In many places on earth, the temperature drops below zero and water freezes for prolonged periods. Plenty of organisms thrive there. How do they survive the freezing conditions? One of the major strategies taken by evolution is production of ice-binding proteins (IBPs) that bind to surfaces of ice crystals and control their growth and shaping. In fish and insects, for example, IBPs depress the freezing temperature of the body fluids below the freezing temperature of the surrounding, preventing freezing of the organism even when ice is all around. In plants and other freeze-tolerant microorganisms, IBPs keep ice crystals small, both inside and out of the cells. The growth of large ice crystals is lethal due to dehydration of the cells and to mechanical damage to cellular membranes. Another role of IBPs in nature was suggested for some Antarctic microorganisms that secrete IBPs to the medium. These IBPs are thought to help their hosts by keeping their surroundings liquid.Citation1
The Antarctic bacterium Marinomonas primoryensis has a peculiar IBP (MpIBP) that does not function in any of the strategies explained above. Rather, this protein helps the bacterium stick to ice and hang on it, as we show in our recent work.Citation2 Our studies focused on visualizing live bacteria in solutions that contained ice crystals in the micron scale. Following the ice crystals over time, we observed that the bacteria swim freely in the solution and bind to the surface of the ice when they accidently bounce it. After the bacteria are bound to the ice, they only leave it if the ice melts, which supports the general hypothesis that the binding of IBPs to ice is an irreversible process.Citation1 The bound bacteria are not frozen to the ice, and many of them continue to rotate their bodies as if they were tethered to the ice by a thread connected to their outer membrane. When an antibody raised against the ice-binding part of MpIBP was added to the solution, the bacteria could not bind the ice anymore, providing evidence that the binding was due to the presence of active MpIBP on the membrane. These findings are in accordance with previous structural and bioinformatics studies conducted on the protein.Citation3
Our discovery was made possible by combining microscopy tools, the microfluidics technology, and temperature-controlled cells which were designed in our lab for the study of IBPs mechanism. One apparatus we used is the mini cold finger (MCF), as shown in . This system consists of a temperature-controlled cell and a microfluidic chip that has a brass wire (functioning as the cold finger) embedded inside it, touching the microfluidic channel from the top. The temperature of the microfluidic device is controlled from two independent positions; one is below the chip and the other is from the cold finger. This configuration allows fine temperature control of the local environment within the microfluidic device and opens the opportunity for experiments that require confining temperature gradients. Using the MCF technology we were able to inject live bacteria into a microfluidic channel that already contained an ice crystal inside it. The cold finger allowed us to do so without melting the crystal inside the channel during the injection and without the need to freeze and thaw the bacteria. The MCF was published in our work on MpIBP for the first time, but the system is applicable also for different kinds of measurements. In a separate study, we used the MCF to follow the affinity of several IBPs to the basal plane of ice over time and to measure their binding kinetics.Citation4
Figure 1. M. primoryensis in the MCF device. A schematic representation of the microfluidic chip with the cold finger is presented in the left. The chip is shown upside down, and the microfluidic channel can be seen. The brass cold-finger (orange) is embedded in the middle of the chip, cooling the central part of the microfluidic well. The ice crystal grows around the tip of the cold finger in a circular shape. On the right is an enlargement of a part of the ice crystal, showing the bacteria bound to the ice surface. The black spot on the left is the tip of the cold finger. The ice-binding proteins are shown in green, and the flagella are in brown.
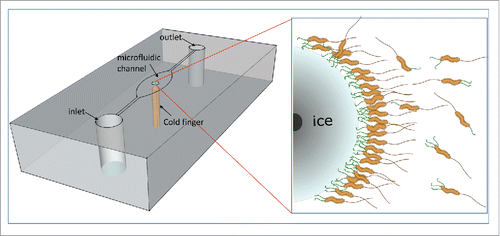
Why would a bacterium that does not like freezing and can swim fast around 0°C want to adhere to ice? It was suggested that this aerobic bacterium wants to keep its position at the upper layer of the lake, where not only ice is abundant, but also oxygen and nutrients.Citation3 It is also possible that the adhesion of M. primoryensis serves other microorganisms living in the same community in keeping their position in brine pockets, where food is abundant. The ability of this bacterium to adhere to ice suggests that biofilm can be formed on ice surface via this protein. Formation of a biofilm in a liquid environment where ice is present and the temperature is around 0°C may take weeks or even longer, as biofilm regularly takes at least a few days to form even in room temperature.
The discovery of bacteria that adheres to ice presents the first example of an organism that uses the adhesion principle to bind to ice surface and broadens the scope of IBPs roles in nature. It seems as if IBPs were evolved not only to protect organisms from freeze damage, but also for other purposes. From the practical point of view, one may think of blocking bacterial adhesion as presented in our work as a strategy to prevent the formation of biofilms in places where they are unwanted, such as in the food industry. We anticipate that other microorganisms with ice adhesion proteins will be discovered, and perhaps IBPs with additional functions that are yet to be revealed.
References
- Bar-Dolev M, Braslavsky I, Davies PL. Annu Rev Biochem. 2016;85:515-42; doi:10.1146/annurev-biochem-060815-014546
- Bar-Dolev M, Bernheim R, Guo S, Davies PL, Braslavsky I. J Roy Soc Interface. 2016;13:20160210; PMID: 27534698; doi:10.1098/rsif.2016.0210
- Shuaiqi G, Garnham C, Whitney J, Graham L, Davies PL. PLoS one. 2012;7:e488705; doi:10.1371/journal.pone.0048805
- Haleva L, Celik Y, Bar-Dolev M, Pertaya-Braun N, Kaner A, Davies PL, Braslavsky I. Biophysical J. 2016;111:1143-50;doi:10.1016/j.bpj.2016.08.003