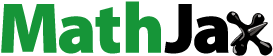
ABSTRACT
We investigated whether and how multiple sclerosis (MS) alters thresholds for perceiving increases and decreases in local skin temperature, as well as the sensitivity to progressively greater temperature stimuli, amongst heat-sensitive people with MS. Eleven MS patients (5 M/6 F; 51.1 ± 8.6 y, EDSS 5.7 ± 1.9) and 11 healthy controls (CTR; 7 M/4 F; 50.3 ± 9.0 y) performed warm and cold threshold tests on a hairy skin site, on both sides of the body. They also underwent a thermosensitivity test where they rated (visual analogue scale) perceived magnitude of 4 local skin stimuli (i.e. 22, 26, 34, 38°C). Individual thresholds and slopes of linear regression for thermosensitivity were z-transformed for each MS patient, and used to determine individual thermosensory abnormalities. When considering both threshold and thermosensitivity, six out of our 11 heat-sensitive patients (54.5%) exhibited skin thermosensory abnormalities. Those abnormalities varied amongst patients in terms of type (threshold vs. thermosensitivity), quality (warm vs. cold), location (left vs. right side of the body) and extent. Each of those six patients presented unique thermosensory profiles. While some patients experienced thermosensory loss in both thresholds and sensitivity and on both sides of the body, others experienced cold thermosensory loss on one side of the body only. The observed individual variability in thermosensory function among heat-sensitive MS patients highlight the need for a patient-centered approach to assessing thermosensory dysfunction and its potential implications for heat stress vulnerability in this patient group.
Introduction
Multiple sclerosis (MS) is an autoimmune neurodegenerative disease characterized by demyelination of the central nervous system, and by a plethora of sensory, motor, cognitive, and autonomic symptoms [Citation1]. There is no cure for MS, and with an increasing 2.5 million people affected worldwide, this disease represents a significant public health challenge [Citation2].
A still little understood signature of MS is the highly prevalent heat sensitivity of its sufferers, where over 60% of patients experience neurological symptom worsening as a result of exercise- and environmental-induced increases in body temperature [Citation3,Citation4]. MS heat sensitivity is a physiological phenomenon, which is primarily driven by temperature-dependent slowing/blocking of neural conduction in demyelinated nerves within the central nervous system due to changes in deep core temperature [Citation4].
Recently, our group has demonstrated that heat sensitivity can trigger a worsening of afferent thermosensory function, i.e. the ability to sense thermal stimuli on the skin, in MS patients [Citation5]. Sensory symptoms are highly prevalent in MS and they represent the first manifestation of the disease in up to 25% of MS cases [Citation6]. Our previous findings indicated that sensory function can be worsened by ~10% by exercise-induced increases in mean body temperature (i.e. weighted mean of core and skin temperatures) as little as of 0.4°C in relapsing-remitting MS patients [Citation5].
In healthy humans, behavioral responses to increases in body temperatures (e.g. changes in clothing insulation, seeking for shade, drinking, etc.) are engaged well in advance of energy-demanding physiological thermoregulatory responses and are key to support thermal resilience [Citation7]. Better understanding how thermosensory function is impacted in heat-sensitive MS patients is therefore important not only to elucidate the characteristics of MS-induced sensory symptoms, but also, to determine the impact of thermosensory deficits on behavioral thermoregulation in those heat-sensitive MS patients who already present a lower resilience to heat stress [Citation8].
Afferent inputs from warm- and cold-sensitive thermoreceptors innervating the skin contribute to the conscious perception of temperature stimuli, i.e. thermal sensations [Citation9], and provide the perceptual drive to trigger thermoregulatory behaviors [Citation10]. Two objective measures define the performance of the afferent thermosensory pathway sub-serving thermal sensations, i.e. the threshold (i.e. smallest amount of temperature change that can be perceived on the skin) and sensitivity (i.e. the ability to discriminate the magnitude of progressively greater temperature stimuli) to thermal stimuli [Citation9]. Assessing whether MS induces deficiencies in threshold for and sensitivity of thermal sensations provides an opportunity to characterize impairments in perceptual thermosensory pathways in heat-sensitive MS patients, which can be relevant to better understand the impact of MS-induced sensory deficits on thermoregulatory behaviors important for heat resilience (e.g. reducing clothing insulation when skin temperature increases).
While representing an important proof of concept, our previous study on afferent thermosensory function during heat stress in MS [Citation5] did not examine the threshold and sensitivity of afferent thermosensation in heat-sensitive MS patients and whether this is impaired under resting conditions. Furthermore, our previous study did not characterize individual differences in perceptual thermosensory deficits amongst those MS patients we tested. It is well known that MS varies greatly in presentation and disease course amongst patients [Citation11]; hence, individualized approaches to symptom evaluation are important as they can provide insights into patient-specific abnormalities, which could be indicative of an increased individual vulnerability to certain stressors (e.g. a patient with pronounced perceptual thermosensory loss may be more vulnerable to heat stress than a patient that does not experience thermosensory abnormalities).
The aim of this study was twofold. First, we investigated individual abnormalities in thresholds for perceiving increases and decreases in local skin temperature amongst heat-sensitive MS patients. We hypothesized that, when compared to an age-matched healthy control group, some MS patients would present greater thresholds for detecting warm and cold stimuli on the skin, which would be indicative of a loss of thermosensory function. Second, we investigated abnormalities in the sensitivity to progressively greater temperature stimuli applied to the skin of the same heat-sensitive MS patients. We hypothesized that, when compared to an age-matched healthy control group, some MS patients would present blunted thermo-sensitivity, which would also be indicative of a loss of thermosensory function.
Methods
Eleven individuals diagnosed with MS and self-reporting heat sensitivity (MS group; 9 relapsing remitting MS, 1 primary and 1 secondary progressive MS; 5 M/6 F; 51.1 ± 8.6 y; 75.5 ± 11.7 kg; 173 ± 12.0 cm; Expanded Disability Status Scale (EDSS) score: 5.7 ± 1.9) and 11 age- and body size-matched, otherwise healthy control individuals (CTR group; 7 M/4 F; 50.3 ± 9.0 y; 77.3 ± 17.0 kg; 172 ± 10 cm), participated in this study. Seven MS participants reported one side of their body is more affected by the disease (5 left sides; 2 right sides). At the time of the study, 7 MS participants were being treated with the following disease-modifying treatments: Tysabri (natalizumab), n = 2; Copaxone (glatiramer acetate), n = 1; Avonex (interferon beta-1a), n = 1; Tecfidera (dimethyl fumarate), n = 2; Lioresal (Baclofen), n = 1. Lioresal is the only drug that might induce numbness or tingling as a side effect. All human testing procedures conformed to the Declaration of Helsinki and received University of Sydney and Loughborough University ethical approval. All participants provided written informed consent.
All participants took part in two experimental trials, separated by a minimum of 48 h, and performed at the same time of the day. During the first trial, participants underwent a thermal threshold detection test for both warm and cold temperatures and on both the left and right sides of the body. During the second trial, participants underwent a thermosensitivity test including both warm and cold temperatures on the left side of the body. Both assessments were based on the quantitative sensory tests we have previously developed and successfully tested in both healthy [Citation12,Citation13] and MS groups [Citation5]. All experiments were performed in an environmental chamber maintained at an ambient temperature of 25°C and 50% relative humidity, and between 7am and 12pm. Participants were required to avoid exercise the night before. Upon arrival at the laboratory, participants changed into t-shirt, running shorts and trainers and entered the environmental chamber. They then rested on a chair for 15 min to allow adaptation to the surrounding environment. During this time, participants were fully familiarized with the testing procedures.
To determine thermal thresholds for both cold and warm sensations on both the left and right side of the body, participants underwent 4 separate tests in a balanced order, with a 5-min break between each test. Thresholds were determined on both sides of the body to determine any laterality of sensory abnormalities, in line with patient’ self-reports of symptoms laterality, as well as from evidence on the impact of MS on the development of body region-specific clinical symptoms [Citation14]. We used a thermosensory analyzer (NTA-2, Physitemp, USA), consisting of a control unit connected to a 25 cm2 square thermal probe (thermode) to deliver local thermal stimuli to the hairy skin of the inner forearm of participants. Hairy skin was preferred over glabrous skin for testing, as it covers the majority of the body and it plays a key role in providing afferent inputs that are key for both behavioral and autonomic thermoregulatory responses [Citation9]. The probe’s contact surface could be set to a precision of 0.1°C within the operating range 15–42°C and was under manual control. Participants were first required to gently place either their left or right inner forearm (i.e. midpoint between antecubital fossa and wrist) over the thermally controlled surface of the thermode, which was placed on a table in front of them, facing upwards. The thermode’s baseline temperature was set to 30°C, to ensure that a thermoneutral sensation would be experienced at the beginning of the test [Citation13]. The participant was instructed to consider the local sensation experienced during this baseline phase as a reference sensation. At this point, a staircase procedure for threshold detection, as previously described [Citation13], was performed. First, when a warm threshold had to be determined, an up-step stimulus of 2°C from the starting temperature was delivered; depending on whether the participant detected or not such change, the successive stimulus was either 0.2°C smaller or greater than the first stimulus. Whenever a stimulus was detected, the following one would be 0.2°C smaller (i.e. down-step) until the participant no longer detected a change from the starting temperature. Whenever this occurred, a reversal in the direction of the following stimulus occurred (i.e. 0.2°C up-step), until the participant again detected a change from the starting temperature. A test ended whenever a participant moved between up- and down-steps 0.2°C apart six consecutive times. Participants reported verbally the detection of a stimulus and upon questioning from the investigator. The same procedure, however, reversed in the direction of temperature changes, was conducted to establish cold thresholds. The mean of six pairs of absolute temperatures at which the subject first sensed and then failed to sense was determined as the participant’s absolute threshold for that stimulus (i.e. warm or cold) and side of the body (i.e. left or right).
To determine the sensitivity in discriminating the magnitude of progressively greater temperature stimuli, participants underwent our recently developed quantitative sensory test [Citation5]. We used the same thermosensory analyzer used in the threshold test and placed this over the hairy skin of the back of the left hand of each participant. The probe was again set to a baseline temperature of 30°C, to ensure that a thermoneutral sensation would be experienced and participants were instructed to consider the local sensation experienced during this baseline phase as a reference sensation.
At this point, four temperature stimuli (i.e. 22, 26, 34, 38°C), each being either 4°C or 8°C above or below the baseline thermode’s temperature, were delivered in a balanced order and with 2-min breaks in between them. Upon delivery of a stimulus and obtainment of the target stimulus temperature, participants were required to report the perceived magnitude of their local thermal sensation used a hand-scored 200-mm visual analogue scale (VAS) [anchor points: Very Hot (200 mm) and Very Cold (0 mm); middle point: Neutral (100 mm)]. Once scoring of thermal sensation took place, the thermode was re-set to 30°C, and after a 2-min break, the next stimulus was delivered.
Threshold data were analyzed individually for each MS patient according to a z-transformation. This transformation allowed for the creation of thermosensory profiles for each individual patient, and for their subsequent assessment against normative data arising from the CTR group. This standardized approach is widely used in the context of assessing sensory loss in individual patients. For a detailed overview of the method, see Rolke et al. [Citation15,Citation16]. Analytical procedures used in this study are detailed below.
First, absolute threshold data for each MS and CTR participants were log-transformed (Log10). Second, log-transformed individual MS thresholds were z-transformed according to the following equation:
This transformation results in a sensory profile where thresholds are presented as standard normal distributions [zero mean, unit variance]. In line with 15, we adjusted the algebraic sign z score values for both cold and warm thresholds, so that it reflects the patient’s sensitivity to each thermal stimulus. Positive z scores indicate a gain of function, where the patient is more sensitive to the tested stimuli compared with controls; negative z scores indicate a loss of function referring to a lower sensitivity of the patient. Thus, elevations of threshold resulted in negative z scores, whereas reductions in thresholds resulted in positive z scores. Once the z transformation is performed, it is easy to compare individual MS patients’ sensory profiles with the group mean of the CTR group. Indeed, the 95% Confidence Interval of a standard normal distribution is given by the following equation:
Accordingly, if a threshold z score for an individual MS patient is >+1.96, then the patient exhibits gain of thermosensory function (i.e. their threshold is smaller than the 95%CI of the CTR group); on the contrary, if a z score for an individual MS patient is >-1.96, then the patient exhibits loss of thermosensory function (i.e. their threshold is greater than the 95%CI of the CTR group). Z scores were calculated and analyzed as above for both cold and warm temperatures, as well as for the left and right sides of the body, and proportions of patients showing thermosensory threshold abnormalities were reported.
Regarding thermosensitivity data, we first assessed the sensitivity to progressively greater thermal stimuli by means of linear regression for each individual MS patient, and tested (F test) whether those differed significantly amongst the 11 patients tested. Second, we assessed the sensitivity to progressively greater thermal stimuli by means of linear regression for each individual CTR patient and calculated the mean slope and standard deviation of the mean slope for the CTR group. Third, we used the mean slope and standard deviation of the mean slope of the CTR group, to z-transform the regression slopes of each individual MS patient, according to the following equation:
In line with that described for the threshold analysis, if a slope z score for an individual MS patient is >+1.96, then the patient exhibits hypersensitivity (i.e. their slope is greater than the 95%CI of the CTR group); on the contrary, if a slope z score for an individual MS patient is >-1.96, then the patient exhibits hyposensitivity (i.e. their slope is smaller than the 95%CI of the CTR group). Proportions of patients showing thermosensory sensitivity abnormalities were reported. In all analyses, p < 0.05 was used to establish statistically significant differences. Analyses were performed in GraphPad Prism 8.
Results
The absolute thermal thresholds for the CTR group were: 30.6 ± 0.7°C (warm – right), 30.5 ± 0.6°C (warm – left), 29.7 ± 0.3°C (cold – right), and 29.7 ± 0.3°C (cold – left). Regarding MS, we found that 5 out of 11 MS patients (45.4%) presented a loss of thermosensory function (i.e. greater thresholds than 95% CI of CTR group), which varied depending on body side and thermal quality of the stimuli (). Overall, this thermosensory loss appeared to be slightly more prevalent for cold than warm temperatures, and for the left than the right side of the body (i.e. proportion of patients with thermosensory loss: 36.4% cold – left & 27.3% cold – right; vs. 27.3% warm – left & 9.2% warm – right). Of these five patients, four exhibited thermosensory loss across more than one of the four thresholds tested; yet the combination of thermosensory loss varied amongst patients. For example, patient #9 presented thermosensory loss for both cold and warm temperatures, and on both body sites; patients #6 and #8 presented thermosensory loss for cold temperatures only and on both body sites; whereas patient #1 presented thermosensory loss of both cold and warm temperatures on the left side only. Besides its quality and location, thermosensory loss also varied in magnitude amongst patients. For example, patient #9 presented the largest thermosensory loss (i.e. thresholds up to 9.5°C greater than CTR), while participant #8 the smallest (i.e. thresholds 0.5°C greater than CTR) ().
Table 1. Individual characteristics of the 11 heat-sensitive MS patients tested in this study, along with their z scores and related absolute temperature values in °C for thermal thresholds (i.e. for both warm and cold and on both left and right side of the body); and z scores and related slope values for thermosensitivity. Proportions (%) of patients affected/showing thermosensory abnormalities are reported under each related column. Grey cells highlight z scores for abnormal thermosensory function (i.e. beyond ±1.96 standard deviation of the CTR group). Legend: Rx: right; Lx: left; RR: relapsing-remitting; PP: primary progressive; SP: secondary progressive
Figure 1. (a) Individual threshold z scores for both cold and warm temperatures and on the left and right side of the body for the 5 MS patients (i.e. P1,4,6,8,9) exhibiting thermosensory abnormalities. Patient number is reported for each value. The gray zone corresponds to the 95% CI of the CTR group, hence values above or below this band indicate a gain or a loss of function, respectively. (b) Thermosensitivity regression lines for the association between stimuli temperature and magnitude estimation, for the CTR group (N = 11) and for the 2 MS patients who exhibited abnormal sensitivity (i.e. slope z score beyond ±1.96 standard deviation of the CTR group)
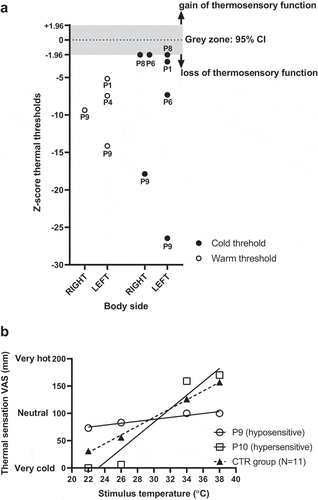
The CTR group presented a significant positive association between progressively greater temperature stimuli and related magnitude estimation of thermal sensation (regression line: Y = 8.09*X – 150.6; slope = 8.09 [95%CI 7.09, 9.10]; p < 0.0001; R2 = 0.86; ). Regarding MS, the slopes of the regression lines for the association between the stimulus intensity and the perceived magnitude estimation of resulting thermal sensations varied significantly amongst MS patients (F11,24 = 5.05; p = <0.001). Slope z-score analysis indicated that 2 out of 11 MS patients (18.2%) exhibited abnormal thermosensitivity (). Specifically, patient #9 presented a large negative slope z score, thereby indicating an abnormal thermosensory profile of hyposensitivity (regression line: Y = 1.78*X + 35.75; slope = 1.78 [95%CI 0.46, 3.09]; p = 0.028; R2 = 0.94; ); whereas participant #10 presented a positive slope z score, thereby indicating an abnormal thermosensory profile of hypersensitivity (regression line: Y = 12.33*X – 286.0; slope = 12.33 [95%CI 1.96, 22.69]; p = 0.036; R2 = 0.93; ).
Discussion
In the present study, we investigated whether and how MS alters thresholds for perceiving increases and decreases in local skin temperature, as well as the sensitivity to progressively greater temperature stimuli applied to the skin, amongst heat-sensitive people with MS.
When considering both threshold and thermosensitivity as indicators of thermosensory function, we observed that 6 out of our 11 heat-sensitive patients (i.e. 54.5%) exhibited skin thermosensory abnormalities (see ). Those abnormalities varied amongst patients in terms of type (i.e. threshold vs. thermosensitivity), quality (warm vs. cold), location (left vs. right side of the body), and extent, and each of those six patients presented unique thermosensory profiles. Nevertheless, the observation that more than half of our sample of heat-sensitive patients presented an altered afferent thermosensory function confirmed our initial hypothesis on the impact of MS on afferent thermosensory function. This is in line with previous studies reporting that abnormalities in sensitivity to temperature (and pain) can be common in MS and be likely due to lesions along the spino-thalamo-cortical tract that subserve afferent thermosensory function [Citation17]. Furthermore, our current findings complement our previous results on the detrimental effects that exercise-induced raises in whole-body temperature (i.e. skin and core) have on patients' cold-sensing afferent function [Citation5]. Taken together, our previous and current results indicate that the detrimental impact of raises in internal body temperature on (de)myelinated afferent pathways subserving cold sensing [Citation5] could represent an exacerbation of an ongoing and broader thermosensory dysfunction (i.e. including warm- and cold-sensing pathways), as observed under the resting conditions tested in the present study.
When considering thermal thresholds, while each individual patient experiencing thermosensory abnormalities presented a somewhat unique sensory profile, some common patterns emerged. Specifically, the left side of those patients’ body appeared to be more commonly affected. Also, cold thresholds were slightly more likely to be impacted than warm ones. Regarding body side, our patients reported the left side of their body as being more negatively affected by their MS than the right side of the body (i.e. 45.4% reported the left side vs. 18.2% reported the right side). Our threshold results are therefore in line with our patients’ experience of an MS-induced symptom laterality. The spatial distribution of MS spinal lesions has been seen to play a greater role in the development of clinical symptoms than lesion burden [Citation14], and neuroanatomical evidence indicates that laterality of symptoms often relates to lesion location (e.g. consider unilateral vision loss resulting from unilateral optic neuritis) [Citation18]. Unfortunately, we did not have access to our patients’ latest Magnetic Resonance Imaging scans, and therefore any potential relationship between neuroanatomical and perceptual laterality within our sample, remains speculative.
Regarding threshold thermal quality, the observation that cold, rather than warm, thresholds were slightly more impacted is in line with our previous findings in heat-sensitive MS patients undergoing cold-selective reductions in thermosensory function as a result of elevations in body temperature [Citation5]. Given that the afferent cold-sensitive pathway is myelinated in humans [Citation9], one could speculate that the demyelinating nature of MS would induce a more prominent impairment in cold, rather than warm (note: ascending warm pathways are non-myelinated), thermosensory function. This hypothesis could be supported by the observation that MS patients experience paradoxical heat sensations upon skin cooling, likely due to a lack of cross-inhibition of warm afferents by malfunctioning central cold pathways. Yet, warm threshold [Citation19] can sometimes [Citation20] be affected more than cold ones in MS patients and so the hypothesis of a preferential impact on cold pathways remain speculative.
When considering thermosensitivity to progressively greater stimuli, we found this to be abnormal in only 18.2% of our patients (N = 2), as compared to the 45.5% of patients who experienced threshold abnormalities (N = 5). Yet, contrary to threshold data, we observed that the two patients who experienced abnormal thermosensitivity presented both loss (i.e. hyposensitivity) and gain (hypersensitivity) of thermosensory function (see ). Sensory hyper-excitability to non-noxious stimuli is common in neurological conditions, and it is also found in MS patients who more frequently present central pain [Citation21]. Unfortunately, we did not assess pain sensitivity in our patient, and so whether the hypersensitivity of patient #10 relates to the presence of central pain, remains untested.
When considering the differential incidence of threshold (45.5%) and thermosensitivity (18.2%) abnormalities, our findings are in line with those of Leocani et al. [Citation6], who found thermal thresholds to be a more sensitive measure of thermosensory loss than magnitude estimation-based assessments alike to our thermosensitivity test (i.e. incidence of thermosensory loss in MS patient sample: 58% threshold vs. 16% thermosensitivity; [Citation6]). Yet, we would like to point out that, while both threshold and thermosensitivity are measures of thermosensory function, they also assess different aspects of this function; hence, we do not believe that one should be preferred over the other, particularly when investigating the implications of heat sensitivity in MS. Readers familiar with the evaluation of human autonomic thermoregulatory function will know well the differential relevance of abnormalities in either onset thresholds and/or sensitivity to changes in body temperature of efferent responses (e.g. vaso- and sudo-motor tone). Accordingly, we suggest that both threshold and thermosensitivity measurements should be considered in the assessment of thermosensory function in heat-sensitive people with MS. For example, a patient may present abnormal thresholds, yet their thermosensitivity may be retained (see e.g. our Patient #1). This means that this patient could experience delays in sensing an initial change in their baseline skin temperature; yet once this occur, any further suprathreshold increase in skin temperature will be detected. It could be therefore argued that in the context of a real-life scenario where one is exposed to a either a warm environment or stimulus, and suprathreshold increases in skin temperature occur (e.g. during sunbathing or while taking a warm shower), a patient with retained thermosensitivity and impaired thresholds, may be less heat vulnerable than a patient who, on the contrary, may have both abnormal thresholds, and blunted thermosensitivity (see e.g. our Patient #9). If one was to only assess thresholds in those heat-sensitive patients, then the second patient may be misjudged as being as vulnerable as the first one (when in fact the latter patient may well be more heat vulnerable).
It is important to note that our thermosensitivity observations arose from testing a single skin site over the entire body, and we recognize that this site was different from the one where thresholds were tested. The evidence we provide should be therefore carefully considered, as patterns of thermosensory loss may well vary across the body of MS patients. In support of this, recent pilot data from our group have indicated that regional differences in magnitude estimation of supra-threshold thermal stimuli across the body are more variable in MS than CTR individuals, with MS inducing patterns of hypo- (and hyper-) sensitivity across different body regions, which could be easily overlooked if thermal assessments are confined to a single skin site [Citation22]. More investigations, including body mapping-like approaches, are therefore needed to determine how thermosensory impairments distribute across the entire body of people with MS.
We know that MS varies widely in clinical presentation, disease course and response to treatments, and that there is significant inter-individual variability in heat sensitivity amongst MS patients [Citation23]; yet as to date, there is no objective method to identify which MS patients are likely to be more heat vulnerable. Our current study, along with our previous investigation [Citation5], provides a practical methodological approach to assess afferent thermosensory function and its inter-individual variability in MS patients, and potentially in other heat-sensitive patients groups (e.g. Parkinson’s Disease). Our approach could be integrated with well-established methods to assess efferent thermoregulatory function (e.g. onset threshold and sensitivity for vasomotor and sudomotor tone) [Citation24], in order to develop a more comprehensive understanding of how both the afferent and efferent components of the thermoregulatory system might be compromised by chronic conditions at a patient level [Citation25].
In conclusion, we demonstrated that more than half of the heat-sensitive MS patients we tested presented afferent thermosensory dysfunction, which varied according to individual profiles of thermosensory loss (or gain). The individual variability observed highlights the need for a patient-centered approach to assessing thermosensory dysfunction and its potential implications for heat stress vulnerability in this patient group. Here, we provide a combination of methods that could help to develop personalized approaches to assess and mitigate the impact of heat-induced symptom worsening in MS.
Author contributions
Experimental testing was performed at the Thermal Ergonomics Laboratory, Faculty of Health Sciences, University of Sydney, Australia and at the THERMOSENSELAB, Loughborough University, UK. All authors contributed to the conception and design of the study. DF, GC, KC, and KF performed data acquisition. DF performed data analysis and drafted the manuscript. All authors contributed to editing significant portions of the manuscript and figures. All authors approved the final version of the manuscript; and agreed to be accountable for all aspects of the work in ensuring that questions related to the accuracy or integrity of any part of the work are appropriately investigated and resolved. All persons designated as authors qualify for authorship, and all those who qualify for authorship are listed.
Acknowledgments
The authors thank Prof George Havenith (Loughborough University) for loaning the thermo-sensory analyser for the Australian portion of the data collection.
Disclosure statement
The authors report no competing interests.
Additional information
Funding
References
- Noseworthy J, Lucchinetti C, Rodriguez M, et al. Multiple Sclerosis. N Engl J Med. 2000;343:938–952.
- Trisolini M, Honeycutt A, Wiener J, et al. Global Economic Impact of Multiple Sclerosis. Mult Scler Int Fed. 2010;May:1–104.
- Christogianni A, Bibb R, Davis S, et al. Temperature sensitivity in multiple sclerosis: an overview of its impact on sensory and cognitive symptoms. Temperature. 2018;5(3):208–223. doi: 10.1080/23328940.2018.1475831.
- Frohman TC, Davis SL, Beh S, et al. Uhthoff’s phenomena in MS - Clinical features and pathophysiology. Nat Rev Neurol. 2013;9:535–540.
- Filingeri D, Chaseling G, Hoang P, et al. Afferent thermosensory function in relapsing-remitting multiple sclerosis following exercise-induced increases in body temperature. Exp Physiol. 2017a;102(8):887–893.
- Leocani L, Martinelli V, Natali-Sora MG, et al. Somatosensory evoked potentials and sensory involvement in multiple sclerosis: comparison with clinical findings and quantitative sensory tests. Mult Scler. 2003;9:275–279.
- Schlader Z, Stannard S, Mündel T. Human thermoregulatory behavior during rest and exercise - a prospective review. Physiol Behav. 2010;99:269–275.
- Schlader ZJ, Sackett JR, Sarker S, et al. Orderly recruitment of thermoeffectors in resting humans. Am J Physiol Integr Comp Physiol. 2018;314:R171–R180.
- Filingeri D. Neurophysiology of skin thermal sensations. Compr Physiol. 2016;6:1429–1491.
- Schlader ZJ, Perry BG, Jusoh MRC, et al. Human temperature regulation when given the opportunity to behave. Eur J Appl Physiol. 2012;113:1291–1301.
- Gafson A, Craner M, Matthews P. Personalised medicine for multiple sclerosis care. Mult Scler. 2017;23:62–369.
- Filingeri D, Morris NB, Jay O. Warm hands, cold heart: progressive whole-body cooling increases warm thermosensitivity of human hands and feet in a dose-dependent fashion. Exp Physiol. 2017b;102(1):100–112.
- Filingeri D, Zhang H, Arens EA. Characteristics of the local cutaneous sensory thermoneutral zone. J Neurophysiol. 2017c;117:1797–1806.
- Eden D, Gros C, Badji A, et al. Spatial distribution of multiple sclerosis lesions in the cervical spinal cord. Brain. 2019;142(3):633–646.
- Rolke R, Magerl W, Campbell K, et al. Quantitative sensory testing: A comprehensive protocol for clinical trials. Eur J Pain. 2016a;10:77–88.
- Rolke R, Baron R, Maier C, et al. Quantitative sensory testing in the German Research Network on Neuropathic Pain (DFNS): standardized protocol and reference values. Pain. 2016b;123:231–243.
- Osterberg A, Boivie J. Central pain in multiple sclerosis - sensory abnormalities. Eur J Pain. 2010;14:104–110.
- Kale N. Optic neuritis as an early sign of multiple sclerosis. Eye Brain. 2016;8:195–202.
- Hasson J, Terry RD, Zimmerman HM. Peripheral neuropathy in multiple sclerosis. Neurology. 1958;8(503):LP–503.
- Iannetti GD, Truini A, Romaniello A, et al. Evidence of a specific spinal pathway for the sense of warmth in humans. J Neurophysiol. 2003;89:562–570.
- Svendsen KB1, Jensen TS, Hansen HJ, et al. Sensory function and quality of life in patients with multiple sclerosis and pain. Pain. 2005;11:473–481.
- Christogianni A, Bibb R, Evangelou N, et al. High-resolution whole-body mapping of warm and cold thermosensitivity in people with multiple sclerosis. 2019. p. 886.
- Waxman SG, Geschwind N. Major morbidity related to hyperthermia in multiple sclerosis. Ann Neurol. 1983;13:348.
- Jessen C. Interaction of Body Temperatures in Control of Thermoregulatory Effector Mechanisms. In: Terjung R, editor. Comprehensive Physiology. 1996.
- Greaney JL, Kenney WL. Measuring and quantifying skin sympathetic nervous system activity in humans. J Neurophysiol. 2017;118:2181–2193.