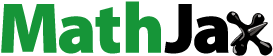
ABSTRACT
The purpose of this study was to assess the heat strain experienced by children during unstructured physical activity outdoors in a temperate continental summer climate. Eighteen children (7 girls, 12.1 ± 1.7 years) performed up to 4 h of outdoor free-play (duration: 218 ± 33 min; air temperature of 24.5 ± 3.9°C and relative humidity of 66.2 ± 9.2%). Urine specific gravity (USG) was measured pre- and post-free-play, while body core temperature (Tco, ingestible pill) and heart rate (HR) were measured continuously. Physiological strain index (PSI) was calculated from Tco and HR (scale: 0 (none) to 10 (very high)). Activity levels were categorized as rest, light, moderate, and vigorous based on the metabolic equivalent of task, estimated from video analysis. Most children were euhydrated pre (78%, USG ≤ 1.020), but not post-free-play (28%, USG ≤ 1.020). Mean and peak Tco, HR, and PSI responses were 37.8 ± 0.3°C and 38.4 ± 0.3°C, 133 ± 14 bpm and 180 ± 12 bpm, and 4.7 ± 1.1 (low) and 7.4 ± 1.0 (high), respectively. All children reached peak Tco≥38.0°C, with seven ≥38.5°C, and the highest at 38.9°C. The children spent 58 ± 15% of free-play engaged in moderate-to-vigorous intensity physical activity. During free-play, all of the children performed moderate-to-vigorous intensity physical activity, which was associated with pronounced elevations in heat strain.
Introduction
Regular physical activity is associated with many health benefits for children including reduced adiposity, improvements in multiple indicators of musculoskeletal and cardiovascular health, in addition to enhanced mood and cognitive function [1]. To achieve these benefits, government and civil society organizations worldwide recommend that children spend a minimum of 60 minutes per day engaged in moderate-to-vigorous intensity physical activity, along with promoting increased participation rates for children who do not meet these requirements [Citation2]. However, compliance rates are generally poor, with only around 20% of children globally meeting this recommendation [Citation3]. While the myriad health benefits of physical activity in children are clear, an exercise-induced heat stress may also result in considerable heat strain (i.e. overall physiological response to a heat stress) in this population, especially in the warm summer months when children are highly engaged in outdoor activities.
Structured outdoor physical activity, such as organized sporting events, may be associated with considerable heat strain in children, especially when performed in hot conditions [Citation4]. Indeed, children may comprise as much as 48% of annual emergency room visits due to heat-related injuries resulting from sports and recreational activities [Citation5]. The risk of heat-related illness is amplified during repeated training sessions or tournament play in the heat when heightened personal motivation and social pressures to keep playing may result in inadequate rest periods or rehydration efforts, pushing children beyond their physiological limits [Citation6]. Despite the potential for considerable heat strain in children during physical activity outdoors in warm ambient conditions, direct measures of body core temperature (Tco) remain sparse in this population outside of controlled laboratory settings. A notable exception is in a group of fourteen physically active children (15.1 ± 1.4 years; 5 girls) engaging in 2 h of organized tennis training in a warm environment (WBGT, 79.9 ± 2.2°F), wherein Tco (measured by ingestible pill) averaged 38.2°C after 40–60 min of training and remained above 38.0°C for the remainder of the session [Citation7]. In another study, the average Tco (measured by ingestible pill) reached 38.7°C by the end of match play (duration = 78.0 ± 8.1 min) in a group of eight young male tennis players (13.9 ± 0.9 years) during singles competition in the heat (WBGT, 29.6 ± 0.4°C) [Citation8]. Both cases demonstrated considerable heat strain in children engaged in structured physical activity in the heat. However, it remains unclear how these findings relate to physical activity that is performed outside of organized sporting events.
Currently, there is a paucity of information relating to the level of heat strain experienced by children during unstructured physical activity (i.e. free play). This is particularly important given that children tend to engage in free play more often during the warm summer months than in fall and winter [Citation9], which may pose a risk to their health and well-being. As such, the purpose of this field study was to assess the heat strain experienced by children (10 to 14 years) during an extended period (up to 4 h) of free play in a continental summer climate.
Materials and methods
Ethical approval
This study was approved by the University of Ottawa Health Sciences and Science Research Ethics Board (REB#: H-04-19-3752) and the Health Canada Research Ethics Board (REB#: 2019–003 H) in accordance with the guidelines set forth by the Declaration of Helsinki. Informed assent and consent were obtained from all children and parents before participation.
Participants
Eighteen healthy, non-obese, children (seven girls) aged 10–14 years participated in this study. All of the children were habitually active (i.e. performed ≥30 min of structured physical activity at least twice per week) [Citation10]. Participant characteristics are presented in .
Table 1. Physical characteristics
Experimental design
All testing took place outdoors in the summer period (August and early September) at sites selected by the participants where they commonly engage in free play (i.e. parks, schoolyards, and recreation centers) in Southern Ontario, Canada. All participants completed one preliminary session and one field testing session, which involved up to 4 hours of monitoring during unstructured physical activity outdoors. During the preliminary session, height was measured using an eye-level physician stadiometer (Model 2391, Detecto Scale Company, Webb City, MO, USA) and body mass was measured using a digital weight scale platform (Model CBU150X, Mettler Toledo Inc., OH, USA). Body surface area was then calculated according to the equation by Du Bois and Du Bois [Citation11].
For the day of field testing, participants were instructed to arrive well hydrated, having eaten their usual meal and having abstained from exercise for 24 hours. All testing started in the late morning or early afternoon and a nearly equal number of participants started the trials during these periods. Upon arrival at the testing site, the children provided a urine sample for the measurement of urine specific gravity (USG), which was measured with a total solids refractometer (TS400, Reichter Inc., Depew, NY) to assess hydration. Core temperature was measured using an ingestible telemetric capsule (BodyCap, e-Celsius Performance, France), which was provided with 500 ml of 37°C water a minimum of 2 h prior to the start of free play. Participants were instrumented with a heart rate (HR) monitor (RS 400 Polar Electro, Kempele, Finland) and 4 wireless skin temperature sensors (DS1921 G-F5 Thermochron, iButtonLink LLC, WI, USA). All participants wore standard athletic clothing consisting of a t-shirt, shorts, socks, and athletic shoes. Following instrumentation, the children sat quietly in temperate conditions for 10 min for baseline measures.
Throughout free play, subjective ratings of perceived exertion (RPE), thermal sensation, and thirst sensation were collected every 15 min. Water consumption was restricted to 200 ml of 37°C water per hour on participant request to maintain adequate hydration levels [Citation12] while minimizing the influence of water ingestion on gastrointestinal pill temperature. At the end of free play, a second urine sample was collected to assess post-play USG and body mass was recorded. The difference between pre- and post-mass, corrected for water and snack consumption and urine output was used to estimate total sweat loss.
Measurements
Air temperature and relative humidity were monitored with a portable weather station (Microtherm heat stress WBGT, Casella Cel ltd., Bedford, UK) and recorded every 15 min throughout free play. The corresponding wet bulb globe temperature (WBGT) was calculated by the weather station according to the following equation:
where Td, Tw, and Tg represent dry-bulb (i.e. air temperature), wet-bulb, and black globe temperatures, respectively. Body core temperature was measured continuously and stored in 10 s intervals using an ingestible capsule and wireless monitoring system (e-Celsius Performance, BodyCap, France). Skin temperature was measured at 4 sites (calf, quadriceps, chest, biceps) every 60 s using iButtons (DS1921 G-F5 Thermochron, iButtonLink LLC, WI, USA) fixed to the skin with medical glue. Heart rate was measured continuously and stored every 5 s using a Polar coded WearLink and transmitter and Polar RS400 interface (Polar Electro, Kempele, Finland).
The Borg scale, ranging from 6 (no exertion at all) to 20 (maximal exertion) was used to quantify RPE [Citation13]. A modified ASHRAE 7-point scale, ranging from 0 (neutral) to 7 (extremely hot) was used to quantify thermal sensation [Citation14]. A 9-point Thirst Sensation scale, ranging from 1 (not thirsty at all) to 9 (very, very thirsty) was used to quantify thirst sensation [Citation15]. The children were familiarized with all of the scales prior to the start of free play. All perceptual scales were measured every 15 min throughout free play.
Data analysis
Hydration status, based on USG, was classified as euhydrated (USG ≤ 1.020) or hypohydrated (USG > 1.020) in accordance with the current American College of Sports Medicine (ACSM) exercise and fluid replacement position stand [Citation16].
Physical activity was categorized as rest, light, moderate, or vigorous based on video analysis with energy expenditure estimations based on the metabolic equivalent of the task (MET), obtained from the Youth Compendium for Physical Activity [Citation17] and cross-referenced with HR, RPE, and field records. These data are presented as the proportion of time spent at each physical effort level during free play.
The Tco, mean skin temperature, and HR are presented as the mean (60 s) response throughout free play and as the highest (60 s) peak response. The proportion of free play at different Tco levels was determined as follows: Level 1: Tco < 37.0°C, Level 2: 37.0°C ≤ Tco < 37.5°C, Level 3: 37.5°C ≤ Tco < 38.0°C, Level 4: 38.0°C ≤ Tco < 38.5°C and Level 5: Tco ≥ 38.5°C [Citation18]. Skin temperature is presented as the weighted mean of the local skin temperatures from the calf (20%), quadriceps (20%), chest (30%), and biceps (30%) [Citation19].
Heart rate responses are presented in absolute values and as the percentage of estimated HR reserve (%HRR), based on the following equation:
where HRt is the heart rate at a given time point, HRbl is the baseline heart rate as measured prior to the start of the experimental session and HRmax is the participant’s estimated maximal heart rate (HRmax = 220 – age). For %HRR, the proportion of free play spent at different levels of %HRR was determined by the ACSM intensity classifications as follows: Level 1 (very light): %HRR < 30%, Level 2 (light): 30% ≤ %HRR < 40%, Level 3 (moderate): 40% ≤ %HRR < 60%, Level 4 (vigorous): 60% ≤ %HRR < 90% and Level 5 (near maximal): %HRR ≥ 90% [Citation20].
The Physiological Strain Index (PSI) was calculated from measurements of Tco and HR using the following equation [Citation21]:
where Tco is the core temperature recorded at a given time point, Tcobl is the core temperature recorded at baseline, HRt is the heart rate recorded at a given time point and HRbl is the heart rate recorded at baseline. In parallel with Tco and HR responses, %HRR and PSI were presented as mean and peak 60 s average responses during free play. The proportion of free play spent at different PSI levels was determined based on the following categories: Level 1 (little or no strain): PSI < 3, Level 2 (low strain): 3 ≤ PSI < 5, Level 3 (moderate strain): 5 ≤ PSI < 7, Level 4 (high strain): 7 ≤ PSI < 9 and Level 5 (very high strain): PSI ≥ 9 [Citation21]. Mean RPE, thermal sensation, and thirst sensation responses were determined by averaging all values recorded during free play. Peak RPE, thermal sensation, and thirst sensation values are presented as the highest single measurement recorded during free play.
To examine associations between physical activity levels and changes in Tco with environmental conditions, Pearson’s correlation coefficients were calculated for comparisons between mean WBGT and the change in Tco from rest to peak and between mean WBGT and the mean METs during free play determined by video analysis. Data are presented as mean ± standard deviation. Statistical significance was set at p < 0.05. All analyses were performed using GraphPad Prism v 8.3 (La Jolla, CA, USA).
Results
Environmental conditions
The children performed an average of 218 ± 33 min of free play (Range: 150–240 min). Mean air temperature and relative humidity during free play were 24.5 ± 3.9°C and 66.2 ± 9.2%, respectively, with a corresponding mean WBGT of 22.1 ± 3.7°C.
Hydration status and body mass loss
Individual USG measurements are presented in . Of the 18 children monitored, 14 (6 girls) began free play euhydrated (prevalence: 78%; USG ≤1.020), while the remaining 4 children (1 girl) were hypohydrated (prevalence: 22%; USG ≥1.020). Conversely, at the end of free play, only 5 children (2 girls) were euhydrated (prevalence: 28%; USG ≤1.020), while 13 (5 girls) were hypohydrated (prevalence: 72%; USG >1.020). At the end of free play, body mass was reduced by 2.0 ± 1.1%.
Figure 1. Urine specific gravity (USG) prior to (Pre) and following (Post) free play. The dashed line at USG = 1.020 represents the threshold between euhydration and hypohydration based on the American College of Sports Medicine exercise and fluid replacement position stand [Citation16]. Values are presented for all children (n = 18; 7 girls)
![Figure 1. Urine specific gravity (USG) prior to (Pre) and following (Post) free play. The dashed line at USG = 1.020 represents the threshold between euhydration and hypohydration based on the American College of Sports Medicine exercise and fluid replacement position stand [Citation16]. Values are presented for all children (n = 18; 7 girls)](/cms/asset/7546393f-7e41-4d3d-b0cf-23df291801f2/ktmp_a_1801120_f0001_b.gif)
Activity levels
The proportions of free play spent at rest and at light, moderate, and vigorous levels of physical activity (METs) were 16.9 ± 12.1%, 25.4 ± 11.3%, 34.5 ± 16.5% and 23.1 ± 18.3%, respectively ()).
Figure 2. The proportion of free play (% time) spent at varying levels of (a) physical activity, (b) core temperature (°C), (c) heart rate reserve (%HRR), and (d) physiological strain index (PSI). Physical activity levels were determined by video analysis with energy expenditure estimates based on the metabolic equivalent of task, defined as the ratio of metabolic work rate to the resting metabolic rate, obtained from the Youth compendium for physical activity [Citation17]. Intensity categories for %HRR are based on the American college of sports medicine guidelines for exercise testing and prescription [Citation20]. PSI categories are based on Moran et al. [Citation21]. Values are presented for all children (n = 18; 7 girls)
![Figure 2. The proportion of free play (% time) spent at varying levels of (a) physical activity, (b) core temperature (°C), (c) heart rate reserve (%HRR), and (d) physiological strain index (PSI). Physical activity levels were determined by video analysis with energy expenditure estimates based on the metabolic equivalent of task, defined as the ratio of metabolic work rate to the resting metabolic rate, obtained from the Youth compendium for physical activity [Citation17]. Intensity categories for %HRR are based on the American college of sports medicine guidelines for exercise testing and prescription [Citation20]. PSI categories are based on Moran et al. [Citation21]. Values are presented for all children (n = 18; 7 girls)](/cms/asset/6b70ced0-c01d-4b78-b205-1520f746b356/ktmp_a_1801120_f0002_b.gif)
Body temperature and cardiovascular responses
Baseline Tco was 36.8 ± 0.3°C and during free play, the mean and peak Tco responses were 37.8 ± 0.3°C and 38.4 ± 0.3°C, respectively. All of the children achieved peak Tco ≥ 38.0°C and seven children (one girl) achieved Tco ≥ 38.5°C. The highest peak Tco response was observed in one boy who reached 38.9°C. This corresponded to 0.1 ± 0.5% of free play spent at Tco below 37.0°C, 16 ± 15% between 37.0°C and <37.5°C, 50 ± 27% between 37.5°C and <38.0°C, 28 ± 22% between 38.0°C and <38.5°C and 7 ± 14% at Tco ≥ 38.5°C ()). Further, the mean change in Tco from baseline to peak was 1.5 ± 0.4°C. Baseline mean skin temperature was 31.8 ± 1.2°C, with average and peak values during free play of 32.6 ± 1.5°C and 34.8 ± 1.4°C, respectively.
Baseline HR was 74 ± 10 bpm. The mean HR during free play was 133 ± 14 bpm, corresponding to 44 ± 10%HRR. Further, peak HR was 180 ± 12 bpm, corresponding to 79 ± 8%HRR. This corresponded to 21 ± 20% of free play spent at a very light intensity, 18 ± 11% at light intensity, 42 ± 16% at moderate intensity, 18 ± 16% at vigorous intensity, and 1 ± 4% at near maximal intensity ()).
The mean and peak PSI values were 4.7 ± 1.1 and 7.4 ± 1.1, respectively, with the mean values being near the threshold between low and moderate strain and peak values reaching a level of high strain. This corresponded to 14 ± 19% of free play spent at little or no strain, 41 ± 23% at low strain, 36 ± 20% at moderate strain, 9 ± 17% at high strain, and 0.4 ± 1.6% at very high strain ()).
Perceptual responses
Baseline thermal sensation and thirst sensation were 1 ± 1 and 3 ± 2, respectively. Mean thermal sensation, thirst sensation, and RPE during free play were 3 ± 1, 5 ± 2, and 12 ± 2, respectively. Furthermore, the children reported average peak values of 5 ± 1 for thermal sensation, 7 ± 2 for thirst sensation, and 16 ± 2 for RPE, respectively. Maximum thermal sensation, thirst sensation, and RPE scores during free play were reported by 17%, 44%, and 6% of the children, respectively.
Correlations
There was no association between mean WBGT and mean physical activity levels during free play (r = −0.391, p = 0.108) ()). Conversely, mean WBGT was positively correlated with the change in Tco from baseline to peak during free play (r = 0.488, p = 0.040) ()).
Figure 3. Correlations between (a) mean wet bulb globe temperature (WBGT) and mean physical activity levels (MET; metabolic equivalent of task) during free play, and between (b) mean WBGT and change in body core temperature from baseline to peak (∆°C) during free play. Values are presented for all children (n = 18; 7 girls), *p < 0.05
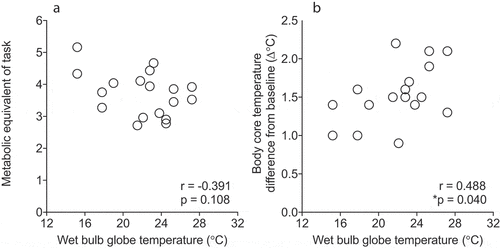
Discussion
This field study is the first to assess the level of heat strain experienced by habitually active children (10–14 years) during outdoor free play in a continental summer climate. During free play, the children spent 58 ± 15% of the time (123 ± 30 min) engaging in moderate-to-vigorous intensity physical activity. This was associated with an average increase in Tco of 1.5°C above baseline (highest peak Tco of 38.9°C) during free play along with pronounced increases in cardiovascular strain in all children. Our results demonstrate that children may experience elevated heat strain during outdoor free play even when the ambient conditions are not overly hot. This raises concerns about how children may respond under more severe environmental or exercise conditions, especially for vulnerable child populations.
Regular physical activity is an important component of the overall health and well-being of children [Citation1]. In the current study, all of the children performed 123 ± 30 min (Range: 74 to 183 min) of moderate-to-vigorous intensity physical activity during free play outdoors, which exceeded the recommended daily minimum of at least 60 minutes. While this highlights the value of free play in advancing child health, the children also experienced considerable heat strain as a result, even when the ambient conditions were relatively mild. Every child reached Tco ≥ 38°C (highest peak Tco = 38.9°C; average of 1.5°C increase from baseline), which peaked within the first 45–60 min of free play. While Tco values were typically lower for the remainder of free play, they stayed elevated by ≥1°C above baseline throughout in association with modest declines in physical activity levels over time. Similar responses were previously observed in a group of fourteen physically active children during 2 h of organized tennis training in a warm environment (WBGT, 79.9 ± 2.2°F), wherein Tco (also measured by ingestible pill) averaged 38.2°C after 40–60 min of training and remained above 38.0°C for the remainder of the session [Citation7]. Here we show that children may readily endure similar elevations in thermal strain during unstructured outdoor physical activity for up to twice as long, even in temperate conditions.
For adults, a Tco of 38°C (~1°C above resting baseline) is a commonly employed threshold for an increased risk of heat-related illness [Citation22]. Indeed, increases in Tco of as little as 0.7°C over the course of an entire work shift are associated with a four times greater chance of developing occupational heat strain compared to work in thermoneutral conditions [Citation23]. The rapid increases in Tco observed in the children during free play occurred even when the ambient conditions were relatively mild and despite the fact that they were able to reduce their physical effort if desired and were not wearing protective clothing like many adult workers. While direct comparisons of thermoregulatory responses during physical activity between children and adults remain inconclusive [Citation24], our findings raise concerns about the level of risk children may face when performing 1) physical activity in hotter ambient conditions, 2) structured activities wherein rest periods may be restricted, and/or 3) sport activities requiring protective equipment (e.g. football, lacrosse, equestrian sports, baseball, others) that would insulate the body.
Overall, there was a positive relationship between the ambient conditions (WBGT Range: 15°C to 27°C) and the Tco increase from baseline. Although, a wide variation in Tco responses was evident across all of the children despite similar mean physical activity levels over the range of ambient conditions in which they were tested. The children also reported similar mean and peak thermal sensation and RPE scores across ambient conditions, which did not appear to be influenced by the magnitude of Tco increase experienced during free play. The children primarily played in open sunlight, with brief rest periods in the shade. However, there were no discernible differences in overall sun exposure across trials that would have been likely to influence Tco or thermal sensation responses. Previous work in children during outdoor physical activity suggested that they may not demonstrate the capacity to make appropriate behavioral adjustments (e.g. self-pacing, reducing work effort and/or interspersing more rest breaks) based on their perception of heat strain [Citation25]. However, reports in adult workers show they may suffer excessive heat strain and heat stroke even when self-pacing is permitted [Citation26]. While a lack of decision-making capacity may put children at a greater risk for heat-related illness, it is also important to highlight the need to consider individual variations in a child’s physiological capacity to dissipate heat in order to identify those who are most susceptible to the deleterious effects of elevated thermal strain under different ambient conditions.
Although most of the children (78%) started testing in a euhydrated state, only 28% remained so by the end of free play. This pattern is consistent with observations in adult workers performing physically demanding jobs (e.g. mining, electric utilities) wherein the majority were severely hypohydrated by the end of a day-long work shift [Citation27]. Elevated hypohydration states are not uncommon in exercising children who do not always adequately replenish fluids during prolonged physical activity in the heat [Citation28]. The children reported mean thirst sensation scores of 5 (“Moderately Thirsty”) and average peak scores of 7 (“Very Thirsty”) during free play, with 44% reporting maximal scores of 9 (“Very, very thirsty”). Although the children clearly perceived elevated levels of thirst, they only drank 61% of the available water on average (200 ml/hr), with only one child drinking the full amount. This may be due to the majority of children not asking for water in the first hour of testing. While some children stated a preference for more water during the latter half of testing, several commented that they did not like the temperature of the water provided (maintained at 37°C to avoid confounding influences on Tco), with some opting not to drink at times for this reason. The observation that some children prioritized palatability over rehydration at times is consistent with prior work showing that adding grape flavoring to water reduced voluntary dehydration in children exercising in the heat over unflavored water, which was further reduced with the addition of carbohydrates and salt [Citation29].
Total sweat loss, as estimated by change in body mass, averaged 2.0% during free play. In adults, this amount of fluid loss is associated with a state of mild hypohydration, although 22% of the children (4 boys) demonstrated body mass changes ≥3%, which are known to exacerbate exercise‐induced hyperthermia in adults [Citation30]. Given that children have a smaller fluid volume per unit surface area, it might be anticipated that a given percentage of body mass loss from dehydration will lead to a greater attenuation in sweat secretion in this population, which generally serves to facilitate blood pressure regulation [Citation31]. In the absence of appropriate adjustments in fluid regulation, which appear to be lacking in some children based on past and current results, such an effect may amplify dehydration-induced increases in Tco during physical activity in this population. Although to our knowledge, direct comparisons with adults during controlled dehydration have yet to be conducted.
All of the children in the current study were non-obese and habitually active. Additionally, they had all been involved in various forms of outdoor physical activity in the months preceding the start of testing in early August. As such, they were likely to be partially or fully acclimatized before participating, improving their physiological capacity to dissipate heat. The study design also allowed the children to freely modulate their physical activity levels without undue influence from parents or researchers. Additionally, the relatively mild environmental conditions during many of the free play sessions compared to average temperatures in Southern Ontario during this time period represent the best-case scenario for children engaged in outdoor physical activity.
Multiple factors may inhibit a child’s ability to dissipate heat, placing some children at a greater risk for heat-related illness during physical activity, especially in the heat. These include poor aerobic fitness, obesity, inadequate acclimatization, the presence of, or ongoing recovery from an acute illness (e.g. cold, flu, infection), chronic health conditions (e.g. type I diabetes, cystic fibrosis), poor sleep quality, and others [Citation32]. As previously mentioned, competitive sporting events may also increase the risk of heat-related illness for children who are even more susceptible during practice or play in the heat [Citation4].
Existing guidelines for protecting children from heat-related illness during physical activity are currently limited and focus on typically modifiable risk factors including adequate acclimatization, sufficient fluid replacement, avoiding excessive exertion, sufficient recovery time between closely scheduled training sessions or competitive events, and removing clothing and protective equipment to minimize heat retention [Citation32]. While these guidelines provide an important common-sense approach to protecting children from heat-related illness, they do not consider the high degree of variability in how different individual children (as defined by differences in age, sex, level of fitness, health status, and other factors) may respond physiologically during physical activity in the same environment, which are not captured in the current one size fits all approach used to define heat protective measures for children. In this context, no study to date has assessed the physiological capacity of children to dissipate heat as a function of elevations in, 1) environmental heat load (higher ambient temperatures increase the requirements for heat dissipation), 2) exercise-induced heat load (increases in metabolic rate augment the rate at which heat must be dissipated) and, 3) their combination. This knowledge would help define safe exposure limits for physical activity in the heat tailored to children, which are currently lacking. This is particularly important given the current global trend of increasing average air temperatures associated with climate change, which may be more detrimental to vulnerable populations, including children [Citation33,Citation34].
In summary, we showed that during free play outdoors in a continental summer climate, children experienced a sustained elevation in heat strain, even when the ambient conditions were not overly hot. This raises concerns about how children may respond under more severe environmental or exercise conditions. Future work is necessary to establish heat stress levels where impaired heat dissipation may occur in children in order to develop more effective heat adaptation and mitigation strategies that are tailored to this potentially vulnerable population.
Author’s contribution
G.W.M. and G.P.K. conceived and designed the experiments. G.W.M., S.S., K.E.K., S.T., B.J.R., M.R.G., and E.R.M. performed data collection and analysis. All authors interpreted the results. G.W.M. prepared figures and drafted the manuscript. All authors edited and revised the manuscript. All authors approved the final version of the manuscript.
Acknowledgments
The authors would like to thank the children and their parents for their enthusiastic involvement in this study.
Disclosure statement
No potential conflict of interest was reported by the authors.
Additional information
Funding
References
- Janssen I, LeBlanc AG. Systematic review of the health benefits of physical activity and fitness in school-aged children and youth. Int J Behav Nutr Phys Act. 2010;7:16.
- Janssen I. Physical activity guidelines for children and youth. Appl Physiol Nutr Metab. 2007;32:S109–S21.
- Hallal PC, Andersen LB, Bull FC, et al. Global physical activity levels: surveillance progress, pitfalls, and prospects. Lancet. 2012;380(9838):247–257.
- Kerr ZY, Casa DJ, Marshall SW, et al. Epidemiology of exertional heat illness among U.S. high school athletes. Am J Prev Med. 2013;44(1):8–14.
- Nelson NG, Collins CL, Comstock D, et al. Exertional heat-related injuries treated in emergency departments in the U.S., 1997-2006. Am J Prev Med. 2011;40(1):54–60.
- Yeargin SW, Kerr ZY, Casa DJ, et al. Epidemiology of exertional heat illnesses in youth, high school, and college football. Med Sci Sports Exerc. 2016;48(8):1523–1529.
- Bergeron MF, Waller JL, Marinik EL. Voluntary fluid intake and core temperature responses in adolescent tennis players: sports beverage versus water. Br J Sports Med. 2006;40(5):406–410.
- Bergeron MF, McLeod KS, Coyle JF. Core body temperature during competition in the heat: national boys’ 14s junior tennis championships. Br J Sports Med. 2007;41(11):5.
- Atkin AJ, Sharp SJ, Harrison F, et al. Seasonal variation in children’s physical activity and sedentary time. Med Sci Sports Exerc. 2016;48(3):449–456.
- Kohl HW, Blair SN, Paffenbarger RS, et al. A mail survey of physical activity habits as related to measured physical fitness. Am J Epidemiol. 1988;127(6):1228–1239.
- Du Bois D, Du Bois EF. A formula to estimate the approximate surface area if height and weight be known. Arch Intern Med. 1916;17(6):863–871.
- Kavouras SA, Bougatsas D, Johnson EC, et al. Water intake and urinary hydration biomarkers in children. Eur J Clin Nutr. 2017;71(4):530–535.
- Borg G. Perceived exertion as an indicator of somatic stress. Scand J Rehabi Med. 1970;2(2):92–98.
- American Society of Heating R, Engineers A-C. Thermal Environmental Conditions for Human Occupancy: ANSI/ASHRAE Standard 55-2017 (Supersedes ANSI/ASHRAE Standard 55-2013) Includes ANSI/ASHRAE Addenda Listed in Appendix N: ASHRAE; 2017.
- Riebe D, Maresh CM, Armstrong LE, et al. Effects of oral and intravenous rehydration on ratings of perceived exertion and thirst. Med Sci Sports Exerc. 1997;29(1):117–124.
- American College of Sports M, Sawka MN, Burke LM, Eichner ER, et al. American college of sports medicine position stand. Exercise and fluid replacement. Med Sci Sports Exerc. 2007;39(2):377–390.
- Butte NF, Watson KB, Ridley K, et al. A youth compendium of physical activities: activity codes and metabolic intensities. Med Sci Sports Exerc. 2018;50(2):246–256.
- Malchaire JB. TLV work-rest regimens for occupational exposure to heat - a review of their development. Ann Occup Hyg. 1979;22(1):55–62.
- Ramanathan NL. New weighting system for mean surface temperature of human body. J Appl Physiol. 1964;19(3):531–533.
- American College of Sports Medicine. ACSM’s guidelines for exercise testing and prescription. Philadelphia, PA: Lippincott Williams & Wilkins; 2013.
- Moran DS, Shitzer A, Pandolf KB. A physiological strain index to evaluate heat stress. A J Physiol. 1998;275(1 Pt 2):R129–34.
- Kenny GP, Wilson TE, Flouris AD, et al. Heat exhaustion. Handb Clin Neurol. 2018;157:505–529.
- Flouris AD, Dinas PC, Ioannou LG, et al. Workers’ health and productivity under occupational heat strain: a systematic review and meta-analysis. Lancet Planet Health. 2018;2(12):e521–e31.
- Notley SR, Akerman AP, Meade RD, et al. Exercise thermoregulation in prepubertal children: a brief methodological review. Med Sci Sports Exerc. 2020.
- Vanos JK, Herdt AJ, Lochbaum MR. Effects of physical activity and shade on the heat balance and thermal perceptions of children in a playground microclimate. Build Environ. 2017;126:119–131.
- Cuddy JS, Ruby BC. High work output combined with high ambient temperatures caused heat exhaustion in a wildland firefighter despite high fluid intake. Wildern Environ Med. 2011;22(2):122–125.
- Meade RD, Lauzon M, Poirier MP, et al. An evaluation of the physiological strain experienced by electrical utility workers in North America. J Occup Environ Hyg. 2015;12(10):708–720.
- American Academy of Pediatrics. Climatic heat stress and the exercising child and adolescent. Pediatrics. 2000;106(1):158–159.
- Wilk B, BarOr O. Effect of drink flavor and NaCl on voluntary drinking and hydration in boys exercising in the heat. J Appl Physiol. 1996;80(4):1112–1117.
- Kenefick RW, Cheuvront SN. Physiological adjustments to hypohydration: impact on thermoregulation. Auton Neurosci-Basic Clin. 2016;196:47–51.
- Persson PB, Persson AB. Water is life. Acta Physiol (Oxf). 2018;224(2):e13173–e.
- Council Sports M. Fitness council Sch H. policy statement-climatic heat stress and exercising children and adolescents. Pediatrics. 2011;128(3):E741–E7.
- Xu ZW, Etzel RA, Su H, et al. Impact of ambient temperature on children’s health: A systematic review. Environ Res. 2012;117:120–131.
- Xu ZW, Sheffield PE, Su H, et al. The impact of heat waves on children’s health: a systematic review. Int J Biometeorol. 2014;58(2):239–247.