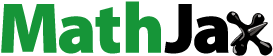
ABSTRACT
Increasing physical activity remains the most widely publicized way of improving health and wellbeing. However, in populations that benefit most from exercise (EX), adherence is often poor and alternatives to EX are important to bring about health improvements. Recent work suggests a role for passive heating (PH) and heat shock proteins (HSP) in improving cardio-metabolic health. The aim of this study was to investigate the expression of HSP70 and interleukin-6 in response to either EX or PH and the subsequent effect on glucose control. Fourteen males volunteered and were categorized lean (BMI 23.5 ± 2.2 kg·m−2) or overweight (29.2 ± 2.7 kg·m−2) and completed 60 minutes of either moderate cycling at a fixed rate of metabolic heat production (EX) or warm water immersion in 40°C water (PH). Extracellular HSP70 increased from baseline in both conditions with no differences between PH (0.98 ± 1.1 ng·mL−1) or EX (0.84 ± 1.0 ng·mL−1, p = 0.814). IL-6 increased following both conditions with a two-fold increase after PH and four-fold after EX. Energy expenditure increased by 61.0 ± 14.4 kcal·h−1 (79%) after PH. Peak glucose concentration after a meal immediately following PH was reduced when compared with EX (6.3 ± 1.4 mmol·L−1 versus 6.8 ± 1.2 mmol·L−1; p < 0.05). There was no difference in 24-hour glucose area under the curve (AUC) between conditions. These data indicate the potential for thermal therapy as an alternative treatment and management strategy for those at risk of developing metabolic disease where adherence, or ability to EX, may be compromised.
Introduction
Overweight and obesity are characterized by chronic inflammation and impairments to insulin sensitivity and glucose control. A multitude of factors contribute to enhancing health and wellbeing; increasing physical activity remains the most widely publicized way of doing so. However, in populations that benefit most from exercise (EX), adherence is often poor, most likely due to medical conditions and disability,Citation1 poor motivation and a lack of convenience.Citation2,Citation3 There are many factors involved in the development and treatment of insulin resistance, with the roles played by both thermal therapy and heat shock proteins (HSPs) receiving increased attention.Citation4-Citation7 Furthermore, it has been suggested that HSPs may provide an early indicator of the onset of metabolic diseases, such as type 2 diabetes (T2DM),Citation8,Citation9 while also acting as a possible biomarker for the chronicity of diabetes.Citation10
HSPs are synthesized in response to a number of physiological stressors,Citation11–Citation13 and are individually characterized by their molecular weight. The most widely studied HSP in humans is the 70 kDa family of HSPs which includes both the constitutive HSP73 and inducible HSP72 forms. HSPs have wide-ranging functions including their roles as thermo-protectants and involvement in protein synthesis.Citation14 The resting level of extracellular HSP70 (eHSP70) appears to be related to adiposity and inflammation with elevations reported in obesity and T2DM, whereas resting intracellular HSP70 (iHSP70) is decreased in T2DM.Citation9 A reduction in iHSP70 is an important component in the vicious cycle of chronic inflammation and reduced insulin signallingCitation15,Citation16 and the development of insulin resistance.Citation17,Citation18 Indeed, a reduction in iHSP70 has been reported in T2DM and correlates to insulin resistance and glucose disposal rates.Citation19,Citation20
eHSP70 is increased following acute EXCitation13 with the magnitude of change dependent on both the duration and intensity.Citation21,Citation22 A core temperature (T c) threshold for elevations in eHSP70 has been suggested, with a 0.8–1.5°C increase in T c being required in order to elevate eHSP70.Citation23,Citation24 Recently, thermal therapy has been demonstrated to stimulate HSP70 production, although to a lesser extent than following EX,Citation4,Citation24 and may offer an alternative way by which the positive effects of HSP may be induced.Citation9
The influence of thermal therapy on glycemic regulation and metabolic disease has primarily been investigated using rodent models.Citation17,Citation18,Citation25–Citation27. However, there are human data to suggest that local heating at the site of insulin infusion improves postprandial insulin actionCitation28,Citation29 and may benefit long-term glycemic controlCitation29,Citation30 and cardiovascular health in humans.Citation31 However, the mechanisms by which such an effect may occur in humans remain to be elucidated. Chung et al. were the first to demonstrate that passive heating (PH) of mice resulted in an elevation in iHSP70 which was protective against the deleterious effects of consuming a high-fat diet.Citation18 They report that in response to a high-fat diet there was an increase in the phosphorylation of c-Jun N-terminal kinase (JNK). Such an increase has been linked to impaired insulin sensitivity due to JNK binding with the insulin receptor substrate, thus preventing the initiation of the insulin signaling cascade and resulting in an elevation in plasma glucose (For review, the reader is directed to ref.Citation[32]). Importantly, Chung et al. demonstrated that PH attenuated hyperinsulinemia, glucose intolerance and insulin resistance and as a direct consequence of elevations in HSP70 and was closely associated with prevention of JNK phosphorylation. Therefore, there is a need to investigate how well data from these rodent models translate into human participants, with a specific focus on the ability of thermal therapy to elevate HSP70 and improve glycemic control.
HSPs may impact on the inflammatory state by directly inducing pro-inflammatory cytokines. Regular EX has been demonstrated to have a positive effect on the inflammatory profile that is suggested to occur as a consequence of the repeated exposure to an anti-inflammatory environment following each EX bout.Citation33 This may occur as a consequence of secretion of pro-inflammatory cytokines, including interleukin-6 (IL-6) that may in turn up-regulate anti-inflammatory cytokines.Citation34 Importantly, it has been shown that HSP70 independently induces pro-inflammatory cytokines.Citation35,Citation36 Therefore, thermal therapy may be able to replicate some of the anti-inflammatory benefits of EX, which could help reduce the level of inflammation evident in many types of chronic disease.
While EX has significant benefits to improving health, individuals with many types of chronic disease often experience low EX tolerance and poor rates of adherence.Citation1,Citation37 Based on the above animal literature, there is evidence to suggest that thermal therapy may replicate some of the health benefits of EX and alleviate some of the comorbidities often associated with chronic diseases such as T2DM.Citation38 If successful, the implementation of thermal therapy may help to lessen some of the financial burden of treating chronic by potentially reducing the dependence on pharmacological interventions. Thermal therapy could offer a simple home-based intervention that may appeal to individuals unable or unwilling to participate in regular EX.
The primary aim of this study was to investigate the expression of eHSP70 in response to either EX or thermal therapy in the form of PH via warm water immersion. A secondary aim was to investigate the effect of body composition on the eHSP70 response. Finally, we wished to investigate the potential effect of PH on glycemic control in response to replicated dietary intake. It was hypothesized that both EX and PH would elevate eHSP70, with a larger magnitude of change following EX. It was further hypothesized that there would be a differential response in eHSP70 between lean and overweight participant groups.
Methods
Participants
A total of 14 males volunteered to participate in the study and were split into two groups: (i) Lean (LEAN, n = 7; BMI < 25 kg·m−2, body fat < 15% and fat mass < 12 kg) and (ii) Overweight (OW, n = 7; BMI > 27.0 kg·m−2, body fat > 20% and fat mass > 25 kg). Participant characteristics are provided in . All participants were healthy non-smokers with no history of cardiovascular, hematological or metabolic disorders and had been weight stable for ≥ 3 months. Participants were habitually inactive; performing less than 1.5 hours of structured physical activity per week and had avoided any hot weather exposure in the previous two months, including frequent sauna or spa use.
Table 1. Participant characteristics.
Ethical approval
Full ethical approval was granted by the Loughborough University Ethical Advisory Committee. All procedures conformed to the principles defined in the Declaration of Helsinki. Participants were fully informed of the experimental protocols and any potential risks were identified before they provided their written consent to participate.
Experimental overview
Participants visited the laboratory on three occasions. The preliminary visit to the laboratory consisted of initial measures including a blood profile, body composition and both submaximal and maximal oxygen uptake tests ( test). Visits two and three comprised the experimental trials which consisted of either 60 minutes of PH via warm water immersion (PH), or 60 minutes of EX at a fixed rate of metabolic heat production (EX) which were completed in a counterbalanced order and were matched for ΔT
c. On arrival, participants were asked to void their bladder and were weighed in minimal clothing and then fully instrumented. The first blood sample was then collected and muscle temperature measured. Participants then completed either PH or EX as detailed below. On completion of each trial, a second blood sample and muscle temperature measure were obtained, with a final blood sample being taken 2 hours after completion (). Experimental trials were separated by a minimum of 5 days to reduce any acclimation effectCitation39 and to minimize any effect of prior EX or heating upon insulin sensitivity.Citation40 All trials were completed at the same time of day to minimize effects of circadian variation.
Preliminary visit
Participants arrived in a fasted state and a capillary blood sample was drawn for assessment of total cholesterol, high-density lipoprotein cholesterol (HDL-C), low-density lipoprotein cholesterol (LDL-C), glucose and triglycerides (TG) (Cardiochek, Polymer Technology Systems, Indianapolis, IN). Participants' height and weight were recorded (Seca 360, Birmingham, UK), and BMI calculated. Body composition was determined with skinfold callipers (Harpenden, Warwickshire, UK) using the seven-site formula.Citation41 Participants then completed a submaximal EX test on an electronically braked cycle ergometer (Lode Excalibur Sport, Groningen, Netherlands) with an online gas analyzer (Metamax 3B, Cortex Biophysik GmbH, Germany). The test comprised four stages, increasing by 20 W every 4 minutes. and
were determined over a 30-second rolling average from the final minute of each stage. On completion of the submaximal test, participants were given a minimum of a 10-minute recovery period prior to commencing the
test. This test used a continual ramp protocol at a rate of 20 W·min−1 until the participant reached volitional exhaustion.
was determined over a 30-second rolling average from the final minute of the test.
Experimental trials
Seventy-two hours prior to the start of each experimental trial, participants reported to the laboratory where they were fitted with a continuous glucose monitor device (CGM; Freestyle Libre, Abbott Laboratories, Berkshire). The sensor was inserted on the posterior aspect of the upper arm, with interstitial glucose measured every 15 minutes. CGM data were analyzed for 2-hours post meal glucose AUC and peak glucose concentration.Citation42 Participants were provided with scales (HoMedics Group Salter, Kent) and detailed instruction on how to measure dietary intake in order to accurately complete a 3-day diet diary during the first trial, which commenced 24 hours before and ended 24 hours after completion of trial 1. Participants were instructed to consume the first meal after each condition following a standardized 3-hour time period. Participants were required to replicate their dietary intake and timing of meals for the second trial.
Exercise trial
Participants performed 1-hour cycling at a fixed rate of metabolic heat production () equivalent to 7 W/kg in an environmental chamber (25.6 ± 0.7°C; 49.8 ± 3.8% relative humidity, rh). The initial workload was determined from the submaximal test and external workload was manipulated throughout the 60 minutes in order to maintain
at 7 W/kg. Participants completed the EX at a self-selected cadence.
were recorded throughout using an online gas analyzer. Airflow was provided by three fans stacked vertically, positioned 2.5 m in front of the ergometer at an air speed of 1.5 m·s−1.
Passive heating trial
Participants were seated in a water bath (40.2 ± 0.2 °C) and immersed up to the waist for 1 hour. Water was circulated throughout to ensure maintenance of water temperature. and
were recorded throughout. Ambient conditions during the trial were 23.9 ± 0.9 °C; 49.9 ± 4.4% rh.
Owing to the effect of temperature and duration on HSP70 and IL-6 expression, we attempted to match the change in core temperature between conditions by eliciting a 1°C increase in core temperature. Furthermore, the duration of both EX and PH was matched to limit the effect of intervention exposure time on key outcome measures.
Instrumentation
Core temperature (T
C) was measured using a rectal thermistor (Grant Instruments Ltd, Cambridge) inserted 10 cm beyond the anal sphincter. Wireless skin sensors (iButtons, DS1922, California, USA) were applied and secured by Medipore tape (3M, Berkshire, UK). Mean skin temperature ( was calculated according to the formula of Ramanathan.Citation43 Participants wore a heart rate monitor throughout (RS800, Kempele, Polar, Finland).
Muscle temperature
Muscle temperature (T m) was measured in the right vastus lateralis using a solid needle probe (MKA08050A275TS Ellab, Copenhagen, Denmark) immediately pre- and post-trial. Following standard sterile procedure, the needle probe was first inserted to an initial depth of 3 cm beyond the muscle fascia where the temperature was allowed to stabilize before the probe was withdrawn to 2 cm and then 1cm depths, with the temperature recorded at each depth.
Blood sampling
Venous blood samples were collected by venepuncture from an antecubital vein in the right arm into a 10 mL Vacutainer that had been pre-cooled and pre-treated with K3EDTA (BD Biosciences, San Diego, USA). Samples were obtained before and on completion of each heating protocol with a final sample taken 120 minutes later (). Samples were stored on ice until they were centrifuged at 3,500 rpm for 10 min at 4°C and the plasma stored at −80°C until subsequent analysis.
Enzyme-linked immunosorbent assays
Plasma HSP70 (ENZ-101, AMP'D® hs HSP70, Enzo Life Sciences, Exeter, UK) and IL-6 (HS600B, Quantikine® HS IL-6, R&D Systems, Abingdon, UK) concentrations were analyzed using enzyme-linked immunosorbent sandwich assays (ELISAs) according to the manufacturer's instructions. Plasma HSP70 and IL-6 concentrations were determined in relation to a four-parameter standard curve (version 6.0 GraphPad Software, La Jolla, CA, USA). All samples were analyzed in duplicate with a mean intra plate coefficient of variation (CV) of 4.5% for HSP70 and 5.8% for IL-6. The inter plate CV was 6.3% for HSP70 and 6.1% for IL-6.
Calculations
Energy expenditure during PH and EX was calculated via indirect calorimetry. Heat balance parameters were estimated via partitional calorimetry and are presented as the mean value for each condition. All parameters were calculated in W/m2 but presented as W/kg where appropriate. The rate of metabolic energy expenditure was estimated as
Mean body temperature () was estimated using the three-compartment model as follows:
Statistical analysis
The normality and distribution of data was assessed using the Shapiro–Wilk normality test. Where data failed to meet the criteria of normal distribution, the data were log transformed. Mean participant characteristics were compared using independent samples t-tests. T
c, , Tm
, HSP70, IL-6 were analyzed using repeated measures ANOVA. Where significance was obtained post hoc tests were completed using Sidak's test for multiple comparisons.
,
and energy expenditure were analyzed using one-way ANOVA with Sidak's test for multiple comparisons. Correlational analysis was done using Pearson's correlation coefficient. Linear regression was used to analyze the relative contributions of ΔT
c, ΔT
sk, ΔT
m and
to ΔHSP70 and ΔIL-6. The R2 value was employed to determine the variance explained by each predictor variable. Where reported, the adjusted R2 value is provided for multiple linear regression in order to account for the number of predictor variables in the model. All statistical analyses were performed using GraphPad Prism (version 6.0 GraphPad Software, La Jolla, CA, USA). All data are presented as mean ± SD unless otherwise stated. p values ≤ 0.05 were considered statistically significant. Effect sizes (ES) corrected for bias using Hedge's g were calculated as the ratio of the mean difference to the pooled standard deviation of the difference, with 95% confidence intervals (95% CI) for differences also presented. The magnitude of the ES was classed as trivial (< 0.2), small (0.2–0.6), moderate (0.6–1.2), large (1.2–2.0) and very large (≥ 2.0).Citation45
Results
. There were differences in
between PH (1.9 ± 0.2 W/kg) and EX (7.3 ± 0.5 W/kg, p < 0.001).
was successfully matched between groups for both PH (LEAN 2.0 ± 0.2 W/kg, OW = 1.8 ± 0.2 W/kg, p = 0.433, CI = −0.03 to 0.43, ES = 0.9) and EX (LEAN 7.3 ± 0.4, OW 7.2 ± 0.5 W/kg; p = 0.763, CI = −0.63 to 0.43, ES = 0.21). However, when lean body mass is accounted for, differences in
were evident (LEAN 8.4 ± 0.4 W/kg/LBM−1; OW 9.8 ± 1.6 W/kg/LBM−1, p < 0.05, CI = 0.04 to 2.76, ES = 1.1).
eHSP70. There was a main effect of time (p < 0.005) but not condition (p = 0.887) on the eHSP70 response (). The increase in eHSP70 following PH and EX was 23% and 24%, respectively (). Across conditions ΔeHSP70 correlated with mean T m (r = 0.485, p < 0.05). ΔeHSP70 was negatively correlated to body mass (r = −0.400, p < 0.05). Of the thermal variables, ΔT m provided the best predictor of ΔHSP70, with the ΔT m explaining 24.7% of the total variance in ΔHSP70 (r = 0.498, p < 0.01). Body mass also predicted ΔHSP70, explaining 16.4% of the total variance (r = −0.405, p < 0.05). When combined, ΔT m and body mass provided the strongest predictor, accounting for 28% of the variance in ΔHSP70 (Adjusted R2 = 0.280, r = 0.585, p < 0.01).
Figure 2. The effect of 60-minutes passive heating at 40°C, exercise at 7 W/kg and body composition on IL-6 and HSP70. (A) The effect of passive heat and exercise on HSP70; (B) the change in IL-6 in response to heating; (C) the effect of passive heat and exercise on IL-6; (D) the change in IL-6 in response to heating. * above the line denotes main effect of time. † denotes difference between passive heating and exercise. Data presented as mean ± SEM.
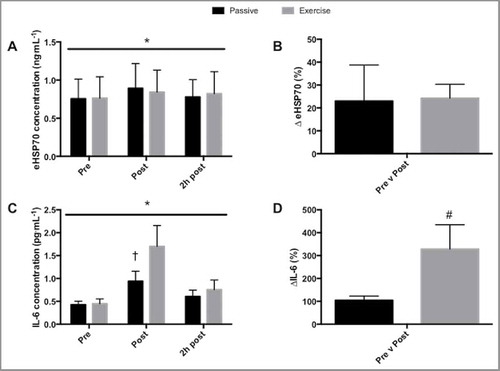
Figure 3. (A) Passive heating resulted in a reduction in peak interstitial glucose concentration compared to exercise in the meal immediately following the intervention. (B) There was no effect of either intervention on the total glucose area under the curve (AUC) in the meal following either passive heating or exercise. * denotes passive different to exercise (p < 0.05). Data presented as mean ± SEM. n = 8.
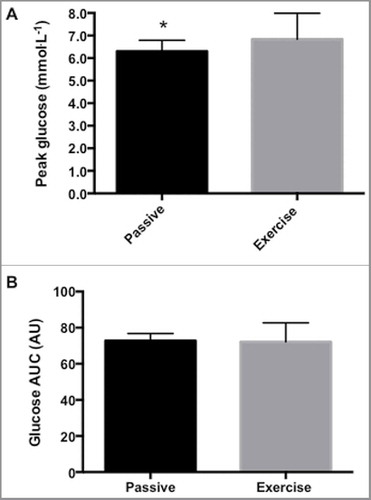
IL-6. There was a main effect of time (P < 0.001), condition (P < 0.05) and an interaction effect (P < 0.01, ) on IL-6 concentration. IL-6 was higher immediately following EX (1.74 ± 1.79 pg·mL−1) compared to PH (0.97 ± 0.86 pg·mL−1, p < 0.0001, ES = 0.5) and equated to an increase of 346% after EX and 118% following PH () from baseline values. IL-6 had returned to baseline at 2-hours post for PH (0.58 ± 0.47 pg·mL−1) and EX (0.73 ± 0.79 pg·mL−1). When considering differences between LEAN and OW, there was a main effect of time (p < 0.05) but not group (p = 0.533) on the IL-6 response. None of the thermal variables, nor body mass, provided an effective model for predicting the change in IL-6.
Energy expenditure
There were no differences in resting metabolic rate between LEAN (1811.2 ± 82.3 kcal·day−1) and OW (1873.9 ± 140.7 kcal·day−1; p = 0.330, ES = 0.5). There was an overall effect of condition on energy expenditure during heating (p < 0.0001) and an interaction effect (p < 0.05). PH increased energy expenditure by 61.0 ± 14.4 kcal·h−1 compared to rest (p < 0.0001, ES = 4.8), which equates to a 79.5% increase. EX resulted in an additional energy expenditure of 556.3 ± 92.0 kcal·h−1 compared to rest (p < 0.0001, ES = 7.1), equating to a 721% increase. EX energy expenditure was greater for OW compared to LEAN (11.3 ± 1.9 kcal·min−1 versus 9.8 ± 0.9 kcal·min−1, respectively, p < 0.05, ES = 1.0), resulting in an additional caloric expenditure from rest of 597.7 ± 107.9 kcal for OW (748%), and 515 ± 52.1 kcal for LEAN (681%). During PH, there was no difference in the increase in energy expenditure between LEAN and OW (2.3 ± 0.3 kcal·min−1 versus 2.3 ± 0.3 kcal·min−1, respectively, p = 0.997, ES = 0.0). Caloric expenditure increased from rest by 63.4 ± 16.6 kcal (84%) and 58.5 ± 12.7 kcal (74%) kcal for LEAN and OW, respectively although there was no difference between groups (p = 0.623, ES = 0.3).
Substrate oxidation
Total EX carbohydrate oxidation was higher for OW (125.3 ± 20.8 g) compared to LEAN (92.5 ± 28.2 g; p < 0.05, ES = 1.3). There was a moderate effect of body mass on total carbohydrate oxidation in PH between OW (7.5 ± 4.2 g) and LEAN (13.2 ± 8.3 g, ES = 0.83) although this did not reach significance (p = 0.13). There was no difference between OW and LEAN in total fat oxidation during EX (p = 0.46). There was a tendency for OW to display a greater total fat oxidation than LEAN in PH, although this did not reach statistical significance (10.6 ± 1.54 g versus 9.0 ± 3.1 g; ES 0.7; p = 0.13).
Continuous glucose monitoring
Owing to instances of poor compliance to diet replication and sensor malfunction and drop out, CGM analysis was only completed on eight participants, with an unequal split between LEAN (n = 3) and OW (n = 5). Therefore, it was only possible to conduct analysis on PH compared to EX. Peak glucose concentration in response to the meal following PH (6.3 ± 1.4 mmol·L−1) was lower compared to the same meal after EX (6.8 ± 1.2 mmol·L−1; p < 0.05, CI = −0.9 to 1.9, ES = 0.4, ). There was no difference in glucose AUC for PH compared to EX following this meal (p = 0.875, CI = −11.2 to 12.4, ES = 0.1 ). There were no differences in either peak glucose (meal 2 p = 0.168, meal 3 p = 0.266) or AUC (meal 2 p = 0.070, meal 3 p = 0.183) consumed in the subsequent 24-hour period. There were also no differences in total 24-hour AUC between PH and EX (p = 0.168)
Figure 4. The effect of 60-minutes passive heating at 40°C, exercise at 7 W/kg and body composition on determinants of mean body temperature. (A) the change in core temperature, (B) change in mean skin temperature, (C) muscle temperature at depths of 1 cm, 2 cm and 3 cm, (D) change in mean body temperature. * above line denotes main effect of time, * denotes difference between lean (LEAN) and overweight (OW) groups in the passive heating condition (p < 0.05). † denotes difference between LEAN and OW groups in the exercise condition (p < 0.05). ** denotes difference between passive heating (PH) and exercise (EX) within each group. Data presented as mean ± SEM.
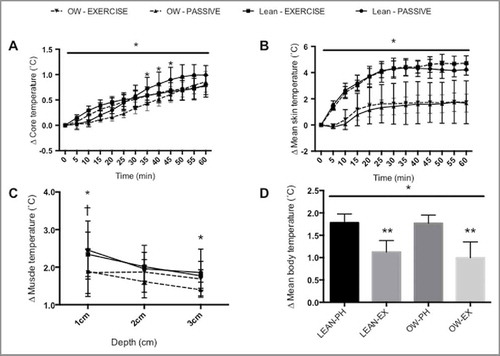
Core temperature
There was a main effect of time (p < 0.0001) and an interaction effect (P < 0.0001), but no main effect of condition on ΔT c (p = 0.112, ). PH ΔTc was lower in OW compared to LEAN from 35 minutes (p < 0.05), although by 60 minutes there was no difference in ΔT c (1.0 ± 0.2°C versus 0.9 ± 0.2°C, LEAN versus OW, respectively p = 0.089, CI = −0.13 to 0.33, ES = 0.5). There were no differences in ΔT c between OW and LEAN during EX at any time point, with ΔT c at 60 minutes being 0.8 ± 0.1°C versus 0.8 ± 0.2°C for LEAN and OW, respectively (p = 0.999).
Skin temperature
There were main effects of time (p < 0.0001), condition (p < 0.0001) and an interaction effect (p < 0.0001) on (). There were no differences in
between OW and LEAN following EX (LEAN 1.7 ± 0.7°C versus OW 1.7 ± 1.6°C, p = 0.999, ES = 0.0) or PH (LEAN 4.2 ± 0.4°C versus OW 4.7 ± 0.6°C, p = 0.095, ES = 0.9).
Muscle temperature
There was an effect of depth on ΔTm (p < 0.001), but no effect of condition (p = 0.285) or interaction (p = 0.111, ). Following PH, the ΔT m was greater for LEAN compared to OW at a depth of 1cm (LEAN, 2.5 ± 0.8°C; OW 1.9 ± 0.6°C, p < 0.0005, CI = −0.2 to 1.4, ES = 0.8) and 3cm (LEAN, 2.3 ± 0.6°C; OW 1.9 ± 0.7, p < 0.005, CI = −0.4 to 1.2, ES = 0.6). After EX, ΔT m was only different at 1cm (LEAN, 2.3 ± 0.6°C; OW 2.0 ± 0.7, p < 0.005, CI = −0.5 to 1.1, ES = 0.4).
Mean body temperature
There was a difference in between conditions (p < 0.0001, ). There were differences between LEAN PH and LEAN EX (1.7 ± 0.3°C versus 1.1 ± 0.3°C, p < 0.005, CI = 0.3 to 1.0, ES = 1.9); OW PH and OW EX (1.8 ± 0.2°C versus 1.0 ± 0.4°C; p < 0.001, CI = 0.4 to 1.2, ES = 2.4). There were no differences between LEAN and OW within the same condition (both p > 0.9).
Heart rate
There were significant effects of time, condition and interaction effect of heart rate (all p < 0.0001). Mean percentage HRmax EX was 78 ± 8% compared to 54 ± 7% in PH (p < 0.0001). There were no differences in the mean percentage of HRmax between groups for either PH (LEAN = 54.0 ± 6.0% versus OW = 55.2 ± 6.2%, p = 0.719) or EX (LEAN = 78.5 ± 7.3%; OW = 81.7 ± 7.4%, p = 0.428).
Discussion
The primary outcome of the present study was that the concentration of eHSP70 in the plasma was similar between EX and PH when both conditions were matched for ΔT c, with ΔT m being the best individual predictor of ΔeHSP70, explaining ∼25% of the total variance. This suggests that changes to T m may in part determine the appearance of eHSP70. An important secondary outcome is the increase in energy expenditure evident as a consequence of PH. This has important implications for the use of PH as a future intervention that may help to moderate body mass, particularly for individuals unable or unwilling to complete regular physical activity. However, we recognize that PH should be coupled with dietary manipulation to maximize any potential benefit to body composition. Finally, the reduction in peak glucose in the meal following PH compared to EX suggests that PH may have a beneficial effect on glycemic regulation and provide an alternative or augmentative intervention by which individuals with metabolic diseases may be able to improve their glycemic control. However, additional research needs to be conducted to confirm these data in larger cohorts over a prolonged period of time.
The HSP70 response may be hindered by excess body mass. Regression analysis demonstrated a link between body mass and the ΔeHSP70. A greater body mass was associated with an impaired eHSP70 response to heating, via either EX or PH, explaining 16% of the total variance. Such variation may be indicative of an impaired HSP response in overweight individuals in the face of physiological stress and would likely be compounded by low aerobic capacity.Citation46 The implications for such a deficiency are wide ranging and may increase susceptibility to metabolic disease,Citation46,Citation47 impaired vascular functionCitation48 and even contribute to the impaired thermoregulatory control evident in individuals with diabetes.Citation49
While there were no clear differences in the HSP70 response between either PH or EX, there were clear differences in the IL-6 response. Previous work has shown that temperature increases independently elevate IL-6.Citation50 However, the present data do not support the view that skeletal muscle may act as a “heat stress sensor” triggering a subsequent cytokine response, as none of the thermal measures taken during the trials were effective predictors of ΔIL-6 and could not account for a significant proportion of the variation in IL-6 following heating. Our data also indicate that the difference in IL-6 between PH and EX is not due to HSP70 induction of IL-6,Citation35,Citation36 as ΔHSP70 was similar following PH and EX. This suggests the IL-6 response to EX occurs independently of HSP70 expression and is more dependent on intensity and duration of EX,Citation51 than exposure to heat stress. IL-6 combined with its receptor (IL-6R), may also have a positive effect on insulin independent glucose uptake at rest.Citation52 Should chronic PH enhance IL-6R expression as is evident following EX training,Citation53 interventions capable of increasing both IL-6 and IL-6R, may provide a therapeutic basis for improving glycemic control. Moreover, it has recently been shown that acute PH elevates the anti-inflammatory cytokines IL-1ra and IL-10,Citation54 suggesting that chronic PH may benefit the inflammatory profile in a similar way to EX. However, long-term investigation of PH is required to corroborate this hypothesis.
Although the sample size for analysis of the glycemic response to PH and EX was somewhat limited, the fact that comparable glycemic profiles were evident between PH and EX is interesting. Given the acute effect of EX on improving glucose control as a consequence of improved insulin sensitivity,Citation55 this is a somewhat surprising result. However, it has recently been shown that heat stress activates insulin independent glucose transport via 5′ adenosine monophosphate-activated protein kinase (AMPK) activation.Citation56 In response to the heat stress stimulus, a reduction in muscle adenosine triphosphate, phosphocreatine and glycogen was reported, indicating a significant energy cost to the heating protocol and hence activation of AMPK. This suggests that, acutely at least, heat stress may improve glucose uptake due to increased energy expenditure. Further work needs to be conducted to establish the exact mechanisms by which PH may exert such an effect.
In addition to benefits to metabolic regulation, PH has been shown to benefit cardiovascular health. In a recent meta-analysis, Laukkanen et al. report that lifelong sauna use reduced cardiovascular and all cause mortality, with the largest benefits associated with more frequent sauna use.Citation57 Although this relationship does not establish causality,Citation31,Citation58 it is nonetheless an interesting link that is supported by recent investigations.Citation59-Citation61 PH appears to improve vascular function, with improvements to flow-mediated dilatation, arterial stiffness and blood pressure reported after 8 weeks of PH.Citation59 Moreover, Thomas et al. have shown that 30 minutes of PH can induce a significant shear stress response and a reduction in mean arterial pressure in patients with peripheral arterial disease.Citation62 Furthermore, in a healthy cohort who underwent matched duration PH or EX, the PH group demonstrated a greater shear stress response compared to that in response to EX.Citation60 Based on heart rate responses, the cardiovascular strain in the present study was comparable to that of Thomas et al., which elicited ∼50% HRmax during their immersion protocol.Citation60 These recent findings indicate that PH has the potential to induce significant systemic benefits, which in some instances may be comparable to or even greater than the effects evident following EX.
There are a few limitations with the present investigation. First, the omission of a measure of iHSP70, either derived from skeletal muscle or leukocytes makes it difficult to draw firm conclusions on the potential intracellular effect of PH and glycemic regulation. Without direct measures of iHSP70, in addition to JNK and other molecules in the insulin-signaling cascade, it is difficult to derive the exact effect of PH on acute glycemic control. Therefore, we are limited to discuss the relationship between eHSP70 and iHSP70 and that an increase in the extracellular component is reflective of changes in the intracellular compartment. Future investigation should employ techniques to measure both iHSP70 and eHSP70 to enable greater understanding of the effect of PH on the intracellular to extracellular ratio. Second, the relatively small sample size of this study, which may limit the statistical power of our experiments. We acknowledge that further experiments with larger cohorts should be conducted in order to replicate the present data.
In summary, the data presented here demonstrate that both acute PH and EX result in comparable increases in HSP70. It is suggested that ΔHSP70 is sensitive to the ΔT m. The elevations in both HSP70 and IL-6 may promote an anti-inflammatory milieu and help combat the chronic inflammation associated with many disease states. The increase in energy expenditure following PH demonstrates a systemic effect of PH, which has the ability to reduce adipose tissue and further contribute to reducing inflammation. However, it should be noted that EX still offers the greatest overall benefit to weight control and metabolic health. Finally, in agreement with that of other authors, the present data indicate that PH might provide an alternative to EX in some individuals who are too physically impaired to undertake prolonged aerobic activity to improve their cardio-metabolic health. The possible therapeutic benefits of PH require more extensive investigation.
Abbreviations
AMPK | = | 5′ adenosine monophosphate-activated protein kinase |
AUC | = | Area under the curve |
BMI | = | Body mass index |
BP | = | blood pressure |
BSA | = | body surface area |
CGM | = | Continuous glucose monitor |
CI | = | confidence interval |
CV | = | coefficient of variation |
eHSP | = | extracellular heat shock protein |
ELISA | = | enzyme-linked immunosorbent sandwich assay |
ES | = | effect size |
EX | = | Exercise trial |
HDL-C | = | High-density lipoprotein cholesterol |
= | Metabolic heat production | |
HSP | = | Heat shock protein |
iHSP | = | Intracellular heat shock protein |
IL-1ra | = | Interleukin 1 receptor antagonist |
IL-6 | = | Interleukin 6 |
IL-10 | = | Interleukin 10 |
JNK | = | c-Jun N-terminal kinase |
LDL-C | = | Low density lipoprotein cholesterol |
LEAN | = | Lean participant group |
OW | = | Overweight participant group |
PH | = | Passive heating trial |
SD | = | Standard deviation |
SEM | = | Standard error of mean |
T2DM | = | Type 2 diabetes mellitus |
Tb | = | body temperature |
Tc | = | core temperature |
Tm | = | muscle temperature |
Tsk | = | skin temperature |
TG | = | Triglyceride |
= | carbon dioxide production | |
= | oxygen uptake | |
= | Maximum volume of oxygen uptake | |
ΔT | = | change in temperature |
Disclosure of potential conflicts of interest
No potential conflicts of interest were disclosed.
Acknowledgments
The authors would like to thank; Prof George Havenith for granting access to the Environmental Ergonomics Research Centre and Dr Caroline Smith for her insightful discussion and comments during the preparation of the manuscript.
Funding
The research was also partly supported by the National Institute for Health Research (NIHR) Diet, Lifestyle & Physical Activity Biomedical Research Unit based at University Hospitals of Leicester and Loughborough University. The views expressed are those of the authors and not necessarily those of the NHS, the NIHR or the Department of Health.
References
- Martin LR, Williams SL, Haskard KB, DiMatteo MR. The challenge of patient adherence. Ther Clin Risk Man. 2005;1(3):189-199.
- Praet SFE, van Rooij E.S.J., Wijtvliet A, Boonman-de Winter LJM, Enneking Th, Kuipers H, Stehouwer CDA, van Loon LJC. Brisk walking compared with an individualised medical fitness programme for patients with type 2 diabetes: a randomised controlled trial. Diabetologia. 2008;51(5):736-746. PMID:18297259; doi:10.1007/s00125-008-0950-y.
- Jill AS. A comparison of views of individuals with type 2 diabetes mellitus and diabetes educators about barriers to diet and exercise. J Health Commun. 2001;6(2):99-115. PMID:11405082; doi:10.1080/10810730116985.
- Febbraio MA, Mesa JL, Chung J, Steensberg A, Keller C, Nielsen HB, Krustrup P, Ott P, Secher NH, Pedersen BK. Glucose ingestion attenuates the exercise-induced increase in circulating heat shock protein 72 and heat shock protein 60 in humans. Cell Stress Chaperones. 2004;9(4):390-396. PMID:15633297; doi:10.1379/CSC-24R1.1.
- McCarty MF. Induction of heat shock proteins may combat insulin resistance. Med Hypotheses. 2006;66(3):527-534. PMID:16309849; doi:10.1016/j.mehy.2004.08.033.
- McCarty MF, Barroso-Aranda J, Contreras F. Regular thermal therapy may promote insulin sensitivity while boosting expression of endothelial nitric oxide synthase – effects comparable to those of exercise training. Med Hypotheses. 2009; 73(1):103-105. PMID:19203842; doi:10.1016/j.mehy.2008.12.020.
- Krause M, Ludwig MS, Heck TG, Takahashi HK. Heat shock proteins and heat therapy for type 2 diabetes: pros and cons. Curr Opin Clin Nutr Metab Care. 2015;18(4):374-380. PMID:26049635; doi:10.1097/MCO.0000000000000183.
- Krause M, Heck TG, Bittencourt A, Scomazzon SP, Newsholme P, Curi R, de Bittencourt PIH. The chaperone balance hypothesis: the importance of the extracellular to intracellular HSP70 ratio to inflammation-driven type 2 diabetes, the effect of exercise, and the implications for clinical management. Mediators Inflamm. 2015;2015:249205. doi:10.1155/2015/249205.
- Rodrigues-Krause J, Krause M, O'Hagan C, De Vito G, Boreham C, Murphy C, Newsholme P, Colleran G. Divergence of intracellular and extracellular HSP72 in type 2 diabetes: does fat matter? Cell Stress Chaperones. 2012;17(3):293-302. PMID:22215518; doi:10.1007/s12192-011-0319-x.
- Molina MN, Ferder L, Manucha W. Emerging role of nitric oxide and heat shock proteins in insulin resistance. Curr Hypertens Rep. 2016;18(1):1-015-0615-4. doi:10.1007/s11906-015-0615-4.
- Ortega E, Bote ME, Besedovsky HO, Rey Ad. Hsp72, inflammation, and aging: causes, consequences, and perspectives. Ann N Y Acad Sci. 2012;1261(1):64-71. PMID:22823395; doi:10.1111/j.1749-6632.2012.06619.x.
- Yamada P, Amorim F, Moseley P, Schneider S. Heat shock protein 72 response to exercise in humans. Sports Med. 2008;38(9):715-733. PMID:18712940; doi:10.2165/00007256-200838090-00002.
- Walsh RC, Koukoulas I, Garnham A, Moseley PL, Hargreaves M, Febbraio MA. Exercise increases serum Hsp72 in humans. Cell Stress Chaperones. 2001;6(4):386-393. PMID:11795476; doi:10.1379/1466-1268(2001)006%3c0386:EISHIH%3e2.0.CO;2.
- Riezman H. Why do cells require heat shock proteins to survive heat stress? Cell Cycle. 2004;3(1):60-62. doi:10.4161/cc.3.1.625
- Hooper PL, Hooper PL. Inflammation, heat shock proteins, and type 2 diabetes. Cell Stress Chaperones. 2009; 14(2):113-115. PMID:18720028; doi:10.1007/s12192-008-0073-x.
- Atalay M, Oksala N, Lappalainen J, Laaksonen DE, Sen CK, Roy S. Heat shock proteins in diabetes and wound healing. Curr Protein Pept Sci. 2009;10(1):85-95. PMID:19275675; doi:10.2174/138920309787315202.
- Gupte AA, Bomhoff GL, Touchberry CD, Geiger PC. Acute heat treatment improves insulin-stimulated glucose uptake in aged skeletal muscle. J Appl Physiol. 2011; 110(2):451-457. PMID:21148343; doi:10.1152/japplphysiol.00849.2010.
- Chung J, Nguyen A, Henstridge DC, Holmes AG, Stanley Chan MH, Mesa JL, Lancaster GI, Southgate RJ, Bruce CR, Duffy SJ, et al. HSP72 protects against obesity-induced insulin resistance. PNAS. 2008;105(5):1739-1744. PMID:18223156; doi:10.1073/pnas.0705799105.
- Bruce CR, Carey AL, Hawley JA, Febbraio MA. Intramuscular heat shock protein 72 and heme oxygenase-1 mRNA are reduced in patients with type 2 diabetes: evidence that insulin resistance is associated with a disturbed antioxidant defense mechanism. Diabetes. 2003; 52(9):2338-2345. PMID:12941774; doi:10.2337/diabetes.52.9.2338.
- Kurucz I, Morva A, Vaag A, Eriksson KF, Huang X, Groop L, Koranyi L. Decreased expression of heat shock protein 72 in skeletal muscle of patients with type 2 diabetes correlates with insulin resistance. Diabetes. 2002; 51(4):1102-1109. doi:10.2337/diabetes.51.4.1102
- Periard JD, Ruell P, Caillaud C, Thompson MW. Plasma Hsp72 (HSPA1A) and Hsp27 (HSPB1) expression under heat stress: influence of exercise intensity. Cell Stress Chaperones. 2012;17(3):375-383. PMID:22222935; doi:10.1007/s12192-011-0313-3.
- Fehrenbach E, Niess AM, Veith R, Dickhuth HH, Northoff H. Changes of HSP72-expression in leukocytes are associated with adaptation to exercise under conditions of high environmental temperature. J Leukoc Biol. 2001; 69(5):747-754. PMID:11358983.
- Gibson O, Dennis A, Parfitt T, Taylor L, Watt P, Maxwell N. Extracellular Hsp72 concentration relates to a minimum endogenous criteria during acute exercise-heat exposure. Cell Stress and Chaperones. 2014;19(3):389-400. PMID:24085588; doi:10.1007/s12192-013-0468-1.
- Iguchi M, Littmann AE, Chang SH, Wester LA, Knipper JS, Shields RK. Heat stress and cardiovascular, hormonal, and heat shock proteins in humans. J Athl Train. 2012; 47(2):184-190. PMID:22488284.
- Bathaie SZ, Jafarnejad A, Hosseinkhani S, Nakhjavani M. The effect of hot-tub therapy on serum Hsp70 level and its benefit on diabetic rats: a preliminary report. Int J Hyperther. 2010;26(6):577-585. doi:10.3109/02656736.2010.485594.
- Karpe PA, Tikoo K. Heat shock prevents insulin Resistance–Induced vascular complications by augmenting angiotensin-(1–7) signaling. Diabetes. 2014;63(3):1124-1139. doi:10.2337/db13-1267.
- Gupte AA, Bomhoff GL, Geiger PC. Age-related differences in skeletal muscle insulin signaling: the role of stress kinases and heat shock proteins. J Appl Physiol (1985). 2008;105(3):839-848. doi:10.1152/japplphysiol.00148.2008.
- Raz I, Weiss R, Yegorchikov Y, Bitton G, Nagar R, Pesach B. Effect of a local heating device on insulin and glucose pharmacokinetic profiles in an open-label, randomized, two-period, one-way crossover study in patients with type 1 diabetes using continuous subcutaneous insulin infusion. Clin Ther. 2009;31(5):980-987. PMID:19539098; doi:10.1016/j.clinthera.2009.05.010.
- Hermanns N, Bitton G, Reimer A, Krichbaum M, Kulzer B, Haak T. Effect of local heating on postprandial blood glucose excursions using the InsuPad device: results of an outpatient crossover study J Diabetes Sci Technol. 2014; 8(6):1126-1132. PMID:25113814; doi:10.1177/1932296814546162.
- Hooper PL. Hot-tub therapy for type 2 diabetes mellitus. N Engl J Med. 1999;341(12):924-925. PMID:10498473; doi:10.1056/NEJM199909163411216.
- Laukkanen T, Khan H, Zaccardi F. The link between sauna bathing and mortality may be noncausal: a reply. JAMA Internal Medicine. 2015;175(10):1719-1719. PMID:26436740; doi:10.1001/jamainternmed.2015.3432.
- Geiger PC, Gupte AA. Heat shock proteins are important mediators of skeletal muscle insulin sensitivity. Exerc Sport Sci Rev. 2011;39(1):34-42. PMID:21088604; doi:10.1097/JES.0b013e318201f236.
- Gleeson M, Bishop NC, Stensel DJ, Lindley MR, Mastana SS, Nimmo MA. The anti-inflammatory effects of exercise: mechanisms and implications for the prevention and treatment of disease. Nat Rev Immunol. 2011;11(9):607-615.PMID:21818123; doi:10.1038/nri3041.
- Steensberg A, Fischer CP, Keller C, Moller K, Pedersen BK. IL-6 enhances plasma IL-1ra, IL-10, and cortisol in humans. Am J Physiol Endocrinol Metab. 2003;285(2):E433-7. PMID:12857678; doi:10.1152/ajpendo.00074.2003.
- Wallin RP, Lundqvist A, More SH, von Bonin A, Kiessling R, Ljunggren HG. Heat-shock proteins as activators of the innate immune system. Trends Immunol. 2002;23(3):130-135. PMID:11864840; doi:10.1016/S1471-4906(01)02168-8.
- Tsan M, Gao B. Cytokine function of heat shock proteins. Am J Physiol Cell Physiol. 2004;286(4):C739-C744. doi:10.1152/ajpcell.00364.2003.
- Kadoglou NP, Iliadis F, Angelopoulou N, Sailer N, Fotiadis G, Voliotis K, Vitta I, Liapis CD, Alevizos M. Cardiorespiratory capacity is associated with favourable cardiovascular risk profile in patients with type 2 diabetes. J Diabetes Complications. 2009;23(3):160-166. PMID:18413173; doi:10.1016/j.jdiacomp.2007.12.008.
- Hooper P, Balogh G, Rivas E, Kavanagh K, Vigh L. The importance of the cellular stress response in the pathogenesis and treatment of type 2 diabetes. Cell Stress and Chaperones. 2014;19(4):447-464. PMID:24523032; doi:10.1007/s12192-014-0493-8.
- Nielsen B, Hales JR, Strange S, Christensen NJ, Warberg J, Saltin B. Human circulatory and thermoregulatory adaptations with heat acclimation and exercise in a hot, dry environment. J Physiol. 1993;460:467-485. doi:10.1113/jphysiol.1993.sp019482.
- Borghouts LB, Backx K, Mensink MF, Keizer HA. Effect of training intensity on insulin sensitivity as evaluated by insulin tolerance test. Eur J Appl Physiol Occup Physiol. 1999;80(5):461-466. PMID:10502080; doi:10.1007/s004210050618.
- Jackson AS, Pollock ML. Generalised equations for predicting body density of men. Br J Nutr 1978;40:497-504. PMID:718832; doi:10.1079/BJN19780152.
- Clarke W, Kovatchev B,. Statistical tools to analyze continuous glucose onitor data. Diabetes Technol Ther. 2009;11(Supp 1):S45–S54.
- Ramanathan NL. A new weighting system for mean surface temperature of the human body. J Appl Physiol. 1964;19:531-533. PMID:14173555.
- Jay O, Gariepy LM, Reardon FD, Webb P, Ducharme MB, Ramsay T, Kenny GP. A three-compartment thermometry model for the improved estimation of changes in body heat content. Am J Physiol Regul Integr Comp Physiol. 2007;292(1):R167-175. PMID:16931653; doi:10.1152/ajpregu.00338.2006.
- Batterham AM, Hopkins WG. Making meaningful inferences about magnitudes. Int J Sports Physiol Perform. 2006;1(1):50-57. PMID:19114737; doi:10.1123/ijspp.1.1.50.
- Rogers RS, Morris EM, Wheatley JL, Archer AE, McCoins CS, White KS, Wilson DR, Meers GM, Koch LG, Britton SL, et al. Deficiency in the heat stress response could underlie susceptibility to metabolic disease. Diabetes. 2016. doi:10.2337/db16-0292.
- Kondo T, Motoshima H, Igata M, Kawashima J, Matsumura T, Kai H, Araki E. The role of heat shock response in insulin resistance and diabetes. Diabetes Metab J. 2014; 38(2):100-106. PMID:24851203; doi:10.4093/dmj.2014.38.2.100.
- Malyshev IY, Bayda LA, Trifonov AI, Larionov NP, Kubrina LD, Mikoyan VD, Vanin AF, Manukhina EB. Cross-talk between nitric oxide and HSP70 in the antihypotensive effect of adaptation to heat. Physiol Res. 2000; 49(1):99-105. PMID:10805410.
- Kenny GP, Sigal RJ, McGinn R. Body temperature regulation in diabetes. Temperature. 2016;3(1):119-145. doi:10.1080/23328940.2016.1230171.
- Welc SS, Phillips NA, Oca-Cossio J, Wallet SM, Chen DL, Clanton TL. Hyperthermia increases interleukin-6 in mouse skeletal muscle. Am J Physiol Cell Physiol. 2012; 303(4):C455-C466. PMID:22673618; doi:10.1152/ajpcell.00028.2012.
- Helge J, Stallknecht B, Pedersen BK, Galbo H, Kiens B, Richter EA. The effect of graded exercise on IL-6 release and glucose uptake in human skeletal muscle. J Physiol (Lond). 2002;546:299-305. doi:10.1113/jphysiol.2002.030437.
- Saini A, Faulkner SH, Moir H, Warwick P, King J, Nimmo MA. Interleukin-6 in combination with the interleukin-6 receptor stimulates glucose uptake in resting human skeletal muscle independently of insulin action. Diabetes, Obes Metab. 2014;16(10):931-936. doi:10.1111/dom.12299.
- Keller C, Steensberg A, Hansen AK, Fischer CP, Plomgaard P, Pedersen BK. Effect of exercise, training, and glycogen availability on IL-6 receptor expression in human skeletal muscle. J Appl Physiol. 2005;99(6):2075-2079. PMID:16099893; doi:10.1152/japplphysiol.00590.2005.
- Leicht CA, Papanagopoulos A, Haghighat S, Faulkner SH. Increasing heat storage by wearing extra clothing during upper body exercise up-regulates heat shock protein 70 but does not modify the cytokine response. J Sports Sci. 2016;1-7; doi:10.1080/02640414.2016.1235795.
- Goodyear LJ, Kahn BB. Exercise, glucose transport and insulin sensitivity. Annu Rev Med. 1998;49(1):235-261. PMID:9509261; doi:10.1146/annurev.med.49.1.235.
- Goto A, Egawa T, Sakon I, Oshima R, Ito K, Serizawa Y, Sekine K, Tsuda S, Goto K, Hayashi T. Heat stress acutely activates insulin‐independent glucose transport and 5′‐AMP‐activated protein kinase prior to an increase in HSP72 protein in rat skeletal muscle. Physiol Rep. 2015;3(11). doi:10.14814/phy2.12601.
- Laukkanen T, Khan H, Zaccardi F, Laukkanen JA. Association between sauna bathing and fatal cardiovascular and all-cause mortality events. JAMA Intern Med. 2015; 175(4):542-548. PMID:25705824; doi:10.1001/jamainternmed.2014.8187.
- Kivimaki M, Virtanen M, Ferrie JE. The link between sauna bathing and mortality may be noncausal. JAMA Internal Medicine. 2015;175(10):1718-1718. PMID:26436738; doi:10.1001/jamainternmed.2015.3426.
- Brunt VE, Howard MJ, Francisco MA, Ely BR, Minson CT. Passive heat therapy improves endothelial function, arterial stiffness and blood pressure in sedentary humans. J Physiol (Lond ). 2016;594(18):5329-5342. PMID:27270841; doi:10.1113/JP272453.
- Thomas KN, van Rij AM, Lucas SJE, Gray AR, Cotter JD. Substantive hemodynamic and thermal strain upon completing lower-limb hot-water immersion; comparisons with treadmill running. Temperature. 2016;3(2):286-297. doi:10.1080/23328940.2016.1156215.
- Romero SA, Gagnon D, Adams AN, Cramer MN, Kouda K, Crandall CG. Acute limb heating improves macro- and microvascular dilator function in the leg of aged humans. Am J Physiol Heart Circ Physiol. 2017;312(1):H89-H97. PMID:27836894; doi:10.1152/ajpheart.00519.2016.
- Thomas KN, van Rij AM, Lucas SJE, Cotter JD. Lower-limb hot-water immersion acutely induces beneficial hemodynamic and cardiovascular responses in peripheral arterial disease and healthy, elderly controls. Am J Physiol Regul Integr Comp Physiol. 2017;312(3):R281-R291. doi:10.1152/ajpregu.00404.2016.