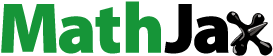
ABSTRACT
A cardiovascular requirement to facilitate thermal homeostasis may partly contribute to the elevated heart rate during eccentric cycling. This study compared the body temperature response to a bout of eccentric (ECC) and concentric (CON) cycling to account for the difference in heart rate. Eight (N = 8) aerobically trained males (age 35 y [SD 8], peak oxygen consumption 3.82 L.min−1 [SD 0.79]) completed an ECC cycling trial (60% PPO) followed by an oxygen consumption/duration matched CON trial (30, 35% RH) on a separate day. Trial termination was determined as an elevation in aural temperature, a surrogate of deep body temperature, by +0.5
during ECC. Mean skin (8-sites) and body temperature (weighting of 80:20 for auditory canal and mean skin temperature) were calculated. Matching the oxygen consumption between the trials increased external work during ECC cycling (CON: 71 [SD 14] ECC: 194 [SD 38] W, p < 0.05) and elevated aural temperature (+0.5
) by 20 min 32 s [SD 9 min 19 s] in that trial. The peak rate of rise in aural temperature was significantly greater in ECC (CON: 0.012 [SD 0.007] ECC: 0.031 [SD 0.002] oC.s−1, p < 0.05). Aural, mean skin and body temperature were significantly higher during the ECC trial (p < 0.05) and this was accompanied by elevated mean heart rate (CON: 103 [SD 14] ECC: 118 [SD 12] b.min−1, p < 0.05) and thermal discomfort (p < 0.05). Moderate load eccentric cycling imposes an elevated thermal strain when compared to concentric cycling. This requirement for dissipating heat, in part, explains the elevated heart rate during eccentric cycling.
Introduction
Skeletal muscle exerts a higher force production during active lengthening compared to either isometric or concentric contractions [Citation1]. In this state of active muscle lengthening, work is imposed upon the tissue and mechanical energy is absorbed. The fate of this energy is determined by the conditions of the lengthening muscle contraction. During normal locomotion, the lengthening muscle contraction is followed by a period of shortening, the energy can be captured by the tissue as elastic recoil and recovered. Nonetheless, if skeletal muscle is solely required to lengthen, in the active state, with large external forces and low metabolic cost (for example, walking down hill), the muscle acts as a shock absorber and the energy is then liberated as heat [Citation2].
Eccentric cycling, whereby an individual resists the forces produced by an external motor, is an excellent example of the pre-dominance of lengthening muscle contractions, and is characterized physiologically by a reduced cardiopulmonary and metabolic cost [Citation3,Citation4]. Yet, despite the acceptance that the energy cost is dramatically modified compared to the concentric equivalent [Citation5], the acute thermal responses to eccentric cycling have been extensively overlooked in favor of exploring the adaptive contractile response of lengthening skeletal muscle [Citation6].
Several studies provide a preliminary understanding of the thermal response to eccentric cycling. Nielsen (1966) studied matched metabolic heat production between concentric and eccentric cycling, and demonstrated that the core temperature of the body was similar, although sweat rate was elevated [Citation7]. In contrast, when eccentric cycling was matched to concentric cycling for a given oxygen consumption, core body temperature was increased [Citation8,Citation9,Citation10] with skin temperature and sweat rate elevated during the muscle lengthening conditions [Citation8,Citation11]. If this is indeed true, such thermal strain could partly explain the observations of an elevated heart rate when oxygen consumption is matched during eccentric and concentric cycling [Citation8,Citation10,Citation12]. This cardiovascular adjustment, to maintain mean arterial blood pressure in the face of altered vasomotor tone, is inherently linked to impaired aerobic performance in hot environments [Citation13]. On this topic, we have recently demonstrated that when eccentric cycling was used during the recovery periods of high-intensity cycling training, heart rate remained elevated, despite an oxygen consumption equivalent to concentric cycling, and this elevation in heart rate occurred in conjunction with increased mean skin temperature [Citation14]. Despite these observations, few studies have been purposefully designed to address these acute thermal differences, and only three studies have used more than three participants across varied external workloads [Citation14,Citation15,Citation16].
Therefore, the current study implemented a well-controlled thermal baseline, to determine if the aural and skin temperature responses would differ during an acute exposure to a single bout of moderate load eccentric and concentric cycling matched for oxygen consumption. We hypothesized that the rate of rise in body temperatures (aural and skin) would be faster during eccentric cycling, and as a consequence heart rate would also be elevated despite the equivalence in oxygen demand.
Materials and methods
Eight (N = 8) aerobically trained [Citation17] male participants (age 35 y [standard deviation (SD) 8], mass 71.7 kg [SD 7.4], stature 1.78 m [SD 0.08], peak oxygen consumption 3.82 L.min−1 [SD 0.79]), each regularly engaging in moderate-to-vigorous intensity physical activity per week, completed medical screening questionnaires and provided voluntary written informed consent. All procedures were approved by the University of Wollongong Human Ethics Research Committee (2019/294).
Participants visited the laboratory on three occasions to: i) determine peak aerobic power and familiarize participants to semi-recumbent eccentric cycling, ii) complete an eccentric (ECC) cycling trial set at 60% of peak power output (PPO), where trial termination criteria were set at a target temperature of +0.5°C increase in aural temperature, and iii) an oxygen matched concentric (CON) cycling trial, where trial termination was set at the identical ECC trial termination time recorded for each individual participant.
On the first visit, to determine PPO, each participant completed an incremental cycling protocol. The cycle ergometer (Excalibur sport, Lode, Groningen, Netherlands) was adjusted for each participant prior to commencing the incremental ramp protocol; cycling at 90 W and then 120 W for 2 min, thereafter workload increased by 1 W·2 s−1 until volitional termination corresponding to PPO. The highest oxygen consumption over a 30-s period was recorded as the peak (L·min−1).
On the second (ECC) and third visits (CON), participants were instrumented with a heart rate monitor, thermistors (skin and auditory canal) and oronasal mask and then rested for 20 min on the same customized recumbent ergometer [Citation18]. In brief, concentric cycling was cadence independent and controlled electromagnetically. The ergometer was also fitted with a 240 V, 0.75 kW asynchronous electric motor (MasterDrive Simovert Vector Control, Siemens, Erlangen, Germany) to facilitate eccentric cycling. Eccentric workload was monitored and measured using instrumented (0–4000 W ± 0.5%) bicycle cranks (modified Science Road Power Meter, SRM, Jülich, Germany) that allowed power (W) to be recorded (1 Hz) using a wireless display unit (Edge 530, Garmin, Kansas, USA).
Upon the commencement of cycling, ECC required each participant to maintain 60% of their PPO. This was carefully monitored using biofeedback and recorded for confirmation (Garmin Edge530, Garmin international Inc., Kansas, USA). Each participant continued to cycle eccentrically until their aural temperature increased from baseline by +0.5°C, confirmed as three consecutive 15 s data recordings. The change in aural temperature (+0.5°C) was intentionally selected so as to minimize the duration of eccentric cycling, and therefore contractile muscle fatigue. Following a minimum of 7-day recovery, the participants returned to the laboratory to complete CON, where the workload was set to achieve the equivalent oxygen consumption obtained in ECC. In the first 2 min of CON, workload was calibrated (± 5 W) with ECC to elicit an identical oxygen consumption. The CON trial was then terminated when the elapsed time recorded in ECC had been attained.
Expired gases were measured continuously and then averaged over a 15-s period for volume, carbon dioxide, and oxygen concentration using an open circuit gas-analyzer (TrueOne 2400, Parvo Medics, East Sandy, UT, USA). Prior to each use, the flowmeter and gas analyzers were calibrated using a 3-L volumetric syringe and a 2-point alpha standard gas calibration, respectively. Heart rate was obtained continuously using ventricular depolarization (800cx, Polar, Finland).
Aural (Edale instruments Ltd., Cambridge, U.K.) and skin (YSI type-EU, Yellow Springs Instruments, Yellow Springs, OH, USA) temperatures were also collected. Aural temperature was recorded using an ear-molded plug that was passively insulated (cotton wool) to shield the thermistor from the thermal environment. Skin thermistors measured temperature from eight sites (forehead, right chest, right scapula, right upper arm, right forearm, right dorsal hand, right anterior thigh, and right calf) that were used to calculate an area-weighted summation of mean skin temperature (ISO 9886, 1992). Mean body temperature was calculated using a weighting of 80:20 for auditory canal and skin temperature. All temperatures were sampled continuously (15-s intervals; 1206 Series Squirrel, Grant Instruments Ltd, Shepreth, Cambridgeshire, UK). Thermistors were calibrated against a certified reference thermometer (Dobros total immersion, Dobbie Instruments, Sydney, Australia) using a stirred water bath, and across physiologically relevant temperatures. In one subject, a representative thermal image of the skin, on the anterior thigh, was recorded with a pre-calibrated camera (Flir C2, Flir Systems, Australia) at baseline and then at the termination for each trial (equivalent time). Thermal sensation (13 point) and discomfort (13 point) and a 15-point rating of perceived exertion (RPE, [Citation19]) for the whole body, legs, and chest were recorded every 5 min during rest, exercise and at trial termination.
All participants were familiarized with the ECC cycling as previously described [Citation14]. Prior to attending the laboratory, participants were asked to consume at least 140 g of carbohydrate, and refrain from atypical strenuous physical activity, alcohol, and caffeine in the 24 hours prior to each of the experimental trials. Body mass (Model fw-150 k, A&D scale, Mercury Pty. Ltd, Thebarton, Australia) and urine specific gravity (USG) (Clinical Refractometer, Model 140, Shibuya Optical, Tokyo, Japan), were recorded upon arrival at the laboratory. All assessments were conducted in a climate-controlled laboratory set at 30 and 35% relative humidity.
This experiment followed a within-subjects study design as each participant performed ECC and CON trials. Two-way repeated-measures analyses of variance (condition x time) were used to identify any differences in physiological variables and subjective responses. The average value for the experimental measures in the last 120 s of each 5 min period and at the point of trial termination was used for statistical analysis. A Tukey’s post-hoc analysis, allowing for multiple comparisons, was utilized to determine statistical significance which was set at alpha <0.05. Data are presented as mean values and standard deviation [SD] for N = 8, unless otherwise stated.
Results
Peak power output (341 W [SD 65]) and peak heart rate (183 b·min−1 [SD 12]) achieved at the point of exhaustion of the peak aerobic power test, confirmed the cohort were aerobically trained [Citation17]. Participants reported to both the ECC and CON trials in a hydrated state (USG <1.01) and there was no difference in the starting body mass (CON: 72.86 [SD 7.66] ECC: 73.14 [SD 7.64] kg, p > 0.05). Importantly, the air temperature (CON: 29.51 [SD 0.29] ECC: 29.37 [SD 0.26] oC, p > 0.05) and relative humidity were equivalent between the trials (CON: 32.90 [SD 4.74] ECC: 35.19 [SD 3.48] %, p > 0.05).
Resting oxygen consumption (mean: CON: 0.38 [SD 0.14] ECC: 0.37 [SD 0.10] L.min−1) and heart rate (mean: CON: 68 [SD 10] ECC: 72 [SD 6] b.min−1) did not differ between the trials (p > 0.05). As predicted, matching the oxygen consumption between the trials (mean: CON: 1.36 [SD 0.30] ECC: 1.41 [SD 0.24] L.min−1) once cycling commenced ()), resulted in an approximate two and half-fold elevation in the external work during the ECC trial (CON: 71 [SD 14] ECC: 194 [SD 38] W, p < 0.05) and ratings of perceived exertion were reflective of the concentric and eccentric conditions (whole body: CON: 10.0 [SD 1.8] ECC 12.3 [SD 1.8], p < 0.05; Legs: CON: 10.1 [SD 2.0] ECC 13.4 [SD 1.5], p < 0.05; Chest: CON: 8.6 [SD 2.0] ECC 10.3 [SD 2.0], p < 0.05). Most notably and relevant for this current investigation, heart rate throughout the ECC trial was significantly elevated compared to the CON (mean: CON: 103 [SD 14] ECC: 118 [SD 12] b.min−1, p < 0.05) ()) despite the equivalent metabolic cost of both trials.
Figure 1. a: Oxygen consumption (L·min−1) and b: heart rate (b·min−1) at baseline (start) and during CON (■) and ECC (●) which were terminated at the equivalent time point (end exercise; +0.5°C increase in aural temperature during the ECC trial). † p < 0.05 CON v ECC during the period of cycling. *p < 0.05 CON v ECC trial at end exercise. Data are expressed as mean ± SD (n = 8)
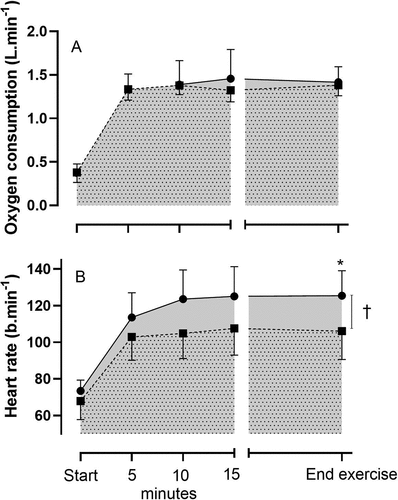
Importantly, baseline temperatures; aural (CON: 36.8 [SD 0.2] ECC: 36.9 [SD 0.3] oC, p > 0.05), mean skin temperature (CON: 33.8 [SD 0.4] ECC: 33.9 [SD 0.4] oC, p > 0.05) and mean body temperature (CON: 35.4 [SD 1.00] ECC: 35.4 [SD 0.9] oC, p > 0.05) were not different between the trials ()). At commencement of ECC, aural temperature rapidly increased at the fifth minute and continued to increase through to the termination of the trial ()). On average, the +0.5°C increase in aural temperature was achieved in 20 min 32 s [SD 9 min 19 s]. In comparison, CON resulted in attainment of a new steady state in aural temperature (lower than ECC) and at the equivalent time of trial termination (end exercise), a significantly lower aural temperature ()). The peak rate of rise in aural temperature (calculated via the peak slope during the cycling period) was significantly greater in ECC (CON: 0.012 [SD 0.007] ECC: 0.031 [SD 0.002] oC.s−1, p < 0.05).
Figure 2. a: Aural temperature (°C) b: mean skin temperature (°C) and C: mean body temperature (°C) at baseline (start) and during CON (■) and ECC (●) cycling which were terminated at the equivalent time point (end exercise; +0.5°C increase in aural temperature during the ECC trial). † p < 0.05 CON v ECC during the period of cycling. *p < 0.05 CON v ECC trial at end exercise. Data are expressed as mean ± SD (n = 8)
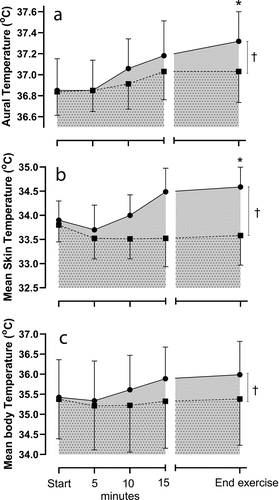
Mean skin temperature also increased in ECC and was significantly elevated compared to the CON trial ()). At the termination of the trials, independent to cycling condition, there was a significant correlation between the mean skin temperature and the heart rate (r2 = 0.43, p < 0.05). As the quadriceps were the primary muscle of activation in both CON and ECC cycling, it was also demonstrated that the skin temperature of the anterior thigh was significantly elevated in the ECC compared to CON (CON: 33.6 [SD 0.8] ECC: 34.8 [SD 1.1] oC, p < 0.05) (). More so, a color metric representation, via thermal imaging of one subject, of these surface temperatures, further demonstrated (visually) the elevated skin temperature overlying the contracting muscle during the ECC trial ().
Figure 3. Thigh skin temperature (°C) at baseline (start) and during CON (■) and ECC (●) cycling terminating at the equivalent time point (end exercise; +0.5°C increase in aural temperature during the ECC trial). A representative thermal image (from one subject) at baseline (start) and equivalent time of termination (end exercise) for ECC and CON trials visually represents the elevated skin temperature directly above the contracting muscle. † p < 0.05 CON v ECC during the period of cycling. *p < 0.05 CON v ECC trial at end exercise. Data are expressed as mean ± SD (n = 8)
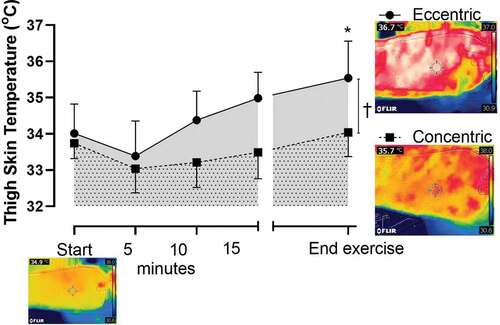
Mean body temperature also increased in the ECC trial and was once again significantly higher than oxygen matched CON cycling over the course of the trial ()). Of note, the average thermal sensation was not significantly different between the trails (CON: 8.7 [SD 0.6] ECC: 9.0 [SD 0.8], p > 0.05) although the participants’ average thermal discomfort was significantly higher during the ECC cycling (CON: 1.9 [SD 0.1] ECC: 2.6 [SD 0.1], p < 0.05).
Discussion
The acute thermal response to moderate load eccentric cycling is augmented, in association with an elevated heart rate [Citation12], compared to an oxygen equivalent concentric cycling condition. These perturbations in the body’s temperatures were most evident in the significantly faster rate of rise in the aural temperature (+0.5°C) above the starting baseline. Furthermore, mean skin and body temperature were significantly increased, along with the participants’ thermal discomfort, during the eccentric cycling and these elevations in temperature were evident within as little as 5 min from the commencement of cycling and most obvious at the time of exercise termination (target temperature) when absolute heart rate was significantly correlated with mean skin temperature.
This study explored acute thermoregulatory responses, and as a consequence heart rate, between separated bouts of continuous eccentric and concentric cycling. Exercise was matched for metabolic demand and total exercise time, determined by eccentric cycling duration to achieve an aural temperature increase of +0.5°C. Most importantly, this investigation implemented a tightly controlled cardiopulmonary and thermal baseline so as to emphasize the dynamic responses, once cycling had commenced, albeit with an offset and phase delay [Citation20]. It is commonly reported that heart rate is elevated during eccentric cycling, when compared to concentric cycling at equivalent metabolic demand [Citation8,Citation10,Citation12]. Notably, the uncoupling of cardiopulmonary responses during oxygen matched eccentric cycling and concentric cycling [Citation12] was also observed in the current study. Greater external work, and thus muscle tension, is required during eccentric exercise in order to match oxygen consumption to that of concentric [Citation5], and no doubt muscle tension (non-metabolic factor) also contributes to the observed increase in heart rate [Citation21]. Nonetheless, with large external forces and low metabolic cost of the eccentric cycling, the eccentrically contracting muscle acts as a shock absorber and the energy is liberated as heat [Citation2].
In this context, significantly higher muscle activation during eccentric cycling has been strongly correlated with a greater cardiac output and heart rate [Citation12], with suggestions of potentially greater exercising muscle temperature and redirection of blood flow to the periphery for thermoregulation [Citation12,Citation22]. This is further supported by an observed smaller arteriovenous oxygen difference during eccentric exercise at matched oxygen consumption to concentric exercise [Citation8,Citation12]. In the current study, the elevation of body temperature during eccentric cycling, although clearly different from the metabolically matched concentric cycling, was confined to the upper thermal neutral zone (mean skin temperature approaching 35°C) [Citation23]. The change in aural temperature (+0.5°C) was intentionally selected so as to minimize the duration of eccentric cycling, and therefore contractile muscle fatigue, whilst rapidly effecting a body temperature change and without meaningful dehydration (mean trial duration of 20 minutes). As such, differences in heart rate between the two trials could be more confidently assigned to thermoregulatory responses, where increased skin temperature is indicative of an increase in skin blood flow [Citation23,Citation24]. Furthermore, not only was the heart rate and mean skin temperature higher at trial termination, but independent of cycling condition, heart rate was significantly correlated with the elevated mean skin temperature (r2=0.43). Recent suggestion of elevated sympathetic nervous system activity, total peripheral resistance, and cardiac drift during prolonged eccentric cycling [Citation25] also support the occurrence of an increased thermal load during eccentric cycling.
In addition to the controlled thermal conditions of the laboratory, the establishment of a thermal baseline enabled immediate time-dependant changes to be compared once cycling commenced. It was obvious that each participant’s aural and skin temperatures increased significantly faster during eccentric cycling, so that at the point of termination, these temperatures were significantly higher as a result of the muscle lengthening activation. While aural temperature is a good surrogate for deep body temperature it is worth highlighting that esophageal temperature, tracking changes in central blood volume, would have exceeded the 0.5°C target temperature monitoring whilst also being offset [Citation20]. Limited studies from the last century confirm the observations made herein. That is, higher heart rates were observed alongside greater increases in skin blood flow and esophageal temperature during eccentric exercise [Citation10], with an increased sweat rate also observed [Citation7]. The current study design (target temperature tracking over a short time duration) was not designed for quantifying sweat rate. No doubt the initiation of sweat production and evaporation would have influenced the skin temperature response [Citation26]. Nonetheless, the establishment of a steady state, allowing for the computations of heat storage, is more appropriate for observing sweating and evaporative heat loss [Citation20], where comparison of both oxygen consumption and external work during eccentric cycling should be the goal [Citation12] of future investigations.
Thermal sensation was equivalent between both trials despite the significant elevation (+0.5°C) in core temperature during eccentric cycling, and reflects some blunting of thermal sensitivity during exercise [Citation27]. However, increased thermal discomfort was reported by participants during the eccentric cycling, presumably influenced by perturbation in skin temperature [Citation27], in what could be described as largely a normothermic state and highlights the human sensitivity to comfort [Citation28]. Nonetheless, further investigation into the perceptual experiences during eccentric cycling may prove useful when implementing this exercise into a practical setting. Although numerous studies have demonstrated that chronic exposure to eccentric exercise improves multiple factors of skeletal muscle morphology [Citation18,Citation29,Citation30], the current investigation serves as a reminder that thermal strain is increased during moderate-intensity eccentric cycling and that patient groups may have an increased risk of cardiovascular and thermoregulatory insufficiency, depending on the combination of workload, duration, and environmental conditions.
In conclusion, the current study has confirmed the hypothesis that eccentric cycling does elicit augmented thermoregulatory responses, when compared to concentric cycling at matched metabolic demand, and is a consequence of muscle acting as a shock absorber with energy liberated as heat. The elevated heart rate during eccentric cycling, acknowledging the contribution of muscle tension [Citation12], is certainly in response to modified homeostatic vasomotor function to enable the dissipation of the absorbed heat. In light of the increased recommendations for eccentric cycling to be included in exercise prescription and rehabilitation for exercise intolerant populations, it is appropriate to consider more specific thermoregulatory responses in both healthy and patients groups, in order to understand the contribution of body temperature to cardiovascular strain and thermal sensation and comfort during eccentric cycling.
Author contribution
GP, HG, and CB conceived and designed research. TE, GP, AH, and CB conducted experiments and data analysis. All authors and wrote the manuscript. All authors read and approved the manuscript.
Acknowledgments
This research did not receive any specific grant from funding agencies in the public, commercial, or not-for-profit sectors.
Disclosure statement
There are no personal, professional, or financial conflicts of interest for any of the authors of this submitted work.
References
- Hill AV. The heat of shortening and the dynamic constants of muscle. Proc R Soc Lond. 1938;126:136–195.
- Lindstedt SL, LaStayo PC, Reich TE. When active muscles lengthen: properties and consequences of eccentric contractions. News Physiol Sci. 2001 Dec;16:256–261.
- Abbott B, Bigland B, Ritchie J. The physiological cost of negative work. J Physiol (Lond). 1952;117:380–390.
- Bigland-Ritchie B, Wood J. Integrated electromyogram and oxygen uptake during positive and negative work. J Physiol (Lond). 1976;260:267–277.
- Dufour SP, Lampert E, Doutreleau S, et al. Eccentric cycle exercise: training application of specific circulatory adjustments. Med Sci Sports Exerc. 2004 Nov;36(11):1900–1906.
- LaStayo P, Marcus R, Dibble L, et al. Eccentric exercise in rehabilitation: safety, feasibility, and application. J Appl Physiol. 2014 Jun;116(11):1426–1434.
- Nielsen B. Regulation of body temperature and heat dissipation at different levels of energy and heat production in man. Acta Physiol Scand. 1966;68(2):215–227.
- Davies C, Barnes CJ, Work. II. Physiological responses to walking uphill and downhill on a motor-driven treadmill. Ergonomics. 1972;15(2):121–131.
- Nadel ER, Bergh U, Saltin B. Body temperatures during negative work exercise. J Appl Physiol. 1972;33(5):553–558.
- Nielsen B, Nielsen SL, Petersen FB. Thermoregulation during positive and negative work at different environmental temperatures. Acta Physiol Scand. 1972;85(2):249–257.
- Smiles KA, Robinson S. Regulation of sweat secretion during positive and negative work. J Appl Physiol. 1971;30(3):409–412.
- Dufour SP, Doutreleau S, Lonsdorfer-Wolf E, et al. Deciphering the metabolic and mechanical contributions to the exercise-induced circulatory response: insights from eccentric cycling. American J Physiol Regul Integr Comp Physiol. 2007 Apr; 292;4:R1641–R8.
- Cheuvront SN, Kenefick RW, Montain SJ, et al. Mechanisms of aerobic performance impairment with heat stress and dehydration. J Appl Physiol (1985). 2010 Dec;109(6):1989–1995.
- Harrison AJ, Burdon CA, Groeller H, et al. The acute physiological responses of eccentric cycling during the recovery periods of a high intensity concentric cycling interval session. Front Physiol. 2020;11:336.
- Pahud P, Ravussin E, Acheson KJ, et al. Energy expenditure during oxygen deficit of submaximal concentric and eccentric exercise. J Appl Physiol. 1980;49(1):16–21.
- Westerlind KC, Byrnes WC, Harris C, et al. Alterations in oxygen consumption during and between bouts of level and downhill running. Med Sci Sport Exercise. 1994;26(9):1144–1152.
- De Pauw K, Roelands B, Cheung SS, et al. Guidelines to classify subject groups in sport-science research. Int J Sports Physiol Perform. 2013 Mar;8(2):111–122.
- Lewis MC, Peoples GE, Groeller H, et al. Eccentric cycling emphasising a low cardiopulmonary demand increases leg strength equivalent to workload matched concentric cycling in middle age sedentary males. J Sci Med Sport. 2018 Dec;21(12):1238–1243.
- Borg G. Psychological basis of physical exertion. Med Sci Sport Exercise. 1982;14(2):377–381.
- Taylor NA, Tipton MJ, Kenny GP. Considerations for the measurement of core, skin and mean body temperatures. J Therm Biol. 2014 Dec;46:72–101.
- Lichtneckert SJA, Thomson DA, Colliander Y. Influence of muscle tension variations and energy absorption on oxygen consumption, heart rate, and cardiac output during negative work. Scand J Clin Lab Invest. 1971;27(3):201–205.
- Peñailillo L, Blazevich AJ, Nosaka K. Factors contributing to lower metabolic demand of eccentric compared with concentric cycling. J Appl Physiol. 2017 Oct 1;123(4):884–893.
- Savage MV, Brengelmann GL. Control of skin blood flow in the neutral zone of human body temperature regulation. J Appl Physiol. 1996;80(4):1249–1257.
- Rowell LB, Marray JA, Brengelmann GL, et al. Human cardiovascular adjustments to rapid changes in skin temperature during exercise. Circ Res. 1969;24:711–724.
- Ritter O, Isacco L, Rakobowchuk M, et al. Cardiorespiratory and autonomic nervous system responses to prolonged eccentric cycling. Int J Sports Med. 2019;40(7):453–461.
- Sawka MN, Leon LR, Montain SJ, et al. Integrated physiological mechanisms of exercise performance, adaptation, and maladaptation to heat stress. Compr Physiol. 2011 Oct;1(4):1883–1928.
- Flouris AD, Schlader ZJ. Human behavioral thermoregulation during exercise in the heat. Scand J Med Sci Sports. 2015 Jun;25:52–64.
- Kingma BR, Frijns AJ, Schellen L, et al. Beyond the classic thermoneutral zone: including thermal comfort. Temperature. 2014 Jul-Sep;1(2):142–149. doi:10.4161/temp.29702.
- Chen TC, Hsieh CC, Tseng KW, et al. Effects of descending stair walking on health and fitness of elderly obese women. Med Sci Sports Exerc. 2017;49(8):1614–1622.
- LaStayo PC, Pierotti DJ, Pifer J, et al. Eccentric ergometry: increases in locomotor muscle size and strength at low training intensities. American J Physiology-Regul Integr Comp Physiol. 2000 May;278(5):R1282–R8.