ABSTRACT
Functional brain imaging of responses to thermal challenge in humans provides a viable method to implicate widespread neuroanatomical regions in the processes of thermoregulation. Thus far, functional neuroimaging techniques have been used infrequently in humans to investigate thermoregulation, although preliminary outcomes have been informative and certainly encourage further forays into this field of enquiry. At this juncture, sustained regional brain activations in response to prolonged changes in body temperature are yet to be definitively characterized, but it would appear that thermoregulatory regions are widely distributed throughout the hemispheres of the human brain. Of those autonomic responses to thermal challenge investigated so far, the loci of associated brainstem responses in human are homologous with other species. However, human imaging studies have also implicated a wide range of forebrain regions in thermal sensations and autonomic responses that extend beyond outcomes reported in other species. There is considerable impetus to continue human functional neuroimaging of thermoregulatory responses because of the unique opportunities presented by the method to survey regions across the whole brain in compliant, conscious participants.
Introduction
Maintenance of body temperature in humans is dependent on sensory, autonomic and behavioral responses. The central nervous system is likely to play a key role in the monitoring of temperature-related signals and the coordination of thermoregulatory responses. Contemporary functional brain imaging techniques provide opportunities to investigate these processes in humans, and there is a growing body of literature that implicates regional brain responses in thermoregulation. This review summarizes the neuroimaging of temperature challenges in order to characterize the regional representation of thermoregulatory-related processes in the human brain.
Neuroimaging approaches to the investigation of thermoregulation in humans
Investigating thermoregulation using functional brain imaging presents unique opportunities in humans, but is also constrained by technical aspects of the imaging methods. The range of neuroimaging studies of thermoregulation reflects this interaction between heuristic objectives and what is feasible, and consequently it is worthwhile devoting some attention to how these factors impact on the research agenda.
The capacity of functional brain imaging techniques to collect information concurrently from all brain regions in conscious humans is a major strength of the method. Albeit at macroscopic levels of spatial resolution, functional images can be used to survey responses across the brain hemispheres at a sampling rate sufficient for the investigation of a multiplicity of motor, sensory and autonomic processes. However, there are constraints on the level of experimental control that can be applied in human experimentation, and functional brain imaging of humans generally provides associative evidence at best. Balanced against procedural constraints and their impact on interpretation of outcomes, is the merit of functional measurements made from the fully integrated nervous system that strengthens the ecological validity of the approach.
Another advantage of human neuroimaging studies is the possibility of collecting complementary information about subjective experience, such as ratings of sensations, which remain latent in animal experiments. These measures can be used to implicate regional brain responses in the coding of sensations with relevance for thermoregulation. The subtleties of language make it possible to examine responses associated with differing discriminative qualities of sensory experience (i.e. coolness, warmth),Citation1,2 as well as the investigation of the hedonic component of temperature sensation (i.e., thermal comfort/discomfort).Citation3,4 These opportunities have been exploited to examine responses to thermal stimuli using functional brain imaging, but the motivation for many studies has been to understand responses to nociceptive levels of stimulation. Frequently, responses to innocuous warming or cooling in neuroimaging studies constitute points of contrast to painful experiences rather than effects of primary interest.Citation5 Furthermore, many of the studies have limited ecological validity for thermoregulation because they involve very small stimulation areas (i.e. 5 to 10 cm2) of brief duration (i.e. 30 sec).Citation6
Temporal factors influence the design of thermoregulatory paradigms used during the acquisition of functional brain images. Implicating regional brain responses in functional processes is dependent on interpretable contrasts of signals measured during different states or events, and these contingencies can occur over a wide time range in the context of thermoregulation. For instance, an isolated sweating event can occur over the course of several seconds. Mapping sweating-related activation in the brain involves measures of regional signal levels during multiple, individual sweating events that are contrasted with signal levels measured during intervening time points when sweating events are not occurring.Citation7-10 This relatively dynamic strategy for the identification of sweating activation is in distinction to the timing of experiments that investigate state-dependent changes in body temperature. Questions about thermoregulation that require contrasts between states such as thermoneutrality, hypothermia and hyperthermia entail the acquisition of functional brain images over tens of minutes to hours in order to gather the data needed for planned comparisons. Most functional brain imaging techniques cannot encompass this temporal range spanning seconds to hours, and consequently the nature of the questions about thermoregulation dictate the type of imaging technique that is used.
Functional magnetic resonance imaging (fMRI) using blood oxygen level-dependent (BOLD) contrast is the most frequently reported functional brain imaging technique reported in the neuroimaging literature. Sequential BOLD images of the whole brain acquired every few seconds at resolutions approximating 40 mm3 are achievable at standard field strengths (i.e. 3 T). The latest ultra-high field scanners (i.e., 7 T) are capable of providing functional information at resolutions less than 1 mm3,Citation11 although this technology has yet to be applied to the investigation of thermoregulatory responses. A time series of sequential images acquired over several minutes can be sufficient to identify regional brain activation in a single participant. However, BOLD signals are of arbitrary intensity and prone to low frequency baseline drifts that make the images incompatible with experimental paradigms involving contrasts over long periods.Citation12 Ideally, contrasts of experimental conditions need to occur in the range of a few seconds to 2 minutes to identify activation using BOLD images. Consequently, standard fMRI is ideal for experiments involving frequent, brief events like sweating, but has serious limitations if applied to comparisons between slowly evolving conditions such as a contrast between thermoneutrality and a hypo or hyperthermia state.
Investigation of regional brain activation associated with contrasts of slowly evolving states is feasible using quantifiable estimates of regional cerebral blood flow (rCBF) or levels of metabolism. Positron emission tomography (PET) using labeled water (H2OCitation15) can be used to estimate rCBF at spatial resolutions of approximately 70 mm3.Citation13 These estimates can be acquired about once every 8 minutes, and averaging of images from multiple participants is usually required to reliably identify regional brain responses.Citation14 Alternatively, the uptake of glucose labeled with 18F (flurodeoxyglucose, FDG) measured with PET provides an index of regional metabolic activity in the brain at a similar spatial resolution to rCBF estimates, but at a much slower temporal resolution.Citation15 The half-life of the compound limits acquisition to a single image from a participant in a scanning session, necessitating samples with multiple participants to show regional brain activation with FDG. Both rCBF and FDG measurements with PET are suitable for experiments examining effects associated with slow changes in body temperature.
Estimates of rCBF can also be obtained using perfusion-fMRI. Arterial spin labeling (ASL) images use radio frequency pulses to magnetically label (tag) water molecules moving in the carotid and vertebral arteries.Citation16 Perfusion levels can be calculated according to the difference in values between paired images of the brain acquired with, and without tagging. Additional parameters are used in combination with the perfusion images to calculate rCBF levels at a temporal resolution of approximately 6 to 8 seconds. Unlike BOLD images, these estimates of rCBF are stable over long periods, which means the technique is suitable for experiments involving slowly changing states.Citation17 The relatively high sampling rate of ASL makes it possible to show activation in a single participant, but large samples are usually needed to show reliable activation because the method has a low level of sensitivity.Citation18
Regional brain responses associated with sustained changes of body temperature
Regional responses in the brain hemispheres to temperature challenge are likely to involve multiple independent and related processes. Sensations arising from body warming or cooling would be expected to have representations in primary and associative cortices that process afferent inputs. Hedonic aspects of temperature sensation are likely to be associated with activity in limbic brain regions. The insula has been implicated in monitoring interoceptive signals and shaping autonomic outputs, and could be involved in responses to temperature changes. Cognitive and behavioral processes precipitated by temperature challenge are likely to have prefrontal correlates that influence responses to cooling or warming. The implication of this speculation is that regional activation associated with thermoregulation would be widely distributed throughout the brain. This proposition has been tested with measures of rCBF and levels of metabolism (FDG) in the brain during thermoneutral, hypothermic and hyperthermic states.
Regional brain responses associated with sustained perturbations of body temperature have been measured with PET and perfusion-fMRI.Citation14,19-21 The objective of these experiments has been to identify brain regions that show significant increases or decreases in activity during whole-body cooling or warming in contrast to a thermoneutral state. The functional roles of brain regions identified by these simple contrasts are by no means definitive, but this approach does indicate those regions that are likely to be involved in temperature monitoring and related processes, and have the potential to reveal a putative thermoregulatory brain network. At this juncture, a clear pattern of response has not emerged among the studies reporting regional responses to temperature challenge.
Two studies involving PET FDG measurement have investigated brain responses associated with whole-body temperature stimuli.Citation19,20 Both studies reported regional responses during hyperthermia and variously described increased metabolism in the cerebellum and bulbar regions. The loci of the brainstem activations are of special interest because bulbar regions have received detailed attention in animal studies, and consequently provide opportunities to compare responses across species. Warming was associated with ventral midbrain activation in one of the human studies,Citation20 at a location approximating the ventral tegmental area. Viral tract tracing and pharmacological studies in animals have indicated that the ventral tegmental area may be involved in responses to hypothermia,Citation22-24 and consequently the reported activity during warming in humans is anomalous. An extensive cluster throughout the rostral and caudal medulla was reported as conjointly activated during both warming and cooling in the second PET FDG study. Accurate localization is necessarily limited in this circumstance, although the dorsal and lateral extent of the rostral component of the cluster was likely to incorporate medullary regions implicated by animal studies in vasomotor responses to coolingCitation25 and thermoregulatory sweating.Citation26-28
Activations in the hypothalamus and posterior cingulate cortex were also reported by one of the PET FDG studies,Citation20 that notably involved a significant increase in core temperature that was not a feature of the alternative study. Another consistency across the studies was a hyperthermia-related deactivation in the insula cortex. Regional activation during body heating has also been assessed using PET and perfusion-fMRI measurement of rCBF.Citation14,21 These studies reported more extensive hemispheric activations that included prefrontal regions (orbitofrontal cortex, dorsolateral prefrontal cortex, inferior frontal gyrus), temporal regions, the posterior parietal cortex, and the anterior and posterior cingulate cortices. However, inconsistencies were also apparent with one study reporting activations in the somatosensory cortex and insula,Citation14 while the other study reported deactivations for these regions.Citation21
Empirical studies of regional brain responses to whole-body cooling have appeared less frequently than studies involving heat stimuli.Citation14,19 The outcomes of the cooling studies are very similar to the regional responses reported for body warming. Measures of PET FDG showed cooling-related activation in the cerebellum and brainstem, and deactivations of the insula and anterior cingulate cortex.Citation19 Extensive hemispheric regions were activated during body cooling in a PET rCBF study that included responses in the prefrontal, temporal and parietal cortices.Citation14 In contradistinction to the study that measured FDG, the measures of rCBF showed activations in the insula and anterior cingulate cortices.
Further replication of studies involving whole-body temperature change will be required before a consensus can be reached on which brain regions are implicated in thermoregulation. However, while outcomes have varied between imaging methods, the distributions of regional responses appear similar for warming and cooling. This is not unexpected, given the spatial resolution of images used to show activation. Individual neurons could feasibly have unitary responses relative to the valence of temperature changes, and it is likely that brain regions involved in thermoregulation would include assemblies of proximate neurones with “cooling” or “warming” related functions. Consequently, a volume of tissue of the voxel dimensions typically measured with PET or perfusion-fMRI could feasibly show both cooling and warming-related activation because constituent neurone populations have heterogeneous thermoregulatory functions. Interestingly, signals from the hypothalamus provide an exception to this general observation. In a single study involving both warming and cooling stimuli, the hypothalamus activated during temperature increases, and deactivated during temperature decreases.Citation14 This outcome is unexpected, given that animal studies consistently report both cooling and warming-related activation, as indexed by c-fos levels, in hypothalamic nuclei.Citation29-32
There are inconsistencies in the literature that are difficult to reconcile. For instance the anterior cingulate cortex has been identified as an activated region by PET rCBF measures,Citation14,21 but has been reported as deactivated during cooling when measured with PET FDG.Citation19 This region has been implicated in somatosensory processing and autonomic responses,Citation6,33 and consequently the report of deactivation in this region is unexpected. Differences between studies in the perceptual and emotional responses to temperature change could provide an explanation for discrepant outcomes. However, analyses of the PET FDG data using sensory parameters were not reported, and consequently this proposition was not tested. Alternatively, the discrepancy between studies could reflect an interaction between the time frames over which the respective imaging methods operate and diminishing levels of activation in the cingulate cortex during sustained stimulation.Citation34 The same explanation would not apply to the reports of deactivation in the insula that were evident during both warming and cooling, and when measured with both PET FDG and perfusion-MRI.Citation19-21 While one study did report insula activation, the more frequent reports of decreased metabolism and rCBF in this region are not expected, given the demonstration of thermosensory functions in the insula in experiments involving small areas of cutaneous stimulation.Citation1
The variability of outcomes across studies makes it difficult to provide defensible summary statements about a putative network of thermoregulatory activation in the human brain. Nevertheless, despite inconsistencies in the direction of signal change (activation, deactivation), the list of brain regions associated with major changes in body temperature conforms to the expectation of a widely distributed network. Furthermore, the locations of these brain regions are also consistent with functional processes that could potentially relate to thermoregulation including processing of thermoafferent inputs (somatosensory, insula, cingulate cortex), autonomic control (insula, cingulate cortex) and planning for behavioral responses (premotor cortex, prefrontal cortex) ().
Figure 1. Regions distributed throughout the hemispheres of the human brain have been implicated in thermoregulation. The primary (SI) and secondary (SII) somatosensory cortices are likely to contribute to sensations associated with temperature changes. The anterior region of the mid cingulate cortex (aMCC) has also been implicated in sensory processing, including coding of the pleasantness or unpleasantness of temperature sensations, as well as a role in the control of autonomic responses. The posterior cingulate cortex (PCC) has also been associated with responses to temperature change, although its role in thermoregulation remains to be established. Activations reported in the premotor and prefrontal cortices during whole body warming and cooling could be related to behavioral responses. In the context of neuroimaging, these behavioral responses are likely to involve efforts to avoid movement in compliance with the experimental protocol, despite thermal sensations prompting actions to restore thermal comfort. Activation in the insula has been identified in association with sensory processing of thermoafferent inputs, which is consistent with the role ascribed the insula in interoception. However, this region has also been reported as deactivated during sustained whole body temperature, although there are also reports of activation in the insula during prolonged warming and cooling.
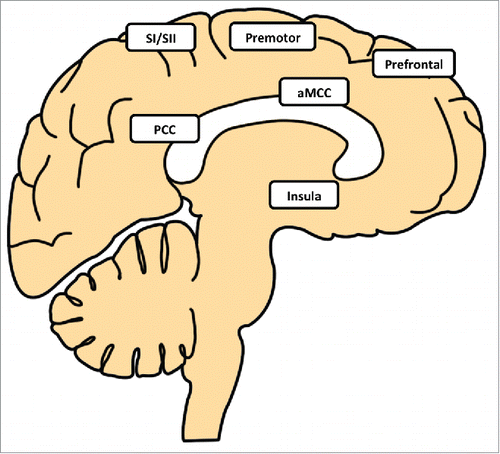
Functional neuroanatomy of temperature sensation
Afferent inputs are critical for thermoregulation. Monitoring of tissue temperature contributes to the control of autonomic effectors of thermoregulatory control, and the sensory experience of temperature change can influence behaviors that impact on energy exchange between body and environment. The investigation of thermoafferent processes in humans has focused on two components of temperature sensation: discrimination and hedonics (comfort/discomfort).
Discriminative dimensions of temperature sensation
Spatially discrete thermal stimuli have been used to identify regional brain responses associated with the sensory experience of temperature change. In most of these reports the small surface area and brief duration of stimuli were not substantive challenges to thermoregulatory stasis, but did evoke discrete temperature sensations. Importantly, investigators have adjusted stimulus temperatures within the innocuous range and manipulated the sites of stimulation to identify brain regions that code stimulus attributes and/or the intensity of perceptual experiences.
The network of brain regions implicated in sensory coding of temperature-related inputs is generally consistent across studies. These regions include the primary and secondary somatosensory cortices, insula, anterior cingulate cortex, and prefrontal cortices.Citation1,35-37 The temperature sensation network is very similar to the regions that activate during the experience of pain, and this common hemispheric anatomy may be a reflection of the shared spinothalamic pathway that conveys both temperature and nociceptive-related inputs from the periphery.
The functional roles of distributed brain regions in the discriminative aspects of temperature sensation are the subject of debate. Data from some studies is consistent with a primary role of the insula in the intensity coding of thermal stimuli.Citation1 Furthermore, the insula shows topographic responses that are consistent with a somatotopic representation of skin temperature changes.Citation35 However, other investigators have provided qualitatively similar outcomes for responses measured in the somatosensory cortices.Citation36,37 Interestingly, the inculpation of either the insula or the somatosensory cortices as the primary temperature-coding cortex appears to be dependent on the valence of temperature change, in that the insula shows graduated responses to increments of skin cooling,Citation1 whereas the intensity of warming stimuli are reflected in the size of signal changes in the somatosensory cortices.Citation36,37 Further experiments will be required to establish the veracity of this possible functional division. In particular, the collection of functional brain images from a single cohort during the application of both cooling and warming stimuli would be a sensible addition to the literature. An early example of this approach has been reported using images with a circumscribed field of view, but must be replicated to provide information about all the salient brain regions.Citation38
Hedonic dimensions of temperature sensation
Thermal stimuli in the non-noxious range can evoke positive or negative affective responses. The experience of temperature change as unpleasant or pleasant is dependent on the prevailing homeostatic state.Citation39 For instance, a cold stimulus applied to the skin when the core temperature is elevated is likely to be described as pleasant, whereas the same stimulus would be rated as unpleasant under hypothermic conditions.Citation40 Arguably, it is this attribute of temperature sensation that could influence the likelihood of behaviors that impact on thermoregulation, such as seeking shelter or clothing choices. Importantly, for the purposes of investigating regional brain responses, the hedonic dimensions of temperature sensation (pleasant, unpleasant) can vary independently of the discriminative attributes of the experience (warm, cool, mild intensity, moderate intensity, etc).Citation39-42 This independent variance makes it possible to search for brain regions where signal changes most accurately match changes in one particular aspect of the multifaceted sensory experience, thus potentially distinguishing between brain regions that code hedonic versus discriminative aspects of temperature sensation.
The earliest functional brain imaging studies of the hedonic dimension of thermal sensation involved cooling or warming of extensive areas of the body surface to evoke prolonged or intermittent experiences of discomfort or comfort.Citation4,43 However, analyses of these studies did not include independent modeling of discriminative aspects of the sensation, and consequently it is unclear to what extent the reported activations were driven by affective processes. Other studies have reported regional brain activations associated with non-painful skin temperature change that partitioned signal variance associated with hedonic ratings from variance associated with discriminative attributes of the stimuli or sensations.Citation3,44,45 All 3 of these studies identified the mid cingulate cortex as a brain region with signal changes predicted by the concurrent level of thermal comfort or discomfort. In addition, one study identified orbitofrontal activations related to hedonic responses, and these activations showed either a medial or lateral localization depending on whether the affective response was positive or negative.Citation45 Collectively, these outcomes are consistent with the important roles posited for the cingulate and orbitofrontal regions in the processing of affective responses and reward contingencies more generally. While yet to be demonstrated definitively, the hedonic dimension of temperature sensation is likely to influence responses that impact on thermoregulation, and prospective contributions from the cingulate and orbitofrontal cortices to motivated behavior is certainly compatible with prevailing knowledge about these brain regions.
Regional brain responses associated with thermoregulatory effectors
Autonomic functions such as vasomotor and sudomotor responses influence body temperature. Animal studies have focused on the hypothalamic and bulbar regions involved in the control of thermoregulatory autonomic responses,Citation46 although it is likely that other hemispheric brain regions are also involved. Human functional brain imaging studies have shown thermoregulatory responses in the hindbrain homologous with other species, and more recently have provided novel insights into the role of hemispheric regions in these processes.
The investigation of bulbar regions involved in thermoregulation in humans presents technical challenges because BOLD images can be adversely influenced by pulsatile movements of the brainstem, and are also subject to geometric distortions related to inhomogeneity of the magnetic field caused by the proximate air filled sinuses.Citation47,48 Additionally, the small sizes of brainstem nuclei approach the limits of spatial resolution of BOLD images. However, optimisation of image acquisitions and additional analytic processes make it feasible to show activation in the brainstem, but usually these approaches do not permit the simultaneous recording of data from both the forebrain and hindbrain. These methods have been applied to the investigation of vasomotor and sudomotor responses during thermal challenge to provide data that supports homology of brainstem nuclei across species. For instance, prolonged cooling of the body leads to graduated BOLD signal increases in the midline, dorsal, rostral medulla consistent with the location of the raphe pallidus nucleus implicated in vasomotor thermoregulatory responses in other animals including the rat and rabbit.Citation49 More recently, human brainstem functional imaging has provided evidence that symmetrical regions of the rostral lateral medulla and the rostral lateral midbrain are involved in the control of sweating ().Citation50 Experiments in cats have previously implicated these regions in sweating control,Citation26-28 although the human brainstem imaging experiment was also able to show that the midbrain and medullary regions were activated during sweating irrespective of whether the impetus was a thermoregulatory or mental challenge.Citation50
Figure 2. Regions of the brainstem have been implicated in the control of thermoregulatory responses in humans. A. The yellows lines indicate the level of axial slices displayed in the remaining panels of the figure. B. An axial slice 5 mm below the anterior commissure (z = −5) has been rendered with activations associated with sweating events in response to a thermal challenge. The yellow arrows point to symmetrical dorsal midbrain regions that show increases in BOLD signal intensity when sweating events occur. C. Sweating activation also occurs in the rostral medulla (z = −49), in symmetrical lateral regions indicated by the yellow arrows. (Data used to create this figure has been presented elsewhere using different analyses and renderings.Citation50)
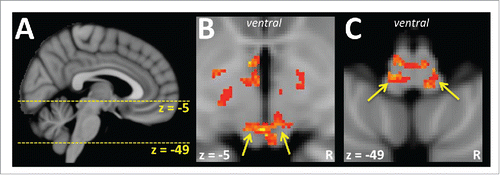
Hemispheric regional activity during sweating events has been investigated using functional brain imaging in humans. A series of studies have reported a distributed network of hemispheric regions with sweating-related signal increases that includes the anterior cingulate cortex, prefrontal cortex, premotor cortex, posterior parietal cortex, insula, and thalamus.Citation7-10 However, all of these studies used mental stress to evoke sweating responses. More recently, a study involving both mental stress and whole body heating was able to directly contrast hemispheric regions activating during sweating events precipitated by the respective challenges.Citation51 This study revealed that many of the regions activated during sweating evoked by mental stress were also activated during sweating events elicited by whole body heating (). However, a region of the mid cingulate cortex was preferentially activated during psychogenic, but not by thermogenic sweating. A body of research has implicated the mid cingulate cortex as a critical region in the control of stress-related autonomic responses.Citation52 The absence of activation in this region during thermogenic sweating could indicate a reduced role of stress and arousal in the context of autonomic outputs contributing to thermoregulatory responses to heating.
Figure 3. Widely distributed brain regions have been implicated in thermoregulation in humans. The panels in this figure include brain activations associated with heating-related sweating as an exemplar of the regions that most consistently show responses during thermal challenges. A. The dotted yellow lines indicate the positions of slices that appear in the remaining panels. Distances in millimeters lateral to the midline of sagittal slices are indicated as ‘x’ values. The axial slice is 12 mm superior of the anterior commissure (z = 12). B. The cingulate cortex frequently activates in studies involving thermal challenge. These activations have been recorded in the posterior cingulate cortex (1) and the anterior cingulate cortex (2). C. The insula cortex (3) has been ascribed a role in temperature sensation and also activates in association with thermoregulatory sudomotor responses. D. The prefrontal cortex (4) is another region that activates during thermal stimulation. (Data used to create this figure has been presented elsewhere using different analyses and renderingsCitation51).
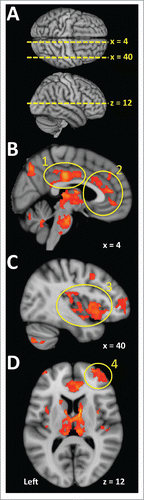
Another notable difference between thermogenic and psychogenic sweating in humans is the appearance of activation in the preoptic area during the former and not the later type of challenge.Citation51 The preoptic area has been implicated by animal experiments as a central control region for thermoregulatory responses, and so it is not surprising that this hypothalamic structure shows activation during thermal challenge in humans. However, the utility of human functional brain imaging to rapidly survey regional activity throughout the brain has provided information about the functional connections of the preoptic area that goes beyond outcomes previously reported in animal research.Citation53 BOLD signals extracted from a region inferior to the anterior commissure that included voxels showing thermogenic sweating activation were used to perform analyses that identified brain regions with signal changes that correlated with the preoptic seed. This analysis revealed widespread brain regions showing levels of functional connectivity with the preoptic area that increased when the body was heated compared to a thermoneutral state, clearly implicating this small hypothalamic region as a key component in a network of neocortical regions that likely contribute to a multiplicity of processes related to thermoregulation.
Brown adipose tissue (BAT) contributes to thermoregulatory responses to cold challenge through thermogenesis, and also plays a role in energy homeostasis. It is only relatively recently that the presence of functional BAT was first demonstrated in adult humans. PET FDG images acquired of the body to screen for cancer provided the first indications of BAT in humans,Citation54 and subsequent studies have examined metabolic responses of BAT to cold challenge in healthy people.Citation55,56 More recently still, PET FDG images have been used to examine metabolic changes in brain regions associated with BAT activation.Citation57,58 However, these 2 studies involved naturalistic comparisons of participants differentiated by the presence or absence of BAT activation at mildly cool temperatures, which may have militated against the demonstration of robust effects. Indeed, there was a virtual absence of corresponding loci of regional brain responses across the studies, with the exception of the inferior parietal lobule that was activated in one instance and deactivated in the other. It appears that additional studies will be required to characterize the hemispheric brain regions in humans that co-activate with BAT.
Disorders of thermoregulation: Regional brain responses associated with hot flashes
Hot flashes are experienced by up to 70% of postmenopausal women and are associated with substantial negative impact on mood, sleep and quality of life.Citation59 The mechanisms contributing to hot flashes remain latent, but there is understandable interest in the role played by the central nervous system in the generation and representation of this symptom. Implicating regional brain signal changes in hot flashes is technically challenging because the events can occur unpredictably, which makes timely image acquisition difficult. Despite these challenges, there have been 2 key publications that report hemodynamic responses measured with BOLD images from brain regions that become active immediately prior to a flash and those regions activating during the vasomotor event itself.Citation60,61 Midbrain regions including the substantia nigra and red nuclei are symmetrically activated approximately 10 seconds prior to the onset of predefined increases in skin conductance levels in groups of symptomatic postmenopausal women.Citation60 The timing of these midbrain responses is compatible with an active role of the regions in the generation of flashes, and differed notably from cingulate, insula and prefrontal regions that activated during the events.Citation60,61 It is notable that electrical and pharmacological stimulations of the substantia nigra and adjacent midbrain regions in animals are associated with heat loss-related responses (i.e. sweating, BAT inhibition). Citation62,63 The timings of later hot flush-related activations are likely to represent sensorimotor responses to sudomotor events, and their loci resonate with the reports of regional brain responses to thermal stimuli discussed in earlier sections.
Conclusion
Functional brain imaging of thermoregulatory responses in humans has revealed 2 main findings. Firstly, bulbar regions implicated in the control of autonomic functions subserving thermoregulation appear to be homologous across species. Discrete bulbar nuclei implicated in both vasomotor and sudomotor responses in humans are localized to regions that correspond with data collected in other animals using alternative imaging techniques. This preservation of functional neuroanatomy lends support to inferences about the likely implications of animal studies for the understanding of human physiology. The second main contribution of human studies is the demonstration of a network of hemispheric regions implicated in thermoregulatory processes. Brain regions including limbic cortex, insula, somatosensory cortex, premotor regions and prefrontal cortex activate in association with thermal challenge. These hemispheric responses in humans add to the body of knowledge contributed by animal studies, which have had a tighter focus on hypothalamic and brainstem regions. The distributed nature of human hemispheric responses is consistent with multiple related functions contributing to thermoregulation. The challenge for future human brain imaging studies is to more accurately dissect the functional neuroanatomy of thermoregulation in hemispheric regions.
Abbreviations
ASL | = | Arterial Spin Labeling |
BAT | = | Brown Adipose Tissue |
BOLD | = | Blood Oxygen Level-Dependent |
FDG | = | Flurodeoxyglucose |
fMRI | = | functional Magnetic Resonance Imaging |
PET | = | Positron Emission Tomography |
rCBF | = | regional Cerebral Blood Flow |
Disclosure of potential conflicts of interest
No potential conflicts of interest were disclosed.
References
- Craig AD, Chen K, Bandy D, Reiman EM. Thermosensory activation of insular cortex. Nat Neurosci 2000; 3:184-90; PMID:10649575; http://dx.doi.org/10.1038/72131
- Davis KD, Pope GE, Crawley AP, Mikulis DJ. Perceptual illusion of “paradoxical heat” engages the insular cortex. J Neurophysiol 2004; 92:1248-51; PMID:15277602; http://dx.doi.org/10.1152/jn.00084.2004
- Farrell MJ, Johnson J, McAllen RM, Zamarripa F, Denton DA, Fox PT, Egan GE. Brain activity associated with ratings of the hedonic component of thermal sensation during whole-body warming and cooling. J Thermal Biol 2011; 36:57-63; http://dx.doi.org/10.1016/j.jtherbio.2010.11.003
- Kanosue K, Sadato N, Okada T, Yoda T, Nakai S, Yoshida K, Hosono T, Nagashima K, Yagishita T, Inoue O, et al. Brain activation during whole body cooling in humans studied with functional magnetic resonance imaging. Neurosci Lett 2002; 329:157-60; PMID:12165401; http://dx.doi.org/10.1016/S0304-3940(02)00621-3
- Davis KD, Moayedi M. Central mechanisms of pain revealed through functional and structural MRI. J Neuroimmune Pharmacol 2013; 8:518-34; PMID:22825710; http://dx.doi.org/10.1007/s11481-012-9386-8
- Farrell MJ, Laird AR, Egan GF. Brain activity associated with painfully hot stimuli applied to the upper limb: a meta-analysis. Human brain mapping 2005; 25:129-39; PMID:15846813; http://dx.doi.org/10.1002/hbm.20125
- Fredrikson M, Furmark T, Olsson MT, Fischer H, Andersson J, Langstrom B. Functional neuroanatomical correlates of electrodermal activity: a positron emission tomographic study. Psychophysiology 1998; 35:179-85; PMID:9529944; http://dx.doi.org/10.1111/1469-8986.3520179
- Nagai Y, Critchley HD, Featherstone E, Trimble MR, Dolan RJ. Activity in ventromedial prefrontal cortex covaries with sympathetic skin conductance level: a physiological account of a “default mode” of brain function. NeuroImage 2004; 22:243-51; PMID:15110014; http://dx.doi.org/10.1016/j.neuroimage.2004.01.019
- Patterson JC, 2nd, Ungerleider LG, Bandettini PA. Task-independent functional brain activity correlation with skin conductance changes: an fMRI study. NeuroImage 2002; 17:1797-806; PMID:12498753; http://dx.doi.org/10.1006/nimg.2002.1306
- Williams LM, Brammer MJ, Skerrett D, Lagopolous J, Rennie C, Kozek K, Olivieri G, Peduto T, Gordon E. The neural correlates of orienting: an integration of fMRI and skin conductance orienting. Neuroreport 2000; 11:3011-5; PMID:11006985; http://dx.doi.org/10.1097/00001756-200009110-00037
- Satpute AB, Wager TD, Cohen-Adad J, Bianciardi M, Choi JK, Buhle JT, Wald LL, Barrett LF. Identification of discrete functional subregions of the human periaqueductal gray. Proc Natl Acad Sci U S A 2013; 110:17101-6; PMID:24082116; http://dx.doi.org/10.1073/pnas.1306095110
- Smith AM, Lewis BK, Ruttimann UE, Ye FQ, Sinnwell TM, Yang Y, Duyn JH, Frank JA. Investigation of low frequency drift in fMRI signal. NeuroImage 1999; 9:526-33; PMID:10329292; http://dx.doi.org/10.1006/nimg.1999.0435
- Fox PT, Raichle ME. Stimulus rate dependence of regional cerebral blood flow in human striate cortex, demonstrated by positron emission tomography. J Neurophysiol 1984; 51:1109-20; PMID:6610024
- Egan GF, Johnson J, Farrell M, McAllen R, Zamarripa F, McKinley MJ, Lancaster J, Denton D, Fox PT. Cortical, thalamic, and hypothalamic responses to cooling and warming the skin in awake humans: a positron-emission tomography study. Proc Natl Acad Sci U S A 2005; 102:5262-7; PMID:15793009; http://dx.doi.org/10.1073/pnas.0409753102
- Kushner MJ, Rosenquist A, Alavi A, Rosen M, Dann R, Fazekas F, Bosley T, Greenberg J, Reivich M. Cerebral metabolism and patterned visual stimulation: a positron emission tomographic study of the human visual cortex. Neurology 1988; 38:89-95; PMID:3257295; http://dx.doi.org/10.1212/WNL.38.1.89
- Williams DS, Detre JA, Leigh JS, Koretsky AP. Magnetic resonance imaging of perfusion using spin inversion of arterial water. Proc Natl Acad Sci U S A 1992; 89:212-6; PMID:1729691; http://dx.doi.org/10.1073/pnas.89.1.212
- Wang J, Aguirre GK, Kimberg DY, Roc AC, Li L, Detre JA. Arterial spin labeling perfusion fMRI with very low task frequency. Magn Reson Med 2003; 49:796-802; PMID:12704760; http://dx.doi.org/10.1002/mrm.10437
- Murphy K, Harris AD, Diukova A, Evans CJ, Lythgoe DJ, Zelaya F, Wise RG. Pulsed arterial spin labeling perfusion imaging at 3 T: estimating the number of subjects required in common designs of clinical trials. Magn Reson Imaging 2011; 29:1382-9; PMID:21546190; http://dx.doi.org/10.1016/j.mri.2011.02.030
- Fechir M, Klega A, Buchholz HG, Pfeifer N, Balon S, Schlereth T, Geber C, Breimhorst M, Maihofner C, Birklein F, et al. Cortical control of thermoregulatory sympathetic activation. Eur J Neurosci 2010; 31:2101-11; PMID:20529117; http://dx.doi.org/10.1111/j.1460-9568.2010.07243.x
- Nunneley SA, Martin CC, Slauson JW, Hearon CM, Nickerson LD, Mason PA. Changes in regional cerebral metabolism during systemic hyperthermia in humans. J Appl Physiol 2002; 92:846-51; PMID:11796700; http://dx.doi.org/10.1152/japplphysiol.00072.2001
- Qian S, Jiang Q, Liu K, Li B, Li M, Li L, Yang X, Yang Z, Sun G. Effects of short-term environmental hyperthermia on patterns of cerebral blood flow. Physiol Behav 2014; 128:99-107; PMID:24530482; http://dx.doi.org/10.1016/j.physbeh.2014.01.028
- Kalivas PW, Nemeroff CB, Miller JS, Prange AJ, Jr. Microinjection of neurotensin into the ventral tegmental area produces hypothermia: evaluation of dopaminergic mediation. Brain Res 1985; 326:219-27; PMID:2982460; http://dx.doi.org/10.1016/0006-8993(85)90031-9
- Smith JE, Jansen AS, Gilbey MP, Loewy AD. CNS cell groups projecting to sympathetic outflow of tail artery: neural circuits involved in heat loss in the rat. Brain Res 1998; 786:153-64; PMID:9554992; http://dx.doi.org/10.1016/S0006-8993(97)01437-6
- Zhang YH, Hosono T, Yanase-Fujiwara M, Chen XM, Kanosue K. Effect of midbrain stimulations on thermoregulatory vasomotor responses in rats. J Physiol 1997; 503 (Pt 1):177-86; PMID:9288685; http://dx.doi.org/10.1111/j.1469-7793.1997.177bi.x
- McAllen RM, Tanaka M, Ootsuka Y, McKinley MJ. Multiple thermoregulatory effectors with independent central controls. Eur J Appl Physiol 2010; 109:27-33; PMID:19949811; http://dx.doi.org/10.1007/s00421-009-1295-z
- Davison MA, Koss MC. Brainstem loci for activation of electrodermal response in the cat. Am J Physiol 1975; 229:930-4; PMID:1190335
- McAllen RM. Action and specificity of ventral medullary vasopressor neurones in the cat. Neuroscience 1986; 18:51-9; PMID:2874523; http://dx.doi.org/10.1016/0306-4522(86)90178-8
- Shafton AD, McAllen RM. Location of cat brain stem neurons that drive sweating. Am J Physiol Regul Integr Comp Physiol 2013; 304:R804-9; PMID:23467325; http://dx.doi.org/10.1152/ajpregu.00040.2013
- Bratincsak A, Palkovits M. Activation of brain areas in rat following warm and cold ambient exposure. Neuroscience 2004; 127:385-97; PMID:15262329; http://dx.doi.org/10.1016/j.neuroscience.2004.05.016
- Kiyohara T, Miyata S, Nakamura T, Shido O, Nakashima T, Shibata M. Differences in Fos expression in the rat brains between cold and warm ambient exposures. Brain Res Bull 1995; 38:193-201; PMID:7583347; http://dx.doi.org/10.1016/0361-9230(95)00093-T
- McKitrick DJ. Expression of fos in the hypothalamus of rats exposed to warm and cold temperatures. Brain Res Bull 2000; 53:307-15; PMID:11113585; http://dx.doi.org/10.1016/S0361-9230(00)00346-4
- Yoshida K, Konishi M, Nagashima K, Saper CB, Kanosue K. Fos activation in hypothalamic neurons during cold or warm exposure: projections to periaqueductal gray matter. Neuroscience 2005; 133:1039-46; PMID:15927405; http://dx.doi.org/10.1016/j.neuroscience.2005.03.044
- Critchley HD, Corfield DR, Chandler MP, Mathias CJ, Dolan RJ. Cerebral correlates of autonomic cardiovascular arousal: a functional neuroimaging investigation in humans. J Physiol 2000; 523 Pt 1:259-70; PMID:10673560; http://dx.doi.org/10.1111/j.1469-7793.2000.t01-1-00259.x
- Owen DG, Bureau Y, Thomas AW, Prato FS, St Lawrence KS. Quantification of pain-induced changes in cerebral blood flow by perfusion MRI. Pain 2008; 136:85-96; PMID:17716820; http://dx.doi.org/10.1016/j.pain.2007.06.021
- Hua leH, Strigo IA, Baxter LC, Johnson SC, Craig AD. Anteroposterior somatotopy of innocuous cooling activation focus in human dorsal posterior insular cortex. Am J Physiol Regul Integr Comp Physiol 2005; 289:R319-R25; PMID:15805097; http://dx.doi.org/10.1152/ajpregu.00123.2005
- Moulton EA, Keaser ML, Gullapalli RP, Greenspan JD. Regional intensive and temporal patterns of functional MRI activation distinguishing noxious and innocuous contact heat. J Neurophysiol 2005; 93:2183-93; PMID:15601733; http://dx.doi.org/10.1152/jn.01025.2004
- Moulton EA, Pendse G, Becerra LR, Borsook D. BOLD responses in somatosensory cortices better reflect heat sensation than pain. J Neurosci 2012; 32:6024-31; PMID:22539862; http://dx.doi.org/10.1523/JNEUROSCI.0006-12.2012
- Davis KD, Kwan CL, Crawley AP, Mikulis DJ. Functional MRI study of thalamic and cortical activations evoked by cutaneous heat, cold, and tactile stimuli. J Neurophysiol 1998; 80:1533-46; PMID:9744957
- Cabanac M. Sensory pleasure. Quarterly Rev Biol 1979; 54:1-29; PMID:379894; http://dx.doi.org/10.1086/410981
- Mower GD. Perceived intensity of peripheral thermal stimuli is independent of internal body temperature. J Comparative Physiol Psychol 1976; 90:1152-5; PMID:993393; http://dx.doi.org/10.1037/h0077284
- Attia M. Thermal pleasantness and temperature regulation in man. Neurosci Biobehavioral Rev 1984; 8:335-42; PMID:6504416; http://dx.doi.org/10.1016/0149-7634(84)90056-3
- Cabanac M. Physiological role of pleasure. Science 1971; 173:1103-7; PMID:5098954; http://dx.doi.org/10.1126/science.173.4002.1103
- Sung EJ, Yoo SS, Yoon HW, Oh SS, Han Y, Park HW. Brain activation related to affective dimension during thermal stimulation in humans: a functional magnetic resonance imaging study. Int J Neurosci 2007; 117:1011-27; PMID:17613111; http://dx.doi.org/10.1080/00207450600934432
- Kulkarni B, Bentley DE, Elliott R, Youell P, Watson A, Derbyshire SW, Frackowiak RS, Friston KJ, Jones AK. Attention to pain localization and unpleasantness discriminates the functions of the medial and lateral pain systems. Eur J Neurosci 2005; 21:3133-42; PMID:15978022; http://dx.doi.org/10.1111/j.1460-9568.2005.04098.x
- Rolls ET, Grabenhorst F, Parris BA. Warm pleasant feelings in the brain. NeuroImage 2008; 41:1504-13; PMID:18468458; http://dx.doi.org/10.1016/j.neuroimage.2008.03.005
- Nakamura K. Central circuitries for body temperature regulation and fever. Am J Physiol Regul Integr Comp Physiol 2011; 301:R1207-28; PMID:21900642; http://dx.doi.org/10.1152/ajpregu.00109.2011
- Brooks JC, Faull OK, Pattinson KT, Jenkinson M. Physiological noise in brainstem FMRI. Front Hum Neurosci 2013; 7:623; PMID:24109446; http://dx.doi.org/10.3389/fnhum.2013.00623
- Pattinson KT, Mitsis GD, Harvey AK, Jbabdi S, Dirckx S, Mayhew SD, Rogers R, Tracey I, Wise RG. Determination of the human brainstem respiratory control network and its cortical connections in vivo using functional and structural imaging. NeuroImage 2009; 44:295-305; PMID:18926913; http://dx.doi.org/10.1016/j.neuroimage.2008.09.007
- McAllen RM, Farrell M, Johnson JM, Trevaks D, Cole L, McKinley MJ, Jackson G, Denton DA, Egan GF. Human medullary responses to cooling and rewarming the skin: a functional MRI study. Proc Natl Acad Sci U S A 2006; 103:809-13; PMID:16407125; http://dx.doi.org/10.1073/pnas.0509862103
- Farrell MJ, Trevaks D, Taylor NA, McAllen RM. Brain stem representation of thermal and psychogenic sweating in humans. Am J Physiol Regul Integr Comp Physiol 2013; 304:R810-7; PMID:23535458; http://dx.doi.org/10.1152/ajpregu.00041.2013
- Farrell MJ, Trevaks D, Taylor NA, McAllen RM. Regional brain responses associated with thermogenic and psychogenic sweating events in humans. J Neurophysiol 2015; 114:2578-87; PMID:26289468; http://dx.doi.org/10.1152/jn.00601.2015
- Critchley HD, Eccles J, Garfinkel SN. Interaction between cognition, emotion, and the autonomic nervous system. Handbook Clin Neurol 2013; 117:59-77; PMID:24095116; http://dx.doi.org/10.1016/B978-0-444-53491-0.00006-7
- Farrell MJ, Trevaks D, McAllen RM. Preoptic activation and connectivity during thermal sweating in humans. Temperature 2014; 1:135-41; http://dx.doi.org/10.4161/temp.29667
- Nedergaard J, Bengtsson T, Cannon B. Unexpected evidence for active brown adipose tissue in adult humans. Am J Physiol Endocrinol Metab 2007; 293:E444-52; PMID:17473055; http://dx.doi.org/10.1152/ajpendo.00691.2006
- van Marken Lichtenbelt WD, Vanhommerig JW, Smulders NM, Drossaerts JM, Kemerink GJ, Bouvy ND, Schrauwen P, Teule GJ. Cold-activated brown adipose tissue in healthy men. N Engl J Med 2009; 360:1500-8; PMID:19357405; http://dx.doi.org/10.1056/NEJMoa0808718
- Virtanen KA, Lidell ME, Orava J, Heglind M, Westergren R, Niemi T, Taittonen M, Laine J, Savisto NJ, Enerback S, et al. Functional brown adipose tissue in healthy adults. N Engl J Med 2009; 360:1518-25; PMID:19357407; http://dx.doi.org/10.1056/NEJMoa0808949
- Huang YC, Hsu CC, Huang P, Yin TK, Chiu NT, Wang PW, Huang SH, Huang YE. The changes in brain metabolism in people with activated brown adipose tissue: a PET study. NeuroImage 2011; 54:142-7; PMID:20682349; http://dx.doi.org/10.1016/j.neuroimage.2010.07.058
- Miao Q, Zhao XL, Zhang QY, Zhang ZY, Guan YH, Ye HY, Zhang S, Zeng MF, Zuo CT, Li YM. Stability in brain glucose metabolism following brown adipose tissue inactivation in chinese adults. AJNR Am J Neuroradiol 2012; 33:1464-9; PMID:22576895; http://dx.doi.org/10.3174/ajnr.A3006
- Gold EB, Colvin A, Avis N, Bromberger J, Greendale GA, Powell L, Sternfeld B, Matthews K. Longitudinal analysis of the association between vasomotor symptoms and race/ethnicity across the menopausal transition: study of women's health across the nation. Am J Public Health 2006; 96:1226-35; PMID:16735636; http://dx.doi.org/10.2105/AJPH.2005.066936
- Diwadkar VA, Murphy ER, Freedman RR. Temporal sequencing of brain activations during naturally occurring thermoregulatory events. Cereb Cortex 2014; 24:3006-13; PMID:23787950; http://dx.doi.org/10.1093/cercor/bht155
- Freedman RR, Benton MD, Genik RJ, 2nd, Graydon FX. Cortical activation during menopausal hot flashes. Fertil Steril 2006; 85:674-8; PMID:16500337; http://dx.doi.org/10.1016/j.fertnstert.2005.08.026
- Hajos M, Engberg G, Nissbrandt H, Magnusson T, Carlsson A. Capsaicin-sensitive vasodilatatory mechanisms in the rat substantia nigra and striatum. J Neural Transm 1988; 74:129-39; PMID:3210010; http://dx.doi.org/10.1007/BF01244779
- Hashimoto M, Arita J, Shibata M. Electrical stimulation of the lower midbrain around retrorubral field decreases temperatures of brown fat and rectum in anesthetized Wistar rats. Neurosci Lett 1998; 246:129-32; PMID:9792609; http://dx.doi.org/10.1016/S0304-3940(98)00206-7