ABSTRACT
Most professional and recreational athletes perform pre-conditioning exercises, often collectively termed a ‘warm-up’ to prepare for a competitive task. The main objective of warming-up is to induce both temperature and non-temperature related responses to optimize performance. These responses include increasing muscle temperature, initiating metabolic and circulatory adjustments, and preparing psychologically for the upcoming task. However, warming-up in hot and/or humid ambient conditions increases thermal and circulatory strain. As a result, this may precipitate neuromuscular and cardiovascular impairments limiting endurance capacity. Preparations for competing in the heat should include an acclimatization regimen. Athletes should also consider cooling interventions to curtail heat gain during the warm-up and minimize dehydration. Indeed, although it forms an important part of the pre-competition preparation in all environmental conditions, the rise in whole-body temperature should be limited in hot environments. This review provides recommendations on how to build an effective warm-up following a 3 stage RAMP model (Raise, Activate and Mobilize, Potentiate), including general and context specific exercises, along with dynamic flexibility work. In addition, this review provides suggestion to manipulate the warm-up to suit the demands of competition in hot environments, along with other strategies to avoid heating-up.
Introduction
Body temperature has always been considered an indicator of health status.Citation1 However, only during the 20th century has thermoregulation of the healthy and active human become a major area of research. This interest was mainly driven by the requirements of the mining industry and the military. Lavoisier (1743–1794) showed that humans generated heat by a combustion process resulting in the production of carbon dioxide. Claude Bernard (1813–1878) then showed that the blood entering the lungs was warmer than that exiting, and that venous blood was warmer than arterial blood in several other organs, suggesting that these tissues were the site of heat production. Muscle heat production and dissipation during exercise and their role in increasing core temperature (Tcore) was described in detail during the 1960s.Citation2 Since then, several researchers and practitioners have discussed the potential benefit for performance of increasing muscle temperature (Tm) through warming-up,Citation3,Citation4 or the potential impairment in performance and increased risk to athlete health of increasing Tcore.Citation5 Interestingly however, this dichotomy is generally considered independently.
From the minimal heat production associated with basal metabolic rate, heat production dramatically increases at the onset of a muscle contraction, doubling over the first minutes of intense dynamic exercise.Citation6 During the first 45 s, heat production mainly induces a large increase in Tm,Citation6 thereafter driving an increase in Tcore.Citation7 Although exact absolute values of Tm are dependent of the depth of measurement, muscle measured, environmental conditions, and intensity of contraction, Tm is ∼35°C at rest and will exceed Tcore within 3–5 min of exercise, remaining 0.65–0.95°C higher than Tcore.Citation7 As detailed in the first section of this manuscript, the initial increase in Tm has several benefits for athletic performance. Although the benefits of increasing Tm in-vivo might not be as important as originally estimated in-vitro, warming-up also offers non temperature-dependent effects.
Yet, depending on both exercise intensity and climatic conditions, the compensability of the environment will vary.Citation8 Maintaining thermal allostasis requires transferring metabolic heat from the core to the skin and then on to the environment. This involves an increase in cutaneous circulationCitation9 and sweating.Citation10 However, the increase in sweat rate necessary for heat dissipation can lead to progressive dehydration if fluid losses are not offset by adequate fluid consumption.Citation11-Citation13 Progressive dehydration precipitates a cascade of events including a decrease in plasma volume and an increase in plasma osmolality,Citation5 a decrease in sweat rate and evaporative heat lossCitation14 and a decrease in cardiac filling.Citation15 As detailed in the second section of this manuscript, the blood flow redistribution and other thermoregulatory demands of exercising in hot and/or humid environments represents a significant stress to the cardiovascular systemCitation16 limiting performance,Citation17 as maintaining a similar relative intensity requires the reduction of absolute intensity (i.e. work load).Citation18 Moreover, as detailed below, hyperthermia may also affect various levels of the neural system.
Lastly, the final sections of this manuscript will present practical sporting applications for (pre)competition in the heat. Despite a long history of research on thermoregulation, the incidence of heat stroke has increased over the past decades and heat is responsible for more deaths than all other natural disasters combined.Citation19 This manuscript will present the current state of the literature to inform creation of a warm-up specific to the requirements of competition. This will be achieved by extracting the principles of a 3-stage systematic approach (raise, activate and mobilise, potentiate; RAMP). Also, it will be discussed how to amend the athlete's warm-up to account for environmental conditions and reduce the risk of encountering heat illness. While the most important countermeasure to protect the health and performance of athletes in hot and/or humid environments is to heat acclimatize,Citation20 there are other countermeasures that can be implemented during the warm-up and throughout exercise that contribute to minimize the increase in Tcore, all the while maintaining optimal locomotor Tm and promoting non-thermal warm-up benefits.
The physiology of warming-up
An increase in body temperatures is not necessarily detrimental to the health and performance of an athlete. For example, most professional and recreational athletes perform a pre-conditioning exercise called a ‘warm-up’ to increase their Tm and prepare for a competitive task. The term warm-up is an appropriate representation of Asmussen & Bøje'sCitation21 seminal work investigating the effects of body temperature on performance. They found that both active (muscular work) and passive (hot water immersion or radio-diathermy) heating improved high-intensity performance, ranging from <15 s to approx. 4–5 min.Citation21 Most of the ergogenic aspects of warming-up seem temperature-dependent, with both increased Tm and Tcore potentially resulting in improved muscle force and power production,Citation22 improved muscle blood flow,Citation23,Citation24 augmented muscle glycogen and carbohydrate (CHO) utilization,Citation25 accelerated oxyhaemoglobin dissociation,Citation26 increased metabolic rate and enzymatic reactions,Citation27 and changes in both mechanical efficiency and muscle fiber conduction velocities.Citation27,Citation28 However, some authors have also highlighted the metabolic importance of warming-up and suggested that it should be called ‘acid-up’.Citation29 Others have highlighted the limited scientific evidence for such a standard pre-activity.Citation3 Notwithstanding, most if not all athletes perform a warm-up to enhance performance and reduce the risk of injury. The current section will present the physiological adjustments associated with warming-up, both temperature or non-temperature related. The practical recommendations to construct a warm up are presented in a later section of this review (see Application to sports – warming up for performance). These recommendations include various stages (raise, activate and mobilise, potentiate; RAMP) and account for the environmental conditions.
Muscle adjustments
As mentioned above, muscle contractions produce heat. This heat production will increase Tm within seconds, before any visible changes in Tcore. While muscle contractions produce heat, muscle contractility itself is also affected by temperature and thus warming-up.
Effect of increasing temperature through warming-up on muscle function
Muscle temperature effects contraction velocity.Citation30 While a decrease in Tm can slow-down chemical reactions,Citation31 delay the cross-bridge cycleCitation32 and decrease actomyosin sensibility to calciumCitation33; an increase in Tm increases the rate of force development of a muscle twitch,Citation34,Citation35 probably in relation to an increase in myosin adenosinetriphosphatase (ATPase) activityCitation36 and calcium sequestration by the sarcoplasmic reticulum.Citation37 In addition, maximum tetanic force can also be improved by increasing Tm,Citation34,Citation38,Citation39 possibly by improving contractile protein binding.Citation39 Of note, slow muscles (eg. soleus) seem to be more sensitive to temperature than fast muscles (e.g. extensor digitorum longus), especially at lower temperatures (i.e., 20°C to 10°C).Citation35,Citation40 However, despite the inverse relationship between Tm and time to peak twitch tension and half relaxation time in vitro,Citation38 the effect of temperature is less marked toward physiological temperatures in vivo.Citation41 Indeed, an increase in Tm across the standard range experienced in vivo (i.e., from 37 to 43°C) has not been observed to modify the absolute force of the muscle fiber.Citation42 As a consequence, an increase in Tm does not necessary modify peak twitch amplitude in human skeletal muscle ().Citation43-Citation45 Consequently, increasing Tm through warming-up might have less benefit for muscle contractility in vivo than suggested by in vitro studies, especially in hot environments.
Figure 1. Muscle twitch at resting basal (plain line, blue) and elevated (dashed line, red) muscle temperature. An increase in muscle temperature generally increases the rate of force development and relaxation without modifying the peak tension. Data extracted with permission from Racinais et al.Citation45
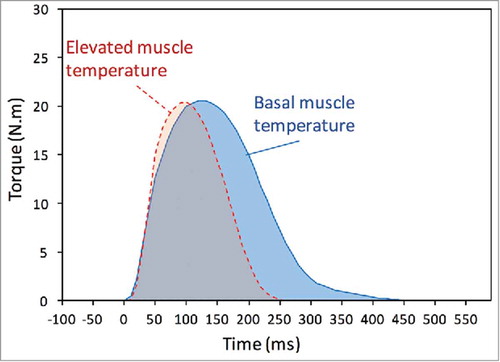
However, in situ muscle responses cannot be limited to the muscle fiber as they also involve non-contractile tissue (e.g., ligaments). Indeed, increasing temperature decreases the viscous resistance of muscle and joints.Citation46,Citation47 In addition, increasing temperature will affect the muscle environment by increasing local vasodilatationCitation48 and increasing both nerve and sarcolemmal action potentials.Citation49 Lastly, as detailed in the next paragraph, warming-up also has non-thermal benefits on the muscle.
Non-thermal effects of warming-up on skeletal muscle
Independently of the increase in Tm, a warm-up can potentially increase performance by ‘pre-conditioning’ the muscle. This phenomena called post-activation potentiation (PAP) is generally obtain by performing a maximal or near maximal contraction,Citation50,Citation51 and has been suggested to have additional benefits relative to a traditional warm-up (i.e., without such contraction) to improve performance in explosive activities.Citation52 The first purported mechanism explaining PAP is a phosphorylation of the myosin regulatory light chains.Citation50,Citation53 However, this phosphorylation is not consistently observed in humans.Citation54 The second mechanism proposed is an increase in spinal synaptic transmission that could last for several minutes following a contraction.Citation52 Synaptic transmission can be indirectly assessed using H-reflex, a monosynaptic spinal reflex representing an electrically evoked variant of the stretch reflex. While homosynaptic post-activation depression initially decreases the H-reflex during the first minute following a contraction, independently of Tm,Citation55 the H-reflex can thereafter be potentiated for up to 10 min following maximal contractions.Citation52,Citation56 However, the efficacy of PAP on real-world athletic performance may still remain inconclusive, when considering the trade-off between, or even coexistence of both fatigue and potentiation actions on the muscle.Citation51,Citation57 Indeed, neural drive following a maximal contraction is a balance between PAP and fatigue,Citation51 with the latter likely being the dominant factor.Citation58 On a separate note, muscles develop actin-myosin cross-bridges at rest to maintain muscle tone and posture.Citation59 These cross-bridges increase muscle rigidity, but muscle contractions during warm-up breakdown those cross-bridges,Citation60 potentially increasing the rate of force development and power. However, muscle rigidity re-increases rapidly once the warm-up is completed.Citation61
Neural drive adjustments
In addition to influencing muscle function, temperature and warming-up can affect the neural drive reaching the muscle.
Effect of increasing temperature through warming-up on neural drive transmission
It has consistently been demonstrated that cold exposure reduces nerveCitation62 and muscleCitation63 conduction velocity. In addition, cold exposure can increase antagonist muscle co-contractions, likely as a protective mechanism for cold muscles and joints.Citation64 Shivering can also affect agonist and antagonist (co-)activation.Citation65 A warm-up increasing body temperatures will therefore counteract these effects and increase performance in cold environments, especially during fast movements.Citation30
However, the benefits of increasing temperature on neural drive decrease as temperature increases, and are overlaid by other negative factors in hot environmental conditions. Indeed, the amplitude of electrically evoked M-waves and H-reflexes decreases at high temperatures, suggesting an alteration in peripheral neural drive transmission.Citation66 This decrease is likely due to a reduction in the opening time of the voltage-gated sodium channels, leading in turn to a decrease in the amplitude, duration and area of the axon potential.Citation67 This is in line with a negative correlation between skin temperature (Tskin) and the amplitude, duration, area and latency of a compound action potential,Citation68 with a Q10 effect on nerve conduction velocity between 1.1 and 2.4.Citation69 As a consequence, there is likely no benefit for neural drive transmission of increasing Tm to elevated levels. Even if defining an optimal temperature is contentious, as it depends on several intrinsic and extrinsic factors, it is generally accepted that a Tm slightly above resting should be aimed for when warming-up in temperate environments.
Non-thermal effects of warming-up on neural drive generation
Warming-up has psychological effects potentially improving mental preparedness. For example, warming-up provides the athlete a dedicated time to focus on the eventCitation70 and practice mental imagery,Citation71 thus offering important psychological effects, beside peripheral thermal and metabolic changes.
Cardiovascular and metabolic adjustments
Effect of increasing temperature through warming-up on substrate provision and utilization
Cold exposure can increase muscle glycolysis and lactate accumulation suggesting a lower muscle efficiency and/or an effect of a lower perfusion in cold muscle.Citation72 Conversely, the increase in local vasodilation due to a temperature increase might benefit substrate delivery and metabolite removal.Citation48 This suggests that warming-up may have metabolic advantages, at least in cold conditions. Moreover, increasing temperature improves oxygen release from hemoglobinCitation73 and myoglobin.Citation74 However, increasing Tm increases ATP utilization with an increase in creatine phosphate degradation and anaerobic glycolysis.Citation75 This might be viewed as a positive pre-conditioning adjustment for short explosive activities, but might have negative consequences for prolonged exercise as this represents an increase in energy demand.
Non-thermal cardiovascular effects of warming-up
Abnormal electrocardiographic (ECG) responses suggestive of cardiac ischemia have been reported in 70% of participants running at a high intensity for 10–15 s without warming-up.Citation76,Citation77 This very high prevalence was observed in asymptomatic males (age 21 to 52 y old) having otherwise normal ECG at heart rates of 170 bpm or higher when the load was progressively increased.Citation76,Citation77 This phenomenon is likely due to an incapacity of the coronary blood flow to adapt fast enough. However, this abnormal ECG response is minimized or even suppressed by warming-up.
Injury prevention
Warming-up is commonly considered as one of the basic tools to reduce injury risk.Citation3 For example, several studies have reported a decrease in injury prevalence in professional athletes after incorporating preventive physiotherapy programs including supervised warm-ups.Citation78,Citation79 However, the specific role of the warm-up in these studies cannot be discerned as it was accompanied by other interventions such as taping, recovery, rehabilitation or core stability exercises.Citation78,Citation79
Effect of increasing temperature through warming-up on injury prevention
In cold environments, there is an increase in antagonist/agonist muscle co-activation slowing movement velocity.Citation64 This slowing impairs performance but could also act as a protective mechanism for muscle injury.Citation31,Citation64 A warm-up increasing body temperatures will counteract these effects and therefore increase performance in cold environments, especially during fast movements.Citation30 In addition, warming-up has also been reported to increase flexibility in winter sports performed in cold environments such as downhill skiing.Citation80 Indeed, increasing temperature increases extensibility in tendonCitation81 and other connective tissues.Citation82 As such, increasing peripheral tissue temperature with a warm-up could reduce injury risk.Citation3,Citation81,Citation82
Other injury-prevention effects of warming-up
Animal studies have shown that preconditioning the muscle by electrically evoked contractions increased its stretch length and the force required to tear the fibers.Citation83 While a part of this effect might be related to the minor (i.e., 1°C) rise in Tm reported by the authors, this study showed that preconditioning contractions reduced injury-risk.Citation83 An active warm-up also often includes stretching activities.Citation84 Stretching during warm-up was traditionally passive but it has shifted toward more dynamic exercises in the last decades.Citation84 While there is not enough data available to conclude on the effect of including stretching in the warm-up routine on injury prevention, some authors have suggested that this practice might reduce injury risk.Citation3,Citation84 Indeed, both passively stretching or electrically contracting a muscle decreases the passive tension of the muscle-tendon unit.Citation85 Moreover, warming-up has been reported to reduce the magnitude of delayed onset muscle soreness (DOMS) 48 h after exercise involving a high eccentric component.Citation86
The physiology of heating-up
A rise in whole-body temperature and in particular Tm enhances explosive skeletal muscle performance (e.g., sprinting and jumping) by improving metabolic and contractile function, nerve conduction, and conformational changes associated with muscle contraction.Citation30,Citation87,Citation88 Conversely, the development of thermal strain, an elevation in Tcore, Tskin and Tm, is associated with increased fatigue development during sustained maximal voluntary isometric contractions (MVCs)Citation66,Citation89-Citation91 and impairs aerobic performance.Citation92,Citation93-Citation96 Moreover, while warming-up has health benefit to prevent cardiac ischemia,Citation76,Citation77 and reduce the risk of injury,Citation79 heating-up carries a health risk. Indeed, the development of hyperthermia increases the risk of exertional heat illness (EHI), which is a serious health hazard.
Neuromuscular function
In contrast to the beneficial effects of warming-up, the development of whole-body hyperthermia impairs neuromuscular function with alterations occurring at both the central and peripheral level. From a central perspective, elevated heat stress can lead to a reduction in voluntary muscle activation and the loss of force production capacity.Citation66,Citation89,Citation97 At the skeletal muscle level, a rise in temperature (e.g., 2–3°C) increases contractile speed (i.e., twitch contraction and relaxation time decrease) and alters the force/frequency relationship.Citation44,Citation98
Central activation failure
Brück & OlschewskiCitation99 were among the first to postulate that heat stress might affect brain function and influence exercise performance. The authors identified 3 factors of discomfort that might counteract motivation and progressively reduce the drive to exercise during hyperthermia. They suggested that the interaction of circulatory, thermal, and muscular discomfort determined endurance time and work rate. Nielsen et al.Citation100,Citation101 subsequently proposed that hyperthermia per se, rather than circulatory failure, was the critical factor causing exhaustion during exercise under heat stress. It was further purported that the attainment of a high Tcore (39.2–39.7°C) might influence the CNS by reducing mental drive (i.e., motivation) for motor performance.Citation100,Citation101 Interestingly however, the capacity to generate force during a brief (3–5 s) MVC of the knee extensors and elbow flexors was unaltered after exhaustive cycling under heat stress.Citation101 This capacity to maintain force production was also preserved during 40 repeated MVCs.Citation89 Notwithstanding, force production was progressively impaired during a sustained (120 s) MVC relative to a contraction performed after exercise in cool conditions. The impairment was attributed to a hyperthermia-induced reduction in voluntary activation.Citation89 Others have also reported that the progressive increase in Tcore via passive heating is paralleled by a decrease in voluntary activation and force production during 3–5 s MVCs.Citation97,Citation102 The authors further suggested that Tcore was the primary thermal input mediating hyperthermia-induced fatigue, as rapid cooling of the skin and muscle failed to improve voluntary drive, despite large reductions in cardiovascular and psychophysical strain. Taken together, these observations in isolated muscle contractions led to the suggestion that reaching a core temperature of ∼40°C may not only influence performance in an MVC due to the development of central fatigue, by may also account for the hyperthermia-induced fatigue that develops during prolonged dynamic exercise in the heat.Citation89,Citation103 However, the development of fatigue during exercise in the heat is multi-factorial and associated with the interaction of several physiologic and psychological processes, and not caused by one single factor.Citation43,Citation93,Citation104 As such, use of the term “critical core temperature” in a reductionist manner is misleading when characterizing the influence of hyperthermia on the development of fatigue during exercise under heat stress.Citation105
Indeed, the development of exercise-induced hyperthermia has been shown to impact on performance at both the central and peripheral levels. In a recent study, Lloyd et al.Citation106 examined the impact of a range of Tm (22 to 38.5°C) on voluntary drive during brief (3 s) and sustained (120 s) MVCs. The authors reported that with Tcore remaining relatively stable, voluntary activation and hence force production, was inversely related to Tm during sustained contractions, but unaffected during brief MVCs. The observed reduction in voluntary drive was assigned to altered peripheral fatigue rates and/or sensitivity to peripheral fatigue occurring during the sustained contraction. It was estimated that a ratio of 5.5 to 1 represented the impact of Tcore and Tm on voluntary muscle activation, respectively.Citation106 Périard et al.Citation107 have also demonstrated that voluntary activation and force production are similarly reduced during a 20 s MVC following self-paced exercise in hot and cool conditions. The authors reported that the post-exercise decline in voluntary activation accounted for ∼20% of the decrease in total force production. It was thus suggested that the 0.8°C higher Tcore (39.0 vs. 39.8°C) at time trial completion in the heat did not exacerbate central fatigue and that the loss of force was mainly of peripheral origin and a consequence of the prolonged contractile activity associated with exercise.Citation107 It has also been shown that a similar increase in Tcore (39.5–39.8°C) either via active or passive heating elicits a similar reduction in voluntary activation during a sustained (45 s) MVC, but that a faster rate of decline in force production capacity occurs following exercise.Citation90 Together, these studies indicate that the loss of force production capacity originates from both central and peripheral fatigue factors, with the combination of heat stress and prior contractile activity (i.e., exercise) exacerbating the rate of decline.
Figure 2. Hyperthermia reduces the ability to sustain force/torque production (upper panel) and neural drive to the muscle (lower panel) during a 120-s maximal voluntary contraction. Data represent mean and 95% confidence-intervals from 14 participants in control or hyperthermic (rectal temperature 39°C) state. Reproduced with permission from Racinais et al.Citation111
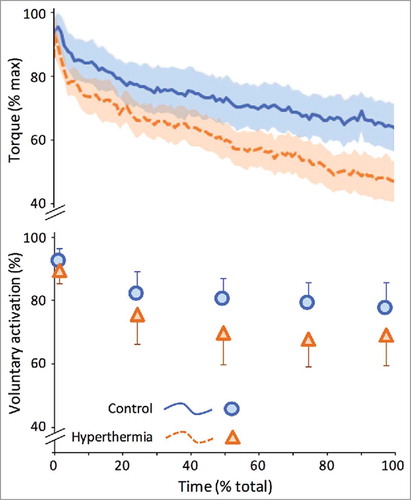
To separate the effect of hyperthermia from the effect of exercise, several studies have used a passive hyperthermia approach. In the absence of exercise, passive hyperthermia has been shown to affect the peripheral nervous system, but also to induce a supraspinal failure when a contraction is prolonged.Citation66 Consequently, hyperthermia reduces voluntary activationCitation66,Citation97,Citation102 (). To identify the mechanism(s) and localize the site of failure in voluntary activation during hyperthermia, Todd et al.Citation108 induced passive hyperthermia (38.5°C) and evaluated brief (2–3 s) and sustained (2 min) MVC performance in the elbow flexors with transcranial magnetic stimulation (TMS). They observed that hyperthermia induced decrements in voluntary torque and cortical activation during both contractions, with decreases being greater in the sustained MVC. Interestingly, the authors noted that peak muscle relaxation rate (measured during the silent period following TMS) during the sustained contraction was ∼20% faster in hyperthermia. This observation of an increase in contractile speed within heated muscle corroborated previous data,Citation98,Citation109,Citation110 and led to the suggestion that the greater central fatigue observed during longer contractions may be indicative of a failure in voluntary drive to account for temperature-related adjustments in muscle contractile speed. Hence, while high motor unit firing rates may be transiently attained during brief MVCs, these may not be sustained during prolonged contractions.Citation108 To further investigate the potential influence of a temperature-induced increase in motor unit firing rate in mediating the additional central fatigue observed during sustained contractions, Périard et alCitation91 actively and passively heated participants from 37.1 to 38.5, and on to 39.4°C. The authors demonstrated that both active and passive hyperthermia increased peak muscle relaxation rate when performing brief (5 s) and sustained (30 s) MVCs. Moreover, an increase from moderate (38.5°C) to severe (39.5°C) passive hyperthermia further increased relaxation rate, but without exacerbating force loss or voluntary muscle and cortical activation. It was thus concluded that centrally mediated rates of activation (i.e., motor unit firing) are sufficient to overcome active and passive hyperthermia-induced increases in peak muscle relaxation within physiologically relevant ranges (i.e., 10–30 Hz).Citation91 As such, the reduction in voluntary muscle activation noted during hyperthermia, whether induced actively or passively, does not appear to originate from a failure in voluntary drive to account for temperature-related adjustments in muscle contractile speed. Rather, voluntary activation failure is partly link to alterations in the peripheral transmission of the neural drive and the supraspinal generation of neural drive when contractions are prolonged.Citation66 Of note, peripheral alterations are not protected by heat acclimationCitation111 and voluntary activation remains depressed.Citation45 However, the supraspinal failure is reverted by acclimation, thus protecting from the additional hyperthermia-induced decrease in force when contractions are prolonged in acclimated humans.Citation111
Peripheral perturbations
High Tcore and Tm have been shown to influence muscle function and cellular metabolism in humans. Indeed, exercise in the heat leads to a greater reliance on muscle glycogen and anaerobic metabolism,Citation112,Citation113 and causes a greater post-exercise accumulation of ammonia as well as muscle and blood lactate.Citation75,Citation112,Citation114 This elevated muscle lactate production is linked with muscle fatigue, and the decline in force observed during work at high glycolytic rates.Citation87 It is also highly correlated with the release of force-depressing free hydrogen ions (H+).Citation115,Citation116 Temperature-induced impairments in sarcoplasmic reticulum function or structural damage compromising sarcoplasmic reticulum calcium ion regulatory capacity may also influence skeletal muscle force production.Citation87,Citation117
Moreover, it is well established that isometric exercise involves partial to complete occlusion of blood flow,Citation118,Citation119 further increasing Tm and stimulating chemoreflexes and mechanoreflexes.Citation120,Citation121 The afferent limb of these reflexes (i.e., group III and IV polymodal fibers) responds to chemical, mechanical and thermal stimulation,Citation122,Citation123 which increases muscle sympathetic nervous activity.Citation124 Depending on the intensity of contraction, the rise in muscle sympathetic nervous activity can alter motor unit excitability, modifying the relationship between central neural drive, motor unit recruitment, and firing rate coding.Citation125,Citation126 Impairment in neuromuscular function may thus relate to failure in the peripheral transmission of neural drive at any level from cortical activity to sarcolemma depolarization.Citation66 Although hyperthermia influences neuromuscular performance, it has been demonstrated recently that chronic exposure to heat stress (i.e., heat acclimation) improves skeletal muscle contractile function.Citation45 This occurs via increases in evoked peak twitch amplitude, torque production at a given voluntary activation as well as improvement in the relative torque/EMG linear relationship ().
Figure 3. Heat acclimation increases peak twitch amplitude in both normothermic (COOL) and hyperthermic (HOT) state (left panel). Heat acclimation also improves the torque/EMG relationship (right panel). Reproduced with permission from Racinais et al.Citation45
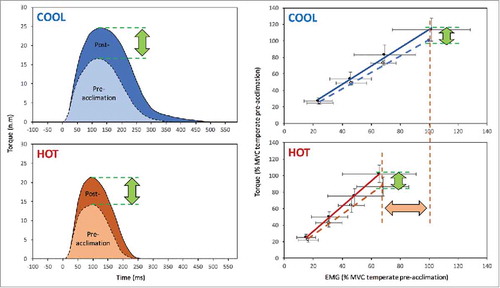
Perceptual and mental perturbations
The reduction in voluntary activation observed under heat stress (i.e., hyperthermia-induced central fatigue) may well represent a psychophysiological phenomenon whereby central neural drive is reduced via alterations in neuromuscular function and motivation. Indeed, considerable effort is required to sustain a maximal contraction, along with a willingness to withstand discomfort and pain. Mild sensations of discomfort are generally sensed at the onset of a contraction, which eventually develop into severe pain that alters the perception of sensations in the contracting musculature.Citation127 As a result, mental fatigue, which involves tiredness, limited attention span and an aversion or decreased commitment to continuing a task or activity,Citation128 may contribute to decrements in voluntary activation. Conscious signals originating from both central and peripheral afferent pathways could mediate behavior and reduce motivation in order to minimize discomfortCitation129 and lead to the abandonment of a task in which the energetic demands (i.e., effort) outweigh the perceived benefits of continued performance.Citation130 Essentially, a lack of motivation can cause central neural drive and motoneurone firing to decline, leading to a loss of force production.Citation131
During dynamic exercise, it has recently been demonstrated that endurance exercise capacity at 80% VO2max in a hot environment (30°C) is impaired by both a passive increase in Tcore prior to exercise, and a 90 min mentally fatiguing task.Citation132 It was further demonstrated that the performance impairment was exacerbated by the combination of these interventions, which acted synergistically to influence the exercise task. In contrast, a mentally fatiguing task undertaken prior to 45 min of moderate cycling and a ∼15 min self-paced cycling effort in 30°C did not influence time trial performance.Citation133 This was likely due to mental faculties already being strained by the combination of high intensity exercise and hot environmental conditions, and the mentally fatiguing task failing to increase negative valence and perceived exertion. A similar mechanism (i.e., failure in down-regulating perceived exertion) was purported to modulate the inability of carbohydrate mouth rinsing to improve 60-min cycling time trial performance in hot-humid conditions, which appears to have been influenced more significantly by the elevated levels of thermal and cardiovascular strain.Citation134 Notwithstanding, Flouris and SchladerCitation135 have suggested that thermal perception influences the rating of perceived exertion and concomitantly work rate at the onset of exercise when only Tskin is elevated. However, as thermal strain increases, factors associated with cardiovascular strainCitation94,Citation95,Citation136 more likely mediate the rating of perceived exertion and the voluntary reduction in work rate.
Cognitive function
A moderate increase in core temperature can initially improve cognitive function.Citation137 Conversely, the development of hyperthermia can impair cognitive function,Citation138,Citation139 with the impairments being task and complexity dependent.Citation140-Citation144 It has been suggested that the performance of cognitive tasks under heat stress deteriorates when the total cognitive resources are insufficient to support both the adequate completion of the task and processing of the thermal stress.Citation139 As such complex tasks are more sensitive to hyperthermia than simple tasks.Citation140,Citation141,Citation143,Citation144 Importantly, variations in temperature have been shown to induce pleasure or displeasure if they favor or perturb homeostasis, respectively.Citation145 Thus, a model was developed linking the decrement in complex task performance to the alliesthesial change accompanying compensatory physiological responses to hot environmental conditions.Citation144 More specifically, increases in temperature during heat exposure generated unpleasant stimuli, as measured by the Positive and Negative Affect Schedule (PANAS), which could be considered as a ‘cognitive load’. It was proposed that this load might reduce the available resources for concurrent cognitive tasks. Interestingly, this model could explain why reducing thermal discomfort, by cooling the head for example, can restore some complex cognitive functions in a hot environment.Citation66,Citation142 Lastly, based on recent data showing that passive hyperthermia increased the rate of false alarms during a sustained attention task,Citation142 and led to faster but false responses during a complex planning task,Citation143 it has been suggested that hyperthermia may increase impulsivity.Citation144
Thermal and circulatory strain
Skin blood flow
The circulatory requirements associated with aerobic exercise under heat stress include an increase in skin blood flow and the maintenance of cerebral and exercising muscle perfusion. Although a transient vasoconstrictor-mediated reduction in skin blood flow occurs at the onset of exercise, cutaneous blood vessels eventually dilate to aid in the dissipation of accumulating metabolic heat. The Tcore threshold at which skin blood flow begins to rise is directly related to exercise intensity, and a delay in active vasodilation shifts this threshold to the right relative to rest.Citation146–Citation149 During prolonged exercise in the heat, the rate of rise in skin blood flow markedly decreases after 20–30 min when Tcore nears 38°C, reaching a virtual plateau at ∼50% of maximum flow capacity.Citation150,Citation151 At this point, the perfusion requirements of exercising muscles take precedence over thermoregulatory control. Despite this attenuation and the potential for splanchnic and renal vasoconstriction to redistribute 600–800 ml.min−1 of blood to the periphery, the large displacement of blood (6–8 l.min−1) to cutaneous vascular beds mediates a progressive decline in arterial pressure, central venous pressure and stroke volume that is accompanied by an increase in heart rate.Citation152,Citation153 Interestingly, the relationship between Tskin and skin blood flow is minimally influenced by Tcore.Citation151,Citation154 However, a strong link exists between the core-to-skin temperature gradient and skin blood flow. For example, a 10°C increment in ambient temperature is associated with a 4.5°C decrease in the core-to-skin temperature gradient.Citation155 This narrowing of the gradient leads to a reflexive rise in skin blood flow,Citation154,Citation156,Citation157 enhancing non-evaporative heat loss to the environment in compensable conditions.
Muscle blood flow and oxygen delivery
In exercising muscles, blood flow requirements relate to a rise in relative exercise intensity whereby the increase in oxygen demand is matched by an increase in systemic and muscle oxygen delivery, as well as perfusion pressure.Citation158 Interestingly, recent studies show that elevated tissue/blood temperatures also induce an increase in skeletal muscle blood flow at rest and during exercise.Citation24,Citation159,Citation160 The mechanism(s) mediating this increase may include an interaction of metabolic and thermal stimuli inducing the release of erythrocyte-derived ATP, a potent vasodilator.Citation160 In absolute terms, muscle blood flow can increase from ∼0.3 l·min−1 at rest, to 10 l·min−1 during maximal exercise in less than 10 s.Citation161 If work rate is stable, a steady-state is reached within 30–90 s and blood flow rises only slightly when exercise intensity increases.Citation162 As such, muscle blood flow and oxygen uptake are quite stable during prolonged exercise at fixed intensities in thermoneutral conditions.Citation163 Under conditions of heat stress however, a rise in thermal strain exacerbates the cardiovascular response as metabolic and thermoregulatory processes compete for cardiac output.Citation152,Citation164,Citation165 The concept of competition or conflict between regulatory systems has recently been suggested to rather represent commensalism, which is an integrated balance of regulatory control where one circulation benefits without substantially affecting the other.Citation166
The classic hypothesis of circulatory/cardiovascular limitations to exercise in the heat proposed by RowellCitation152 lies with the redistribution and peripheral pooling of blood, which reduces central blood volume and concomitantly ventricular filling pressure, end-diastolic volume, and stroke volume. A more contemporary hypothesis suggests that the reduction in stroke volume is primarily due to an increase in intrinsic heart rate,Citation167-Citation169 mediated by the direct effects of temperature on the sinoatrial node, and/or baroreflex modulation of sympathetic and parasympathetic activity.Citation170 In effect, the decline in stroke volume during moderate intensity prolonged exercise in the heat likely originates from both a reduction in central blood volume reducing cardiac filling pressure, and a shorter diastolic filling time reducing ventricular end-diastolic volume. Indeed, when exercise is sustained in highly motivated individuals, the attainment of maximum or near maximum heart rate is a well-documented response.Citation92 Concurrently, maximum cardiac output is decreased,171172 and the cardiovascular system is forced toward a functional limit at submaximal workloads and oxygen uptake.Citation173-Citation175 It is suggested that this reduction in cardiovascular reserve is the primary factor limiting constant rate aerobic exercise, and manifested as an increase in relative exercise intensity and perceived exertion for a given workload.Citation43,Citation129,Citation171,Citation172,Citation176
Cheuvront et al.Citation93 have previously highlighted that the Tcore tolerated at exhaustion is inversely related to whole-body skin blood flow requirements, suggesting that fatigue during exercise in the heat may correspond with adjustments in muscle and cerebral perfusion related to increases in cardiovascular strain. During short but intense cycling at 80% of peak power output, Gonzalez-AlonsoCitation177 noted that to exhaustion was associated with a reduction in systemic and exercising muscle blood flow, oxygen delivery and uptake. These reductions were exacerbated by heat stress, which accelerated the decline in mean arterial pressure and cardiac output, reducing VO2max. It has also been shown that during maximal incremental and constant rate exercise, systemic oxygen delivery is blunted by a plateau or decrease in cardiac output at intensities below VO2max.Citation178 From 50–90% VO2max, systemic and exercising muscle blood flow, along with oxygen delivery, match the rise in VO2. However, beyond 90% VO2max a levelling off occurs that attenuates the rate of rise in VO2, despite maximum increases in arteriovenous oxygen difference and heart rate. This impairment indicates an inability of the cardiovascular system to sustain a linear increase in oxygen delivery to exercising muscles. Aerobic power and capacity are therefore impaired by reductions in cardiac output and oxygen delivery to the exercising musculature, partly due to an enhanced muscle sympathetic nervous activity which attenuates leg blood flow.Citation178-Citation180 These findings have been corroborated during maximal and supramaximal intensity cyclingCitation181 and although metabolic energy requirements are greater in supramaximal exercise, a plateau in cardiac output and exercising muscle vascular conductance was noted at similar levels of oxygen delivery at both intensities. Taken together, these observations challenge the postulate that muscle oxygen delivery increases linearly from rest to VO2max.Citation158,Citation182,Citation183 During prolonged submaximal exercise in the heat, a similar compromise in oxygen delivery appears to occur, especially as heart rate nears maximum.
The impairment in prolonged self-paced exercise occurring in the heat () has also been attributed to this detrimental increase in circulatory/cardiovascular strain.Citation93-Citation95,Citation136,Citation184,Citation185 It has been shown that a thermoregulatory-mediated rise in cardiovascular strain is associated with reductions in VO2max and power output during prolonged (60 min) intense self-paced cycling in the heat ().Citation94 Although exercise in both hot and cool conditions leads to a progressive decrease in VO2max, the extent of the decrement is greater in the heat.Citation95 Despite the larger decrease in aerobic capacity and concomitantly work rate (e.g., power output), relative exercise intensity (i.e., %VO2max) in the heat is maintained within a fairly narrow range (i.e., 2–5%), similar to that of cooler conditions. This range widens under heat stress however, as exercise becomes protracted and a disassociation develops between relative exercise intensity and heart rate and RPE.Citation95
Figure 4. Power output and cardiovascular response during a 40-km cycling time trial in Hot (35°C) and Cool (20°C) conditions. Values are means ± SD for 8 subjects. ∗Significantly different from Cool (P < 0.05). §Significantly higher than previous (P<0.01). †Significantly lower than 10 min (P < 0.05). Reproduced with permission from Périard et al.Citation17
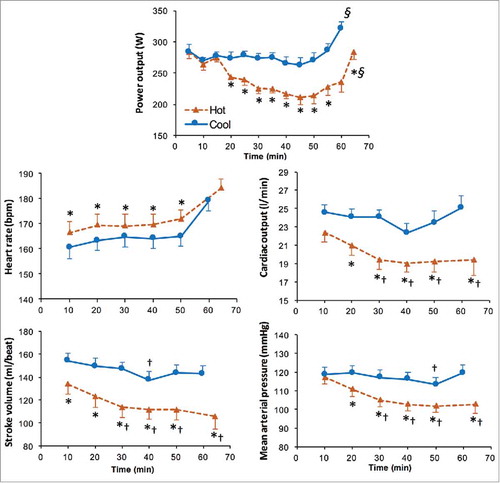
Cerebral blood flow
The development of hyperthermia is associated with a progressive reduction in cerebral blood flow during self-paced exercise (), attributed in part to an increase in cutaneous blood flow, decreases in cardiac output and arterial blood pressure, and hyperventilation-induced hypocapnia.Citation184,Citation186-Citation188 Interestingly, reductions in cerebral blood flow and concomitantly oxygen delivery to the brain, have been suggested to compromise central neural drive to exercising muscles during strenuous exercise in the heat.Citation186,Citation189,Citation190 However, the development of fatigue during such exercise is associated with an enhanced cerebral metabolism,Citation191 manifested by a compensatory increase in oxygen extraction in the brain.Citation192 Moreover, comparable impairments in force production capacity have been observed following self-paced cycling (40 km) in hot and cool conditions,Citation107 and exhaustive incremental exercise has been shown to decrease voluntary activation by the same extent in hot and cool conditions.Citation43 It therefore does not appear that an exacerbated reduction in cerebral blood flow under heat stress mediates performance decrements via central inhibition. Notwithstanding, the development of hyperthermia does influence the ability to generate muscle force.
Figure 5. Middle cerebral artery mean blood velocity (MCA Vmean) during a self-paced time trial (750 kJ) in HOT and COOL conditions. Values are means ± SEM. *Significant difference between HOT and COOL (P < 0.05). §Significantly higher than all other values (P < 0.05). Reproduced with permission from Périard and Racinais.Citation184
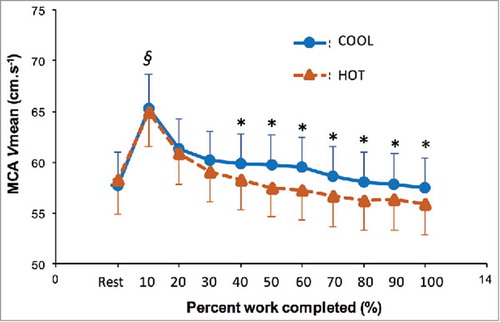
Exertional heat illness
Environmental heat stress and physical activity interact to increase the risk of EHI. This risk represents a continuum of medical conditions with potentially severe consequences that can affect physically active individuals in both hot and temperate environments. The severity of EHI can escalate from relatively benign symptoms such as muscle cramping, to heat exhaustion, heat injury and heat stroke.Citation5,Citation19,Citation193 Heat exhaustion is considered a mild to moderate EHI associated with a Tcore of 38.5 to 40°C and an inability to maintain cardiac output. Heat injury is defined as a moderate to severe illness characterized by organ (e.g., liver and renal) and tissue (e.g., gut and muscle) damage with a high body temperature, typically >40°C. The most severe EHI, heat stroke, is characterized by high body temperatures, profound central nervous system dysfunction (e.g., combativeness, delirium, seizures and coma), as well as organ and tissue damage, and can lead to death. Unlike classic heat stroke, which is primarily observed in vulnerable and immunocompromised populations during seasonal heat waves, exertional heat stroke occurs in healthy young individuals performing strenuous physical activity (e.g., exercise or occupational tasks). Notwithstanding, heat stroke and other EHIs often occur in the presence of certain risk factors, such as extreme environmental conditions, medication and drug use, compromised health status, and underlying genetic conditions.Citation19 Heat stroke often occurs in individuals considered low risk and performing routine physical activities under heat stress.Citation5 Accordingly, it is difficult to identify individuals that may be at risk of EHI. Although the prevalence of EHI increases during exercise performed in the heat, athletes can adopt various strategies to mitigate the influence of heat stress on performance, as well as the risk of heat illness.Citation20 As detailed below, these involve following an individualized hydration regimen, utilizing cooling strategies and most importantly, acclimatizing to the heat.Citation5,Citation194,Citation195
Application to sports – warming-up for performance
Types of warm-up
There are many preconditioning or priming techniques at an individual's disposal prior to competition. These involve preparing the body physically and psychologically. Psychological warm-up strategies (e.g. mental imagery, mental rehearsal) are catered towards enhancing cognitive function, a function that is specifically key in team sports where decision making is one of the primary factors contributing toward successful performance outcome.
A common physical warm-up may consist of progressive intensity building with a range of explosive movements utilizing the stretch shortening cycle, while also utilizing stretching interventions aimed at relieving muscle tension and improving range of motion. While certain strategies are beneficial for maximal strength and force production, others may well be detrimental, which will be discussed in sections below.
Structuring a warm-up
An effective warm-up can last for more than 30-minCitation196 and should include a main component of general exercise (usually sport specific), as well as context specific exercises and flexibility work.Citation80 Such a protocol is commonly used in the field and involves targeting 4 physiological pillars and in 3 specific stagesCitation197: Raise, Activate and Mobilize, Potentiate (RAMP; ). This model, incorporated with appropriate exercise selections that replicate the biomechanical and psychological demands of the chosen competition, should provide the necessary tools for effective warm-up design. Under heat stress, the duration of each stage may differ due to the detrimental effects of heating up. Accordingly, certain adjustments in the warm-up protocol are advised ().
Figure 6. Warming-up for short-, intermediate- and long-duration events and the impact of environmental heat stress on the duration of ‘RAMP’ based warm-up protocols. Physiological underpinning of the “Raise,” “Activate and Mobilize,” and “Potentiate” stages are highlighted for each sporting duration, in addition to demonstration of the potential impact of post-warm up passive heating strategies.
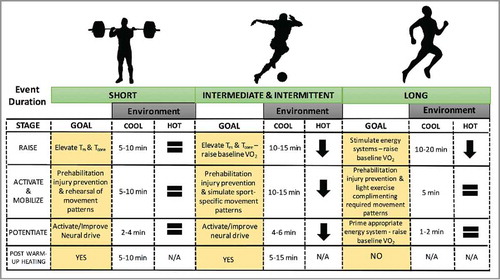
Raise
The first stage (Raise) specifically targets the thermoregulatory homeostatic systems of the body by increasing muscle and by extension core temperature. This stage also impacts upon the heart rate response, blood flow viscosity and ventilation. At the onset of exercise Tm rapidly increases, usually within 3–5 min. It is after 10–20 min of moderate intensity steady-state exercise (80–100% lactate threshold) that Tm plateausCitation198 with elevated temperatures (i.e., 2–4°C) shown to significantly enhance performance.Citation22,Citation198 The increased maximum shortening velocities and development of tension,Citation35 alongside viscosity changesCitation46 in warmer muscles likely prepares the athlete for the next stages of the warm-up. When undertaken in hot climatic conditions, the Raise stage of the warm-up may be shortened for intermediate to prolonged events (), so as to avoid and excessive increase in whole-body temperature.
Activate and mobilize
The second warm-up stage (Activate and Mobilize) can be broken down into 2 parts. Activation is highly specific to not only the working muscle groups, but also the specific function and movement patterns the working muscles carry out during competition. Typically, “activation” can be achieved using now termed “prehabilitation” exercises, defined as “preparing an individual to withstand a stressful event through enhancement of functional capacity”.Citation199 This stage often utilizes light loads, aiming to stimulate the neuromuscular and proprioceptive responses to competitive exercise through simulated movement patterns – a key determinant for chronic-exercise induced neural adaptation.Citation200 Duration of this phase will depend on the individual and competition demands. “Mobilization” usually involves dynamic movements, aiming to activate key muscle groups involved in successful performance outcome. Early research suggested static stretching and proprioceptive neuromuscular facilitation techniques may contribute to improvements in both injury prevention and exercise performance.Citation3,Citation84 More recent observations suggest that while long-term muscle function and performance may be improved from regular stretching interventions,Citation201 there may be little effect of either static stretching or proprioceptive neuromuscular facilitation on various performance outcomes, across a range of intensities.Citation202-Citation204 Recent data suggest high baseline athlete flexibility (e.g. gymnasts), and the specific stretching protocol employed (intermittent vs continuous) may improve the impact of static stretching on performance.Citation205 Dynamic-based stretching and loading likely remains a more effective approach when aiming to maximize athletic readiness during the mobilization stage.Citation206 Given the nature of the activates undertaken in the Activate and Mobilize phases, the types of events most likely to impose a modification of this stage are those of intermediate duration (e.g., team-sports; ).
Post-activation potentiation
Prior to commencing PAP, a warm-up induced functional change to both temperature- and load-dependent actions on muscle and tendinous tissues should be present. This yields a protective effect as greater forces and length of stretch are required to tear isometrically preconditioned (warm) animal muscleCitation83 when compared to cold muscle. PAP can then involve high rates of force production and muscle-length changes at high velocity, thereby involving muscles containing an abundance of fast-twitch fibers. These muscle fiber types in particular may be more susceptible to injury due to their differing functional capacities.Citation207 While increases in Tm lead to improvements in muscle fiber conduction velocity,Citation27,Citation28 these findings do not necessarily relate to muscular activity.Citation208 Unlike the inhibitory neural conduction rate responses that occur when Tm is reduced,Citation23 findings of increased neural conduction rates are not necessarily present when Tm is elevated.Citation208 PAP therefore usually involves performing intense sports-specific movements in order to fully simulate the demands of performance, in turn stimulating the necessary neural pathways.
The PAP phenomenon can be usefully applied prior to events that require production of high forces and power outputs, especially in conditions of elevated TmCitation209. PAP for the power based athlete may be utilized through relatively high-resistance movements,Citation210 with 2 recent studies demonstrating significantly beneficial effects on countermovement jumpCitation211 and vertical jumpCitation212 performances when 1–3 repetitions of resistance conditioning activities ranging from 80–93% 1RM were performed 2–6 min prior to testing. It is recommended that individualized intensities and exercise modalities be employed to suit the capability (maximal strength) of the athlete.
Depending on the demands of the event, the duration and intensity of PAP will vary dramatically. The potentiating benefit in endurance athletes would aim to preserve fatigue at submaximal intensities, through altering low frequency firing rates and force development at the level of the motor unit.Citation210 This would theoretically allow the athlete to achieve higher force for lower calcium activation.Citation213 While potentiation is capable of compensating for low firing frequency at submaximal workloads, potentiation-induced alteration of high frequency forces is not necessarily observed.Citation57 This implies any PAP-induced improvement in sustained workload during fatiguing endurance exercise would be resultant from increased low frequency force, in-turn compensating for the gradual decline in high frequency force that occurs in fatiguing tasks. Indeed, in states of elevated Tm, a rightward shift in the force-frequency relationship occursCitation44 and therefore the benefit of PAP on endurance performance would be counteracted by elevated Tm.210 For the strength- or power-based athlete however, PAP induced under conditions of elevated Tm would be additive (see protocol specificity for more).
Protocol specificity
How long is too long?
The “optimal” warm-up strategy likely varies between sports.Citation4 It also depends on environmental conditions (, ). Indeed, warm-up duration might be reduced before intermediate or prolonged exercise in hot environments (). Warm-ups should be designed to activate the energy systems of interest, while at the same time challenging sport-specific movement patterns the athlete is likely to experience throughout the competition period. This follows recommendations that neural activation is one of the most important mechanisms of warming-up for an athlete.Citation214
Figure 7. Altering a warm-up to accommodate for hot ambient conditions. Some applied protocol recommendations for altering the RAMP protocol; the use of post warm-up passive heating/cooling strategies and altering nutritional intake to reduce the impact of heat stress on subsequent performance. CHO = carbohydrate.
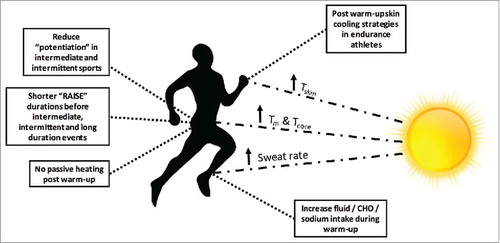
While Raise and Activate and Mobilize may be necessary for most competing athletes, the importance of neural activation is larger for strength- or power-based athletes compared to endurance athletes.Citation210 Equally, longer durations of prior aerobic exercise can have deleterious effects on maximal power production, especially at warm-up intensities >60% VO2max.Citation215 This followed further observations that maximal cycling peak power output was achieved after warm-up sets performed at between 33 and 48% VO2max.Citation215 It may therefore be advisable for strength- and power-athletes to focus on performing light-intensity (< 50% VO2max) exercise during the Raise stage. Once the warm-up is complete, heated garments may be utilized with the aim of maintaining elevated Tm and Tcore prior to strength- or power-based events (see ‘Time between warm-up and competition’). Such post warm-up heating is however not necessary in hot ambient condition and could even be replaced by some cooling strategies in particular circumstances ().
The endurance athlete, depending on the competitive ambient environment, may wish to predominate the undertaking of the Raise and Activate and Mobilize stages. The modality and movement pattern requirements, should dictate emphasis of activation and mobilization. Endurance events that utilize cyclical activities with one predominant movement pattern (i.e., cycling) may reap less benefit from Activation and Mobilization compared with the endurance cross-country runner, or team-sport athlete that constantly undertake multi-directional movements, performed across a range of joint angles and velocities. High load explosive Potentiation prior to competition may not be necessary in endurance athletes. While a prior 6-min exercise bout in endurance trained cyclists can accelerate phase II VO2 kinetics during a subsequent heavy-domain (85% VO2max) exercise task,Citation216 this phenomenon occurs after both moderate- (∼52% VO2max) and high-intensity (85% VO2max) priming.Citation216 As previously alluded to regarding co-activation of both potentiation and endurance-induced fatigue, PAP may naturally occur in fatigued states, even when performing submaximal contractions (i.e., endurance competition). While training status may have influence on this mechanism, the antagonistic nature of elevated Tm on the efficacy of PAP in the endurance athlete, may provide rationale for designing a warm-up that aims to target an efficient priming of aerobic energy systems, rather than focusing on high-intensity potentiating conditioning bouts, or elevating Tm to optimal temperatures to improve sprint- or power-based performance.
The trade-off between priming the body and inducing fatigue is of vital consideration. Limiting variables to performance are highly contrasting between sprint- or power-based tasks and endurance events (e.g., marathon running or cycling races). Therefore, warm-ups should be designed following a needs analysis of each individual sport ().
Time between warm-up and competition
Tm has been observed to decrease exponentially after warming-up,Citation22 and approximately 70% of the warm-up induced elevation in Tcore can be lost during a 15-min transition period between the warm-up and performance task.Citation217 In temperate conditions (≤ 19°C) strategies to maintain Tm and Tcore through either localized muscle heating elementsCitation22 or application of blizzard survival jacketsCitation217 have been shown to be effective interventions in improving power-based exercise tasks. Indeed, applying external heating elements to the limbs can maintain Tm.Citation22,Citation218 However, there is no further benefit in applying such heating elements to the working muscles during the warm-up itselfCitation22 and their use should be restricted between the warm-up and the event. The issue of maintaining the benefits of warming-up is of particular interest to swimmers who cannot swim for ∼20 min after exiting the warm-up pool prior to their event. In this setting, maintaining Tcore and Tm via dry-land exercises may benefit performance, and using heated jackets in addition to conducting sports specific dry-land exercises can further improve performance.Citation219,Citation220 Naturally, event duration plays a pivotal role in the decision to apply heating elements. It is proposed that for a 1°C rise in Tm at depths between 1–4 cm, improvements of 4–10% in peak power output (PPO) could occur.Citation22 Conversely, when assessing more prolonged intermittent-exercise tasks, lower body temperatures may delay the onset of fatigue.Citation221
The classic study by Sargeant & DolanCitation215 showed a decrease in peak power output after a 6 min warm-up at 87% VO2max, with baseline values (i.e., without warm-up) being recovered following just 1 min of rest, but maximal peak power output (9.6% above baseline) being observed after 6 min of recovery.Citation215 This supports recommendations that power- and sprint-based athletes should plan a recovery period of 5–10 min between warm-up completion and competition, first to maintain the benefit of potentiating conditioning workCitation57 and second to preserve elevated Tcore and Tm,22 while simultaneously allowing for sufficient phosphocreatine (PCr) and anaerobic energy system resynthesis.Citation222,Citation223 Endurance athletes on the other hand may wish to simply focus on commencing competition once energy system repletion occurs following appropriate priming warm-up exercises, with the goal of avoiding early onset fatigue via elevated temperature-related mechanisms.Citation221 Indicative durations for each warm-up stages in both cool and hot environments are suggested in .
Warm-up strategies and heat stress
While warm-up strategies should aim to augment performance of previously highlighted metabolic, musculoskeletal and cardiovascular systems via manipulation of intensity, duration and movement patterns; the consideration of first the demands of competition and second environmental stressors, should dictate the specific prescription of the warm-up protocol. In cool conditions, power-based and team-sport athletes may wish to focus on raising Tm and Tcore through intense sports-specific drills that simulate the demands of competition, in turn sufficiently stimulating neural activation following performance of applicable motor recruitment patterns. With passive heating interventions also at an athlete's disposal, the use of Tm maintenance for a power-based athlete (aiming to maintain a 2–4°C Tm elevation) may also improve quality of work. When these sports are undertaken in the heat over, passive heat maintenance is likely unnecessary and may be eliminated.
Endurance-based athletes (e.g., triathletes, marathon runners, cyclists) on the other hand may wish to take a different approach to their pre-competition strategy. The goal of the warm-up in these populations is to optimize physiological readiness through activation of predominant energy systems and movement patterns, without producing unnecessary metabolic heat that may become more performance-limiting the longer an event lasts.Citation224 The impact of a potentiating and temperature elevating warm-up prior to competition is likely intensity dependent, with power and sprint-type tasks showing the greatest improvements.Citation22,Citation196,Citation217
Application to sports – warming-up or cooling-down?
The undertaking of necessary mechanical work, capable of stimulating the responses described above undoubtedly results in thermal homeostatic perturbations, leading to increases in both Tm and Tcore.Citation196,Citation225 In hot and/or humid ambient conditions, these increases can be exacerbated and bring the athlete from a ‘warmed-up’ to a ‘heated-up’ state. The section below focuses on how to minimize thermal strain by employing various cooling techniques as part of the warm-up routine. It also includes recommendations on hydration during warm-up and competition, as well as some suggestions regarding half-time in team-sports.
Cooling-down while warming-up
Skin cooling can reduce cardiovascular strain during exercise in the heat, while whole-body cooling can decrease organ and skeletal muscle temperatures. Several studies carried out in controlled laboratory environments (e.g., uncompensable heat-stress), with or without fanning during exercise, have reported that pre-cooling can improve endurance,Citation92,Citation226-Citation231 and high-intensityCitation232 exercise performance. However, the benefits of pre-cooling on intermittent or repeated-sprint exercise performance vary, with observations of performance enhancementCitation233-Citation236 and no changesCitation233,Citation237-Citation239 in a range of studies. Given its effect of lowering Tm, whole body cooling appears to be detrimental to performance during a single sprint, or the first few repetitions of an effort involving multiple sprints.Citation240,Citation241
Several reviews conclude that prolonged exercise capacity in the heat can be improved following cooling interventions,Citation242-Citation249 however its effectiveness when comparing hot laboratory and competitive environments appears to differ, with suggestions that the benefits of pre-cooling may be overestimated in outdoor settings.Citation250 Hence, the impact of cooling in competition settings remains equivocal and recommendations are currently limited to prolonged exercise bouts in hot ambient conditions, or conditions with limited air flow.
Cold-water immersion
A range of cold water immersion (CWI) protocols are available when aiming to optimize physiological readiness for competition (for reviews see:Citation247,Citation251-Citation253). Notwithstanding, the most common techniques for effectively lowering Tcore include whole-body CWI, usually for ∼30 min at water temperatures between 22−30°C, or body segment (e.g., legs) immersion at lower temperatures (10−18°C).Citation247 More extreme external cooling protocols will lead to more rapid reductions in Tm, in turn having deleterious effects on important priming mechanisms such as nerve conduction rate and muscle contraction velocities.Citation30 Athletes employing these more “aggressive” CWI strategies may therefore need to plan an active re-warming strategy into their competition preparation prior to commencing the event. Aggressive cooling of locomotor muscles will undoubtedly reduce the initial development of thermal and cardiovascular strain, however may hamper performance in the early stages of exercise. As such, various new products such as cooling garments, specifically designed to cool the torso, have been designed to reduce Tcore (or limit its increase) without affecting Tm.
Cooling garments
The original use of ice towels to promote cooling lead to the development of several ice-cooling jacket devices to cool athletes prior to or during exercise in the heat.Citation228,Citation233,Citation254,Citation255 CWI induces greater reductions in Tcore when compared to cooling vest application,Citation249 however cooling vests can effectively lower Tskin, in turn having advantageous effects on both cardiovascular strain and heat storage,Citation256 without deceasing Tm. Cooling through cooling garments carries higher ecological validity as athletes can more easily apply these garments during warm-up periods, or recovery breaks, depending on environmental and competition demands.
Mixed methods cooling strategies
It is suggested that the combined use of internal (e.g., cold beverages) and external (e.g., CWI) cooling strategies can lead to greater cooling capacity when compared to using the same techniques in isolation.Citation249 Mixed cooling methods have so far proven beneficial to football performance in tropical environments,Citation257 lacrosse players training in hot environments,Citation258 and laboratory-based cycling tasks.Citation230 The combination of mixed cooling methods is practically applicable to athletes. Valid mixed cooling methods may integrate the use of ice-slurry beverages, cooling-vests and fanning during or after warm-up.
Hydration during warm-up
It is common for rested, well-fed humans to be sufficiently hydrated.Citation259 However, as fluid replacement capacity is limited while exercising in the heat, it is recommended to avoid being is a state of dehydration before competing in the heat,Citation20 especially during warm-up. A prescribed fluid intake of 5–6 ml of water per kg of body mass, at a frequency of every 2–3 h, as well as 2–3 h prior to either training or competing in the heat is advisable. As with other strategies, this hydration protocol should be practiced prior to its implementation during competition as it will increase urinary output.
While cold fluid ingestion prior to exercise can potentially improve performance,Citation260,Citation261 it is thought that fluid-induced activation of thermoreceptors, probably located in the abdominal area,Citation262 can cause reductions in sweating, reducing the potential for evaporative cooling in dry heat.Citation263 As such, there does not seem to be advantageous performance responses when cold fluids are ingested during exercise.Citation264,Citation265 This practice could however be beneficial during warm-up to support cooling and fluid preservation before the event.
Ice slurry beverages may be a more effective athlete cooling strategy, based on the theory of enthalpy. Ice requires substantially more heat energy (334 J/g) to cause a phase change from solid to liquid (at 0°C) compared with the energy required to increase the temperature of water (4.2 J/g/°C). Several reports recently supported the consumption of ice slurry beverages prior toCitation231,Citation266,Citation267 or duringCitation268 exercise. In order to observe benefits in endurance or intermittent-sprint exercise tasks, ∼1 L crushed ice at ≤ 4°C may be advisable. However, the practicality and evidence for using ice-slurry require additional study. In the meantime, as for cold drink, ice-slurry is likely more suitable during warm-up than competition.
Half-time strategies
Re-warming up
While half-time intervals may provide recovery opportunities for team-sport athletes, previous observations reveal soccer players cover less distanceCitation269 or produce less medium intensity running (11.1 – 19 kph) efforts in the second-half of matches compared with first-half performances.Citation270 This observed decrement could be due to various (thermoregulatory and metabolic) factors. As previously alluded to in this review, reduced Tm is correlated with reduced sprint performance capacity during soccer matches.Citation196,Citation271 Firstly, a 35-min sports specific warm-up lead to a 3°C rise in Tm compared to rest (39.4°C vs. 36°C). After the first half, a 15-min half-time interval produced a natural 2°C reduction in Tm (39.7°C to 37.7°C) in the control group. When an active re-warming (moderate intensity) strategy was introduced 7-min into the half-time break, the natural decline in Tm was reduced to just 0.5°C (39.7°C to 39.2°C), resulting in a similar starting Tm when compared to pre-game temperature (39.2°C vs 39.0°C, respectively). Subsequently, second-half sprint performance was maintained in the active re-warm group.Citation196 In temperate environments, athletes that perform high-intensity efforts should aim to maintain warm-up induced elevations in Tm through either passive heating interventionsCitation22 or moderate intensity active re-warming strategies.Citation196
When environmental heat-stress is added to the competitive environment (i.e., performing in 40°C ambient conditions), increased glycolysis, leading to more rapid depletion of muscle glycogen occurs when compared to exercising in the cool.Citation75 The risk of exercise-induced hyperthermia increases with rising environmental air temperature.Citation271 As such, implementation of half-time cooling techniques (discussed below), rather than interventions aiming to maintain elevated Tm, may help to prolong exercise capacity and avoid early onset of fatigue in hot ambient conditions.Citation221
The first half of soccer matches may lead to significant muscle glycogen depletion by half-timeCitation272 potentially having detrimental effects on performance capacity in temperate conditions.Citation273 These findings are corroborated by Winnick et al.Citation274 showing regular CHO feeding during rest-intervals improves both peripheral and CNS function between 45 and 60 minutes of team-sport activity when compared to placebo feeding.Citation274 In addition, both external heat-stress and higher internal Tm result in increased CHO oxidation rates for a given workload during exercise. As such, appropriate nutritional refeeding during this time-period may therefore contribute to enhance second-half performance (45–90 minutes), both in temperate and hot environments.
Cooling down
The influence of external cooling on reducing skin blood flow and promoting a rise in the central circulation,Citation275 along with improvements in perceptual responses to heat stress,Citation276 may ultimately provide the impetus for enhancing performance. A pre-cooling strategy utilizing CWI for between 5–12 min in 14°C water during a 15-min period between bouts can potentially improve intense exercise in the subsequent effort in hot environments.Citation277,Citation278 The translation between reducing internal heat strain, though ingestion of cold waterCitation279 or ice-slurryCitation280 does not necessarily translate to improved subsequent bout performance outcomes. Together, studies on body cooling (internal or external) suggest that cooling might aid recovery from intense exercise in uncompensable heat-stress in laboratories and, in some cases, might improve performance in subsequent intense exercise bouts. The effects of aggressive cooling versus simply resting in the prevailing hot ambient conditions, or in cooler conditions, remains to be validated in a competition setting (e.g., half-time in team-sports).
Other recommendations to avoid heating-up
Hydration
The development of hyperthermia during exercise in hot ambient conditions is associated with a rise in sweat rate, which can lead to progressive dehydration. Exercise-induced hypo-hydrated states are associated with reductions in plasma volume and an increase in plasma osmolality that are proportional to the reduction in total body water.Citation5 The increase in the Tcore threshold for vasodilation and sweating at the onset of exercise is closely linked to the ensuing hyperosmolality and hypovolemia.Citation281,Citation282 In addition, dehydration decreases cardiac filling and challenges blood pressure regulation.Citation15,Citation283,Citation284 The rate of heat storage and cardiovascular strain is therefore exacerbated and the capacity to tolerate exercise in the heat is reduced.Citation285-Citation287 In competition settings, however, hydration is dependent on several factors, including fluid availability and the demands of the events. As such, hydration is easier during warm-up than competition. Of note, although it is recommended for competitive athletes to minimize their body mass losses, recreational athletes involved in prolonged exercise should be cautious not to overhydrate during the exercise.
Heavy sweaters may choose to deliberately increase sodium intake prior to either training or competing in the heat. Increasing sodium (i.e., salt) intake (e.g., 3.0 g of salt added to 0.5 L of a carbohydrate-electrolyte drink) can help maintain plasma sodium balance in heavy sweaters, as the main electrolyte lost in sweat is indeed sodium (20–70 mEq/L).Citation288,Citation289 In athletes experiencing muscle cramping, it is recommended to increase the sodium supplementation to 1.5 g/L of fluid.Citation290 Athletes should also aim to include 30–60 g/h of carbohydrates in their hydration regimen for exercise lasting longer than 1 h,Citation291 and up to 90 g/h for events lasting over 2.5 h.Citation292 This can be achieved through a combination of fluids and solid foods.
Heat acclimatization
Appropriate preparation for exercising in the heat starts a long time before warming-up. Repeated training in the heat increasing body (Tcore and Tskin) temperature, sweating and skin blood flow induces heat acclimatization.Citation5,Citation293-Citation297 Heat acclimatization can lead to various advantageous physiological responses when exercising in the heat. These include increased sweating and cutaneous skin blood flow responses, better matching of fluid-electrolyte balance, plasma volume expansion subsequently improving the ability to sustain blood pressure and cardiac output.Citation5,Citation194,Citation293 These changes enhance submaximal and maximal aerobic exercise performance in warm-hot conditions.Citation101,Citation298,Citation299 Most adaptations occur within the first week of training in the heat and continue to change at slower rates over the following 2-weeks.Citation300-Citation302 If an athlete is constrained by time leading into a competition, they may well therefore benefit from only a few days of acclimatization.Citation303-Citation305 In order to achieve near complete cardiovascular and sudomotor adaptations, however, as much as 2 weeks training may be neededCitation101,Citation298,Citation306 to optimize aerobic performance in hot conditions.Citation299 Athletes who do not have the possibility to travel to naturally hot ambient conditions (so called “acclimatization”) can train in an artificially hot indoor environment (so called “acclimation”).
In addition, it is worth mentioning that someCitation298,Citation307 but not allCitation308,Citation309 studies have reported an increase in exercise capacity in cool conditions. This cross benefit is currently debated,Citation310,Citation311 but seems to be supported by both early,173312 and recentCitation313,Citation314 observations. For example, field studies have observed performance improvements in temperate conditions following pre-season training camps in the heat.Citation315-Citation317 While the mechanisms, magnitude and populations benefiting of such cross adaptations remain to be clarified, it should be acknowledged that there is no evidence that heat acclimation impairs cool weather performance. As such, heat acclimation should be implemented by default before a competition with uncertain environmental conditions potentially changing from temperate to hot.
Conclusion
One of the primary aims of warming-up is to increase Tm. This has several benefits for performance, to which we should add the non-temperature related benefits of warming-up (e.g., psychological preparedness). However, the concomitant increases in thermal strain (i.e., Tskin and Tcore) might be detrimental to prolonged exercise capacity in hot ambient conditions via the development of cardiovascular strain. The warm-up routine should therefore be adapted to the environmental conditions. For example, cooling interventions (e.g., cooling-vest) can minimize the increase in Tskin and Tcore during the warm-up. Athletes should also minimize dehydration during the warm-up. Athletes and coaches should keep in mind that warming-up induces both temperature and non-temperature related responses and is an important part of the pre-competition preparation in all environmental conditions. However, while an increase in whole-body temperature is beneficial to performance in cold environments, the warm-up should be adapted before exercising in the heat to promote muscle adaptations while minimizing the increase in Tskin and Tcore based on the event.
Abbreviations
CHO | = | carbohydrate |
EHI | = | exertional heat illness |
MVCs | = | maximum isometric voluntary contractions |
PAP | = | post-activation potentiation |
RAMP model | = | Raise, Activate and Mobilize, Potentiate |
Tcore | = | core temperature |
Tm | = | muscle temperature |
Tskin | = | skin temperature |
VO2 kinetics | = | oxygen uptake kinetics |
VO2max | = | maximal oxygen uptake |
Disclosures of potential conflicts of interest
No potential conflicts of interest were disclosed.
About the authors
Dr Sébastien Racinais is the head of the Athlete Health and Performance Research Centre at Aspetar. His research focus on the adaptations of the human body to hot ambient conditions and their repercussions on sport performance. He runs the Special Interest Group (SIG) in environmental physiology and has been consulting for, or collaborating with, various international sports federations to protect athlete health and performance in extreme environmental conditions.
Scott Cocking is a PhD candidate at the Athlete Health and Performance Research Centre at Aspetar, in conjunction with Liverpool John Moores University. His current research investigations assess the effect of ischaemic preconditioning, both on exercise performance and vascular function. His research interests extend to pre-competition performance strategies, and nutritional ergogenic aids.
Associate Professor Julien Périard is a Research Professor at the University of Canberra Research Institute for Sport and Exercise. His research examines the integrative physiological mechanisms that mediate health and performance in adverse environmental conditions (i.e., heat and altitude). He has worked with both amateur and professional athletes from various disciplines, along with National and International Federations.
References
- Milton AS. Thermal physiology: Brief history and perspectives. In Blatteis CM, editor. Physiology and pathophysiology of temperature regulation. Singapore: World Scientific; 1996. p. 3-12.
- Saltin B, Hermansen L. Esophageal, rectal, and muscle temperature during exercise. J Appl Physiol. 1966;21:1757-62. PMID:5929300.
- Shellock FG, Prentice WE. Warming-up and stretching for improved physical performance and prevention of sports-related injuries. Sports Med. 1985;2:267-78. doi:10.2165/00007256-198502040-00004. PMID:3849057.
- Bishop D. Warm up II: Performance changes following active warm up and how to structure the warm up. Sports Med. 2003;33:483-98. doi:10.2165/00007256-200333070-00002. PMID:12762825.
- Sawka MN, Leon LR, Montain SJ, Sonna LA. Integrated physiological mechanisms of exercise performance, adaptation, and maladaptation to heat stress. Compr Physiol. 2011;1:1883-928. doi:10.1002/cphy.c100082. PMID:23733692.
- González-Alonso J, Quistorff B, Krustrup P, Bangsbo J, Saltin B. Heat production in human skeletal muscle at the onset of intense dynamic exercise. J Physiol. 2000;524 Pt 2:603-15. doi:10.1111/j.1469-7793.2000.00603.x. PMID:10766936.
- Saltin B, Gagge AP, Stolwijk JA. Muscle temperature during submaximal exercise in man. J Appl Physiol. 1968;25:679-88. PMID:5727193.
- Montain SJ, Sawka MN, Cadarette BS, Quigley MD, McKay JM. Physiological tolerance to uncompensable heat stress: Effects of exercise intensity, protective clothing, and climate. J Appl Physiol. 1994;77:216-22. PMID:7961236.
- Roberts MF, Wenger CB. Control of skin circulation during exercise and heat stress. Med Sci Sports. 1979;11:36-41. PMID:481154.
- Kerslake DM. Factors concerned in the regulation of sweat production in man. J Physiol. 1955;127:280-96. doi:10.1113/jphysiol.1955.sp005257. PMID:14354672.
- American College of Sports Medicine, Sawka MN, Burke LM, Eichner ER, Maughan RJ, Montain SJ, Stachenfeld NS. American College of Sports Medicine position stand. Exercise and fluid replacement. Med Sci Sports Exerc. 2007;39:377-90.
- Maughan RJ, Shirreffs SM. Dehydration and rehydration in competative sport. Scand J Med Sci Sports. 2010;20 Suppl 3:40-7. doi:10.1111/j.1600-0838.2010.01207.x. PMID:21029189.
- Périard JD, Racinais S, Knez WL, Herrera CP, Christian RJ, Girard O. Coping with heat stress during match-play tennis: Does an individualised hydration regimen enhance performance and recovery? Br J Sports Med. 2014;48 Suppl 1:i64-70. doi:10.1136/bjsports-2013-093242. PMID:24668383.
- Montain SJ, Latzka WA, Sawka MN. Control of thermoregulatory sweating is altered by hydration level and exercise intensity. J Appl Physiol. 1995;79:1434-9. PMID:8593998.
- González-Alonso J, Mora-Rodríguez R, Below PR, Coyle EF. Dehydration reduces cardiac output and increases systemic and cutaneous vascular resistance during exercise. J Appl Physiol. 1995;79:1487-96. PMID:8594004.
- Dill DB, Edwards HT, Bauer PS, Levenson EJ. Physical performance in relation to external temperature. Arbeitsphysiologie. 1931;4(6):508-18.
- Périard JD, Cramer MN, Chapman PG, Caillaud C, Thompson MW. Cardiovascular strain impairs prolonged self-paced exercise in the heat. Exp Physiol. 2011;96:134-44. doi:10.1113/expphysiol.2010.054213. PMID:20851861.
- Périard JD, Racinais S. Self-paced exercise in hot and cool conditions is associated with the maintenance of %V̇O2peak within a narrow range. J Appl Physiol. 2015;118:1258-65. doi:10.1152/japplphysiol.00084.2015. PMID:25814635.
- Leon LR, Bouchama A. Heat stroke. Compr Physiol. 2015;5:611-47. doi:10.1002/cphy.c140017. PMID:25880507.
- Racinais S, Alonso JM, Coutts AJ, Flouris AD, Girard O, González-Alonso J, Hausswirth C, Jay O, Lee JK, Mitchell N, et al. Consensus recommendations on training and competing in the heat. Scand J Med Sci Sports. 2015;25:6-19. doi:10.1111/sms.12467. PMID:25943653.
- Asmussen E, Bøje O. Body temperature and capacity for work. Acta Physiol. 1945;10:1-22. doi:10.1111/j.1748-1716.1945.tb00287.x.
- Faulkner SH, Ferguson RA, Gerrett N, Hupperets M, Hodder SG, Havenith G. Reducing muscle temperature drop after warm-up improves sprint cycling performance. Med Sci Sports Exerc. 2013;45:359-65. doi:10.1249/MSS.0b013e31826fba7f. PMID:22935735.
- Clarke RS, Hellon RF, Lind AR. The duration of sustained contractions of the human forearm at different muscle temperatures. J Physiol. 1958;143:454-73. doi:10.1113/jphysiol.1958.sp006071. PMID:13588567.
- Chiesa ST, Trangmar SJ, Kalsi KK, Rakobowchuk M, Banker DS, Lotlikar MD, Ali L, González-Alonso J. Local temperature-sensitive mechanisms are important mediators of limb tissue hyperemia in the heat-stressed human at rest and during small muscle mass exercise. Am J Physiol Heart Circ Physiol. 2015;309:H369-80. doi:10.1152/ajpheart.00078.2015. PMID:25934093.
- Starkie RL, Hargreaves M, Lambert DL, Proietto J, Febbraio MA. Effect of temperature on muscle metabolism during submaximal exercise in humans. Exp Physiol. 1999;84:775-84. doi:10.1111/j.1469-445X.1999.01815.x. PMID:10481233.
- Barcroft J, King WO. The effect of temperature on the dissociation curve of blood. J Physiol. 1909;39:374-84. doi:10.1113/jphysiol.1909.sp001345. PMID:16992990.
- Gray SR, De Vito G, Nimmo MA, Farina D, Ferguson RA. Skeletal muscle ATP turnover and muscle fiber conduction velocity are elevated at higher muscle temperatures during maximal power output development in humans. Am J Physiol Regul Integr Comp Physiol. 2006;290:R376-82. doi:10.1152/ajpregu.00291.2005. PMID:16166210.
- Ferguson RA, Ball D, Sargeant AJ. Effect of muscle temperature on rate of oxygen uptake during exercise in humans at different contraction frequencies. J Exp Biol. 2002;205:981-7. PMID:11916993.
- Gerbino A, Ward SA, Whipp BJ. Effects of prior exercise on pulmonary gas-exchange kinetics during high-intensity exercise in humans. J Appl Physiol. 1996;80:99-107. PMID:8847338.
- Racinais S, Oksa J. Temperature and neuromuscular function. Scand J Med Sci Sports. 2010;20 Suppl 3:1-18. doi:10.1111/j.1600-0838.2010.01204.x. PMID:21029186.
- Oksa J, Rintamäki H, Mäkinen T, Martikkala V, Rusko H. EMG-activity and muscular performance of lower leg during stretch-shortening cycle after cooling. Acta Physiol Scand. 1996;157:1-8. doi:10.1046/j.1365-201X.1996.452172000.x. PMID:8735656.
- Asmussen E, Bonde-Petersen F, Jorgensen K. Mechano-elastic properties of human muscles at different temperatures. Acta Physiol Scand. 1976;96:83-93. doi:10.1111/j.1748-1716.1976.tb10173.x. PMID:1251749.
- Hartshorne DJ, Barns EM, Parker L, Fuchs F. The effect of temperature on actomyosin. Biochim Biophys Acta. 1972;267:190-202. doi:10.1016/0005-2728(72)90150-8. PMID:4259758.
- Close R, Hoh JF. Influence of temperature on isometric contractions of rat skeletal muscles. Nature. 1968;217:1179-80. doi:10.1038/2171179a0. PMID:5643098.
- Ranatunga KW. Temperature-dependence of shortening velocity and rate of isometric tension development in rat skeletal muscle. J Physiol. 1982;329:465-83. doi:10.1113/jphysiol.1982.sp014314. PMID:7143257.
- Bárány M. ATPase activity of myosin correlated with speed of muscle shortening. J Gen Physiol. 1967;50:Suppl:197-218. doi:10.1085/jgp.50.6.197.
- Stein RB, Gordon T, Shriver J. Temperature dependence of mammalian muscle contractions and ATPase activities. Biophys J. 1982;40:97-107. doi:10.1016/S0006-3495(82)84464-0. PMID:6216923.
- Segal SS, Faulkner JA, White TP. Skeletal muscle fatigue in vitro is temperature dependent. J Appl Physiol. 1986;61:660-5. PMID:3745058.
- Stephenson DG, Williams DA. Calcium-activated force responses in fast- and slow-twitch skinned muscle fibres of the rat at different temperatures. J Physiol. 1981;317:281-302. doi:10.1113/jphysiol.1981.sp013825. PMID:7310735.
- Ranatunga KW. The force-velocity relation of rat fast- and slow-twitch muscles examined at different temperatures. J Physiol. 1984;351:517-29. doi:10.1113/jphysiol.1984.sp015260. PMID:6747875.
- Bennett AF. Thermal dependence of muscle function. Am J Physiol. 1984;247:R217-29. PMID:6380314.
- Place N, Yamada T, Zhang S-J, Westerblad H, Bruton JD. High temperature does not alter fatigability in intact mouse skeletal muscle fibres. J Physiol. 2009;587:4717-24. doi:10.1113/jphysiol.2009.176883. PMID:19675072.
- Racinais S, Girard O. Neuromuscular failure is unlikely to explain the early exercise cessation in hot ambient conditions. Psychophysiology. 2012;49:853-65. doi:10.1111/j.1469-8986.2012.01360.x. PMID:22416901.
- Périard JD, Racinais S, Thompson MW. Adjustments in the force-frequency relationship during passive and exercise-induced hyperthermia. Muscle Nerve. 2014;50:822-9. doi:10.1002/mus.24228. PMID:24615660.
- Racinais S, Wilson MG, Périard JD. Passive heat acclimation improves skeletal muscle contractility in humans. Am J Physiol Regul Integr Comp Physiol. 2017;312:R101-7. doi:10.1152/ajpregu.00431.2016. PMID:27903515.
- Buchthal F, Kaiser E, Knappeis GG. Elasticity, viscosity and plasticity in the cross striated muscle fibre. Acta Physiol. 1944;8:16-37. doi:10.1111/j.1748-1716.1944.tb03048.x.
- Wright V, Johns RJ. Quantitative and qualitative analysis of joint stiffness in normal subjects and in patients with connective tissue diseases. Ann Rheum Dis. 1961;20:36-46. doi:10.1136/ard.20.1.36. PMID:13786829.
- Karvonen J. Warming up and its physiological effects. Acta Universitatis Ouluensis. 1978;31.
- Ward MR, Thesleff S. The temperature dependence of action potentials in rat skeletal muscle fibres. Acta Physiol Scand. 1974;91:574-6. doi:10.1111/j.1748-1716.1974.tb05714.x. PMID:4432768.
- Hodgson M, Docherty D, Robbins D. Post-activation potentiation: Underlying physiology and implications for motor performance. Sports Med. 2005;35:585-95. doi:10.2165/00007256-200535070-00004. PMID:16026172.
- Tillin NA, Bishop D. Factors modulating post-activation potentiation and its effect on performance of subsequent explosive activities. Sports Med. 2009;39:147-66. doi:10.2165/00007256-200939020-00004. PMID:19203135.
- Güllich A, Schmidtbleicher D. MVC-induced short-term potentiation of explosive force. New Studies Athletics. 1996;11:67-81.
- Moore RL, Stull JT. Myosin light chain phosphorylation in fast and slow skeletal muscles in situ. Am J Physiol. 1984;247:C462-71. PMID:6548609.
- Smith JC, Fry AC. Effects of a ten-second maximum voluntary contraction on regulatory myosin light-chain phosphorylation and dynamic performance measures. J Strength Cond Res. 2007;21:73-6. doi:10.1519/00124278-200702000-00014. PMID:17313284.
- Racinais S, Cresswell AG. Temperature affects maximum H-reflex amplitude but not homosynaptic postactivation depression. Physiol Rep. 2013;1:e00019. doi:10.1002/phy2.19. PMID:24303108.
- Trimble MH, Harp SS. Postexercise potentiation of the H-reflex in humans. Med Sci Sports Exerc. 1998;30:933-41. PMID:9624654.
- Rassier DE, MacIntosh BR. Coexistence of potentiation and fatigue in skeletal muscle. Braz J Med Biol Res. 2000;33:499-508. doi:10.1590/S0100-879X2000000500003. PMID:10775880.
- Behm DG, Button DC, Barbour G, Butt JC, Young WB. Conflicting effects of fatigue and potentiation on voluntary force. J Strength Cond Res. 2004;18:365-72. PMID:15141999.
- Davidoff RA. Skeletal muscle tone and the misunderstood stretch reflex. Neurology. 1992;42:951-63. doi:10.1212/WNL.42.5.951. PMID:1579249.
- Proske U, Morgan DL, Gregory JE. Thixotropy in skeletal muscle and in muscle spindles: A review. Prog Neurobiol. 1993;41:705-21. doi:10.1016/0301-0082(93)90032-N. PMID:8140258.
- Lakie M, Robson LG. Thixotropic changes in human muscle stiffness and the effects of fatigue. Q J Exp Physiol. 1988;73:487-500. doi:10.1113/expphysiol.1988.sp003169. PMID:3174910.
- Paintal AS. Effects of temperature on conduction in single vagal and saphenous myelinated nerve fibres of the cat. J Physiol. 1965;180:20-49. PMID:5860224.
- Bigland-Ritchie B, Donovan EF, Roussos CS. Conduction velocity and EMG power spectrum changes in fatigue of sustained maximal efforts. J Appl Physiol. 1981;51:1300-5. PMID:7298467.
- Oksa J, Rintamäki H, Mäkinen T, Hassi J, Rusko H. Cooling-induced changes in muscular performance and EMG activity of agonist and antagonist muscles. Aviat Space Environ Med. 1995;66:26-31. PMID:7695547.
- Bawa P, Matthews P, Mekjavic IB. Electromyographic activity during shivering of muscles acting at the human elbow. J Therm Biol. 1987;12:1-4. doi:10.1016/0306-4565(87)90014-3.
- Racinais S, Gaoua N, Grantham J. Hyperthermia impairs short-term memory and peripheral motor drive transmission. J Physiol. 2008;586:4751-62. doi:10.1113/jphysiol.2008.157420. PMID:18703579.
- Rutkove SB, Kothari MJ, Shefner JM. Nerve, muscle, and neuromuscular junction electrophysiology at high temperature. Muscle Nerve. 1997;20:431-6. doi:10.1002/(SICI)1097-4598(199704)20:4%3c431::AID-MUS5%3e3.0.CO;2-B. PMID:9121500.
- Bolton CF, Sawa GM, Carter K. The effects of temperature on human compound action potentials. J Neurol Neurosurg Psychiatry. 1981;44:407-13. doi:10.1136/jnnp.44.5.407. PMID:7264687.
- Denys EH. AAEM minimonograph #14: The influence of temperature in clinical neurophysiology. Muscle Nerve. 1991;14:795-811. doi:10.1002/mus.880140902. PMID:1656252.
- Bishop D. Warm up I: Potential mechanisms and the effects of passive warm up on exercise performance. Sports Med. 2003;33:439-54. doi:10.2165/00007256-200333060-00005. PMID:12744717.
- Malareki I. Investigation of physiological justification of so-called warming up. Acta Physiol Pol. 1954;5:543-6. PMID:14376058.
- Blomstrand E, Bergh U, Essén-Gustavsson B, Ekblom B. Influence of low muscle temperature on muscle metabolism during intense dynamic exercise. Acta Physiol Scand. 1984;120:229-36. doi:10.1111/j.1748-1716.1984.tb00128.x. PMID:6711338.
- McCutcheon LJ, Geor RJ, Hinchcliff KW. Effects of prior exercise on muscle metabolism during sprint exercise in horses. J Appl Physiol. 1999;87:1914-22. PMID:10562637.
- Theorell H. The effect of temperature on myoglobin. Biochem Z. 1934;73:268.
- Febbraio MA, Snow RJ, Stathis CG, Hargreaves M, Carey MF. Effect of heat stress on muscle energy metabolism during exercise. J Appl Physiol. 1994;77:2827-31. PMID:7896628.
- Barnard RJ, Gardner GW, Diaco NV, MacAlpin RN, Kattus AA. Cardiovascular responses to sudden strenuous exercise–heart rate, blood pressure, and ECG. J Appl Physiol. 1973;34:833-7. PMID:4711594.
- Barnard RJ, MacAlpin R, Kattus AA, Buckberg GD. Ischemic response to sudden strenuous exercise in healthy men. Circulation. 1973;48:936-42. doi:10.1161/01.CIR.48.5.936. PMID:4751958.
- Ekstrand J, Gillquist J, Liljedahl SO. Prevention of soccer injuries. Supervision by doctor and physiotherapist. Am J Sports Med. 1983;11:116-20. doi:10.1177/036354658301100302. PMID:6346912.
- Hadala M, Barrios C. Different strategies for sports injury prevention in an America's Cup yachting crew. Med Sci Sports Exerc. 2009;41:1587-96. doi:10.1249/MSS.0b013e31819c0de7. PMID:19568201.
- Whelan KM, Gass EM, Moran CC. Warm-up: Efficacy of a program designed for downhill skiing. Aust J Physiother. 1999;45:279-88. doi:10.1016/S0004-9514(14)60357-3. PMID:11676777.
- Lehmann JF, Masock AJ, Warren CG, Koblanski JN. Effect of therapeutic temperatures on tendon extensibility. Arch Phys Med Rehabil. 1970;51:481-7.
- Sapega AA, Quedenfeld TC, Moyer RA, Butler RA. Biophysical factors in range-of-motion exercise. Phys Sportsmed. 1981;9:57-65. doi:10.1080/00913847.1981.11711229. PMID:27452706.
- Safran MR, Garrett WE, Seaber AV, Glisson RR, Ribbeck BM. The role of warmup in muscular injury prevention. Am J Sports Med. 1988;16:123-9. doi:10.1177/036354658801600206. PMID:3377095.
- Behm DG, Blazevich AJ, Kay AD, McHugh M. Acute effects of muscle stretching on physical performance, range of motion, and injury incidence in healthy active individuals: A systematic review. Appl Physiol Nutr Metab. 2016;41:1-11. doi:10.1139/apnm-2015-0235. PMID:26642915.
- Taylor DC, Brooks DE, Ryan JB. Viscoelastic characteristics of muscle: Passive stretching versus muscular contractions. Med Sci Sports Exerc. 1997;29:1619-24. doi:10.1097/00005768-199712000-00011. PMID:9432095.
- Law R, Herbert RD. Warm-up reduces delayed-onset muscle soreness but cool-down does not: A randomised controlled trial. Aust J Physiothe. 2007;53:91-5. doi:10.1016/S0004-9514(07)70041-7.
- Fitts RH. Cellular mechanisms of muscle fatigue. Physiol Rev. 1994;74:49-94. PMID:8295935.
- Allen DG, Lamb GD, Westerblad H. Skeletal muscle fatigue: Cellular mechanisms. Physiol Rev. 2008;88:287-332. doi:10.1152/physrev.00015.2007. PMID:18195089.
- Nybo L, Nielsen B. Hyperthermia and central fatigue during prolonged exercise in humans. J Appl Physiol. 2001;91:1055-60. PMID:11509498.
- Périard JD, Caillaud C, Thompson MW. Central and peripheral fatigue during passive and exercise-induced hyperthermia. Med Sci Sports Exerc. 2011;43:1657-65. doi:10.1249/MSS.0b013e3182148a9a. PMID:21364487.
- Périard JD, Christian RJ, Knez WL, Racinais S. Voluntary muscle and motor cortical activation during progressive exercise and passively induced hyperthermia. Exp Physiol. 2014;99:136-48. doi:10.1113/expphysiol.2013.074583. PMID:24036591.
- González-Alonso J, Teller C, Andersen SL, Jensen FB, Hyldig T, Nielsen B. Influence of body temperature on the development of fatigue during prolonged exercise in the heat. J Appl Physiol. 1999;86:1032-9. PMID:10066720.
- Cheuvront SN, Kenefick RW, Montain SJ, Sawka MN. Mechanisms of aerobic performance impairment with heat stress and dehydration. J Appl Physiol. 2010;109:1989-95. doi:10.1152/japplphysiol.00367.2010. PMID:20689090.
- Périard JD, Cramer MN, Chapman PG, Caillaud C, Thompson MW. Cardiovascular strain impairs prolonged self-paced exercise in the heat. Exp Physiol. 2011;96:134-44. doi:10.1113/expphysiol.2010.054213. PMID:20851861.
- Périard JD, Racinais S. Self-paced exercise in hot and cool conditions is associated with the maintenance of %V̇O2peak within a narrow range. J Appl Physiol. 2015;118:1258-65. doi:10.1152/japplphysiol.00084.2015. PMID:25814635.
- Périard JD, Racinais S. Performance and pacing during cycle exercise in hyperthermic and hypoxic conditions. Med Sci Sports Exerc. 2016;48:845-53. doi:10.1249/MSS.0000000000000839. PMID:26656777.
- Morrison S, Sleivert GG, Cheung SS. Passive hyperthermia reduces voluntary activation and isometric force production. Eur J Appl Physiol. 2004;91:729-36. doi:10.1007/s00421-004-1063-z. PMID:15015001.
- Davies CT, Young K. Effect of temperature on the contractile properties and muscle power of triceps surae in humans. J Appl Physiol. 1983;55:191-5. PMID:6885568.
- Brück K, Olschewski H. Body temperature related factors diminishing the drive to exercise. Can J Physiol Pharmacol. 1987;65:1274-80. doi:10.1139/y87-203. PMID:3621076.
- Nielsen B, Savard G, Richter EA, Hargreaves M, Saltin B. Muscle blood flow and muscle metabolism during exercise and heat stress. J Appl Physiol. 1990;69:1040-6. PMID:2246151.
- Nielsen B, Hales JR, Strange S, Christensen NJ, Warberg J, Saltin B. Human circulatory and thermoregulatory adaptations with heat acclimation and exercise in a hot, dry environment. J Physiol. 1993;460:467-85. doi:10.1113/jphysiol.1993.sp019482. PMID:8487204.
- Thomas MM, Cheung SS, Elder GC, Sleivert GG. Voluntary muscle activation is impaired by core temperature rather than local muscle temperature. J Appl Physiol. 2006;100:1361-9. doi:10.1152/japplphysiol.00945.2005. PMID:16339343.
- Nielsen B, Nybo L. Cerebral changes during exercise in the heat. Sports Med. 2003;33:1-11. doi:10.2165/00007256-200333010-00001. PMID:12477374.
- Nybo L, Rasmussen P, Sawka MN. Performance in the heat-physiological factors of importance for hyperthermia-induced fatigue. Compr Physiol. 2014;4:657-89. doi:10.1002/cphy.c130012. PMID:24715563.
- Nybo L, González-Alonso J. Critical core temperature: A hypothesis too simplistic to explain hyperthermia-induced fatigue. Scand J Med Sci Sports. 2015;25:4-5. doi:10.1111/sms.12444. PMID:25943652.
- Lloyd A, Hodder S, Havenith G. The interaction between peripheral and central fatigue at different muscle temperatures during sustained isometric contractions. Am J Physiol Regul Integr Comp Physiol. 2015;309:R410-20. doi:10.1152/ajpregu.00061.2015. PMID:26041110.
- Périard JD, Cramer MN, Chapman PG, Caillaud C, Thompson MW. Neuromuscular function following prolonged intense self-paced exercise in hot climatic conditions. Eur J Appl Physiol. 2011;111:1561-9. doi:10.1007/s00421-010-1781-3. PMID:21188412.
- Todd G, Butler JE, Taylor JL, Gandevia SC. Hyperthermia: A failure of the motor cortex and the muscle. J Physiol. 2005;563:621-31. doi:10.1113/jphysiol.2004.077115. PMID:15613373.
- Davies CT, Mecrow IK, White MJ. Contractile properties of the human triceps surae with some observations on the effects of temperature and exercise. Eur J Appl Physiol Occup Physiol. 1982;49:255-69. doi:10.1007/BF02334074. PMID:6889502.
- Ranatunga KW, Sharpe B, Turnbull B. Contractions of a human skeletal muscle at different temperatures. J Physiol. 1987;390:383-95. doi:10.1113/jphysiol.1987.sp016707. PMID:3443940.
- Racinais S, Wilson MG, Gaoua N, Periard J. Heat acclimation has a protective effect on the central but not peripheral nervous system. J Appl Physiol. 2017. doi:10.1152/japplphysiol.00430.2017. PMID:28684590.
- Febbraio MA, Snow RJ, Hargreaves M, Stathis CG, Martin IK, Carey MF. Muscle metabolism during exercise and heat stress in trained men: Effect of acclimation. J Appl Physiol. 1994;76:589-97. PMID:8175568.
- Febbraio MA, Carey MF, Snow RJ, Stathis CG, Hargreaves M. Influence of elevated muscle temperature on metabolism during intense, dynamic exercise. Am J Physiol. 1996;271:R1251-5. PMID:8945960.
- Dolny DG, Lemon PW. Effect of ambient temperature on protein breakdown during prolonged exercise. J Appl Physiol. 1988;64:550-5. PMID:3372413.
- Sahlin K, Alvestrand A, Brandt R, Hultman E. Intracellular pH and bicarbonate concentration in human muscle during recovery from exercise. J Appl Physiol 1978;45:474-80. PMID:29868.
- Metzger JM, Greaser ML, Moss RL. Variations in cross-bridge attachment rate and tension with phosphorylation of myosin in mammalian skinned skeletal muscle fibers. Implications for twitch potentiation in intact muscle. J Gen Physiol. 1989;93:855-83. doi:10.1085/jgp.93.5.855. PMID:2661721.
- Schertzer JD, Green HJ, Tupling AR. Thermal instability of rat muscle sarcoplasmic reticulum Ca(2+)-ATPase function. Am J Physiol Endocrinol Metab. 2002;283:E722-8. doi:10.1152/ajpendo.00204.2002. PMID:12217889.
- Barcroft H, Millen JL. The blood flow through muscle during sustained contraction. J Physiol. 1939;97:17-31. doi:10.1113/jphysiol.1939.sp003789. PMID:16995147.
- Edwards RH, Hill DK, McDonnell M. Myothermal and intramuscular pressure measurements during isometric contractions of the human quadriceps muscle. J Physiol. 1972;224:58P-9P. PMID:5071409.
- Barnes WS. The relationship between maximum isometric strength and intramuscular circulatory occlusion. TERG. 1980;23:351-7. doi:10.1080/00140138008924748.
- Sejersted OM, Hargens AR, Kardel KR, Blom P, Jensen O, Hermansen L. Intramuscular fluid pressure during isometric contraction of human skeletal muscle. J Appl Physiol Respir Environ Exerc Physiol. 1984;56:287-95. PMID:6706739.
- Kalia M, Mei SS, Kao FF. Central projections from ergoreceptors (C fibers) in muscle involved in cardiopulmonary responses to static exercise. Circ Res. 1981;48:I48-62. PMID:7226464.
- Kniffeki KD, Mense S, Schmidt RF. Muscle receptors with fine afferent fibers which may evoke circulatory reflexes. Circ Res. 1981;48:I25-31. PMID:7014023.
- Seals D, Victor R. Regulation of muscle sympathetic nerve activity during exercise in humans. Exerc Sport Sci Rev. 1991;19:313-49. doi:10.1249/00003677-199101000-00009. PMID:1936089.
- Bigland-Ritchie BR, Dawson NJ, Johansson RS, Lippold OC. Reflex origin for the slowing of motoneurone firing rates in fatigue of human voluntary contractions. J Physiol. 1986;379:451-9. doi:10.1113/jphysiol.1986.sp016263. PMID:3560001.
- Woods JJ, Furbush F, Bigland-Ritchie B. Evidence for a fatigue-induced reflex inhibition of motoneuron firing rates. J Neurophysiol. 1987;58:125-37. PMID:3039078.
- Bigland-Ritchie B, Jones DA, Hosking GP, Edwards RH. Central and peripheral fatigue in sustained maximum voluntary contractions of human quadriceps muscle. Clin Sci Mol Med. 1978;54:609-14. PMID:657729.
- Hockey GR. Compensatory control in the regulation of human performance under stress and high workload; a cognitive-energetical framework. Biol Psychol. 1997;45:73-93. doi:10.1016/S0301-0511(96)05223-4. PMID:9083645.
- Cabanac M. Sensory pleasure optimizes muscular work. Clin Invest Med. 2006;29:110-6. PMID:16737087.
- Boksem MAS, Tops M. Mental fatigue: Costs and benefits. Brain Res Rev. 2008;59:125-39. doi:10.1016/j.brainresrev.2008.07.001. PMID:18652844.
- Enoka RM, Stuart DG. Neurobiology of muscle fatigue. J Appl Physiol. 1992;72:1631-48. PMID:1601767.
- Otani H, Kaya M, Tamaki A, Watson P. Separate and combined effects of exposure to heat stress and mental fatigue on endurance exercise capacity in the heat. Eur J Appl Physiol. 2017;117:119-29. doi:10.1007/s00421-016-3504-x. PMID:27864637.
- Van Cutsem J, De Pauw K, Buyse L, Marcora S, Meeusen R, Roelands B. Effects of mental fatigue on endurance performance in the heat. Med Sci Sports Exerc. 2017;49:1677-87. doi:10.1249/MSS.0000000000001263. PMID:28282326.
- Cramer MN, Thompson MW, Périard JD. Thermal and cardiovascular strain mitigate the potential benefit of carbohydrate mouth rinse during self-paced exercise in the heat. Front Physio. 2015;6:354. doi:10.3389/fphys.2015.00354.
- Flouris AD, Schlader ZJ. Human behavioral thermoregulation during exercise in the heat. Scand J Med Sci Sports. 2015;25 Suppl 1:52-64. doi:10.1111/sms.12349. PMID:25943656.
- Ely BR, Ely MR, Cheuvront SN, Kenefick RW, Degroot DW, Montain SJ. Evidence against a 40°C core temperature threshold for fatigue in humans. J Appl Physiol. 2009;107:1519-25. doi:10.1152/japplphysiol.00577.2009. PMID:19713430.
- Schmit C, Hausswirth C, Le Meur Y, Duffield R. Cognitive functioning and heat strain: Performance responses and protective strategies. Sports Med. 2017;47:1289-302. doi:10.1007/s40279-016-0657-z. PMID:27988874.
- Cian C, Barraud PA, Melin B, Raphel C. Effects of fluid ingestion on cognitive function after heat stress or exercise-induced dehydration. Int J Psychophysiol. 2001;42:243-51. doi:10.1016/S0167-8760(01)00142-8. PMID:11812391.
- Hocking C, Silberstein RB, Lau WM, Stough C, Roberts W. Evaluation of cognitive performance in the heat by functional brain imaging and psychometric testing. Comp Biochem Physiol Part A Mol Integr Physiol. 2001;128:719-34. doi:10.1016/S1095-6433(01)00278-1.
- Pilcher JJ, Nadler E, Busch C. Effects of hot and cold temperature exposure on performance: A meta-analytic review. TERG. 2002;45:682-98. doi:10.1080/00140130210158419.
- Hancock PA, Vasmatzidis I. Effects of heat stress on cognitive performance: The current state of knowledge. Int J Hyperthermia. 2003;19:355-72. doi:10.1080/0265673021000054630. PMID:12745975.
- Gaoua N, Racinais S, Grantham J, Massioui El F. Alterations in cognitive performance during passive hyperthermia are task dependent. Int J Hyperthermia. 2011;27:1-9. doi:10.3109/02656736.2010.516305. PMID:21070137.
- Gaoua N, Grantham J, Massioui El F, Girard O, Racinais S. Cognitive decrements do not follow neuromuscular alterations during passive heat exposure. Int J Hyperthermia. 2011;27:10-9. doi:10.3109/02656736.2010.519371. PMID:21070138.
- Gaoua N, Grantham J, Racinais S, Massioui El F. Sensory displeasure reduces complex cognitive performance in the heat. J Environ Psycho. 2012;32:158-63. doi:10.1016/j.jenvp.2012.01.002.
- Cabanac M. Physiological role of pleasure. Science. 1971;173:1103-7. doi:10.1126/science.173.4002.1103. PMID:5098954.
- Kellogg DL, Johnson JM, Kosiba WA. Control of internal temperature threshold for active cutaneous vasodilation by dynamic exercise. J Appl Physiol. 1991;71:2476-82. PMID:1778949.
- Smolander J, Saalo J, Korhonen O. Effect of work load on cutaneous vascular response to exercise. J Appl Physiol. 1991;71:1614-9. PMID:1757390.
- Johnson JM. Exercise and the cutaneous circulation. Exerc Sport Sci Rev. 1992;20:59-97. PMID:1623893.
- Kenney WL, Johnson JM. Control of skin blood flow during exercise. Med Sci Sports Exerc. 1992;24:303-12. doi:10.1249/00005768-199203000-00005. PMID:1549024.
- Johnson JM, Rowell LB, Brengelmann GL. Modification of the skin blood flow-body temperature relationship by upright exercise. J Appl Physiol. 1974;37:880-6. PMID:4436219.
- Brengelmann GL, Johnson JM, Hermansen L, Rowell LB. Altered control of skin blood flow during exercise at high internal temperatures. J Appl Physiol. 1977;43:790-4. PMID:591471.
- Rowell LB. Human cardiovascular adjustments to exercise and thermal stress. Physiol Rev. 1974;54:75-159. PMID:4587247.
- Johnson JM, Rowell LB. Forearm skin and muscle vascular responses to prolonged leg exercise in man. J Appl Physiol. 1975;39:920-4. PMID:1213973.
- Johnson JM, Park MK. Reflex control of skin blood flow by skin temperature: Role of core temperature. J Appl Physiol. 1979;47:1188-93. PMID:536288.
- Kenefick RW, Cheuvront SN, Palombo LJ, Ely BR, Sawka MN. Skin temperature modifies the impact of hypohydration on aerobic performance. J Appl Physiol. 2010;109:79-86. doi:10.1152/japplphysiol.00135.2010. PMID:20378704.
- Johnson JM. Regulation of skin circulation during prolonged exercise. Ann N Y Acad Sci. 1977;301:195-212. doi:10.1111/j.1749-6632.1977.tb38199.x. PMID:270916.
- Johnson JM. Nonthermoregulatory control of human skin blood flow. J Appl Physiol. 1986;61:1613-22. PMID:3536836.
- Astrand PO, Cuddy TE, Saltin B, Stenberg J. Cardiac output during submaximal and maximal work. J Appl Physiol. 1964;19:268-74. PMID:14155294.
- Pearson J, Low DA, Stöhr E, Kalsi K, Ali L, Barker H, González-Alonso J. Hemodynamic responses to heat stress in the resting and exercising human leg: Insight into the effect of temperature on skeletal muscle blood flow. Am J Physiol Regul Integr Comp Physiol. 2011;300:R663-73. doi:10.1152/ajpregu.00662.2010. PMID:21178127.
- González-Alonso J, Calbet JAL, Boushel R, Helge JW, Søndergaard H, Munch-Andersen T, van Hall G, Mortensen SP, Secher NH. Blood temperature and perfusion to exercising and non-exercising human limbs. Exp Physiol. 2015;100:1118-31. doi:10.1113/EP085383. PMID:26268717.
- Saltin B, Rådegran G, Koskolou MD. Skeletal muscle blood flow in humans and its regulation during exercise. Acta Physiol Scand. 1998;162:421-36. doi:10.1046/j.1365-201X.1998.0293e.x.
- Rådegran G, Saltin B. Muscle blood flow at onset of dynamic exercise in humans. Am J Physiol. 1998;274:H314-22. PMID:9458882.
- Savard G, Kiens B, Saltin B. Limb blood flow in prolonged exercise: Magnitude and implication for cardiovascular control during muscular work in man. Can J Appl Sports Sci. 1987;12:S89-S101.
- Montain SJ, Coyle EF. Influence of graded dehydration on hyperthermia and cardiovascular drift during exercise. J Appl Physiol. 1992;73:1340-50. PMID:1447078.
- Crandall CG, González-Alonso J. Cardiovascular function in the heat-stressed human. Acta Physiol (Oxf). 2010;199:407-23. doi:10.1111/j.1748-1716.2010.02119.x. PMID:20345414.
- Kenney WL, Stanhewicz AE, Bruning RS, Alexander LM. Blood pressure regulation III: What happens when one system must serve two masters: Temperature and pressure regulation? Eur J Appl Physiol. 2014;114:467-79. doi:10.1007/s00421-013-2652-5. PMID:23636697.
- Fritzsche RG, Switzer TW, Hodgkinson BJ, Coyle EF. Stroke volume decline during prolonged exercise is influenced by the increase in heart rate. J Appl Physiol 1999;86:799-805. PMID:10066688.
- Coyle EF, González-Alonso J. Cardiovascular drift during prolonged exercise: New perspectives. Exerc Sport Sci Rev. 2001;29:88-92. doi:10.1097/00003677-200104000-00009. PMID:11337829.
- Trinity JD, Pahnke MD, Lee JF, Coyle EF. Interaction of hyperthermia and heart rate on stroke volume during prolonged exercise. J Appl Physiol. 2010;109:745-51. doi:10.1152/japplphysiol.00377.2010. PMID:20595543.
- Wilson TE, Crandall CG. Effect of thermal stress on cardiac function. Exerc Sport Sci Rev. 2011;39:12-7. doi:10.1097/JES.0b013e318201eed6. PMID:21088607.
- Bassett DR, Howley ET. Limiting factors for maximum oxygen uptake and determinants of endurance performance. Med Sci Sports Exerc. 2000;32:70-84. doi:10.1097/00005768-200001000-00012. PMID:10647532.
- di Prampero PE. Factors limiting maximal performance in humans. Eur J Appl Physiol. 2003;90:420-9. doi:10.1007/s00421-003-0926-z. PMID:12910345.
- Sawka MN, Young AJ, Cadarette BS, Levine L, Pandolf KB. Influence of heat stress and acclimation on maximal aerobic power. Eur J Appl Physiol Occup Physiol. 1985;53:294-8. doi:10.1007/BF00422841. PMID:4039255.
- Arngrïmsson SA, Stewart DJ, Borrani F, Skinner KA, Cureton KJ. Relation of heart rate to percent VO2 peak during submaximal exercise in the heat. J Appl Physiol. 2003;94:1162-8. doi:10.1152/japplphysiol.00508.2002. PMID:12391114.
- Wingo JE, Lafrenz AJ, Ganio MS, Edwards GL, Cureton KJ. Cardiovascular drift is related to reduced maximal oxygen uptake during heat stress. Med Sci Sports Exerc. 2005;37:248-55. doi:10.1249/01.MSS.0000152731.33450.95. PMID:15692320.
- Robertson RJ. Central signals of perceived exertion during dynamic exercise. Med Sci Sports Exerc. 1982;14:390-6. doi:10.1249/00005768-198205000-00014. PMID:7154895.
- González-Alonso J, Calbet JAL. Reductions in systemic and skeletal muscle blood flow and oxygen delivery limit maximal aerobic capacity in humans. Circulation. 2003;107:824-30. doi:10.1161/01.CIR.0000049746.29175.3F. PMID:12591751.
- Mortensen SP, Dawson EA, Yoshiga CC, Dalsgaard MK, Damsgaard R, Secher NH, González-Alonso J. Limitations to systemic and locomotor limb muscle oxygen delivery and uptake during maximal exercise in humans. J Physiol. 2005;566:273-85. doi:10.1113/jphysiol.2005.086025. PMID:15860533.
- Knight DR, Poole DC, Schaffartzik W, Guy HJ, Prediletto R, Hogan MC, Wagner PD. Relationship between body and leg VO2 during maximal cycle ergometry. J Appl Physiol. 1992;73:1114-21. PMID:1400024.
- Rosenmeier JB, Hansen J, González-Alonso J. Circulating ATP-induced vasodilatation overrides sympathetic vasoconstrictor activity in human skeletal muscle. J Physiol. 2004;558:351-65. doi:10.1113/jphysiol.2004.063107. PMID:15155791.
- Mortensen SP, Damsgaard R, Dawson EA, Secher NH, González-Alonso J. Restrictions in systemic and locomotor skeletal muscle perfusion, oxygen supply and VO2 during high-intensity whole-body exercise in humans. J Physiol. 2008;586:2621-35. doi:10.1113/jphysiol.2007.149401. PMID:18372307.
- Saltin B, Stenberg J. Circulatory response to prolonged severe exercise. J Appl Physiol. 1964;19:833-8. PMID:14207729.
- Ekblom B, Hermansen L. Cardiac output in athletes. J Appl Physiol. 1968;25:619-25. PMID:4879852.
- Périard JD, Racinais S. Heat stress exacerbates the reduction in middle cerebral artery blood velocity during prolonged self-paced exercise. Scand J Med Sci Sports. 2015;25:135-44. doi:10.1111/sms.12379.
- Périard JD, Racinais S. Performance and pacing during cycle exercise in hyperthermic and hypoxic conditions. Med Sci Sports Exerc. 2016;48:845-53. doi:10.1249/MSS.0000000000000839. PMID:26656777.
- Nybo L, Nielsen B. Middle cerebral artery blood velocity is reduced with hyperthermia during prolonged exercise in humans. J Physiol. 2001;534:279-86. doi:10.1111/j.1469-7793.2001.t01-1-00279.x. PMID:11433008.
- Nybo L, Møller K, Volianitis S, Nielsen B, Secher NH. Effects of hyperthermia on cerebral blood flow and metabolism during prolonged exercise in humans. J Appl Physiol. 2002;93:58-64. doi:10.1152/japplphysiol.00049.2002. PMID:12070186.
- Rasmussen P, Nybo L, Volianitis S, Møller K, Secher NH, Gjedde A. Cerebral oxygenation is reduced during hyperthermic exercise in humans. Acta Physiol (Oxf). 2010;199:63-70. doi:10.1111/j.1748-1716.2010.02084.x. PMID:20102344.
- Nybo L, Rasmussen P. Inadequate cerebral oxygen delivery and central fatigue during strenuous exercise. Exerc Sport Sci Rev. 2007;35:110-8. doi:10.1097/jes.0b013e3180a031ec. PMID:17620929.
- Rasmussen P, Dawson EA, Nybo L, van Lieshout JJ, Secher NH, Gjedde A. Capillary-oxygenation-level-dependent near-infrared spectrometry in frontal lobe of humans. J Cereb Blood Flow Metab. 2007;27:1082-93. doi:10.1038/sj.jcbfm.9600416. PMID:17077816.
- González-Alonso J, Dalsgaard MK, Osada T, Volianitis S, Dawson EA, Yoshiga CC, Secher NH. Brain and central haemodynamics and oxygenation during maximal exercise in humans. J Physiol. 2004;557:331-42. doi:10.1113/jphysiol.2004.060574. PMID:15004212.
- Trangmar SJ, Chiesa ST, Stock CG, Kalsi KK, Secher NH, González-Alonso J. Dehydration affects cerebral blood flow but not its metabolic rate for oxygen during maximal exercise in trained humans. J Physiol. 2014;592:3143-60. doi:10.1113/jphysiol.2014.272104. PMID:24835170.
- Sonna LA, Sawka MN, Lilly CM. Exertional heat illness and human gene expression. Prog Brain Res. 2007;162:321-46. doi:10.1016/S0079-6123(06)62016-5. PMID:17645926.
- Périard JD, Racinais S, Sawka MN. Adaptations and mechanisms of human heat acclimation: Applications for competitive athletes and sports. Scand J Med Sci Sports. 2015;25:20-38. doi:10.1111/sms.12408. PMID:25943654.
- Périard JD, Travers GJS, Racinais S, Sawka MN. Cardiovascular adaptations supporting human exercise-heat acclimation. Auton Neurosci. 2016;196:52-62. doi:10.1016/j.autneu.2016.02.002. PMID:26905458.
- Mohr M, Krustrup P, Nybo L, Nielsen JJ, Bangsbo J. Muscle temperature and sprint performance during soccer matches–beneficial effect of re-warm-up at half-time. Scand J Med Sci Sports. 2004;14:156-62. doi:10.1111/j.1600-0838.2004.00349.x. PMID:15144355.
- Jeffreys I. Warm-up revisited: The ramp method of optimizing warm-ups. Prof Strength Cond. 2007;6:12-8.
- Kilduff LP, Finn CV, Baker JS, Cook CJ, West DJ. Preconditioning strategies to enhance physical performance on the day of competition. Int J Sports Physiol Perform. 2013;8:677-81. doi:10.1123/ijspp.8.6.677. PMID:23689163.
- Shaarani SR, O'Hare C, Quinn A, Moyna N, Moran R, O'Byrne JM. Effect of prehabilitation on the outcome of anterior cruciate ligament reconstruction. Am J Sports Med. 2013;41:2117-27. doi:10.1177/0363546513493594. PMID:23845398.
- Enoka RM. Neural adaptations with chronic physical activity. J Biomech. 1997;30:447-55. doi:10.1016/S0021-9290(96)00170-4. PMID:9109556.
- Shrier I. Warm-up and stretching in the prevention of muscular injury. Sports Med. 2008;38:879; authorreply 879-80. doi:10.2165/00007256-200838100-00006. PMID:18803439.
- Baxter C, Mc Naughton LR, Sparks A, Norton L, Bentley D. Impact of stretching on the performance and injury risk of long-distance runners. Res Sports Med. 2017;25:78-90. doi:10.1080/15438627.2016.1258640. PMID:27912252.
- Faigenbaum AD, Bellucci M, Bernieri A, Bakker B, Hoorens K. Acute effects of different warm-up protocols on fitness performance in children. J Strength Cond Res. 2005;19:376-81. PMID:15903378.
- Vetter RE. Effects of six warm-up protocols on sprint and jump performance. J Strength Cond Res. 2007;21:819-23. PMID:17685698.
- Bogdanis GC, Donti O, Tsolakis C, Smilios I, Bishop DJ. Intermittent but not continuous static stretching improves subsequent vertical jump performance in flexibility-trained athletes. J Strength Cond Res. 2017. doi:10.1519/JSC.0000000000001870.
- Gelen E. Acute effects of different warm-up methods on sprint, slalom dribbling, and penalty kick performance in soccer players. J Strength Cond Res. 2010;24:950-6. doi:10.1519/JSC.0b013e3181cb703f. PMID:20300033.
- Garrett WE, Califf JC, Bassett FH. Histochemical correlates of hamstring injuries. Am J Sports Med. 1984;12:98-103. doi:10.1177/036354658401200202. PMID:6234816.
- Pearce AJ, Rowe GS, Whyte DG. Neural conduction and excitability following a simple warm up. J Sci Med Sport. 2012;15:164-8. doi:10.1016/j.jsams.2011.09.001. PMID:22018523.
- Sale D. Postactivation potentiation: Role in performance. Br J Sports Med. 2004;38:386-7. doi:10.1136/bjsm.2002.003392. PMID:15273166.
- Sale DG. Postactivation potentiation: Role in human performance. Exerc Sport Sci Rev. 2002;30:138-43. doi:10.1097/00003677-200207000-00008. PMID:12150573.
- Scott DJ, Ditroilo M, Marshall P. Complex training: The effect of exercise selection and training status on post-activation potentiation in rugby league players. J Strength Cond Res. 2016. doi:10.1519/JSC.0000000000001722.
- Gołaś A, Wilk M, Statsny P, Maszczyk A, Pajerska K, Zajac A. Optimizing half squat post activation potential load in squat jump training for eliciting relative maximal power in ski jumpers. J Strength Cond Res. 2017. doi:10.1519/JSC.0000000000001917.
- Fowles JR, Green HJ. Coexistence of potentiation and low-frequency fatigue during voluntary exercise in human skeletal muscle. Can J Physiol Pharmacol. 2003;81:1092-100. doi:10.1139/y03-114. PMID:14719027.
- Gambetta V. Athletic development: The art and science of functional sports conditioning. Champaign (IL): Human Kinetics; 2007.
- Sargeant AJ, Dolan P. Effect of prior exercise on maximal short-term power output in humans. J Appl Physiol. 1987;63:1475-80. PMID:3693183.
- Faisal A, Beavers KR, Robertson AD, Hughson RL. Prior moderate and heavy exercise accelerate oxygen uptake and cardiac output kinetics in endurance athletes. J Appl Physiol. 2009;106:1553-63. doi:10.1152/japplphysiol.91550.2008. PMID:19246653.
- Kilduff LP, West DJ, Williams N, Cook CJ. The influence of passive heat maintenance on lower body power output and repeated sprint performance in professional rugby league players. J Sci Med Sport. 2013;16:482-6. doi:10.1016/j.jsams.2012.11.889. PMID:23246444.
- Raccuglia M, Lloyd A, Filingeri D, Faulkner SH, Hodder S, Havenith G. Post-warm-up muscle temperature maintenance: Blood flow contribution and external heating optimisation. Eur J Appl Physiol 3rd ed. 2016;116:395-404. doi:10.1007/s00421-015-3294-6.
- McGowan CJ, Pyne DB, Thompson KG, Rattray B. Warm-Up strategies for sport and exercise: Mechanisms and applications. Sports Med 5 ed. 2015;45:1523-46. doi:10.1007/s40279-015-0376-x.
- Wilkins EL, Havenith G. External heating garments used post-warm-up improve upper body power and elite sprint swimming performance. Proc IMechE. 2016;231:91-101.
- Gregson WA, Batterham A, Drust B, Cable NT. The influence of pre-warming on the physiological responses to prolonged intermittent exercise. J Sports Sci. 2005;23:455-64. doi:10.1080/02640410410001730214. PMID:16194994.
- Bogdanis GC, Nevill ME, Boobis LH, Lakomy HK. Contribution of phosphocreatine and aerobic metabolism to energy supply during repeated sprint exercise. J Appl Physiol. 1996;80:876-84. PMID:8964751.
- Glaister M. Multiple sprint work: Physiological responses, mechanisms of fatigue and the influence of aerobic fitness. Sports Med. 2005;35:757-77. doi:10.2165/00007256-200535090-00003. PMID:16138786.
- González-Alonso J, Crandall CG, Johnson JM. The cardiovascular challenge of exercising in the heat. J Physiol. 2008;586:45-53. doi:10.1113/jphysiol.2007.142158. PMID:17855754.
- Racinais S, Blonc S, Hue O. Effects of active warm-up and diurnal increase in temperature on muscular power. Med Sci Sports Exerc. 2005;37:2134-9. doi:10.1249/01.mss.0000179099.81706.11. PMID:16331141.
- Booth J, Marino F, Ward JJ. Improved running performance in hot humid conditions following whole body precooling. Med Sci Sports Exerc. 1997;29:943-9. doi:10.1097/00005768-199707000-00014. PMID:9243494.
- Quod MJ, Martin DT, Laursen PB, Gardner AS, Halson SL, Marino FE, Tate MP, Mainwaring DE, Gore CJ, Hahn AG. Practical precooling: Effect on cycling time trial performance in warm conditions. J Sports Sci. 2008;26:1477-87. doi:10.1080/02640410802298268. PMID:18949661.
- Duffield R, Green R, Castle P, Maxwell N. Precooling can prevent the reduction of self-paced exercise intensity in the heat. Med Sci Sports Exerc. 2010;42:577-84. doi:10.1249/MSS.0b013e3181b675da. PMID:19952819.
- Ihsan M, Landers G, Brearley M, Peeling P. Beneficial effects of ice ingestion as a precooling strategy on 40-km cycling time-trial performance. Int J Sports Physiol Perform. 2010;5:140-51. doi:10.1123/ijspp.5.2.140. PMID:20625187.
- Ross MLR, Garvican LA, Jeacocke NA, Laursen PB, Abbiss CR, Martin DT, Burke LM. Novel precooling strategy enhances time trial cycling in the heat. Med Sci Sports Exerc. 2011;43:123-33. doi:10.1249/MSS.0b013e3181e93210. PMID:20508537.
- Siegel R, Maté J, Watson G, Nosaka K, Laursen PB. Pre-cooling with ice slurry ingestion leads to similar run times to exhaustion in the heat as cold water immersion. J Sports Sci. 2012;30:155-65. doi:10.1080/02640414.2011.625968. PMID:22132792.
- Marsh D, Sleivert G. Effect of precooling on high intensity cycling performance. Br J Sports Med. 1999;33:393-7. doi:10.1136/bjsm.33.6.393. PMID:10597847.
- Duffield R, Marino FE. Effects of pre-cooling procedures on intermittent-sprint exercise performance in warm conditions. Eur J Appl Physiol. 2007;100:727-35. doi:10.1007/s00421-007-0468-x. PMID:17476523.
- Castle P, Mackenzie RW, Maxwell N, Webborn ADJ, Watt PW. Heat acclimation improves intermittent sprinting in the heat but additional pre-cooling offers no further ergogenic effect. J Sports Sci. 2011;29:1125-34. doi:10.1080/02640414.2011.583673. PMID:21777052.
- Minett GM, Duffield R, Marino FE, Portus M. Volume-dependent response of precooling for intermittent-sprint exercise in the heat. Med Sci Sports Exerc. 2011;43:1760-9. doi:10.1249/MSS.0b013e318211be3e. PMID:21311362.
- Brade C, Dawson B, Wallman K. Effects of different precooling techniques on repeat sprint ability in team sport athletes. European J Sport Sc. 2014;14 Suppl 1:S84-91. doi:10.1080/17461391.2011.651491.
- Duffield R, Dawson B, Bishop D, Fitzsimons M, Lawrence S. Effect of wearing an ice cooling jacket on repeat sprint performance in warm/humid conditions. Br J Sports Med. 2003;37:164-9. doi:10.1136/bjsm.37.2.164. PMID:12663361.
- Cheung S, Robinson A. The influence of upper-body pre-cooling on repeated sprint performance in moderate ambient temperatures. J Sports Sci. 2004;22:605-12. doi:10.1080/02640410310001655813. PMID:15370490.
- Brade C, Dawson B, Wallman K. Effect of precooling and acclimation on repeat-sprint performance in heat. J Sports Sci. 2013;31:779-86. doi:10.1080/02640414.2012.750006. PMID:23215944.
- Sleivert GG, Cotter JD, Roberts WS, Febbraio MA. The influence of whole-body vs. torso pre-cooling on physiological strain and performance of high-intensity exercise in the heat. Comp Biochem Physiol Part A Mol Integr Physiol. 2001;128:657-66. doi:10.1016/S1095-6433(01)00272-0.
- Castle PC, Macdonald AL, Philp A, Webborn A, Watt PW, Maxwell NS. Precooling leg muscle improves intermittent sprint exercise performance in hot, humid conditions. J Appl Physiol. 2006;100:1377-84. doi:10.1152/japplphysiol.00822.2005. PMID:16339344.
- Marino FE. Methods, advantages, and limitations of body cooling for exercise performance. Br J Sports Med. 2002;36:89-94. doi:10.1136/bjsm.36.2.89. PMID:11916888.
- Quod MJ, Martin DT, Laursen PB. Cooling athletes before competition in the heat: Comparison of techniques and practical considerations. Sports Med. 2006;36:671-82. doi:10.2165/00007256-200636080-00004. PMID:16869709.
- Duffield R. Cooling interventions for the protection and recovery of exercise performance from exercise-induced heat stress. Med Sport Sci. 2008;53:89-103. doi:10.1159/000151552. PMID:19209001.
- Jones PR, Barton C, Morrissey D, Maffulli N, Hemmings S. Pre-cooling for endurance exercise performance in the heat: A systematic review. BMC Med. 2012;10:166. doi:10.1186/1741-7015-10-166. PMID:23249542.
- Siegel R, Laursen PB. Keeping your cool: Possible mechanisms for enhanced exercise performance in the heat with internal cooling methods. Sports Med. 2012;42:89-98. doi:10.2165/11596870-000000000-00000. PMID:22175533.
- Ross M, Abbiss C, Laursen P, Martin D, Burke L. Precooling methods and their effects on athletic performance: A systematic review and practical applications. Sports Med. 2013;43:207-25. doi:10.1007/s40279-012-0014-9. PMID:23329610.
- Tyler CJ, Sunderland C, Cheung SS. The effect of cooling prior to and during exercise on exercise performance and capacity in the heat: A meta-analysis. Br J Sports Med. 2015;49:7-13. doi:10.1136/bjsports-2012-091739. PMID:23945034.
- Bongers CCWG, Thijssen DHJ, Veltmeijer MTW, Hopman MTE, Eijsvogels TMH. Precooling and percooling (cooling during exercise) both improve performance in the heat: A meta-analytical review. Br J Sports Med. 2015;49:377-84. doi:10.1136/bjsports-2013-092928. PMID:24747298.
- Morrison SA, Cheung S, Cotter JD. Importance of airflow for physiologic and ergogenic effects of precooling. J Athl Train. 2014;49:632-9. doi:10.4085/1062-6050-49.3.27. PMID:25144598.
- Leeder J, Gissane C, van Someren K, Gregson W, Howatson G. Cold water immersion and recovery from strenuous exercise: A meta-analysis. Br J Sports Med. 2012;46:233-40. doi:10.1136/bjsports-2011-090061. PMID:21947816.
- DeGroot DW, Gallimore RP, Thompson SM, Kenefick RW. Extremity cooling for heat stress mitigation in military and occupational settings. J Therm Biol. 2013;38:305-10. doi:10.1016/j.jtherbio.2013.03.010.
- Versey NG, Halson SL, Dawson BT. Water immersion recovery for athletes: Effect on exercise performance and practical recommendations. Sports Med. 2013;43:1101-30. doi:10.1007/s40279-013-0063-8. PMID:23743793.
- Cotter JD, Sleivert GG, Roberts WS, Febbraio MA. Effect of pre-cooling, with and without thigh cooling, on strain and endurance exercise performance in the heat. Comp Biochem Physiol Part A Mol Integr Physiol. 2001;128:667-77. doi:10.1016/S1095-6433(01)00273-2.
- Arngrïmsson SA, Petitt DS, Stueck MG, Jorgensen DK, Cureton KJ. Cooling vest worn during active warm-up improves 5-km run performance in the heat. J Appl Physiol. 2004;96:1867-74. doi:10.1152/japplphysiol.00979.2003. PMID:14698992.
- Cheuvront SN, Kolka MA, Cadarette BS, Montain SJ, Sawka MN. Efficacy of intermittent, regional microclimate cooling. J Appl Physiol. 2003;94:1841-8. doi:10.1152/japplphysiol.00912.2002. PMID:12679347.
- Duffield R, Coutts A, McCall A, Burgess D. Pre-cooling for football training and competition in hot and humid conditions. Euro J Sport …. 2013;13:58-67. doi:10.1080/17461391.2011.589474.
- Duffield R, Steinbacher G, Fairchild TJ. The use of mixed-method, part-body pre-cooling procedures for team-sport athletes training in the heat. J Strength Cond Res. 2009;23:2524-32. doi:10.1519/JSC.0b013e3181bf7a4f. PMID:19910821.
- Institute of Medicine (U.S.). DRI, dietary reference intakes for water, potassium, sodium, chloride, and sulfate. Washington (DC): The national academic press; 2004.
- Lee JKW, Shirreffs SM, Maughan RJ. Cold drink ingestion improves exercise endurance capacity in the heat. Med Sci Sports Exerc. 2008;40:1637-44. doi:10.1249/MSS.0b013e318178465d. PMID:18685527.
- Byrne C, Owen C, Cosnefroy A, Lee JKW. Self-paced exercise performance in the heat after pre-exercise cold-fluid ingestion. J Athl Train. 2011;46:592-9. doi:10.4085/1062-6050-46.6.592. PMID:22488183.
- Morris NB, Bain AR, Cramer MN, Jay O. Evidence that transient changes in sudomotor output with cold and warm fluid ingestion are independently modulated by abdominal, but not oral thermoreceptors. J Appl Physiol. 2014;116:1088-95. doi:10.1152/japplphysiol.01059.2013. PMID:24577060.
- Bain AR, Lesperance NC, Jay O. Body heat storage during physical activity is lower with hot fluid ingestion under conditions that permit full evaporation. Acta Physiol (Oxf). 2012;206:98-108. doi:10.1111/j.1748-1716.2012.02452.x. PMID:22574769.
- Lee JKW, Shirreffs SM. The influence of drink temperature on thermoregulatory responses during prolonged exercise in a moderate environment. J Sports Sci. 2007;25:975-85. doi:10.1080/02640410600959947. PMID:17497398.
- Lee JKW, Maughan RJ, Shirreffs SM. The influence of serial feeding of drinks at different temperatures on thermoregulatory responses during cycling. J Sports Sci. 2008;26:583-90. doi:10.1080/02640410701697388. PMID:18344129.
- Siegel R, Maté J, Brearley MB, Watson G, Nosaka K, Laursen PB. Ice slurry ingestion increases core temperature capacity and running time in the heat. Med Sci Sports Exerc. 2010;42:717-25. doi:10.1249/MSS.0b013e3181bf257a. PMID:19952832.
- Yeo ZW, Fan PWP, Nio AQX, Byrne C, Lee JKW. Ice slurry on outdoor running performance in heat. Int J Sports Med. 2012;33:859-66. doi:10.1055/s-0032-1304643. PMID:22730052.
- Stevens CJ, Dascombe B, Boyko A, Sculley D, Callister R. Ice slurry ingestion during cycling improves Olympic distance triathlon performance in the heat. J Sports Sci. 2013;31:1271-9. doi:10.1080/02640414.2013.779740. PMID:23506436.
- Rienzi E, Drust B, Reilly T, Carter JE, Martin A. Investigation of anthropometric and work-rate profiles of elite South American international soccer players. J Sports Med Phys Fitness. 2000;40:162-9. PMID:11034438.
- Di Salvo V, Baron R, Tschan H, Calderon Montero FJ, Bachl N, Pigozzi F. Performance characteristics according to playing position in elite soccer. Int J Sports Med. 2007;28:222-7. doi:10.1055/s-2006-924294. PMID:17024626.
- Mohr M, Nybo L, Grantham J, Racinais S. Physiological responses and physical performance during football in the heat. PLoS ONE. 2012;7:e39202. doi:10.1371/journal.pone.0039202. PMID:22723963.
- Bangsbo J, Iaia FM, Krustrup P. Metabolic response and fatigue in soccer. Int J Sports Physiol Perform. 2007;2:111-27. doi:10.1123/ijspp.2.2.111. PMID:19124899.
- Bergström J, Hermansen L, Hultman E, Saltin B. Diet, muscle glycogen and physical performance. Acta Physiol Scand. 1967;71:140-50. doi:10.1111/j.1748-1716.1967.tb03720.x. PMID:5584523.
- Winnick JJ, Davis JM, Welsh RS. Carbohydrate feedings during team sport exercise preserve physical and CNS function. Med Sci Sports Exerc. 2015:37(2):306-15.
- Vaile J, O'Hagan C, Stefanovic B, Walker M, Gill N, Askew CD. Effect of cold water immersion on repeated cycling performance and limb blood flow. Br J Sports Med. 2011;45:825-9. doi:10.1136/bjsm.2009.067272. PMID:20233843.
- Hornery DJ, Papalia S, Mujika I, Hahn A. Physiological and performance benefits of halftime cooling. J Sci Med Sport. 2005;8:15-25. doi:10.1016/S1440-2440(05)80020-9. PMID:15887897.
- Yeargin SW, Casa DJ, McClung JM, Knight JC, Healey JC, Goss PJ, Harvard WR, Hipp GR. Body cooling between two bouts of exercise in the heat enhances subsequent performance. J Strength Cond Res. 2006;20:383-9. PMID:16686568.
- Peiffer JJ, Abbiss CR, Watson G, Nosaka K, Laursen PB. Effect of a 5-min cold-water immersion recovery on exercise performance in the heat. Br J Sports Med. 2010;44:461-5. doi:10.1136/bjsm.2008.048173. PMID:18539654.
- Lee JKW, Yeo ZW, Nio AQX, Koh ACH, Teo YS, Goh LF, Tan PM, Byrne C. Cold drink attenuates heat strain during work-rest cycles. Int J Sports Med. 2013;34:1037-42. doi:10.1055/s-0033-1337906. PMID:23670358.
- Stanley J, Leveritt M, Peake JM. Thermoregulatory responses to ice-slush beverage ingestion and exercise in the heat. Eur J Appl Physiol. 2010;110:1163-73. doi:10.1007/s00421-010-1607-3. PMID:20714767.
- Nadel ER, Fortney SM, Wenger CB. Effect of hydration state of circulatory and thermal regulations. J Appl Physiol. 1980;49:715-21. PMID:7440285.
- Fortney SM, Wenger CB, Bove JR, Nadel ER. Effect of hyperosmolality on control of blood flow and sweating. J Appl Physiol. 1984;57:1688-95. PMID:6511544.
- González-Alonso J, Calbet JA, Nielsen B. Muscle blood flow is reduced with dehydration during prolonged exercise in humans. J Physiol. 1998;513(Pt 3):895-905. doi:10.1111/j.1469-7793.1998.895ba.x. PMID:9824726.
- Stöhr EJ, González-Alonso J, Pearson J, Low DA, Ali L, Barker H, Shave R. Dehydration reduces left ventricular filling at rest and during exercise independent of twist mechanics. J Appl Physiol. 2011;111:891-7. doi:10.1152/japplphysiol.00528.2011. PMID:21700893.
- Sawka MN, Toner MM, Francesconi RP, Pandolf KB. Hypohydration and exercise: Effects of heat acclimation, gender, and environment. J Appl Physiol. 983;55:1147-53.
- Sawka MN. Physiological consequences of hypohydration: Exercise performance and thermoregulation. Med Sci Sports Exerc. 1992;24:657-70. doi:10.1249/00005768-199206000-00008. PMID:1602938.
- González-Alonso J, Mora-Rodríguez R, Coyle EF. Stroke volume during exercise: Interaction of environment and hydration. Am J Physiol Heart Circ Physiol. 2000;278:H321-30. PMID:10666060.
- Costill DL. Sweating: Its composition and effects on body fluids. Ann N Y Acad Sci. 1977;301:160-74. doi:10.1111/j.1749-6632.1977.tb38195.x. PMID:270913.
- Verde T, Shephard RJ, Corey P, Moore R. Sweat composition in exercise and in heat. J Appl Physiol 1982;53:1540-5. PMID:7153150.
- Bergeron MF. Heat cramps: Fluid and electrolyte challenges during tennis in the heat. J Sci Med Sport. 2003;6:19-27. doi:10.1016/S1440-2440(03)80005-1. PMID:12801207.
- Duvillard von SP, Braun WA, Markofski M, Beneke R, Leithäuser R. Fluids and hydration in prolonged endurance performance. Nutrition. 2004;20:651-6. doi:10.1016/j.nut.2004.04.011. PMID:15212747.
- Burke LM, Hawley JA, Wong SHS, Jeukendrup AE. Carbohydrates for training and competition. J Sports Sci. 2011;29 Suppl 1:S17-27. doi:10.1080/02640414.2011.585473. PMID:21660838.
- Sawka MN, Wenger CB, Pandolf KB. Thermoregulatory responses to acute exercise-heat stress and heat acclimation. In: Fregly MJ, Blatteis CM, editors. Handbook of physiology. New York, NY: Comprehensive …; 1996. pp. 157-85.
- Gisolfi C, Robinson S. Relations between physical training, acclimatization, and heat tolerance. J Appl Physiol. 1969;26:530-4. PMID:5781602.
- Nadel ER, Pandolf KB, Roberts MF, Stolwijk JA. Mechanisms of thermal acclimation to exercise and heat. J Appl Physiol. 1974;37:515-20. PMID:4414615.
- Roberts MF, Wenger CB, Stolwijk JA, Nadel ER. Skin blood flow and sweating changes following exercise training and heat acclimation. J Appl Physiol. 1977;43:133-7. PMID:893254.
- Armstrong LE, Pandolf KB. Physical training, cardiorespiratory physical fitness and exercise-heat tolerance. In: Pandolf KB, Sawka MN, Gonzalez RR, editors. Physiology and environmental medicine at terrestrial extremes. Indianapolis (IN): Physiology and Environmental Medicine at Terrestrial Extremes; 1988.
- Lorenzo S, Halliwill JR, Sawka MN, Minson CT. Heat acclimation improves exercise performance. J Appl Physiol. 2010;109:1140-7. doi:10.1152/japplphysiol.00495.2010. PMID:20724560.
- Racinais S, Périard JD, Karlsen A, Nybo L. Effect of heat and heat acclimatization on cycling time trial performance and pacing. Med Sci Sports Exerc. 2015;47:601-6. doi:10.1249/MSS.0000000000000428. PMID:24977692.
- Robinson S, Turrell ES, Belding HS, Horvath SM. Rapid acclimatization to work in hot climates. Am J Physiol. 1943;140:168-76.
- Ladell WS. Assessment of group acclimatization to heat and humidity. J Physiol. 1951;115:296-312. doi:10.1113/jphysiol.1951.sp004672. PMID:14898513.
- Flouris AD, Poirier MP, Bravi A, Wright-Beatty HE, Herry C, Seely AJ, Kenny GP. Changes in heart rate variability during the induction and decay of heat acclimation. Eur J Appl Physiol. 2014;114:2119-28. doi:10.1007/s00421-014-2935-5. PMID:24957416.
- Sunderland C, Morris JG, Nevill ME. A heat acclimation protocol for team sports. Br J Sports Med. 2008;42:327-33. doi:10.1136/bjsm.2007.034207. PMID:18460609.
- Garrett AT, Rehrer NJ, Patterson MJ. Induction and decay of short-term heat acclimation in moderately and highly trained athletes. Sports Med. 2011;41:757-71. doi:10.2165/11587320-000000000-00000. PMID:21846164.
- Chalmers S, Esterman A, Eston R, Bowering KJ, Norton K. Short-term heat acclimation training improves physical performance: A systematic review, and exploration of physiological adaptations and application for team sports. Sports Med. 2014;44:971-88. doi:10.1007/s40279-014-0178-6. PMID:24817609.
- Karlsen A, Nybo L, Norgaard SJ, Jensen MV, Bonne T, Racinais S. Time course of natural heat acclimatization in well-trained cyclists during a 2-week training camp in the heat. Scand J Med Sci Sports. 2015;25 Suppl 1:240-9. doi:10.1111/sms.12449. PMID:25943675.
- Scoon GSM, Hopkins WG, Mayhew S, Cotter JD. Effect of post-exercise sauna bathing on the endurance performance of competitive male runners. J Sci Med Sport. 2007;10:259-62. doi:10.1016/j.jsams.2006.06.009. PMID:16877041.
- Karlsen A, Racinais S, Jensen MV, Norgaard SJ, Bonne T, Nybo L. Heat acclimatization does not improve VO2max or cycling performance in a cool climate in trained cyclists. Scand J Med Sci Sports. 2015;25:269-76. doi:10.1111/sms.12409. PMID:25943678.
- Keiser S, Flück D, Hüppin F, Stravs A, Hilty MP, Lundby C. Heat training increases exercise capacity in hot but not in temperate conditions: A mechanistic counter-balanced cross-over study. Am J Physiol Heart Circ Physiol. 2015;309:H750-61. PMID:26150574.
- Minson CT, Cotter JD. CrossTalk proposal: Heat acclimatization does improve performance in a cool condition. J Physiol. 2016;594:241-3. doi:10.1113/JP270879. PMID:26668072.
- Nybo L, Lundby C. CrossTalk opposing view: Heat acclimatization does not improve exercise performance in a cool condition. J Physiol. 2016;594:245-7. doi:10.1113/JP270880. PMID:26667955.
- Shvartz E, Shapiro Y, Magazanik A, Meroz A, Birnfeld H, Mechtinger A, Shibolet S. Heat acclimation, physical fitness, and responses to exercise in temperate and hot environments. J Appl Physiol. 1977;43:678-83. PMID:908683.
- Hue O, Antoine-Jonville S, Sara F. The effect of 8 days of training in tropical environment on performance in neutral climate in swimmers. Int J Sports Med. 2007;28:48-52. doi:10.1055/s-2006-923958. PMID:16761217.
- Neal RA, Corbett J, Massey HC, Tipton MJ. Effect of short-term heat acclimation with permissive dehydration on thermoregulation and temperate exercise performance. Scand J Med Sci Sports. 2016;26(8):875-84. doi: 10.1111/sms.12526. PMID:26220213.
- Buchheit M, Voss SC, Nybo L, Mohr M, Racinais S. Physiological and performance adaptations to an in-season soccer camp in the heat: Associations with heart rate and heart rate variability. Scand J Med Sci Sports. 2011;21:e477-85. doi:10.1111/j.1600-0838.2011.01378.x. PMID:22092960.
- Buchheit M, Racinais S, Bilsborough J, Hocking J, Mendez-Villanueva A, Bourdon PC, Voss S, Livingston S, Christian R, Périard J, et al. Adding heat to the live-high train-low altitude model: A practical insight from professional football. Br J Sports Med. 2013;47 Suppl 1:i59-69. doi:10.1136/bjsports-2013-092559. PMID:24282209.
- Racinais S, Buchheit M, Bilsborough J, Bourdon PC, Cordy J, Coutts AJ. Physiological and performance responses to a training camp in the heat in professional Australian football players. Int J Sports Physiol Perform. 2014;9:598-603. doi:10.1123/ijspp.2013-0284. PMID:24088292.