ABSTRACT
Obesity and associated metabolic dysfunction have reached epidemic proportions worldwide. The current theory linking metabolic disease and obesity involves ischemic adipose tissue initiating an inflammatory cascade that results in systemic insulin resistance and may eventually lead to type II diabetes mellitus. Diabetes and associated metabolic dysfunction increase the risk of developing cardiovascular disease and fatal cardiovascular events. By targeting key steps in this process, ischemia and inflammation, this cascade may be prevented or reversed and thus metabolic and cardiovascular health may be preserved in obesity. Regular heat exposure (termed ‘heat therapy’) offers potential to improve cardiometabolic health in obese individuals through a variety of mechanisms that include but are not limited to heat shock proteins, hypoxia-inducible factor 1α, and hemodynamic effects. The purpose of this review is to highlight the cardiometabolic decline in obese individuals stemming from adipose tissue dysfunction, and examine the ways in which heat therapy and associated cellular and systemic adaptations can intersect with this decline in function to improve or restore cardiovascular and metabolic health.
Obesity and associated disease rates have reached epidemic proportions, with nearly two billion people worldwide being classified as overweight or obese.Citation1 For example, as of 2012, 33.7% of men and 36.5% of women in the United States were classified as obese (BMI ≥ 30),Citation2 and 9.3% of the U.S. population suffered from type II diabetes, a disease closely associated with excess fat mass and a sedentary lifestyle. With rates of obesity and diabetes continuing to rise, these classifications are risk factors for the development of cardiovascular disease in both men and women.Citation3 Thus, obese individuals are a high-risk population for both cardiovascular and metabolic disease (termed ‘cardiometabolic disease’), and interventions aimed at improving cardiometabolic health in obese populations are sorely needed. Current lifestyle interventions for obesity and cardiometabolic disease emphasize dietary modification and exercise training. While changing diet and exercise patterns can be effective strategies for reducing body mass, improving vascular health, and enhancing insulin sensitivity, compliance is often low in clinical populations.Citation4 Barriers to exercise include physical limitations associated with obesity, injury, motivation, body image, and socioeconomic constraints.Citation4 In extreme cases (morbid obesity, or obesity with comorbidities), surgical intervention through roux-en-Y gastric bypass, vertical sleeve gastrectomy, or other bariatric procedures is another technique to reduce excess mass and improve health. While weight loss is sustained and comorbidities are reduced in the majority of patients, this remains an expensive procedure with some patients continuing to deal with weight regain and/or unresolved diabetes, hypertension, and dyslipidemia.Citation5,Citation6
Chronic heat exposure may offer an alternative or supplemental therapy to improve metabolic health and provide protection from cardiovascular disease in obese individuals. There are a variety of potential mechanisms for the observed improvements in cardiovascular and metabolic health with chronic, intermittent heat exposure (most commonly termed ‘heat therapy’, but also ‘hyperthermic conditioning’, or ‘thermotherapy’). The purpose of this review is to highlight the cardiometabolic decline in obese individuals stemming from adipose tissue dysfunction, and examine the ways in which heat therapy and associated cellular and systemic adaptations can intersect with this decline in function to improve or restore cardiovascular and metabolic health. Finally, we review the current evidence of cardiometabolic improvement with heat therapy using different heating methods in humans.
Obesity, inflammation, and insulin resistance
While the growing obesity epidemic and associated metabolic disease rates have been well-documented and characterized, the causal link between obesity and metabolic dysfunction is continually being described and explored. Evidence points to an increase in systemic inflammation in obesity being a primary culprit in both metabolic and cardiovascular dysfunction. Early case studies noted improvement in diabetes mellitus and glycosuria with anti-inflammatory (salicylate) therapy, but no potential mechanism was proposed.Citation7 Inflammation and activation of innate immunity in obese humans was first described in 1985, with researchers noting that morbidly obese individuals had elevated leukocyte counts.Citation8 In an animal model of obesity and diabetes, HotamisligilCitation9 demonstrated that elevated systemic and adipose tissue tumor necrosis factor α (TNFα) abundance were associated with impaired glucose tolerance and adipocyte glucose uptake. In humans, obesity has since been found to be associated with higher levels of C-reactive protein, interleukin 6 (IL-6), fibrinogen, TNFα, plasminogen activator inhibitor 1, and several other inflammatory proteins.Citation10 These proteins may be secreted by adipose tissue, liver, and skeletal muscle, and have implications in both metabolic and cardiovascular dysfunction. Weight loss through bariatric surgery,Citation11 diet,Citation12 or diet and exerciseCitation11 results in decreases in inflammatory markers including C-reactive protein, plasminogen activator inhibitor 1, IL-6, and TNFα. Additionally, anti-inflammatory therapy (salicylates) has been shown to reverse insulin resistance in obese rodents.Citation13 These findings led to the current theory that inflammation drives obesity-induced insulin resistance.
The pathophysiological link between obesity and metabolic disease relates to increased triglyceride storage in adipocytes causing adipose tissue hypoxia through compression of capillary networks and inadequate blood supply relative to cell size.Citation14,Citation15 This initiates a cascade of adipocyte apoptosis, followed by a pro-inflammatory immune response (see ). The immune response involves a variety of chemokines, adipokines, and immune cells, which alter the profile of obese adipose tissue to a pro-inflammatory phenotype.Citation16 The change in immune cell profile includes an increase in M1 macrophages forming a crown-like structure around the adipocyteCitation17 and releasing pro-inflammatory cytokines in the adipose tissue. Adipocytes act in a synergistic paracrine fashion with resident macrophages to increase inflammatory cytokine release by the other tissue. These cytokines are thought to disrupt insulin signaling in adipose tissue through serine phosphorylation of the insulin receptor substrate (IRS), which blocks tyrosine binding sites needed to activate the IRS within the cell and allow insulin signaling to occur. The primary function of insulin in adipose tissue is suppression of free fatty acid release, so the result of this impairment is increased fatty acids in circulation.Citation18 These circulating fatty acids can accumulate in the liver and skeletal muscle and produce fatty acid intermediates such as diacylglycerol, ceramides, and long-chain fatty acid-Acyl CoA,Citation19 all of which can inhibit intracellular insulin signaling by activation of c-Jun NH2-terminal KinaseCitation20 (JNK) or Inhibitor of kappa B kinase β (IKKβ).Citation13,Citation21 JNK and IKKβ similarly impair intracellular insulin signaling by serine phosphorylation of IRS.Citation22 This results in systemic insulin resistance with an impaired ability of cells to transport glucose or suppress glucose production, creating a more metabolically inflexible profile.Citation23 In addition, adipokines such as leptin and adiponectin are altered in obesity,Citation24 with adiponectin in particular at much lower circulating levels in obese individuals.Citation25 Adiponectin is positively correlated with insulin sensitivity,Citation26 potentially acting by changing macrophage polarization toward an anti-inflammatory profile.Citation27 The end result is a hyper-insulinemic and meta-inflammatory profile in obesity that vastly increases the risk of developing both metabolic and cardiovascular disease.Citation28
Figure 1. An overview of inflammation and ischemia in obese adipose tissue. Excess fat storage in obese individuals leads to adipose tissue expansion as compared to lean individuals, and the blood supply does not adequately match this tissue expansion. This adipocyte hypertrophy and inadequate blood supply causes adipocyte hypoxia, inflammatory cytokine release (IL-6, TNFα) by adipocytes and M1 macrophages, a reduction in adiponectin release, and impaired insulin action. Low adiponectin promotes the pro-inflammatory profile of macrophages, and adipocytes and macrophages act in a paracrine fashion to further increase cytokine release from neighboring adipose tissue. Insulin resistance in adipocytes causes impaired suppression of lipolysis, and fatty acids are released and deposited in other insulin target tissues such as skeletal muscle and liver. Partially oxidized fatty acids increase inflammation through proteins such as JNK and IKKβ in the liver and skeletal muscle, impairing insulin signaling in these tissues. Impaired insulin action in the liver leads to an increase in glucose release (impaired suppression of glycolysis), and in skeletal muscle leads to decreased glucose uptake (impaired GLUT-4 translocation). The end result is hyperglycemia, hyperlipidemia, meta-inflammation, and insulin resistance. Filled arrows with + signs represent increases in release or uptake, while unfilled arrows with – signs represent decreases in release or uptake.
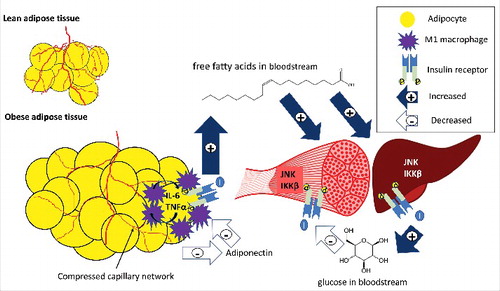
Within the central nervous system, inflammation and hyperinsulinemia are associated with increased sympathetic nervous system (SNS) outflow.Citation29 IL-6 receptors are present on sympathetic gangliaCitation30,Citation31 and IL-6 infusions have been shown in to increase SNS activity in humans.Citation32 Further, elevated TNFα increases the expression of IL-6 receptors on sympathetic neurons,Citation30 and both cytokines are elevated in obese humans.Citation33,Citation34 Insulin also acts centrally to increase sympathetic outflow,Citation35,Citation36 increasing the risk of hypertension in insulin-resistant populations.Citation37 High sympathetic outflow increases blood pressure through cardiac, renal, and arterial innervation, and SNS over-activity is considered an important risk factor for development of cardiovascular disease.Citation38 In addition, obesity-induced SNS over-activity contributes to end-organ damage in the kidney, blood vessels, and heart, increasing cardiovascular morbidity and mortality, even in the absence of hypertension.Citation39 Specific adipokines may influence the sympathetic overactivity,Citation29 creating a vicious cycle of dysfunction that likely contributes to the cardiovascular and metabolic disturbances observed with obesity.
Systemic insulin resistance is also associated with impaired endothelium-dependent dilation and microvascular function,Citation40,Citation41 observed in impairment of insulin's actions on blood vesselsCitation42 as well as reduction in bioavailable nitric oxide (NO) due to the high oxidative stress seen in hyperinsulinemic individuals.Citation43 The meta-inflammatory state of obesity additionally causes impaired vascular remodeling, resulting in increased arterial stiffnessCitation44 and intima media thickness.Citation45 Intima media thickening is also associated with the dyslipidemia seen in obesity throughout the lifespan.Citation46
While all obese individuals are at an elevated risk of cardiometabolic dysfunction compared to healthy weight counterparts, there appears to be a sex difference, placing women with diabetes at an elevated risk for cardiovascular death as compared to obese, diabetic men.Citation3 Within the population of obese women, diagnosis with polycystic ovary syndrome (PCOS) additionally carries a disproportionate risk of cardiovascular disease, diabetes, and cardiovascular death.Citation47 PCOS is an endocrine disorder characterized by androgen excess, menstrual dysfunction, and polycystic ovaries upon ultrasound examination, and this syndrome affects up to 15% of women. The metabolic, autonomic, and hormonal profiles in women with PCOS greatly increase the risk for obesity, insulin resistance, and cardiovascular disease.Citation48 Sympathetic over-activityCitation49,Citation50 may underlie the pathogenesis of PCOS and additionally increase risk of cardiovascular disease, so the potential for chronic heat to alter autonomic outflow is particularly promising in this population.
How can chronic heat therapy help?
Regular heat exposure, through sauna use, hot water immersion, or combined exercise heat stress, is associated with a variety of cellular and systemic adaptations that have potential to improve cardiometabolic health in obese individuals. In animal work, passive heat exposure with marked elevation in core temperature is associated with changes in protein expression and abundance that lead to enhanced cardiovascular and metabolic health, as well as cellular protection from a multitude of stressors.Citation51,Citation52 Long-term passive heat acclimation (30 days) has been shown in animal models to initiate cellular pathways such as Heat Shock Proteins (HSP) and Hypoxia-inducible Factor 1α (HIF1α)Citation51,Citation52 that enhance blood supply, protect cells from stressors such as ischemia,Citation53 and reduce inflammation.Citation54 In addition, altered expression of adipokines such as leptin and adiponectin have been observed in animals following repeated heat exposure.Citation55 As such, there are multiple possible mechanisms by which heat therapy in humans could attenuate or prevent the development of insulin resistance, diabetes, and cardiovascular disease in obesity. These mechanisms may work synergistically to intersect with the obesity-inflammation cascade to potentially reduce ischemia, inflammation, insulin resistance, and vascular dysfunction.
Ischemia, inflammation, and insulin resistance
Seminal work in animals using long-term heat acclimation first described heat acclimation cross-tolerance, where long-term passive heat acclimation protected cells from a multitude of stressors, including ischemia/hypoxia. This was first described in the rat heart, with a reduced infarct size in response to ischemia-reperfusion injury in heat-acclimated animals.Citation53 Since then, interest in heat/hypoxia cross-tolerance has expandedCitation56 to include acute human studies of ischemia-reperfusionCitation57 and human performance models.Citation58,Citation59 It is thought that both HSPs and HIF1α play a role in protection from ischemic injury.Citation52
In obesity, adipocyte ischemia is among the first steps leading to cardiometabolic dysfunction. Chronic heat provides multiple avenues to improve blood supply (see ). For example, one downstream target of HIF-1α is vascular endothelial growth factor (VEGF),Citation53 which stimulates microvascular angiogenesis. Recent human work examining acute heat exposure demonstrated increased expression of various angiogenic signals including VEGF and angiopoietin after one 90-minute leg heating session, with concomitant increases in Hsp90 expression.Citation60 Hsp90 can also act through stabilizing endothelial nitric oxide synthase,Citation61 and NO acts as another angiogenic signal.Citation62 In addition, NO production in endothelial cells is enhanced through shear stress,Citation63 as observed during acute heating. If blood supply to adipocytes is improved through some combination of these mechanisms, adipocytes are less likely to become ischemic, which may attenuate the inflammatory response that comes from ischemia-induced hypoxia. While acute heat exposure may result in transient increases in pro-inflammatory compounds such as IL-6Citation64 and JNK,Citation65 chronic heat treatment has been shown to decrease intracellular levels of inflammatory proteins such as JNK and IKKβ.Citation66 Heat shock proteins have been linked with altered expression of pro- and anti-inflammatory cytokines,Citation67,Citation68 and decreases in other inflammatory compounds such as JNK and IKKβ in skeletal muscleCitation66 in response to repeated heat exposure. IL-6 and TNFα, both targets of HSPs, are associated with impaired insulin signaling in adipose tissue,Citation69–71 and JNK and IKKβ have been shown to impair insulin signaling in skeletal muscle, as well as liver and adipose tissue.Citation21,Citation72,Citation73
Figure 2. The potential pathways through which chronic heat exposure can reduce inflammation, improve blood flow, and reduce insulin resistance. The increases in shear, HSP90, and HIF1α all offer potential to improve blood flow and reduce adipose tissue hypoxia, through mechanisms such as increased nitric oxide (NO) and vascular endothelial growth factor (VEGF). In addition, HSP70 reduces inflammatory markers in both adipose tissue (nuclear factor kappa-B [NFκB], IL-6, and TNFα) and skeletal muscle (JNK), along with HSP27 (IKKβ). In addition, HSP70 is involved in protein refolding of the insulin receptor. In concert, these mechanisms can reduce inflammation, improve insulin signaling, increase glucose uptake and reduce fatty acid release, and increase adiponectin secretion, improving the metabolic health profile in obesity. While the changes in protein abundance and expression have been experimentally observed in human or animal models (denoted with boxes), some downstream effects have not specifically been examined in response to chronic heat.
![Figure 2. The potential pathways through which chronic heat exposure can reduce inflammation, improve blood flow, and reduce insulin resistance. The increases in shear, HSP90, and HIF1α all offer potential to improve blood flow and reduce adipose tissue hypoxia, through mechanisms such as increased nitric oxide (NO) and vascular endothelial growth factor (VEGF). In addition, HSP70 reduces inflammatory markers in both adipose tissue (nuclear factor kappa-B [NFκB], IL-6, and TNFα) and skeletal muscle (JNK), along with HSP27 (IKKβ). In addition, HSP70 is involved in protein refolding of the insulin receptor. In concert, these mechanisms can reduce inflammation, improve insulin signaling, increase glucose uptake and reduce fatty acid release, and increase adiponectin secretion, improving the metabolic health profile in obesity. While the changes in protein abundance and expression have been experimentally observed in human or animal models (denoted with boxes), some downstream effects have not specifically been examined in response to chronic heat.](/cms/asset/cc0d90ae-e092-41b8-a264-eb5a1d7883f4/ktmp_a_1384089_f0002_oc.jpg)
HSP levels have been linked to insulin sensitivity in humansCitation74 through a variety of mechanisms. Individuals with type II diabetes exhibit reduced levels of HSPs in adipose tissueCitation75 and skeletal muscle.Citation74 Animal work using regular heat exposure examined the relationship between various HSPs and insulin signaling in rat skeletal muscle, and found both Hsp27 and Hsp70 decreased inflammatory proteins such as JNK and IKKβ, both known to impair insulin signaling through serine phosphorylation of IRS-1.Citation66 In adipose tissue, Hsp70 decreases the expression of nuclear factor kappa-B, which in turn reduces the release of pro-inflammatory cytokines such as IL-1β, IL-6, and TNFα.Citation76 Hsp70 is additionally involved in the protein refolding of the insulin receptor when denatured by stress,Citation77 providing another mechanism through which heat shock proteins can improve or maintain insulin signaling in populations with impaired metabolic health. In skeletal muscle, mild heating increases expression of genes encoding mitochondrial biogenesis,Citation78 which can increase energy flux in the cell and reduce the accumulation of the fatty acid intermediates linked to inflammation and insulin resistance. In addition, animal work has suggested that as little as five days of passive heat exposure in mice increased serum adiponectin levels,Citation55 which is associated with enhanced insulin sensitivity and reduce inflammation.Citation25
Vascular dysfunction
In advance of overt cardiovascular disease, obesity and insulin resistance can increase cardiovascular dysfunction and blood pressure through impaired vascular remodeling,Citation25,Citation79 endothelial dysfunction,Citation40 and elevated sympathetic nervous system activity.Citation29,Citation80 While the relative contributions of obesity, inflammation, and metabolic dysfunction are difficult to tease apart, these elements combine to create an elevated risk of cardiovascular disease and cardiovascular deathCitation3 in obesity.
Heat therapy offers potential to attenuate or reverse impairment through a variety of mechanisms (see ). First, acute heating, through hot water immersion or sauna, promotes increases in cardiac output and redistribution of blood flow to the periphery as a cooling mechanism. This increase in skin blood flow alters the shear pattern of arterial blood flow through conduit vessels to increase anterograde shear and reduce retrograde shear.Citation63,Citation81,Citation82 This altered shear pattern has been shown to enhance vascular remodeling and endothelial function following exercise trainingCitation83,Citation84 and passive heating.Citation81,Citation83 Acute leg heating has also been shown in patients with symptomatic peripheral artery disease to enhance lower limb blood flow, reduce blood pressure and decrease circulating endothelin-1,Citation85 all of which can improve vascular health and function, particularly if heat is repeatedly applied over time.
Figure 3. The potential pathways through which chronic heat exposure can improve cardiovascular health in the heart, macrovasculature, and microvasculature and autonomic activity in the brain. Increases in HSP90 and shear stress with heat therapy act to increase nitric oxide (NO), which can decrease sympathetic outflow, increase vasodilation in the microvasculature, and, along with HIF1α/VEGF, increase angiogenesis in the microvasculature. Increases in angiotensin II type 2 receptors (AT2) and adiponectin in the central nervous system can additionally reduce sympathetic outflow, which can decrease heart rate and peripheral resistance, reducing stress on the cardiovascular system. HSP27 and HSP70 decrease intimal and vascular smooth muscle (VSM) hyperplasia, and HSPs and HIF1α improve ischemic tolerance in the heart, reducing the risk or severity of cardiovascular events. Together, these mechanisms can reduce sympathetic outflow, reduce blood pressure, increase ischemic tolerance, and enhance vascular remodeling to improve the cardiovascular risk profile in obesity. Changes in shear stress, protein abundance and expression have been experimentally observed in human or animal models (indicated with boxes); however; some downstream effects have not specifically been examined in response to chronic heat.
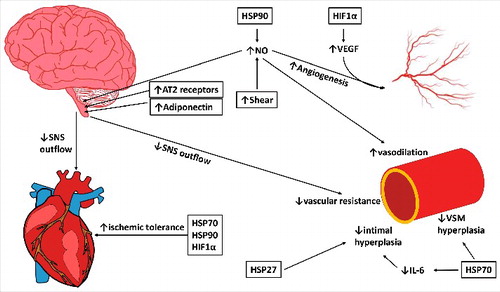
Heat shock proteins also play an important role in cardiovascular protection. In vascular remodeling, Hsp27 reduces intimal hyperplasia,Citation86 an early step in formation of atherosclerotic plaques. Hsp72, through inhibition of Angiotensin II, reduces vascular smooth muscle hypertrophy.Citation87 Heat exposure has additionally been associated with reductions in IL-6Citation88 and increases in adiponectin,Citation55 which promote and inhibit vascular inflammation, respectively.
Endothelial function, in healthy populations, is predominantly dependent on the production of bioavailable NO.Citation89 Hsp90 is an essential cofactor for nitric oxide synthase stability,Citation61 so increases in Hsp90 expression would likely lead to an increase in endothelial NO production, as seen in animal models.Citation90,Citation91 In human models of vascular function, this increased NO production would enhance vasodilation as assessed by techniques such as flow-mediated dilationCitation92 and cutaneous local heating.Citation93 Since endothelialCitation40 and cutaneous microcirculatory functionCitation94 are impaired in obesity, even in advance of overt cardiovascular disease or hyperglycemia,Citation94 improving microcirculatory function is a promising means to improve cardiovascular health in obese individuals.
Sympathetic nervous system activity is regulated through a variety of neurological, neurohumoral, and psychological inputs, and can be modulated by a variety of hormones and compounds including adiponectin,Citation95 Angiotensin II,Citation96 and NO.Citation97 In addition to the cardiovascular consequences of high sympathetic activity, sympathetic outflow is inter-related with metabolic function in obesity.Citation29 High circulating epinepherine suppresses insulin release from the pancreas and increases lipolysis and fatty acid release in to the bloodstream.Citation98 In turn, inflammation and hyperinsulinemia increase sympathetic outflow,Citation35,Citation36 creating a positive feedback loop for both cardiovascular and metabolic decline in obesity. Chronic heat exposure offers potential to reduce sympathetic outflow through reductions in inflammation and insulin resistance as previously described, and can additionally reduce sympathetic activity through increasing circulating adiponectinCitation55 and enhancing NO production.Citation90,Citation91,Citation99 Heat acclimation has additionally been shown, in murine models, to increase Angiotensin II receptor subtype 2 (AT2) in the hypothalamus,Citation100 which acts to reduce sympathetic outflow.Citation101
In combination, heat therapy offers the potential to improve metabolic and cardiovascular function and risk through a variety of mechanisms. To date, research in humans, particularly in obese individuals, has been limited.
Current evidence in humans
A variety of heating methods, timelines, and cardiometabolic outcome measures have been examined in healthy and obese populations. Hot water immersion was one of the first therapeutic methods to be studied in humans, with HooperCitation102 examining glucose control in eight obese, diabetic individuals following three weeks of regular hot tub use (30 minutes per session). The subjects experienced a large decrease in fasting glucose and glycosylated hemoglobin, and the researchers postulated that this was due to the increased blood flow to skeletal muscles during heating. While multiple alternative mechanisms have since been explored, this study was among the first to examine health benefits of passive heating in obese individuals. Local heating of abdominal adipose tissue, similar to that experienced during hot water immersion, has also been shown to reduce visceral fat storage and improve glucose tolerance in obese, diabetic individuals.Citation103
Acute hot water immersion has since been studied as a means to improve glucose control. Faulkner and colleaguesCitation64 compared the glucose response to a meal after either a 60-min hot bath or 60-min moderate intensity exercise in lean and overweight men, and found that heat decreased peak post-prandial glucose compared to exercise, with no difference in 24-h glucose control between heat and exercise. The authors postulated that increased HSP production in response to heat drove the improved glucose control through enhanced insulin signaling.
Vascular health and function have also been examined in response to repeated passive heat exposure in healthy, inactive men and women. Brunt and colleaguesCitation92 examined the effect of 8 weeks of hot water immersion (4–5 times per week for ∼90 min per session, with core temperature increase of ∼1.5˚C) and observed improvements in endothelial function, arterial stiffness, wall thickness, and blood pressure. In a companion study, this group also investigated cutaneous vasodilation in response to local heating as a model of microvascular function and specifically examined the role of NO,Citation93 and observed an increase in cutaneous vascular conductance to thermal hyperemia that was primarily mediated by NO.
Sauna has also been investigated both as an intervention in clinical populations and in prospective cohort studies. Classic Finnish saunas involve air temperatures of 80–100°C with low humidity, and individuals spend 5–30 minutes at a time in the sauna with brief breaks in a thermoneutral room between multiple bouts. A 30-min bout in an 80°C sauna quickly increases skin temperature and heart rate, and raises rectal temperature ∼0.9°C.Citation104 Two weeks of thermal therapy (60°C far-infrared sauna 6 days per week) in men with elevated cardiovascular risk significantly improved endothelial function, assessed via flow-mediated dilation.Citation105 A study in men with congestive heart failure underwent the same therapy and similar improvements in flow-mediated dilation were observed.Citation106 In addition, brain natriuretic peptide (a marker of cardiac dysfunction) was significantly reduced following thermal therapy.
A large prospective cohort study (2,315 Finnish men) examined frequency and duration of sauna use and the correlation with mortality rates during a 20-year follow-up.Citation107 Increased frequency and duration of sauna use were associated with substantially reduced hazard ratios for sudden cardiac death, fatal coronary heart disease, fatal cardiovascular disease, and all-cause mortality. While this study only examined men, did not include subject that did not regularly use sauna, and did not specifically examine death related to metabolic diseases such as diabetes, it is the largest and longest study to date on the potential long-term cardiovascular health benefits of regular passive heat exposure.
Performing yoga in a hot room may offer an alternative to passive immersions in more able-bodied populations, with the additional benefit of low to moderate intensity exercise. Research is mixed on the increase in core temperature observed during a 90-min Bikram yoga class (a series of 26 postures in a room set to 40.5°C, 40% relative humidity), with values ranging from mild hyperthermia [0.6–1.0°C increase in novice and experienced practitioners, respectivelyCitation108] to increases more similar to those seen in hot water immersion [1.7–2.5°C in men and women, respectivelyCitation109]. While core temperature elevation in hot yoga is highly dependent on both ambient temperature and level of intensity (metabolic heat production), some promising results of hot yoga training have been seen in obese populations.
Hunter et al.Citation110 examined the effect of an 8-week hot yoga intervention (three 90-minute sessions per week) on body composition and glucose tolerance. Following this intervention, a significant reduction in glucose area under the curve was noted in obese men and women, with no change in lean individuals. Fasting glucose did not change in either population, and body composition was additionally unaltered. Since this study did not have an exercise-only, heat-only, or time control, it is unclear whether the exercise, the high temperature, or the combination of both stressors drove these changes. However, the findings do suggest that hot yoga may improve glucose tolerance in obese individuals.
In a similar intervention study from the same research group, arterial stiffness, blood pressure, cholesterol, and insulin levels were examined in healthy younger and older individuals in response to an 8-week Bikram yoga practice.Citation111 Again, this study lacked a control group to tease apart the effects of yoga, heat, or time, but younger individuals experienced a decrease in arterial stiffness, while older individuals experienced a decrease in fasting insulin and low-density lipoprotein-cholesterol. Blood pressure did not change in either group after the 8-week Bikram yoga intervention.
Guo et alCitation112 performed a longer-duration study examining markers of body composition, cardiovascular health, and psychological well-being follow one year of Bikram yoga (4 sessions per week) in obese individuals. The yoga intervention led to significant reductions in skinfold thickness, waist circumference, blood pressure (MAP decreased ∼3mmHg), total cholesterol, low-density lipoprotein-cholesterol, and triglycerides, and led to increases in vital capacity, high-density lipoprotein-cholesterol, and subjective scores of well being. No study has yet examined the combination of metabolic and cardiovascular health markers following hot yoga training, but these early studies suggest a benefit for cardiometabolic health in obese populations.
Summary & perspectives
The multifaceted decline in cardiovascular and metabolic function that occurs in obesity has been well-described, and despite treatment options including diet, exercise, surgery, and a variety of medications, obesity remains a global epidemic with extremely high associated healthcare costs.Citation113 Chronic heat exposure as heat therapy offers potential as a novel or adjunctive therapy to improve cardiometabolic health in obesity and to potentially reduce the medical burden of obesity. While not offering a direct path to weight reduction, the reductions in inflammation, improvements in glucose tolerance, and improvements in vascular function that have been observed in human and animal models with chronic heat exposure provide a variety of avenues through which cardiometabolic health can be improved in the absence of weight changes.
Future work exploring heat therapy as a means to improve cardiometabolic health should examine a dose-response and timeline, as a wide variety of acute and chronic timelines have been utilized, resulting in a large range of core temperature responses, and total heat exposure time ranging from 9 hours over 3 weeksCitation102 to over 300 hours in a 1-year intervention.Citation112 The underlying mechanisms to explain the observed health benefits also require further study in humans, with blood samples, tissue biopsies, and complementary cell work providing promising avenues for exploration of changes in protein abundance, gene transcription, and downstream targets. In addition, more work is needed examining passive heat in at-risk and understudied populations, including obese women with or without PCOS, and those at increased cardiometabolic risk but with limited ability to gain the full benefits of exercise, such as individuals living with spinal cord injury.
Abbreviations
AT2 | = | angiotensin II receptor subtype 2 |
HIF1α | = | hypoxia inducible factor 1α |
HSP | = | heat shock protein |
IKKβ | = | inhibitor of kappa B kinase β |
IL-6 | = | interleukin-6 |
IRS | = | insulin receptor substrate |
JNK | = | c-Jun NH2-terminal kinase |
NO | = | nitric oxide |
PCOS | = | polycystic ovary syndrome |
SNS | = | sympathetic nervous system |
TNFα | = | tumor necrosis factor α |
VEGF | = | vascular endothelial growth factor |
Disclosure of potential conflicts of interest
No potential conflicts of interest were disclosed.
Additional information
Funding
References
- Swinburn BA, Sacks G, Hall KD, McPherson K, Finegood DT, Moodie ML, Gortmaker SL. The global obesity pandemic: Shaped by global drivers and local environments. Lancet. 2011;378(9793):804–14. doi:10.1016/S0140-6736(11)60813-1. PMID:21872749
- Ogden CL, Carroll MD, Kit BK, Flegal KM. Prevalence of obesity among adults: United States, 2011–2012. NCHS Data Brief. 2013;131(131):1–8.
- Huxley R. Excess risk of fatal coronary heart disease associated with diabetes in men and women: Meta-analysis of 37 prospective cohort studies. BMJ. 2006;332(7533):73–8. doi:10.1136/bmj.38678.389583.7C. PMID:16371403
- Burgess E, Hassmen P, Welvaert M, Pumpa KL. Behavioural treatment strategies improve adherence to lifestyle intervention programmes in adults with obesity: A systematic review and meta-analysis. Clin Obes. 2017;7(2):105–14. doi:10.1111/cob.12180. PMID:28199047
- Buchwald H, Avidor Y, Braunwald E, Jensen MD, Pories W, Fahrbach K, Schoelles K. Bariatric surgery: A systematic review and meta-analysis. Surg. 2004;292(14):1724–37.
- Sjostrom L, Lindroos A-K, Peltonen M, Torgerson J, Bouchard C, Carlsson B, Dahlgren S, Larsson B, Narbro K, Sjostrom C, et al. New England Journal of Medicine. Group. 2004;351(26):2683–93.
- Williamson R. On the treatment of glycosuria and diabetes mellitus with sodium salicylate. Br J Med. 1901;1(2100):760–2. doi:10.1136/bmj.1.2100.760. PMID:20759517
- Nanji AA, Freeman JB, Nair G. Postoperative leukocytosis in morbidly obese patients: Relationship to serum cholesterol. Am J Hematol. 1985;20(4):417–8. doi:10.1002/ajh.2830200415. PMID:4073016
- Hotamisligil GS, Shargill NS, Spiegelman BM. Adipose expression of tumor necrosis factor-α : Direct role in obesity-linked insulin resistance. Science.2017;259(5091):87–91.
- Berg AH, Scherer PE. Adipose tissue, inflammation, and cardiovascular disease. Circ Res. 2005;96:939–49. doi:10.1161/01.RES.0000163635.62927.34. PMID:15890981
- Nicoletti G, Giugliano G, Pontillo A, Cioffi M, D?Andrea F, Giugliano D, Esposito K. Effect of a multidisciplinary program of weight reduction on endothelial functions in obese women. J Endocrinol Invest. 2003;26:RC5–8. doi:10.1007/BF03345154. PMID:12809165
- Heilbronn L, Noakes M, Clifton P. Energy restriction and weight loss on very-low-fat diets healthy women. Arterioscler Thrombpsisn Vasc Biol. 2001;21:968–70. doi:10.1161/01.ATV.21.6.968. PMID:11397705
- Yuan M, Konstantopoulos N, Lee J, Hansen L, Li Z, Karin M, Shoelson S. Reversal of obesity- and diet-induced insulin resistance with salicylates or targeted disruption of IkkB. Science (80-). 2001;293:1673–7. doi:10.1126/science.1061620.
- Trayhurn P, Wang B, Wood IS. Hypoxia in adipose tissue: A basis for the dysregulation of tissue function in obesity? Br J Nutr. 2008;100(2):227–35. doi:10.1017/S0007114508971282. PMID:18397542
- Ye J. Emerging role of adipose tissue hypoxia in obesity and insulin resistance. Int J Obes. 2009;33(1):54–66. doi:10.1038/ijo.2008.229. PMID:19050672
- Osborn O, Olefsky JM. The cellular and signaling networks linking the immune system and metabolism in disease. Nat Med. 2012;18(3):363–74. doi:10.1038/nm.2627. PMID:22395709
- Cinti S. Adipocyte death defines macrophage localization and function in adipose tissue of obese mice and humans. J Lipid Res. 2005;46(11):2347–55. doi:10.1194/jlr.M500294-JLR200. PMID:16150820
- Zierath JR, Livingston JN, Thorne A, Bolinder J, Reynisdottir S, Lonnqvist F, Arner P. Regional difference in insulin inhibition of non-esterified fatty acid release from human adipocytes: Relation to insulin receptor phosphorylation and intracellular signalling through the insulin receptor substrate-1 pathway. Diabetologia. 1998;41:1343–54. doi:10.1007/s001250051075. PMID:9833943
- Koves TR, Ussher JR, Noland RC, Slentz D, Mosedale M, Ilkayeva O, Bain J, Stevens R, Dyck JR, Newgard CB, et al. Mitochondrial overload and incomplete fatty acid oxidation contribute to skeletal muscle insulin resistance. Cell Metab. 2008;7(1):45–56. doi:10.1016/j.cmet.2007.10.013. PMID:18177724
- Nguyen MTA, Satoh H, Favelyukis S, Babendure JL, Imamura T, Sbodio JI, Zalevsky J, Dahiyat BI, Chi NW, Olefsky JM. JNK and tumor necrosis factor-α mediate free fatty acid-induced insulin resistance in 3T3-L1 adipocytes. J Biol Chem. 2005;280(42):35361–71. doi:10.1074/jbc.M504611200. PMID:16085647
- Arkan MC, Hevener AL, Greten FR, Maeda S, Li Z, Long JM, Wynshaw-boris A, Poli G, Olefsky J, Karin M. IKK- β links inflammation to obesity-induced insulin resistance. Nat Med. 2005;11(2):191–8.
- Yin M-J, Yamamoto Y, Gaynor RB. The anti-inflammatory agents aspirin and salicylate inhibit the activity of I(kappa)B kinase-beta. Nature. 1998;396(6706):77–80. doi:10.1038/23948. PMID:9817203
- Galgani JE, Moro C, Ravussin E. Metabolic flexibility and insulin resistance. Am J Physiol Endocrinol Metab. 2008;295(5):E1009–17. doi:10.1152/ajpendo.90558.2008. PMID:18765680
- Jung UJ, Choi M-S. Obesity and its metabolic complications: The role of adipokines and the relationship between obesity, inflammation, insulin resistance, dyslipidemia and nonalcoholic fatty liver disease. Int J Mol Sci. 2014;15(4):6184–223. doi:10.3390/ijms15046184. PMID:24733068
- Ouchi N, Kihara S, Funahashi T, Matsuzawa Y, Walsh K. Obesity, adiponectin and vascular inflammatory disease. Curr Opin Lipidol. 2003;14(6):561–6. doi:10.1097/00041433-200312000-00003. PMID:14624132
- Díez JJ, Iglesias P. The role of the novel adipocyte-derived hormone adiponectin in human disease. Eur J Endocrinol. 2003;148(3):293–300. doi:10.1530/eje.0.1480293. PMID:12611609
- Ohashi K, Parker JL, Ouchi N, Higuchi A, Vita JA, Gokce N, Pedersen AA, Kalthoff C, Tullin S, Sams A, et al. Adiponectin promotes macrophage polarization toward an anti-inflammatory phenotype. J Biol Chem. 2010;285(9):6153–60. doi:10.1074/jbc.M109.088708. PMID:20028977
- Facchini FS, Hua N, Abbasi F, Reaven GM. Insulin resistance as a predictor of age-related diseases. J Clin Endocrinol Metab. 2001;86(8):3574–8. doi:10.1210/jcem.86.8.7763. PMID:11502781
- Smith MM, Minson CT. Obesity and adipokines: Effects on sympathetic overactivity. J Physiol. 2012;590(8):1787–801. doi:10.1113/jphysiol.2011.221036. PMID:22351630
- Marz P, Gadient RA, Otten U. Expression of interleukin-6 receptor (IL-6R) and gp130 mRNA in PC12 cells and sympathetic neurons: Modulation by tumor necrosis factor α (TNF-α). Brain Res. 1996;706(1):71–79. doi:10.1016/0006-8993(95)01210-9. PMID:8720493
- Gadient RA, Otten U. Postnatal expression of interleukin-6 (IL-6) and IL-6 receptor (IL-6R) mRNAs in rat sympathetic and sensory ganglia. Brain Res. 1996;724(1):41–46. doi:10.1016/0006-8993(96)00264-8. PMID:8816254
- Torpy DJ, Papanicolaou DA, Lotsikas AJ, Wilder RL, Chrousos GP, Pillemer SR. Responses of the sympathetic nervous system and the hypothalamic-pituitary-adrenal axis to interleukin-6: A pilot study in fibromyalgia. Arthritis Rheum. 2000;43(4):872. doi:10.1002/1529-0131(200004)43:4<872::AID-ANR19>3.0.CO;2-T. PMID:10765933
- Roytblat L, Rachinsky M, Fisher A, Greemberg L, Shapira Y, Douvdevani A, Gelman S. Raised interleukin-6 levels in obese patients. Obes Res. 2000;8(9):673–5. doi:10.1038/oby.2000.86. PMID:11225716
- Tzanavari T, Giannogonas P, Karalis KP. TNF-alpha and obesity. Curr Dir Autoimmun. 2010;11:145–56. doi:10.1159/000289203. PMID:20173393
- Anderson EA, Balon TW, Hoffman RP, Sinkey CA, Mark AL. Insulin increases sympathetic activity but not blood pressure in borderline hypertensive humans. Hypertension. 1992;19(6):621–6. doi:10.1161/01.HYP.19.6.621. PMID:1592458
- Rowe JW, Young JB, Minaker KL, Stevens AL, Pallotta J, Landsberg L. Effect of insulin and glucose infusions on sympathetic nervous system activity in normal man. Diabetes. 1981;30(3):219–25. http://diabetes.diabetesjournals.org/content/30/3/219. doi:10.2337/diab.30.3.219. PMID:7009270
- Landsberg L. Insulin and the sympathetic nervous system in the pathophysiology of hypertension. Blood Press Suppl. 1996;1:25–29. http://www.ncbi.nlm.nih.gov/pubmed/9162434. PMID:9162434
- Malpas SC. Sympathetic nervous system overactivity and its role in the development of cardiovascular disease. Physiol Rev. 2010;90(2):513–57. doi:10.1152/physrev.00007.2009. PMID:20393193
- Lambert E, Sari CI, Dawood T, Nguyen J, McGrane M, Eikelis N, Chopra R, Wong C, Chatzivlastou K, Head G, et al. Sympathetic nervous system activity is associated with obesity-induced subclinical organ damage in young adults. Hypertension. 2010;56(3):351–8. doi:10.1161/HYPERTENSIONAHA.110.155663. PMID:20625075
- Steinberg HO, Chaker H, Leaming R, Johnson A, Brechtel G, Baron AD. Obesity/insulin resistance is associated with endothelial dysfunction. Implications for the syndrome of insulin resistance. J Clin Invest. 1996;97(11):2601–10. doi:10.1172/JCI118709. PMID:8647954
- Scherrer U, Sartori C. Defective nitric oxide synthesis: A link between metabolic insulin resistance, sympathetic overactivity and cardiovascular morbidity. Eur J Endocrinol. 2000;142(4):315–23. doi:10.1530/eje.0.1420315. PMID:10754469
- Laakso M, Edelman SV, Brechtel G, Baron AD. Decreased effect of insulin to stimulate skeletal muscle blood flow in obese man. A novel mechanism for insulin resistance. J Clin Invest. 1990;85(6):1844–52. doi:10.1172/JCI114644. PMID:2189893
- Williams I, Wheatcroft S, Shah A, Kearney M. Obesity, atherosclerosis and the vascular endothelium: Mechanisms of reduced nitric oxide bioavailability in obese humans. Int J Obes Relat Metab Disord. 2002;26:754–64. doi:10.1038/sj.ijo.0801995. PMID:12037644
- Safar ME, Czernichow S, Blacher J. Obesity, arterial stiffness, and cardiovascular risk. J Am Soc Nephrol. 2006;17(4 Suppl 2):S109–11. doi:10.1681/ASN.2005121321. PMID:16565231
- Dalmas E, Kahn J-F, Giral P, Abdennour M, Bouillot JL, Fellahi S, Oppert JM, Clément K, Guerre-Millo M, Poitou C. Intima-media thickness in severe obesity links with BMI and metabolic status but not with systemic or adipose tissue inflammation. Diabetes Care. 2013;36:3793–802. doi:10.2337/dc13-0256. PMID:24062328
- Magnussen CG, Venn A, Thomson R, Juonala M, Srinivasan SR, Viikari JSA, Berenson GS, Dwyer T, Raitakari OT. The association of pediatric low- and high-density lipoprotein cholesterol dyslipidemia classifications and change in dyslipidemia status with carotid intima-media thickness in adulthood. J Am Coll Cardiol. 2009;53(10):860–9. doi:10.1016/j.jacc.2008.09.061. PMID:19264243
- Wild RA, Carmina E, Diamanti-Kandarakis E, Dokras A, Escobar-Morreale HF, Futterweit W, Lobo R, Norman RJ, Talbott E, Dumesic DA. Assessment of cardiovascular risk and prevention of cardiovascular disease in women with the polycystic ovary syndrome: A consensus statement by the androgen excess and polycystic ovary syndrome (AE-PCOS) society. J Clin Endocrinol Metab. 2010;95(5):2038–49. doi:10.1210/jc.2009-2724. PMID:20375205
- Luque-Ramírez M, Escobar-Morreale HF. Polycystic ovary syndrome as a paradigm for prehypertension, prediabetes, and preobesity. Curr Hypertens Rep. 2014;16(12):1–10. doi:10.1007/s11906-014-0500-6.
- Lansdown A, Rees DA. The sympathetic nervous system in polycystic ovary syndrome: A novel therapeutic target? Clin Endocrinol (Oxf). 2012;77(6):791–801. doi:10.1111/cen.12003. PMID:22882204
- Ribiero VB, Kogure GS, Reis RM, Gastaldi ADAC, De Araujo JE, Mazon JH, Borghi A, Souza HCD. Polycystic ovary syndrome presents higher sympathetic cardiac autonomic modulation that is not altered by strength training. Int J Exerc Sci. 2016;9(5):554–66. PMID:27990221
- Horowitz M. Heat acclimation, epigenetics, and cytoprotection memory. Compr Physiol. 2014;4(1):199–230. doi:10.1002/cphy.c130025. PMID:24692139
- Horowitz M, Assadi H. Heat acclimation-mediated cross-tolerance in cardioprotection: Do HSP70 and HIF-1α play a role? Ann N Y Acad Sci. 2010;1188:199–206. doi:10.1111/j.1749-6632.2009.05101.x. PMID:20201904
- Maloyan A, Eli-Berchoer L, Semenza GL, Gerstenblith G, Stern MD, Horowitz M. HIF-1alpha-targeted pathways are activated by heat acclimation and contribute to acclimation-ischemic cross-tolerance in the heart. Physiol Genomics. 2005;23(1):79–88. doi:10.1152/physiolgenomics.00279.2004. PMID:16046617
- Stice J, Knowlton A. Estrogen, NFkappaB, and the heat shock response. Mol Med. 2008;14(7–8):517–27. doi:10.2119/2008-00026.Stice. PMID:18431462
- Morera P, Basiricò L, Hosoda K, Bernabucci U. Chronic heat stress up-regulates leptin and adiponectin secretion and expression and improves leptin, adiponectin and insulin sensitivity in mice. J Mol Endocrinol. 2012;48(2):129–38. doi:10.1530/JME-11-0054. PMID:22279186
- Ely BR, Lovering AT, Horowitz M, Minson CT. Heat acclimation and cross tolerance to hypoxia. Temperature (Austin). 2014;1(2):107–14. doi:10.4161/temp.29800. PMID:27583292
- Brunt VE, Jeckell AT, Ely BR, Howard MJ, Thijssen DHJ, Minson CT. Acute hot water immersion is protective against impaired vascular function following forearm ischemia-reperfusion in young healthy humans. Am J Physiol–Regul Integr Comp Physiol. 2016;311(6):R1060–7. doi:10.1152/ajpregu.00301.2016. PMID:27707723
- Heled Y, Peled A, Yanovich R, Shargal E, Pilz-Burstein R, Epstein Y, Moran DS. Heat acclimation and performance in hypoxic conditions. Aviat Sp Environ Med. 2012;83(7):649–53. doi:10.3357/ASEM.3241.2012. PMID:22779306
- White AC, Salgado RM, Astorino TA, Loeppky JA, Schneider SM, McCormick JJ, McLain TA, Kravitz L, Mermier CM. The effect of 10 days of heat acclimation on exercise performance in acute hypobaric hypoxia (4350 m). Temperature. 2016;3(1):176–85. doi:10.1080/23328940.2015.1072659.
- Kuhlenhoelter AM, Kim K, Neff D, Nie Y, Blaize AN, Wong BJ, Kuang S, Stout J, Song Q, Gavin TP, et al. Heat therapy promotes the expression of angiogenic regulators in human skeletal muscle. Am J Physiol Regul Integr Comp Physiol. 2016;311(2):R377–91. http://ajpregu.physiology.org/content/311/2/R377.full.pdf+html. doi:10.1152/ajpregu.00134.2016
- Averna M, Stifanese R, De Tullio R, Passalacqua M, Salamino F, Pontremoli S, Melloni E. Functional role of HSP90 complexes with endothelial nitric-oxide synthase (eNOS) and calpain on nitric oxide generation in endothelial cells. J Biol Chem. 2008;283(43):29069–76. doi:10.1074/jbc.M803638200. PMID:18682401
- Papapetropoulos A, García-Cardeña G, Madri JA, Sessa WC. Nitric oxide production contributes to the angiogenic properties of vascular endothelial growth factor in human endothelial cells. J Clin Invest. 1997;100(12):3131–9. doi:10.1172/JCI119868. PMID:9399960
- Thomas KN, van Rij AM, Lucas SJE, Cotter JD. Lower-limb hot-water immersion acutely induces beneficial hemodynamic and cardiovascular responses in peripheral arterial disease and healthy, elderly controls. Am J Physiol–Regul Integr Comp Physiol. 2017;312(3):R281–91. doi:10.1152/ajpregu.00404.2016. PMID:28003211
- Faulkner SH, Jackson S, Fatania G, Leicht CA. The effect of passive heating on heat shock protein 70 and interleukin-6 : A possible treatment tool for metabolic diseases? Temperature. 2017;4(3):xxx–xxx. doi:10.1080/23328940.2017.1288688.
- Moon B, Duddy N, Ragolia L, Begum N. Stimulation of glycogen synthesis by heat shock in L6 skeletal-muscle cells: Regulatory role of site-specific phosphorylation of glycogen-associated protein phosphatase 1. Biochem J. 2003;371:857–66. https://www.ncbi.nlm.nih.gov/pmc/articles/PMC1223329/pdf/12540292.pdf. doi:10.1042/bj20021644. PMID:12540292
- Gupte AA, Bomhoff GL, Swerdlow RH, Geiger PC. Heat treatment improves glucose tolerance and prevents skeletal muscle insulin resistance in rats fed a high-fat diet. Diabetes. 2009;58:567–78. doi:10.2337/db08-1070. PMID:19073766
- Cardillo MR, Ippolito F. Interleukin-6, interleukin-10 and heat shock protein-90 expression in renal epithelial neoplasias and surrounding normal-appearing renal parenchyma. Int J Immunopathol Pharmacol. 2007;20(1):37–46. doi:10.1177/039463200702000105. PMID:17346426
- Dokladny K, Lobb R, Wharton W, Ma TY, Moseley PL. LPS-induced cytokine levels are repressed by elevated expression of HSP70 in rats: Possible role of NF-kappaB. Cell Stress Chaperones. 2010;15(2):153–63. doi:10.1007/s12192-009-0129-6. PMID:19551494
- Kern PA, Ranganathan S, Li C, Wood L, Ranganathan G. Adipose tissue tumor necrosis factor and interleukin-6 expression in human obesity and insulin resistance. Am J Physiol–Endocrinol Metab. 2001;280(5):E745–51. http://ajpendo.physiology.org/content/280/5/E745. PMID:11287357
- Hotamisligil GS, Budavari A, Murray D, Spiegelman BM. Reduced tyrosine kinase activity of the insulin receptor in obesity-diabetes. Central role of tumor necrosis factor-alpha. J Clin Invest. 1994;94(4):1543–9. doi:10.1172/JCI117495. PMID:7523453
- Greenberg A, Nurdan RP, Mclntosh J, Carlos Calvo J, Scow RO, Jablons D. Interleukin 6 reduces lipoprotein lipase activity in adipose tissue of mice in vivo and in 3T3-L1 adipocytes: A possible role for interleukin 6 in cancer cachexia. Cancer Res. 1992;52:4113–6. PMID:1638523
- Masharani UB, Maddux BA, Li X, Sakkas GK, Mulligan K, Schambelan M, Goldfine ID, Youngren JF. Insulin resistance in non-obese subjects is associated with activation of the JNK pathway and impaired insulin signaling in skeletal muscle. Federici M, ed. PLoS One. 2011;6(5):e19878. doi:10.1371/journal.pone.0019878. PMID:21589939
- Hirosumi J, Tuncman G, Chang L, Gorgun CZ, Uysal KT, Maeda K, Karin M, Hotamisligil GS. A central role for JNK in obesity and insulin resistance. Nature. 2002;420(6913):333–6. doi:10.1038/nature01137. PMID:12447443
- Bruce CR, Carey AL, Hawley JA, Febbraio MA. Intramuscular heat shock protein 72 and heme oxygenase-1 mRNA are reduced in patients with type 2 diabetes: Evidence that insulin resistance is associated with a disturbed antioxidant defense mechanism. Diabetes. 2003;52(9):2338–45. doi:10.2337/diabetes.52.9.2338. PMID:12941774
- Hooper PL, Hooper PL. Inflammation, heat shock proteins, and type 2 diabetes. Cell Stress Chaperones. 2009;14(2):113–5. doi:10.1007/s12192-008-0073-x. PMID:18720028
- Dokladny K, Lobb R, Wharton W, Ma TY, Moseley PL. LPS-induced cytokine levels are repressed by elevated expression of HSP70 in rats: Possible role of NF-?B. Cell Stress Chaperones. 2010;15(2):153–63. doi:10.1007/s12192-009-0129-6. PMID:19551494
- Zachayus JL, Benatmane S, Plas C. Role of Hsp70 synthesis in the fate of the insulin-receptor complex after heat shock in cultured fetal hepatocytes. J Cell Biochem. 1996;61(2):216–29. doi:10.1002/(SICI)1097-4644(19960501)61:2<216::AID-JCB5>3.0.CO;2-X. PMID:9173085
- Liu C-T, Brooks GA. Mild heat stress induces mitochondrial biogenesis in C2C12 myotubes. J Appl Physiol. 2012;112(3):354–61. doi:10.1152/japplphysiol.00989.2011. PMID:22052865
- Osmond JM, Mintz JD, Dalton B, Stepp DW. Vascular remodeling, and severity of stroke in the. Vascular. 2010;53(2):381–6.
- Canale MP, Manca Di Villahermosa S, Martino G, Rovella V, Noce A, De Lorenzo A, Di Daniele N. Obesity-related metabolic syndrome: Mechanisms of sympathetic overactivity. Int J Endocrinol. 2013;2013:865965. doi:10.1155/2013/865965. PMID:24288531
- Carter HH, Spence AL, Atkinson CL, Pugh CJA, Naylor LH, Green DJ. Repeated core temperature elevation induces conduit artery adaptation in humans. Eur J Appl Physiol. 2014;114(4):859–65. doi:10.1007/s00421-013-2817-2. PMID:24399113
- Thomas KN, van Rij AM, Lucas SJE, Gray AR, Cotter JD. Substantive hemodynamic and thermal strain upon completing lower-limb hot-water immersion; comparisons with treadmill running. Temperature (Austin). 2016;3(2):286–97. doi:10.1080/23328940.2016.1156215. PMID:27857958
- Tinken TM, Thijssen DHJ, Hopkins N, Black MA, Dawson EA, Minson CT, Newcomer SC, Laughlin MH, Cable NT, Green DJ. Impact of shear rate modulation on vascular function in humans. Hypertension. 2009;54:278–85. doi:10.1161/HYPERTENSIONAHA.109.134361. PMID:19546374
- Laughlin MH, Newcomer SC, Bender SB. Importance of hemodynamic forces as signals for exercise-induced changes in endothelial cell phenotype. J Appl Physiol. 2008;104(3):588–600. doi:10.1152/japplphysiol.01096.2007. PMID:18063803
- Neff D, Kuhlenhoelter AM, Lin C, Wong BJ, Motaganahalli RL, Roseguini BT. Thermotherapy reduces blood pressure and circulating endothelin-1 concentration and enhances leg blood flow in patients with symptomatic peripheral artery disease. Am J Physiol Regul Integr Comp Physiol. 2016;311(2):R391–400. doi:10.1152/ajpregu.00147.2016.
- Connolly EM, Kelly CJ, Chen G, O'Grady T, Kay E, Leahy A, Bouchier-Hayes DJ. Pharmacological induction of HSP27 attenuates intimal hyperplasia in vivo. Eur J Vasc Endovasc Surg. 2003;25(1):40–47. doi:10.1053/ejvs.2002.1793. PMID:12525810
- Zheng Y, Im C-N, Seo J-S. Inhibitory effect of Hsp70 on angiotensin II-induced vascular smooth muscle cell hypertrophy. Exp Mol Med. 2006;38(5):509–18. doi:10.1038/emm.2006.60. PMID:17079867
- Kim I, Shin H-M, Baek W. Heat-shock response is associated with decreased production of interleukin-6 in murine aortic vascular smooth muscle cells. Naunyn Schmiedebergs Arch Pharmacol. 2005;371(1):27–33. doi:10.1007/s00210-004-1007-5. PMID:15655672
- Tousoulis D, Kampoli AM, Tentolouris C, Papageorgiou N, Stefanadis C. The role of nitric oxide on endothelial function. Curr Vasc Pharmacol. 2012;10(1):4–18. doi:10.2174/157016112798829760. PMID:22112350
- Bharati J, Dangi SS, Bag S, Maurya VP, Singh G, Kumar P, Sarkar M. Expression dynamics of HSP90 and nitric oxide synthase (NOS) isoforms during heat stress acclimation in Tharparkar cattle. Int J Biometeorol. 2017;61(8):1461–9 ]. doi:10.1007/s00484-016-1281-1 10.1007/s00484-017-1323-3. PMID:28265771
- Harris MB, Mitchell BM, Sood SG, Webb RC, Venema RC. Increased nitric oxide synthase activity and Hsp90 association in skeletal muscle following chronic exercise. Eur J Appl Physiol. 2008;104(5):795–802. doi:10.1007/s00421-008-0833-4. PMID:18784937
- Brunt VE, Howard MJ, Francisco MA, Ely BR, Minson CT. Passive heat therapy improves endothelial function, arterial stiffness and blood pressure in sedentary humans. J Physiol. 2016;594(18):5329–42. doi:10.1113/JP272453. PMID:27270841
- Brunt VE, Eymann TM, Francisco MA, Howard MJ, Minson CT. Passive heat therapy improves cutaneous microvascular function in sedentary humans via improved nitric oxide-dependent dilation. J Appl Physiol. 2016;121(3):716–23. doi:10.1152/japplphysiol.00424.2016. PMID:27418688
- Kraemer-Aguiar LG, Laflor CM, Bouskela E. Skin microcirculatory dysfunction is already present in normoglycemic subjects with metabolic syndrome. Metabolism. 2008;57(12):1740–6. doi:10.1016/j.metabol.2008.07.034. PMID:19013299
- Tanida M, Shen J, Horii Y, Matsuda M, Kihara S, Funahashi T, Shimomura I, Sawai H, Fukuda Y, Matsuzawa Y, et al. Effects of adiponectin on the renal sympathetic nerve activity and blood pressure in rats. Exp Biol Med (Maywood). 2007;232(3):390–7. PMID:17327472
- Wong PC, Bernard R, Timmermans PBMWM. Effect of blocking angiotensin II receptor subtype on rat sympathetic nerve function. Hypertension. 1992;19:663–7. doi:10.1161/01.HYP.19.6.663. PMID:1592464
- Patel KP, Li Y-F, Hirooka Y. Role of nitric oxide in central sympathetic outflow. Exp Biol Med (Maywood). 2001;226(9):814–24. doi:10.1177/153537020122600902. PMID:11568303
- Porte D. A receptor mechanism for the inhibition of insulin release by epinephrine in man. J Clin Invest. 1967;46(1):86–94. doi:10.1172/JCI105514. PMID:6018752
- Pritchard KA. Heat shock protein 90 mediates the balance of nitric oxide and superoxide anion from endothelial nitric-oxide synthase. J Biol Chem. 2001;276(21):17621–4. doi:10.1074/jbc.C100084200. PMID:11278264
- Horowitz M, Kaspler P, Simon E, Gerstberger R. Heat acclimation and hypohydration: Involvement of central angiotensin II receptors in thermoregulation. Am J Physiol. 1999;277(1 Pt 2):R47–55. PMID:10409257
- Abdulla MH, Johns EJ. The role of brain angiotensin II (type 2) receptors and nitric oxide in the renal sympathoinhibitory response to acute volume expansion in conscious rats. J Hypertens. 2017;35(2):338–47. doi:10.1097/HJH.0000000000001154. PMID:27820727
- Hooper PL. Hot-tub therapy for type 2 diabetes mellitus. N Engl J Med. 1992;327(10):742–7. PMID:1495543
- Kondo T, Ono K, Kitano S, Matsuyama R, Goto R, Suico MA, Kawasaki S, Igata M, Kawashima J, Motoshima H, et al. Mild electrical stimulation with heat shock reduces visceral adiposity and improves metabolic abnormalities in subjects with metabolic syndrome or type 2 diabetes: Randomized crossover trials. EBioMedicine. 2014;1(1):80–89. doi:10.1016/j.ebiom.2014.11.001. PMID:26137510
- Leppaluoto J. Leppaluoto human thermoregulation in sauna.pdf. Ann Clin Res. 1988;20:240–3. PMID:3218894
- Imamura M, Biro S, Kihara T, Yoshifuku S. Repeated thermal therapy improves impaired vascular endothelial function in patients with coronary risk factors. J Am Coll Cardiol. 2001;38(4):1083–8. doi:10.1016/S0735-1097(01)01467-X. PMID:11583886
- Kihara T, Biro S, Imamura M, Yoshifuku S. Repeated sauna treatment improves vascular endothelial and cardiac function in patients with chronic heart failure. J Am Coll Cardiol. 2002;39(5):754–9. doi:10.1016/S0735-1097(01)01824-1. PMID:11869837
- Laukkanen T, Khan H, Zaccardi F, Laukkanen JA. Association between sauna bathing and fatal cardiovascular and all-cause mortality events. JAMA Intern Med. 2015;175(4):E1–E6. doi:10.1001/jamainternmed.2014.8187. PMID:26030724
- Pate JL, Buono MJ. The physiological responses to Bikram yoga in novice and experienced practitioners. Altern Ther Health Med. 2014;20(4):12–18. PMID:25141359
- Quandt E, Porcari JP, Steffen J, Felix M, Foster C. Heart rate and core temperature responses to Bikram yoga. Gunderson Med J. 2015;9(1):7–11.
- Hunter SD, Dhindsa M, Cunningham E, Tarumi T, Alkatan M, Tanaka H. Improvements in glucose tolerance with Bikram yoga in older obese adults: A pilot study. J Bodyw Mov Ther. 2013;17(4):404–7. doi:10.1016/j.jbmt.2013.01.002. PMID:24138995
- Hunter SD, Dhindsa MS, Cunningham E, Tarumi T, Alkatan M, Nualnim N, Tanaka H. The effect of Bikram yoga on arterial stiffness in young and older adults. J Altern Complement Med. 2013;19(12):930–4. doi:10.1089/acm.2012.0709. PMID:23738677
- Guo YH, Wang F, Hu JP, Wang Y, Zhang LY. Effect of high temperature yoga exercise on improving physical and mental well-being of overweight middle-aged and young women. Int J Clin Exp Med. 2014;7(12):5842–6. PMID:25664117
- Withrow D, Alter DA. The economic burden of obesity worldwide: A systematic review of the direct costs of obesity. Obes Rev. 2011;12(2):131–41. doi:10.1111/j.1467-789X.2009.00712.x. PMID:20122135