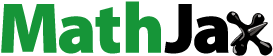
ABSTRACT
White adipose tissue (WAT) thermogenic activity may play a role in whole-body energy balance and two of its main regulators are thought to be environmental temperature (Tenv) and exercise. Low Tenv may increase uncoupling protein one (UCP1; the main biomarker of thermogenic activity) in WAT to regulate body temperature. On the other hand, exercise may stimulate UCP1 in WAT, which is thought to alter body weight regulation. However, our understanding of the roles (if any) of Tenv and exercise in WAT thermogenic activity remains incomplete. Our aim was to examine the impacts of low Tenv and exercise on WAT thermogenic activity, which may alter energy homeostasis and body weight regulation. We conducted a series of four experimental studies, supported by two systematic reviews and meta-analyses. We found increased UCP1 mRNA (p = 0.03; but not protein level) in human WAT biopsy samples collected during the cold part of the year, a finding supported by a systematic review and meta-analysis (PROSPERO review protocol: CRD42019120116). Additional clinical trials (NCT04037371; NCT04037410) using Positron Emission Tomography/Computed Tomography (PET/CT) revealed no impact of low Tenv on human WAT thermogenic activity (p > 0.05). Furthermore, we found no effects of exercise on UCP1 mRNA or protein levels (p > 0.05) in WAT biopsy samples from a human randomized controlled trial (Clinical trial: NCT04039685), a finding supported by systematic review and meta-analytic data (PROSPERO review protocol: CRD42019120213). Taken together, the present experimental and meta-analytic findings of UCP1 and SUVmax, demonstrate that cold and exercise may play insignificant roles in human WAT thermogenic activity.
Abbreviations: WAT:White adipose tissue; Tenv: Environmental temperature; UCP1: Uncoupling protein one; BAT: Brown adipose tissue; BMI:Body mass index; mRNA: Messenger ribonucleic acid; RCT: Randomized controlled trial; WHR: Waist-to-hip ratio; PRISMA: Preferred Reporting Items for Systematic Reviews and Meta-analyses; PET/CT: Positron Emission Tomography and Computed Tomography; REE: Resting energy expenditure; 18F-FDG: F18 fludeoxyglucose; VO2peak:Peak oxygen consumption; 1RM: One repetition maximum; SUVmax: Maximum standardized uptake value; Std: Standardized mean difference
Introduction
White adipose tissue (WAT) thermogenic activity may play a role in whole-body energy balance and two of its main regulators are thought to be environmental temperature (Tenv) and exercise [Citation1]. Uncoupling protein one (UCP1; the main biomarker of thermogenic activity) was, originally, thought to be expressed exclusively in brown adipose tissue (BAT) [Citation2]. Its expression in white adipocytes indicates that WAT may be a thermogenically active endocrine organ with the potential to exert BAT-like functions with associated health benefits for body weight regulation and obesity phenotype[Citation1], including reduced body mass index (BMI) [Citation3], fat mass [Citation4] and increased metabolic rate[Citation5]. Some evidence suggests that low Tenv (i.e. cold exposure) increases the expression of UCP1 mRNA and protein in human subcutaneous WAT [Citation6]. However, subsequent studies failed to confirm a connection of UCP1 [Citation7] and WAT thermogenic activity [Citation8] with cold exposure. They also suggested that exercise increases WAT thermogenic activity [Citation9], perhaps due to increased demand for oxidation during exercise to maintain energy supply [Citation10]. Yet, this is mainly supported by animal research [Citation11–15], whereas human studies failed to confirm a significant impact of exercise on UCP1 in WAT [Citation16–18]. Uncovering the impacts of low Tenv and exercise on WAT thermogenic activity will shed light on the potential contribution of this endocrine organ on energy homeostasis and body weight regulation, with associated spill-over effects for obesity treatment and metabolic pathologies.
Materials and methods
We conducted a series of six studies: two clinical trials, one cross-sectional study, one randomized controlled trial (RCT), and two systematic reviews and meta-analyses. The experimental studies conformed to the standards set by the Declaration of Helsinki and were approved by the Bioethics Review Boards of the University of Thessaly, Department of Exercise Science (protocol numbers 698/13-3-2013 & 1257/7-6-2017), University of Wolverhampton (protocol number 92,944/13-6-2013) and Biomedical Research Foundation Academy of Athens (protocol numbers 7/16-3-2017 and 35/26-3-2019).
Study 1 (Cross-sectional)
Written consent was obtained from 32 healthy (no chronic disease and/or being under medication treatment) nonsmoking and non-regularly exercisers adult men [age: 36.06 ± 7.36 (years); BMI: 27.60 ± 4.63 (kg/m2)]. Table 1 in the supplement [Citation19] shows the participant characteristics. Participants visited the laboratory once between 07:00 and 09:00 am and underwent measurements for body height using a Seca (Hamburg, Germany) stadiometer, body weight using a precision scale (KERN & Sohn GmbH, Version 5.3, Germany), waist-to-hip ratio (WHR) using a tape measure and body composition using a bioelectrical impedance body composition monitor (Fresenius Medical Care AG & Co. KGaA D-61,346 Bad Hamburg, Germany). We also randomly collected nutritional data (telephone diet recall) for two weekdays and one weekend day, within a week period. The data collection for diet was conducted by an independent investigator who was blinded to the aim of the study. To assess UCP1 mRNA/protein expression of WAT cells, participants also underwent a subcutaneous WAT biopsy, following standard guidelines [Citation20], while they were instructed to refrain from exercise, alcohol, and passive smoking in 72-h prior the biopsy procedure. Briefly, the subcutaneous WAT samples were taken 5 cm to the left or right of the navel via a surgical technique using a standardized methodology [Citation21]. All samples were collected between June 2013 and May 2014.
Study 2 (Systematic Review and Meta-analysis)
We conducted two systematic reviews and meta-analyses (PROSPERO review protocol: study 2 CRD42019120116; study 6 CRD42019120213) to test the results of our experimental studies against the state of the art.
Following the Preferred Reporting Items for Systematic Reviews and Meta-analyses (PRISMA) guidelines [Citation22], we searched the PubMed central, Embase, and Cochrane Library (trials) databases from the date of their inception to 21 April 2020, for both studies that evaluated the effect of Tenv and/or cold exposure on UCP1 of human WAT (study 2) and studies that evaluated the effect of exercise on both human and animal UCP1 of WAT (study 6). No date, participants’ health status, language, or study design limits were applied. The searching procedure, screening of the titles, abstracts, and full texts for eligibility as well as the selection of the included studies were conducted independently by two investigators (PCD and LGI) and any conflicts were resolved through discussion with a third investigator (ADF). We also supplemented the electronic database searches with manual searches for published studies in relevant websites. We excluded reviews and magazine articles, but we included published conference proceedings. We also searched the reference lists of the included studies to identify potential eligible studies. The PRISMA flowcharts can be found in for the UCP1 and cold exposure systematic review (study 2) and in for the UCP1 and exercise systematic review (study 6) in the supplement [Citation19]. Also, the searching algorithm for both systematic reviews can be found in the supplement [Citation19].
Two independent investigators (PCD and LGI) evaluated the risk of bias of the included studies in both systematic reviews. Conflicts in the risk of bias assessment were resolved by an independent referee investigator (ADF). As all included studies conducted in humans used an observational design, we evaluated the risk of bias via the 13-item of Research Triangle Institute item bank [Citation23] which is designed for observational studies and has previously shown median inter-rater agreement of 75% [Citation24] and 93.5% [Citation25]. Regarding studies conducted in animals, we evaluated the risk of bias of the included studies via the Systematic Review Centre for Laboratory Animal Experimentation (SYRCLE) tool [Citation26]. The description of grouping the components of both the aforementioned risk of bias tools can be found in the supplement [Citation19].
Independent data extraction was performed by two investigators (PCD and LGI) and conflicts were resolved through consensus and supervision by a third researcher (ADF). When necessary, additional information was requested from the journals and/or the study authors via e-mail. For all studies, we extracted the author name(s), year of publication, cold exposure status or physical activity/exercise intervention, data either on the participants’ number, age, sex, BMI, or on animal species and diet status. Finally, we extracted the main outcome of WAT UCP1 (i.e. means±standard deviations/standard error). The data extraction tables for both systematic reviews can be found in the supplement [Citation19].
We conducted continuous, inverse variance, random-effect model meta-analysis via the RevMan 5.3 software. We used means and standard deviations of UCP1 mRNA and protein expressions of WAT, to test: (a) mean differences between cold exposure and thermoneutral conditions, either pre and post cold exposure or between a cold and a warm period and (b) mean differences between exercise and non-exercise, either pre- and post-exercise or between exercise and control (non-exercise) groups. The study effect sizes were synthesized to account for heterogeneity due to differences in study populations, interventions, study duration, and other factors. We evaluated the 95% confidence interval (CI) and heterogeneity between studies using the I2 statistic. We considered a statistically significant result for heterogeneity when p < 0.10, while interpretation of I2 index was made based on previous guidelines [Citation27]. Where pertinent, standard error (SE) was converted to standard deviation (SD) using the following formula: SD = SE*√n [Citation27].
Study 3 (Clinical Trial)
We conducted a clinical trial (registration number: NCT0403737), using Positron Emission Tomography and Computed Tomography (PET/CT) to assess the association of human subcutaneous WAT thermogenic activity with the Tenv, using a cooling protocol.
Participants recruited through an advertisement in local newspapers or by word of mouth. An initial appointment was made to inform participants about the study and determine eligibility. A health pre-screening was done by interview through a suitable medical history questionnaire. We obtained written consent from 20 healthy (no chronic disease and/or being under medication treatment) and nonsmoking adult men [age: 36.30 ± 5.33 (y); BMI: 28.46 ± 5.49 (kg/m2)]. Table 4 in the supplement [Citation19] displays participants’ characteristics.
On the day of the measurement’s participants advised to transport themselves by car to the PET/CT Laboratory at 07:30 am, following at least a 12-h fasting. At 8 am, the participants consumed a meal consisted of 50% carbohydrates, 20% proteins, and 30% fat to avoid a thermic effect of food on BAT and WAT activity [Citation28]. Also, to avoid a thermic effect of food on the resting energy expenditure (REE) measurements, the caloric value of the meal was 15% of the total energy requirements of each participant [Citation29]. The total energy requirement was calculated following the previous methodology [Citation30]. We have adopted the above-mentioned approach to avoid extending participants´ fasting hours, to control their diet for at least 19 hours before the measurements and to avoid a thermic effect of food on REE and WAT thermogenic activity. At 14:00 pm participants underwent measurements for body height using a Seca (Hamburg, Germany) stadiometer, body weight using a precision scale (KERN & Sohn GmbH, Version 5.3, Germany) and WHR using a tape measure. We also measured blood pressure using a Standard Aneroid sphygmomanometer (Medisave, UK) according to standard guidelines [Citation31]. Blood glucose levels were determined via a Contour NEXT/ASCENSIA monitor. Participants who displayed blood glucose >140 mg/dl and blood pressure >140 mm/Hg were excluded from the study [Citation32].
Immediately after the aforementioned measurements, participants wore specific clothes (long-sleeve sweatshirt and sweatpants) that offered 0.49 clo insulation [Citation4]. Participants then undertook an REE assessment. Following the REE measurement, they remained in a sitting position in a cold room at 16.7 ± 0.6°C (humidity: 49.0 ± 11.1%) for 2 h [Citation4]. The room temperature and humidity were continuously monitored, while an investigator checked every 15 min participants’ muscle shivering status, according to previous methodology [Citation33]. The muscle shivering was tested using a scale, none (no tension of muscles reported), mild (slight muscle tonus in masseter muscle), moderate (real shivering in proximal muscles), severe (uncontrolled shivering in whole body) [Citation33]. In case of moderate or severe shivering, participants were covered with a blanket for the next 15 minutes. The results of the muscle shivering measurements can be found in Table 5 in the supplement [Citation19]. After the first hour of cold exposure, an appropriate dose – according to participants’ body weight – of F18 fludeoxyglucose (18F-FDG) (Table 4 in the supplement) [Citation19] was injected. Following the 2-h cooling protocol, participants undertook the PET/CT examination. A Siemens Biograph 6 high-resolution Erlangen Germany (2006) PET/CT was used to obtain images from the top of the skull to the upper third of the thigh. Six to eight bed positions (5 min per bed position) were required.
PET/CT imaging analysis for BAT activity is described elsewhere [Citation3]. WAT activity analysis (dorso-lumbar region near vertebrae L3 and abdominal region) was performed by two independent trained physicians (AG and SC), using previous methodology [Citation8]. A representative image can be found in in the supplement [Citation19]. Body composition measurements, REE assessments, and Tenv recording are described in the supplement [Citation19].
Study 4 (Clinical Trial)
We have conducted a clinical trial (registration number: NCT04037410), using PET/CT to assess the association of human subcutaneous WAT thermogenic activity with the Tenv, using a non-cooling protocol. We retrospectively evaluated the association of WAT thermogenic activity with Tenv of 202 (male = 126) patients. Patients’ characteristics can be found in Table 6 in the supplement [Citation19]. These patients undertook a PET/CT examination in order to define their disease status. They were given instructions in advance to refrain from smoking, exercise, and excessive muscle stressors 24-h prior to their examination. On the day of the measurements, they were advised to transport themselves by car to the PET/CT Laboratory between 08:00 am to 12:00 pm, following at least a 6-h fasting. Patients were first injected with an appropriate dose of 18F-FDG (Table 6 in the supplement) [Citation19] and then they remained in a sitting relaxed position in a quiet room of 22–24°C for 60 minutes. Following this period, patients undertook the PET/CT examination, which included on average eight bed positions, while 4-min scanning per bed position was used. PET/CT imaging analysis for WAT activity analysis, body composition measurements, REE assessments, and Tenv recording was the same as study 3.
Study 5 (Clinical Randomized Control Trial)
To assess the effects of exercise on human WAT thermogenic activity, we have conducted a parallel RCT (clinical trial registration number: NCT04039685) of an 8-wk of moderate-intensity exercise training followed by an 8-wk of de-training period. A priori power calculation was conducted using an online software (DSS Research) to test statistical power based on previous data of UCP1 mRNA [(baseline = 0.00010 ± 0.0003), (post-exercise = 0.00020 ± 0.00005)] that were reported in a similar controlled trial conducted in humans [Citation17]. The power calculation analysis showed a required sample size of at least seven participants per group. This result ensured 100% of statistical power (alpha error 5%). Participants were recruited by advertisements in a local newspaper in the area of Trikala, Thessaly, Greece; the experimental protocol started in July 2013 and ended in June 2014. Participants were asked to call the researcher in charge (PCD) for an initial interview in order to identify eligibility. The inclusion criteria were: healthy adult men not participating in a regular exercise program, nonsmoker, no chronic disease, and/or being under medication treatment. Information on the health status was retrieved through a medical history questionnaire via interview. Information on the physical activity levels was retrieved via the International Physical Activity Questionnaire [Citation34]. Participants, who were healthy, not participated in a regular exercise program and displayed low or moderate physical activity levels were eligible to participate in the study and they were given a period of 7 d to decide their participation. Upon inclusion, a participant was randomly assigned to a group using an online computer software [Citation35]. The initial allocation and enrollment of the participants to an experimental group were completed by the researcher in charge (PCD) while the supervision of the exercise interventions was completed by three assistant investigators, one for each exercise group who were blinded to the aim of the study. The selection process along with reasons for exclusions as well as participant dropouts in the RCT design appear in a Consolidated Standards of Reporting Trials diagram in in the supplement [Citation19].
The RCT involved four groups: an aerobic exercise group, a resistance exercise group, a combined exercise group (aerobic+resistance), and a non-exercise control group. Measurements for anthropometry, body composition, REE, and subcutaneous fat biopsy were obtained at baseline, after the exercise (wk 8) and the de-training (wk 16) periods while physical activity (pedometer) and nutritional data (diet recall) were randomly collected for two weekdays and one weekend day at baseline, 8th, and 16th wk. The data collection for physical activity and diet was conducted by an independent investigator who was blinded to the aim of the study. Peak oxygen consumption (VO2peak) and one-repetition maximum (1RM) were measured at baseline, 4th, 8th, and 16th wk. The details of the experimental protocol are displayed in in the supplement [Citation19]. The participants' characteristics, anthropometry, assessment of physical activity and exercise programs, REE assessments, VO2peak and 1RM tests, diet assessments, and mRNA and protein analyses for this RCT are described in the supplement [Citation19].
Study 6 (Systematic Review and Meta-analysis)
All methods regarding the systematic review process and the meta-analysis are the same as study 2.
Statistical analyses for experimental studies
All analyses were conducted with SPSS (v24; SPSS Inc., Chicago, IL, USA) using a p ≤ 0.05 level of significance. All variables were tested for normal distribution using Kolmogorov–Smirnov. We used Pearson Correlation coefficients or Spearman’s correlation tests to evaluate the associations between variables. Linear regression analysis was used to calculate R2 and Cohen’s f2 effect size analysis (f2 = R2/1-R2) was used to estimate effect sizes for associations between variables. Independent sample T-tests were used to examine mean differences in UCP1 between cold and warm periods of the year and Mann–Whitney U tests to examine mean differences in SUVmax between cold and warm periods of the year.
Given the non-normal distribution of the variables examined in the RCT (study 5), delta scores were calculated and they were tested for normal distribution using Kolmogorov–Smirnov. The delta scores displayed normal distribution. The effects of exercise and de-training on the delta scores of the variables were tested using analyses of variance (ANOVA) with Bonferroni post hoc t-tests. Following the previous methodology, the 50th percentile of each variable was used to define the low and high responders in order to test the effects of exercise and de-training on the high responders [Citation36]. Chi-square tests were used to assess differences between the experimental groups and the variables examined based on low and high responders’ status. Kruskal–Wallis analysis of variance with post hoc Mann–Witney U tests were used to assess the effects of exercise and de-training of high responders on the variables examined. Pooled Cohen’s d effect size analysis was used to calculate effect sizes between and within groups using the following formula: (N = group sample size, M = mean, SD = standard deviation) [Citation37]. Finally, Wilcoxon Signed Rank tests were used to calculate differences within groups between time points given the abnormal distribution of some variables.
Results
Subcutaneous WAT biopsies obtained from healthy men (study 1) showed that the daily or monthly Tenv in which they lived in was not associated with UCP1 mRNA or protein expression (p > 0.05, Cohen’s f2 < 0.20; representative images and photographic films for UCP1 measurements in the supplement) [Citation19]. Also, we found no mean differences (p > 0.05, Cohen’s dsmall = 0.43) in UCP1 protein expression between a cold period (1 Dec–31 Mar) and a warm period (1 Apr–30 Nov) of the days that the biopsies obtained, even though UCP1 protein expression was higher in the cold period (UCP1 mean: 1.25 ± 0.70, average Tenv = 7.95 ± 2.23°C) than in the warm period (UCP1 mean: 0.95 ± 0.68, average Tenv = 22.61 ± 4.40°C). Nevertheless, the UCP1 mRNA expression was higher [t(30) = 2.23, p = 0.03, Cohen’s d = 0.81, ] and BMI had a tendency to be lower [t(30) = −1.25, p = 0.22, Cohen’s d = 0.55] during the cold (Dec 1-Mar 31; Tenv = 7.95 ± 2.23°C) versus the warm (Apr 1-Nov 30; Tenv = 22.61 ± 4.40°C) periods of the year (for the Northern hemisphere). However, these differences in UCP1 mRNA expression revealed no association with the differences in BMI (p > 0.05). Furthermore, no mean differences (p > 0.05) were found for UCP1 mRNA/protein expression between the cold and the warm period of the year within each BMI category (i.e. normal BMI<25 kg/m2, UCP1 mRNA Cohen’s dsmall = 0.49, UCP1 protein Cohen’s dsmall = 0.41; overweight BMI = 25.1–30 kg/m2, UCP1 mRNA Cohen’s dmoderate = 0.55, UCP1 protein Cohen’s dmoderate = 0.54; and obese BMI>30 kg/m2, UCP1 mRNA Cohen’s dlarge = 1.39, UCP1 protein Cohen’s d = 0.13). We also found that UCP1 mRNA/protein expression displayed no associations with percent fat mass and BMI (p > 0.05, Cohen’s f2 < 0.20). These findings were not influenced by diet (fat/carbohydrate/protein/caffeine content, energy intake, total food mass were similar in the two periods of the year; p > 0.05); suggesting that the influence of low Tenv during the cold period on UCP1 mRNA had an insignificant impact on body weight regulation.
Figure 1. Mean differences in uncoupling protein one (UCP1) mRNA expression and protein concentrations between cold (Dec 1-Mar 31) and warm (Apr 1-Nov 30) periods of the year (for the Northern hemisphere). *Significant differences. Values are presented as means and standard deviations
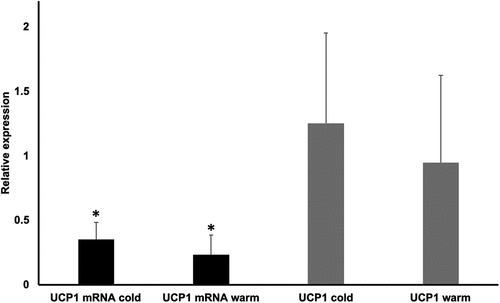
The finding from study 1 that low Tenv may play an insignificant role in WAT UCP1 mRNA/protein and body weight regulation was evaluated against the state-of-the-art through a systematic review and meta-analysis (study 2). The search process identified five published studies reporting results for UCP1 mRNA but no studies reporting results for UCP1 protein levels. Three of these studies [Citation6,Citation7,Citation38] together with data from study 1 provided data for a meta-analysis showing that low Tenv increases UCP1 mRNA in human WAT [standardized mean difference (Std) = 1.81, confidence interval (CI) = 0.50 to 3.13, p = 0.007 ()]. One of the eligible studies [Citation6] and study 1 provided data for a second meta-analysis showing that low Tenv attenuates BMI [mean difference = −1.04, CI = −1.63 to −0.44, p = 0.0007 (see supplement)] [Citation19]. These findings confirm that low Tenv increases UCP1 mRNA in human WAT, however with a questionable impact on WAT thermogenic activity and body weight regulation.
Figure 2. Forest plot of main effect of cold exposure on uncoupling protein 1 (UCP1) mRNA expression in subcutaneous human white fat. SD: standard deviation; TWF: thigh white fat; AWF: abdominal white fat
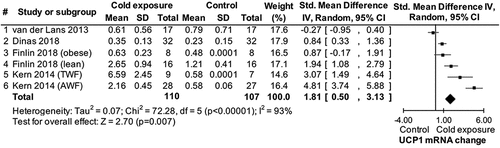
Thermogenic activity in WAT can be evaluated using the maximum Standardized Uptake Values (SUVmax) recorded during PET/CT scans [Citation8]. In a cohort of 20 healthy men (study 3), we found a moderate inverse association between percent fat mass (rho = −0.67, p = 0.001, Cohen’s f2moderate = 0.69) and BMI (r = −0.71, p = 0.0001, Cohen’s f2moderate = 0.75) with SUVmax in WAT (), indicating that WAT thermogenic activity may be associated with a leaner phenotype. However, there was no effect of either low Tenv or the cold period (Tenv = 12.00 ± 4.57°C) of the year on the SUVmax values recorded following a 2-h cooling protocol (p > 0.05). Also, no mean differences (p > 0.05) were found for SUVmax between the cold and the warm period within each BMI category (i.e. normal BMI<25 kg/m2, Cohen’s d = 0.01; overweight BMI = 25.1–30 kg/m2, Cohen’s d = 0.15; and obese BMI>30 kg/m2, Cohen’s d = 0.01). These findings may be attributed to the 2-h cooling protocol used prior to the PET/CT scans. In our study, 11 out of the 20 participants were positive (SUVmax>1.5) in BAT activity, suggesting that the increased SUVmax in BAT caused by the 2-h cooling protocol adopted may have masked a potentially small, yet significant, impact of low Tenv on the SUVmax values recorded in WAT. To test whether this masking effect occur, we proceeded in a subsequent similar PET/CT study without a cooling protocol.
Figure 3. PET/CT cooling protocol study; associations of SUVmax-WAT-activity with %fat mass (a) and body mass index (b)
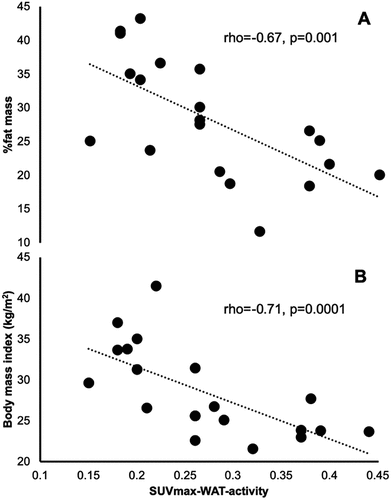
In a cohort of 202 patients (study 4) who undertook a PET/CT without a cooling protocol, we found no effect of either low Tenv or the cold period (Tenv = 8.66 ± 3.75°C) of the year on the SUVmax values recorded and on BMI (p > 0.05; Cohen’s d < 0.20). Confirming study 3 which used a cooling protocol prior to the PET/CT, we found a minor inverse association between BMI and SUVmax in WAT (rho = −0.24, p = 0.001, Cohen’s f2 < 0.20, ), indicating that WAT thermogenic activity may be associated with a leaner phenotype, similarly to BAT thermogenic activity [Citation1,Citation3,Citation4]. We found however, no mean differences (p > 0.05) for SUVmax between the cold and the warm period within each BMI category (i.e. normal BMI<25 kg/m2, Cohen’s d = 0.06; overweight BMI = 25.1–30 kg/m2, Cohen’s d = 0.01; and obese BMI>30 kg/m2, Cohen’s dsmall = 0.28). Taken together, studies 1 through 4 show that low Tenv may increase UCP1 mRNA in human WAT, however with an insignificant impact on WAT thermogenic activity and body weight regulation.
Regarding the impact of moderate-intensity exercise on UCP1 mRNA and protein expression in subcutaneous WAT biopsies obtained from healthy men in a RCT (study 5), we found no differences between and within groups in UCP1 mRNA or protein expressions neither between baseline and post-exercise (wk 8) nor between post-exercise (wk 8) and post-de-training (wk 16; p > 0.05). Additional analyses of UCP1 mRNA and protein expressions for low- (<50th percentile) and high- (>50th percentile) responders to the moderate-intensity exercise and to the de-training stimuli also showed no differences between the experimental groups at all time points (p > 0.05). From a clinical viewpoint, the 8-wk moderate-intensity exercise interventions improved VO2peak and 1RM, but had no effect on REE, BMI, percent fat mass, fat-free mass, and dietary habits (p > 0.05; see supplement) [Citation19].
The finding from study 5 that exercise does not impact WAT UCP1 mRNA and protein expressions was evaluated against the state-of-the-art through a systematic review and a series of meta-analyses (study 6). The search process identified eight eligible studies conducted in humans and 19 eligible studies conducted in animals (see supplement) [Citation19]. Four of the studies in humans [Citation16–18,Citation39] together with data from study 5 provided data for a meta-analysis showing no effect of chronic exercise on UCP1 mRNA (p > 0.05; no data were available to test this hypothesis for UCP1 protein expression). Seven of the studies in animals identified in the systematic review that used a normal diet [Citation11–15,Citation40,Citation41] provided data for a meta-analysis showing no effect of chronic exercise on UCP1 mRNA (p > 0.05). An additional meta-analysis using data from four studies [Citation11,Citation42–44] for UCP1 protein expression showed a significant impact of chronic exercise in normal diet animals (Std = 0.41, CI = −0.01–0.83, p = 0.05, ). With respect to acute exercise (i.e. a single exercise bout) in animals consuming a normal diet, a meta-analysis using data from two eligible studies [Citation45,Citation46] revealed a significant impact of exercise on UCP1 mRNA (Std = 1.76, CI = 0.48–3.04, p = 0.007, ). In animals consuming a high-fat diet, a meta-analysis using data from four eligible studies [Citation14,Citation43,Citation44,Citation47] showed significant impacts of chronic exercise on both UCP1 mRNA (Std = 2.94, CI = 0.24–5.65, p = 0.03, ) and protein (Std = 2.06, CI = 0.07–4.05, p = 0.04, Figure 14 in the supplement) [Citation19] expression.
Figure 5. Forest plot of main effect of chronic exercise on uncoupling protein 1 (UCP1) expression in white fat of normal diet animals. SD: standard deviation; MIT: moderate-intensity training; HIIT: high-intensity interval training
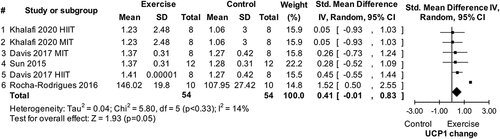
Discussion
In this series of four experimental and two meta-analytic studies, we aimed to shed light on the impacts of low Tenv and exercise on human WAT thermogenic activity. Regarding the effects of low Tenv on human UCP1 of subcutaneous WAT, we found that cold exposure may be unable to increase WAT thermogenic capacity (i.e. UCP1 levels) and consequently to alter energy homeostasis and body weight regulation. This finding is well supported by our meta-analysis of previous evidence; however, there is a lack of evidence in humans for UCP1 protein expression in WAT in response to low Tenv.
Taken together, the available evidence for the impact of exercise on WAT mRNA/protein expression suggests distinct differences between humans and animals. The findings in humans demonstrate that exercise does not impact WAT UCP1 mRNA and protein expressions. In contrast, studies conducted in animals show that both chronic and acute exercise increase UCP1 activation in WAT independently of the diet consumed. These contradictory findings may be explained by the heterogeneity (i.e. molecular signature) of WAT depots and the challenge to isolate those that exhibit brown-like properties in humans [Citation10]. Also, anatomical differences in both brown and brown-like adipocytes were observed between humans and animals [Citation2,Citation10]. Another reason could be that obese animals displayed more UCP1 than lean animals, the opposite trend of humans [Citation48], which indicates differences in UCP1 adaptations to exercise between animals and humans.
Our approach in this series of studies has a number of strengths. We used systematic reviews and meta-analyses to test our findings from the experimental studies, rather than selecting previous similar studies to elucidate our results. In our studies 1 and 5, we analyzed UCP1 protein expression in addition to UCP1 mRNA, so that we test the actual stimulus of thermogenesis in WAT, which entirely depends on the UCP1 protein [Citation49]. In this regard, in study 5 we have tested the associations between REE and UCP1 mRNA/protein expressions at baseline, post-exercise, and post-detraining. Results showed an inverse association of REE with UCP1 protein expression (rho = −0.36, p = 0.05, Cohen´s f2 = 0.15) post-exercise. This finding does not support the hypothesis that UCP1 may increase REE, which could alter energy homeostasis. We used biopsies to obtain the best available UCP1 evidence for human subcutaneous WAT, while a PET/CT approach allowed us to also obtain robust evidence for the human WAT thermogenic activity. Regarding the systematic review approach, we used appropriate algorithms with standardized indexing terms to search relevant to the aims of the systematic review databases. Standardized indexing terms can retrieve records that may use different words to describe the same term beyond that may be contained in the words of the title and abstract [Citation27]. Furthermore, our systematic reviews used a robust methodology for systematic literature searching [Citation50–52] while we used well-established tools [Citation23,Citation26] to evaluate the quality of the included studies. Also, to reduce bias two investigators worked independently on the searching, screening, inclusion of eligible studies, data extraction, and risk of bias assessment. Finally, we have not excluded studies based on language and we considered conference proceeding to expand our data collection pool.
Our studies are not without their limitations. Regarding the actual exposure to low Tenv of our participants in their everyday life, this was not tested; for instance, the time the participants spent in outdoor activities (e.g. work) and their clothing insulation during a cold period of the year. From a practical point of view however, these assessments can be very challenging. Also, we only included body temperature measurements in study 3, as a precaution for the exposure of the participants in the cold environment. Body temperature in study 3 showed no associations with SUVmax (p > 0.05). The association of body temperature with UCP1 and SUVmax, however, was not tested in studies 1, 4, and 5. Regarding study 1, we were unable to locate previous data to perform sample size calculation for UCP1 protein values. However, we performed a post-measurement power calculation using an online software (DSS Research) to test statistical power based on previous similar data of UCP1 mRNA (mean±standard deviation = 6.59 ± 2.4) [Citation6]. This analysis revealed 100% statistical power for study 1. In our PET/CT studies (studies 3 and 4) we were unable to perform either a pre or post-measurements power calculation, given the absence of relevant studies in the literature, since the only similar study [Citation8] used SUVmean as a measurement value for WAT activity, which is different from our PET/CT studies (SUVmax). In our PET/CT cooling protocol study 3 we used only men, therefore, gender differences could not be determined. Furthermore, in our PET/CT non-cooling protocol study 4 the measurements were performed only in patients; however, patients have been previously recruited in several studies related to 18F-FDG BAT activity [Citation3,Citation53–55]. A limitation could be that the exercise programs in our RCT study were based on 65% intensity throughout the exercise period, which may be relatively low intensity for biological adaptations in healthy populations. However, the exercise programs were effective in terms of VO2peak and 1RM adaptations (see supplement) [Citation19].
Regarding the systematic reviews, we found no RCTs eligible studies conducted in humans, while there was only one RCT among the eligible studies that conducted in animals. This may have lowered the strength of the available evidence used in our meta-analyses. Furthermore, the heterogeneity of our meta-analyses was relatively high, which implies a variation in the studies used in each included meta-analysis. In this regard, there was a small number of studies to be included in each meta-analysis (2–5), which did not allow for funnel plot generation to assess publication bias [Citation27]. The overall risk of bias in the studies used for the meta-analyses included in study 2 was low or unclear, which suggests interpreting the results of these meta-analyses with relative confidence. However, the overall risk of bias in the studies used for the meta-analyses included in study 6, was high. As such, the results of these meta-analyses should be interpreted with caution.
Cold exposure may stimulate WAT to increase UCP1 gene expression via sympathetic nervous activation and norepinephrine stimulation [Citation56]. This may cause a “browning” process of WAT with the potential to exert BAT-like functions [Citation1]. It is suggested, however, that “browning” of WAT may also occur via chronic stimulation of β3 adrenergic agents [Citation56]. There is also a possibility of some “browning” agents to disrupt UCP1 expression in WAT [Citation56], which may lead to low UCP1 detection in the tissue. This implies that the presence or absence of these pathways may have affected the UCP1 gene expression and the thermogenic activity of WAT in our participants. Therefore, the fact that we were unable to examine these pathways may pose a limitation in our studies.
In conclusion, the findings presented in this series of six studies improve our understanding of white-fat thermogenesis. Taken together, the present experimental and meta-analytic findings of UCP1 and SUVmax, demonstrate that cold and exercise may play insignificant roles in human WAT thermogenic activity. As such, the role of human WAT thermogenesis in energy homeostasis and body weight regulation (if any) remains under investigation. Although our findings on UCP1 and SUVmax are complemented by nutritional and body measurements, other factors such as environmental temperatures out of the range tested in our study may play a role in human WAT thermogenic capacity and should be explored in future studies.
Abbreviations
Supplemental Material
Download MS Word (13.3 MB)Acknowledgments
We thank Dr Vassilios Chachopoulos and Dr Fani Chasioti for assisting with the fat biopsies.
Disclosure statement
The authors have declared that no conflict of interest exists.
Supplemental material
Supplemental data for this article can be accessed here.
Additional information
Funding
References
- Valente A, Jamurtas AZ, Koutedakis Y, et al. Molecular pathways linking non-shivering thermogenesis and obesity: focusing on brown adipose tissue development. Biol Rev Camb Philos Soc. 2015;90(1):77–88.
- Wu J, Bostrom P, Sparks LM, et al. Beige adipocytes are a distinct type of thermogenic fat cell in mouse and human. Cell. 2012;150(2):366–376.
- Dinas PC, Nikaki A, Jamurtas AZ, et al. Association between habitual physical activity and brown adipose tissue activity in individuals undergoing PET-CT scan. Clin Endocrinol (Oxf). 2015;82(1):147–154. .
- van Marken Lichtenbelt WD, Vanhommerig JW, Smulders NM, et al. Cold-activated brown adipose tissue in healthy men. N Engl J Med. 2009;360(15):1500–1508.
- Steiner AA. The dynamic nature of resting metabolic rate. Temperature. 2017;4(3):206–207.
- Kern PA, Finlin BS, Zhu B, et al. The effects of temperature and seasons on subcutaneous white adipose tissue in humans: evidence for thermogenic gene induction. J Clin Endocrinol Metab. 2014;99(12):E2772–9.
- van den Beukel JC, Grefhorst A, Hoogduijn MJ, et al. Women have more potential to induce browning of perirenal adipose tissue than men. Obesity (Silver Spring, Md). 2015;23(8):1671–1679.
- Hanssen MJ, van der Lans AA, Brans B, et al. Short-term cold acclimation recruits brown adipose tissue in obese humans. Diabetes. 2016;65(5):1179–1189.
- Bostrom P, Wu J, Jedrychowski MP, et al. A PGC1-alpha-dependent myokine that drives brown-fat-like development of white fat and thermogenesis. Nature. 2012;481(7382):463–468.
- Aldiss P, Betts J, Sale C, et al. Exercise-induced ‘browning’ of adipose tissues. Metabolism. 2018;81:63–70.
- Davis RAH, Halbrooks JE, Watkins EE, et al. High-intensity interval training and calorie restriction promote remodeling of glucose and lipid metabolism in diet-induced obesity. Am J Physiol Endocrinol Metab. 2017;313(2):E243–E56.
- Knudsen JG, Murholm M, Carey AL, et al. Role of IL-6 in exercise training- and cold-induced UCP1 expression in subcutaneous white adipose tissue. PloS One. 2014;9(1):e84910.
- Reisi J, Ghaedi K, Rajabi H, et al. Can resistance exercise alter irisin levels and expression profiles of FNDC5 and UCP1 in rats? Asian J Sports Med. 2016;7(4):e35205.
- Xu X, Ying Z, Cai M, et al. Exercise ameliorates high-fat diet-induced metabolic and vascular dysfunction, and increases adipocyte progenitor cell population in brown adipose tissue. Am J Physiol Regul Integr Comp Physiol. 2011;300(5):R1115–25.
- Zhang J, Valverde P, Zhu X, et al. Exercise-induced irisin in bone and systemic irisin administration reveal new regulatory mechanisms of bone metabolism. Bone Res. 2017;5:16056.
- Nakhuda A, Josse AR, Gburcik V, et al. Biomarkers of browning of white adipose tissue and their regulation during exercise- and diet-induced weight loss. Am J Clin Nutr. 2016;104(3):557–565.
- Norheim F, Langleite TM, Hjorth M, et al. The effects of acute and chronic exercise on PGC-1alpha, irisin and browning of subcutaneous adipose tissue in humans. FEBS J. 2014;281(3):739–749.
- Otero-Diaz B, Rodriguez-Flores M, Sanchez-Munoz V, et al. Exercise induces white adipose tissue browning across the weight spectrum in humans. Front Physiol. 2018;9:1781.
- Dinas PC, Krase A, Nintou E, et al. Supplement to the original contribution_human white-fat thermogenesis: experimental and meta-analytic findings. 2020.
- Campbell KL, Makar KW, Kratz M, et al. A pilot study of sampling subcutaneous adipose tissue to examine biomarkers of cancer risk. Cancer Prev Res (Phila). 2009;2(1):37–42.
- Chachopoulos V, Dinas PC, Chasioti M, et al. A technique for subcutaneous abdominal adipose tissue biopsy via a non-diathermy method. J Vis Exp. 2017;(127).
- Moher D, Liberati A, Tetzlaff J, et al. Preferred reporting items for systematic reviews and meta-analyses: the PRISMA statement. PLoS Med. 2009;6(7):e1000097.
- Viswanathan M, Berkman ND, Dryden DM, et al. Assessing risk of bias and confounding in observational studies of interventions or exposures: further development of the RTI item bank. Rockville, MD; 2013.
- Margulis AV, Pladevall M, Riera-Guardia N, et al. Quality assessment of observational studies in a drug-safety systematic review, comparison of two tools: the Newcastle-Ottawa scale and the RTI item bank. Clin Epidemiol. 2014;6:359–368.
- Al-Saleh MA, Armijo-Olivo S, Thie N, et al. Morphologic and functional changes in the temporomandibular joint and stomatognathic system after transmandibular surgery in oral and oropharyngeal cancers: systematic review. J Otolaryngol Head Neck Surg. 2012;41(5):345–360.
- Hooijmans CR, Rovers MM, de Vries RB, et al. SYRCLE’s risk of bias tool for animal studies. BMC Med Res Methodol. 2014;14:43.
- Higgins JP, Green S. Cochrane handbook for systematic reviews of interventions. London, UK: The Cochrane Collab; 2011.
- Lee P, Smith S, Linderman J, et al. Temperature-acclimated brown adipose tissue modulates insulin sensitivity in humans. Diabetes. 2014;63(11):3686–3698.
- Reed GW, Hill JO. Measuring the thermic effect of food. Am J Clin Nutr. 1996;63(2):164–169.
- Gerrior S, Juan W, Basiotis P. An easy approach to calculating estimated energy requirements. Prev Chronic Dis. 2006;3(4):A129.
- Williams B, Poulter NR, Brown MJ, et al. British hypertension society guidelines for hypertension management 2004 (BHS-IV): summary. BMJ. 2004;328(7440):634–640.
- Chen KY, Cypess AM, Laughlin MR, et al. Brown adipose reporting criteria in imaging STudies (BARCIST 1.0): recommendations for standardized FDG-PET/CT experiments in humans. Cell Metab. 2016;24(2):210–222.
- Vanderstappen I, Vandermeersch E, Vanacker B, et al. The effect of prophylactic clonidine on postoperative shivering. A large prospective double-blind study. Anaesthesia. 1996;51(4):351–355.
- Karolinska I International physical activity questionnaire. 2012. [accessed date 2013 March 15]. Available from: http://www.ipaq.ki.se/
- Random.org. True random number service. 1998–2015. [accessed date 2013 April 18]. Available from: https://www.random.org/
- Prestes J, da Cunha Nascimento D, Tibana RA, et al. Understanding the individual responsiveness to resistance training periodization. Age (Dordr). 2015;37(3):9793.
- Cohen J. Statistical power analysis for the behavioral sciences. 2nd ed. New Jersey, US: Lawrence Erlbaum Associates; 1988.
- Finlin BS, Memetimin H, Confides AL, et al. Human adipose beiging in response to cold and mirabegron. JCI Insight. 2018;3(15). DOI:10.1172/jci.insight.121510.
- Brandao CFC, de Carvalho FG, Souza AO, et al. Physical training, UCP1 expression, mitochondrial density, and coupling in adipose tissue from women with obesity. Scand J Med Sci Sports. 2019;29(11):1699–1706.
- Balagova L, Graban J, Puhova A, et al. Opposite effects of voluntary physical exercise on beta3-adrenergic receptors in the white and brown adipose tissue. Horm Metab Res. 2019;51(9):608–617.
- Daneshyar S, Afshari S, Kadivar M, et al. The effect of exercise training on the signaling pathway of Microrna196-A to uncoupling protein 1 in white adipose tissue. Sci Sports. 2018;6:33.
- Khalafi M, Mohebbi H, Symonds ME, et al. The impact of moderate-intensity continuous or high-intensity interval training on adipogenesis and browning of subcutaneous adipose tissue in obese male rats. Nutrients. 2020;12(4):925.
- Rocha-Rodrigues S, Rodriguez A, Gouveia AM, et al. Effects of physical exercise on myokines expression and brown adipose-like phenotype modulation in rats fed a high-fat diet. Life Sci. 2016;165:100–108.
- Sun C, Zeng R, Cao G, et al. Vibration training triggers brown adipocyte relative protein expression in rat white adipose tissue. Biomed Res Int. 2015;2015:919401.
- Ringholm S, Grunnet Knudsen J, Leick L, et al. PGC-1alpha is required for exercise- and exercise training-induced UCP1 up-regulation in mouse white adipose tissue. PloS One. 2013;8(5) ( no pagination):e64123.
- Shen Y, Zhou H, Jin W, et al. Acute exercise regulates adipogenic gene expression in white adipose tissue. Biol Sport. 2016;33(4):381–391.
- Schaalan MF, Ramadan BK, Abd Elwahab AH. Synergistic effect of carnosine on browning of adipose tissue in exercised obese rats; a focus on circulating irisin levels. J Cell Physiol. 2018;233(6):5044–5057.
- Madden CJ. Brown fat in obesity: uncoupling protein-1 versus thermogenic activity. Temperature. 2017;4(2):126–127.
- Nedergaard J, Cannon B. UCP1 mRNA does not produce heat. Biochim Biophys Acta. 2013;1831(5):943–949.
- Khan KS, Kunz R, Kleijnen J, et al. Five steps to conducting a systematic review. J R Soc Med. 2003;96(3):118–121.
- Liberati A, Altman D, Tetzlaff J, et al. The PRISMA statement for reporting systematic reviews and meta-analyses of studies that evaluate healthcare interventions: explanation and elaboration. BMJ Res methods reporting. 2009;339:b2700
- Harris JD, Quatman CE, Manring MM, et al. How to write a systematic review. Am J Sports Med. 2014;42(11):2761–2768.
- Cypess AM, Lehman S, Williams G, et al. Identification and importance of brown adipose tissue in adult humans. N Engl J Med. 2009;360(15):1509–1517.
- Persichetti A, Sciuto R, Rea S, et al. Prevalence, mass, and glucose-uptake activity of (1)(8)F-FDG-detected brown adipose tissue in humans living in a temperate zone of Italy. PloS One. 2013;8(5):e63391.
- Baba S, Jacene HA, Engles JM, et al. CT hounsfield units of brown adipose tissue increase with activation: preclinical and clinical studies. J Nucl Med. 2010;51(2):246–250.
- Nedergaard J, Cannon B. The browning of white adipose tissue: some burning issues. Cell Metab. 2014;20(3):396–407.