cells. J Neurochem 1990; 54:1200-7; PMID:1968958; http://dx.doi.org/10.1111/j.1471-4159.1990.tb01949.x
- Jo SH, Hong HK, Chong SH, Lee HS, Choe H. H(1) antihistamine drug promethazine directly blocks hERG K(+) channel. Pharm Res 2009; 60:429-37; PMID:19497368; http://dx.doi.org/10.1016/j.phrs.2009.05.008
- Tanaka H, Habuchi Y, Nishimura M, Sato N, Watanabe Y. Blockade of Na+ current by promethazine in guinea-pig ventricular myocytes. Br J Pharmacol 1992; 106:900-5; PMID:1327391; http://dx.doi.org/10.1111/j.1476-5381.1992.tb14432.x
- Kolahian S, Jarolmasjed SH. Antiemetic efficacy of promethazine on xylazine-induced emesis in cats. Can Vet J 2012; 53:193-5; PMID:22851784
- Cooper JR, Mattsson JL. Control of radiation-induced emesis with promethazine, cimetidine, thiethylperazine, or naloxone. Am J Vet Res 1979; 40:1057-61; PMID:525908
- Morita M, Takeda N, Kubo T, Yamatodani A, Wada H, Matsunaga T. Effects of anti-motion sickness drugs on motion sickness in rats. Orl J 1988; 50:330-3; PMID:3186231; http://dx.doi.org/10.1159/000276008
- Packman EW, Rossi GV, Harrison JW. The effect of histamine and antihistamines on body temperature. J Pharm Pharmacol. 1953; 5:301-10; PMID:13062099; http://dx.doi.org/10.1111/j.2042-7158.1953.tb13990.x
- Sen AP, Bhattacharya SK. Thermic response of selective muscarinic agonists and antagonists in rat. Ind J Exp Biol 1991; 29:131-5; PMID:1869296
- Kandasamy SB. Effect of ondansetron and ICS 205-930 on radiation-induced hypothermia in rats. Radiation Res 1997; 147:741-6; PMID:9189174; http://dx.doi.org/10.2307/3579489
- Mazzola-Pomietto P, Aulakh CS, Murphy DL. Temperature, food intake, and locomotor activity effects of a 5-HT3 receptor agonist and two 5-HT3 receptor antagonists in rats. Psychopharmacol 1995; 121:488-93; PMID:8619014; http://dx.doi.org/10.1007/BF02246499
- Riley AL, Jacobs WJ, Lolordo VM. Drug exposure and acquistion and retention of a conditioned taste-aversion. J Comp Physiol Psych 1976; 90:799-807; http://dx.doi.org/10.1037/h0077251
- Ossenkopp KP, Rabi YJ, Eckel LA, Hargreaves EL. Reductions in body-temperature and spontaneous activity in rats exposed to horizontal rotation - abolition following chemical labyrinthectomy. Physiol Behav 1994; 56:319-24; PMID:7938244; http://dx.doi.org/10.1016/0031-9384(94)90201-1
- Ossenkopp KP, Parker LA, Limebeer CL, Burton P, Fudge MA. Vestibular lesions selectively abolish body rotation-induced, but not lithium-induced, conditioned taste aversions (oral rejection responses) in rats. Behav Neurosci 2003; 117:105-12; PMID:12619913; http://dx.doi.org/10.1037/0735-7044.117.1.105
- Wang JJ, Dutia MB. Effects of histamine and betahistine on rat medial vestibular nucleus neurones: possible mechanism of action of anti-histaminergic drugs in vertigo and motion sickness. Exp Brain Res 1995; 105:18-24; PMID:7589314; http://dx.doi.org/10.1007/BF00242178
- Ujihara H, Akaike A, Sasa M, Takaori S. Electrophysiological evidence for cholinoceptive neurons in the medial vestibular nucleus: studies on rat brain stem in vitro. Neurosci Lett 1988; 93:231-5; PMID:3241648; http://dx.doi.org/10.1016/0304-3940(88)90087-0
- Billig I, Yates BJ, Rinaman L. Plasma hormone levels and central c-Fos expression in ferrets after systemic administration of cholecystokinin. Am J Physiol 2001; 281:R1243-55; PMID:11557633
- Bernstein IL, Chavez M, Allen D, Taylor EM. Area postrema mediation of physiological and behavioral-effects of lithium-chloride in the rat. Brain Res 1992; 575:132-7; PMID:1324085; http://dx.doi.org/10.1016/0006-8993(92)90432-9
- Bhandari P, Bingham S, Andrews PL. The neuropharmacology of loperamide-induced emesis in the ferret: the role of the area postrema, vagus, opiate and 5-HT3 receptors. Neuropharmacol 1992; 31:735-42; PMID:1326727; http://dx.doi.org/10.1016/0028-3908(92)90034-M
- Eglen RM, Lee CH, Smith WL, Johnson LG, Clark R, Whiting RL, Hegde SS. Pharmacological characterization of RS 25259-197, a novel and selective 5-HT3 receptor antagonist, in vivo. Br J Pharmacol 1995; 114:860-6; PMID:7773547; http://dx.doi.org/10.1111/j.1476-5381.1995.tb13283.x
- Rudd JA, Ngan MP, Wai MK. 5-HT3 receptors are not involved in conditioned taste aversions induced by 5-hydroxytryptamine, ipecacuanha or cisplatin. Eur J Pharmacol 1998; 352:143-9; PMID:9716348; http://dx.doi.org/10.1016/S0014-2999(98)00359-8
- Mele PC, Mcdonough JR, Mclean DB, Ohalloran KP. Cisplatin-induced conditioned taste-aversion - attenuation by dexamethasone but not zacopride or Gr38032f. Eur J Pharmacol 1992; 218:229-36; PMID:1330594; http://dx.doi.org/10.1016/0014-2999(92)90173-2
- Pratt GD, Bowery NG, Kilpatrick GJ, Leslie RA, Barnes NM, Naylor RJ, Jones BJ, Nelson DR, Palacids JM, Slater P, et al. Consensus meeting agrees distribution of 5-HT3 receptors in mammalian hindbrain. Trends Pharm Sci 1990; 11:135-7; PMID:2333665; http://dx.doi.org/10.1016/0165-6147(90)90058-G
- McKinnon NK, Reeves DC, Akabas MH. 5-HT3 receptor ion size selectivity is a property of the transmembrane channel, not the cytoplasmic vestibule portals. J Gen Physiol 2011; 138:453-66; PMID:21948949; http://dx.doi.org/10.1085/jgp.201110686
- Romanovsky AA. Thermoregulation: some concepts have changed. Functional architecture of the thermoregulatory system. AmJ Physiol 2007; 292:R37-46; PMID:17008453
- Morrison SF, Nakamura K. Central neural pathways for thermoregulation. Front Biosci 2011; 16:74-104; http://dx.doi.org/10.2741/3677
- Farmer AD, Ban VF, Coen SJ, Sanger GJ, Barker GJ, Gresty MA, Giampietro VP, Williams SC, Webb DL, Hellstrom PM, et al. Visually induced nausea causes characteristic changes in cerebral, autonomic and endocrine function in humans. J Physiol 2015; 593:1183-96; PMID:25557265; http://dx.doi.org/10.1113/jphysiol.2014.284240
- Napadow V, Sheehan JD, Kim J, Lacount LT, Park K, Kaptchuk TJ, Rosen BR, Kuo B. The brain circuitry underlying the temporal evolution of nausea in humans. Cerebral Cortex 2013; 23:806-13; PMID:22473843; http://dx.doi.org/10.1093/cercor/bhs073
- Kim J, Napadow V, Kuo B, Barbieri R. A combined HRV-fMRI approach to assess cortical control of cardiovagal modulation by motion sickness. Proc Conf IEEE Eng Med Biol Soc 2011:2825-8; PMID:22254929
- Ito H, Seki M. Ascending projections from the area postrema and the nucleus of the solitary tract of Suncus murinus: anterograde tracing study using Phaseolus vulgaris leucoagglutinin. Okajimas Folia Anat Jpn 1998; 75:9-31; PMID:9715082; http://dx.doi.org/10.2535/ofaj1936.75.1_9
- Lotti VJ, Lomax P, George R. Temperature responses in the rat following intracerebral microinjection of morphine. J Pharm Exp Ther 1965; 150:135-9; PMID:5322549
- Cunningham CL, Niehus JS. Drug-induced hypothermia and conditioned place aversion. Behav Neurosci 1993; 107:468-79; PMID:8329136; http://dx.doi.org/10.1037/0735-7044.107.3.468
- Faunt JE, Crocker AD. The effects of selective dopamine receptor agonists and antagonists on body temperature in rats. Eur J Pharmacol 1987; 133:243-47; PMID:2951265; http://dx.doi.org/10.1016/0014-2999(87)90019-7
- Ruskin DN, Anand R, LaHoste GJ. Menthol and nicotine oppositely modulate body temperature in the rat. Eur J Pharmacol 2007; 559:161-4; PMID:17303112; http://dx.doi.org/10.1016/j.ejphar.2007.01.006
- Woods AJ, Stock MJ, Gupta AN, Wong TT, Andrews PL. Thermoregulatory effects of resiniferatoxin in the rat. Eur J Pharmacol 1994; 264:125-33; PMID:7851474; http://dx.doi.org/10.1016/0014-2999(94)00445-5
- Liu E, Lewis K, Al-Saffar H, Krall CM, Singh A, Kulchitsky VA, Corrigan JJ, Simons CT, Petersen SR, Musteata FM, et al. Naturally occurring hypothermia is more advantageous than fever in severe forms of lipopolysaccharide- and Escherichia coli-induced systemic inflammation. Am J Physiol 2012; 302:R1372-83; PMID:22513748
- Romanovsky AA, Shido O, Sakurada S, Sugimoto N, Nagasaka T. Endotoxin shock-associated hypothermia. How and why does it occur? Ann N Y Acad Sci 1997; 813:733-7; PMID:9100963; http://dx.doi.org/10.1111/j.1749-6632.1997.tb51775.x
- Gordon CJ. Response of the thermoregulatory system to toxic insults. Front Biosci 2010; 2:293-311; PMID:20036879; http://dx.doi.org/10.2741/e91
- Treisman M. Motion sickness: an evolutionary hypothesis. Science 1977; 197:493-5; PMID:301659; http://dx.doi.org/10.1126//science.301659