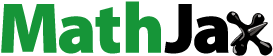
ABSTRACT
Heat strain impairs performance across a broad spectrum of sport disciplines. The impeding effects of hyperthermia and dehydration are often ascribed to compromised cardiovascular and muscular functioning, but expert performance also depends on appropriately tuned sensory, motor and cognitive processes. Considering that hyperthermia has implications for central nervous system (CNS) function and fatigue, it is highly relevant to analyze how heat stress forecasted for the upcoming Olympics may influence athletes. This paper proposes and demonstrates the use of a framework combining expected weather conditions with a heat strain and motor-cognitive model to analyze the impact of heat and associated factors on discipline- and scenario-specific performances during the Tokyo 2021 games. We pinpoint that hyperthermia-induced central fatigue may affect prolonged performances and analyze how hyperthermia may impair complex motor-cognitive performance, especially when accompanied by either moderate dehydration or exposure to severe solar radiation. Interestingly, several short explosive performances may benefit from faster cross-bridge contraction velocities at higher muscle temperatures in sport disciplines with little or no negative heat-effect on CNS fatigue or motor-cognitive performance. In the analyses of scenarios and Olympic sport disciplines, we consider thermal impacts on “motor-cognitive factors” such as decision-making, maximal and fine motor-activation as well as the influence on central fatigue and pacing. From this platform, we also provide perspectives on how athletes and coaches can identify risks for their event and potentially mitigate negative motor-cognitive effects for and optimize performance in the environmental settings projected.
Introduction
The climatic conditions for the upcoming Tokyo Olympic Games (Tokyo 2021) are expected to set the scene for one of the hottest summer games in the modern era with particular heat problems related to the hot humid conditions expected for many of the venues [Citation1–4]. With climate changes and a rise in the mean global temperature expected to continue toward the end of the 21st century [Citation5,Citation6], future Summer Olympics and other major sport events are likely facing frequent and escalating environmental heat-load scenarios. All sports ranging from endurance to strength- and cognitively-dominated disciplines are susceptible to the detrimental effects of heat stress related to elevated environmental air temperature [Citation7,Citation8], high humidity [Citation9,Citation10], and solar radiation [Citation11–13]; although via quite different mechanisms. These may include hyperthermia-induced impacts on the central nervous systems (CNS fatigue or function) or peripheral (cardiovascular and muscular) effects and operate both independently as well as in interaction [Citation7,Citation8,Citation14–18]. While heat-related cardiovascular strain and the resultant limitation to oxygen delivery will impair performance in several endurance disciplines, short-lasting disciplines (e.g., sprint and precision disciplines) are unlikely to be affected by compromised aerobic energy turnover [Citation7,Citation15–24]. However, these disciplines may be affected by hyperthermia in combination with hydration and solar radiation effects on CNS function and central fatigue [Citation11–13,Citation22,Citation25–27] with the potential negative effects depending on the interaction between the exposure scenario (indoor/outdoor environmental settings; duration and timing of the event) and the combined effects on the physiological and motor-cognitive parameters of importance for performance in the given sport.
For endurance sports, it is well established that environmental heat stress may impede the aerobic exercise capacity [Citation19] as the cardiovascular load provoked by thermal stress may eventually limit arterial oxygen delivery to the exercising muscles [Citation7,Citation8,Citation18,Citation23–25]. In contrast, short duration activities may benefit from elevated environmental temperatures, as CNS drive does not seem to be impaired during short maximal efforts (e.g. repeated short maximal voluntary contractions [MVCs] [Citation8] or sprints [Citation28,Citation29]), as muscle function (i.e. faster muscle cross-bridge cycling) is facilitated by elevated muscle temperatures [Citation7]. However, central fatigue (inadequate neural drive of motoneurons to secure optimal muscle activation) may be an issue during prolonged efforts [Citation8,Citation19,Citation30,Citation31] and repeated sprints [Citation28,Citation29,Citation32]. Furthermore, during long-lasting events, dehydration and exposure to high levels of solar radiation (including effects from ultra-violet [UV]-radiation exposure [Citation33] and the thermal load from the entire spectrum of visible and non-visible light) may also become a problem for athletes. Furthermore, dehydration have shown to hamper exercise performance [Citation20,Citation26,Citation27,Citation34–37], due to impeded thermoregulation [Citation8,Citation22,Citation25,Citation37–42] and lowering of cerebral blood flow [Citation25,Citation43,Citation44] and solar radiation (≥ 800 W/m2) have been shown to negatively affect exercise performance [Citation11–13].
In addition to the central and peripheral factors affecting hyperthermia related fatigue, it is also important to consider the effect of heat-strain on central nervous processes underlying perception, decision-making and motor output [Citation45]. We use the terms motor-cognitive and cognitively-dominated performance as the vast majority of actions in all aspects of life and particularly in sports, involve both motor outputs orchestrated by the CNS as well as cognitive processing. The impact of hyperthermia on motor-cognitive performance seems to be determined by both the complexity of the task and the rise in internal (deep body core) temperature. Neither high environmental temperatures per se nor minor to moderate elevations in deep body core temperature (~1°C above resting levels), hamper cognitive tasks performance [Citation46–56]. However, if individuals develop severe hyperthermia (i.e. more than 2°C increase above rest), complex task performance deteriorate [Citation47–50,Citation52,Citation56–60]. Importantly, performance is particularly affected when tasks involve alternating and/or complex motor-cognitive elements, whereas more stereotypical simple tasks are less affected [Citation61]. Furthermore, modest levels of dehydration can aggravate the influence of hyperthermia on complex motor-cognitive performances [Citation62–70], and also simple motor tasks are negatively affected when dehydration is superimposed on hyperthermia [Citation66,Citation70–72]. Another important factor to consider is solar radiation, as we recently provided evidence suggesting that high levels of simulated sunlight (from ~800 to ~1000 W/m2 – especially directed toward the head) have a negative impact on the performance of a complex motor-cognitive task [Citation73]. In addition, Otani and colleagues recently provided evidence that solar radiation effected endurance capacity [Citation13] and self-regulated exercise intensity [Citation12]. When combined with direct exposure of the head to strong (simulated) sunlight modest hyperthermia (~1°C above baseline core temperature) induces a significant impairment in motor-cognitive performance, otherwise not observed with similar radiative heat loads applied to the lower body [Citation73].
Taken together, environmental heat stress, hyperthermia and derived factors such as dehydration may therefore not only impact sport performance, due to aggravated cardiovascular strain, peripheral or central fatigue (see proposed integrative fatigue model in ref #56), but also influence sports performance via effects on fine sensorimotor control and cognitively dominated functions. To provide a framework for evaluating this impact and identify sports of particular relevance (vulnerable to heat stress related impacts on motor-cognitive performance), we evaluate how the environmental conditions expected for the upcoming Olympic games may influence the different sport disciplines depending on exposure (duration and integrated environmental and exercise-induced heat stress) and complexity of the sport. To this aim, we propose a model/framework that can be used to translate laboratory motor-cognitive findings (described above) into heat effects on sports performance in ecological settings. This involved a rough categorization of all sport disciplined based on their complexity via evaluation of 3 main parameters: 1) predictability of the sport (ranging from simple and closed tasks to open tasks where appropriate motor-activation continuously alternates depending on the sensory environment in the specific situation), 2) the demands for decision-making (e.g., tactical aspects), and 3) the technical difficulty of movements. The three parameters are independently scored from low [Citation1] to high [Citation4] and combined into one score (i.e. each discipline receive an accumulated score ranging from 3 to 12 points), signifying the overall task complexity and divided into the following subcategories: simple [total score ≤6], moderate [scores from 7 to 9] and complex [scores from 10 to 12] (see methods section for detailed description). To determine the integrated (exercise and environmental) heat stress, we utilized the Predicted Heat Strain (PHS) model in combination with the estimate heat stress of Tokyo in all individual sports included in the Summer Olympics. Combining the complexity categorization with the PHS model (allow risk assessment of athletes becoming dehydrated, developing high levels of hyperthermia or moderate hyperthermia or in combination with exposure to solar radiation), we evaluate the integrated heat stress risk for impairment in motor-cognitive performances for the sports included in the Tokyo Summer Olympics games 2021. Several of the issues are indeed also relevant for future and the current para-Olympics (as high heat exposure also will be relevant for this event [Citation74]), however, since the PHS model and evidence for motor-cognitive effects have only been investigated in able-bodied participant we have confined the analyses to this group of athletes.
Methods
Methodological approach
As outlined in the introduction, we use categorization of sports complexity in combination with risk assessment for developing hyperthermia, risk of developing dehydration and level of solar radiation exposure in each of the sports in Tokyo 2021, given the forecasted scenarios (projected climatic scenarios).
Assessing the integrated environmental and exercise induced heat strain
We used a modified version of the Predictive Heat Strain model (PHS [Citation75]; – described below) to evaluate the integrated heat strain (considering the combined metabolic and environmental heat load) as well as estimating the risk of developing dehydration (estimating sweat rates and considering the duration and ability to hydrate during a given competition) for each sport [or range of disciplines]. The environmental input parameters (air temperature, wind speed, humidity and solar radiation) for the upcoming 2021 Tokyo Olympic Games were average hourly meteorological parameters, based on the two week period (24th July to 9th August) from 19 years of historical data extracted from Gerrett et al. [Citation1]. For full method see Gerrett et al. [Citation1] and data summarized in (see below). To calculate the mean radiant temperature, we used the solar radiation values per hour of day for outdoor events (full sun exposure), while no solar radiation was assumed for indoor events (or scenarios with full shadow). Subsequently, mean radiant temperature was determined from the calculated black globe temperature (Tglobe) using the Liljegren and colleagues method [Citation76] and substituting the calculated Tglobe for mean radiant temperature as recommended by Ramsey and Bernard [Citation77]. For all other environmental parameters (air temperature, air velocity and humidity), the mean value for that hour of day was used. For outdoor events the air velocity at 10 m was corrected to 2 m air velocity using the equation: vair,2m = 0.67 * vair,10m assuming that the ground surface is neither heated by radiation or cooled in an urban environment [Citation76]. Furthermore, the PHS model simulation used the following input parameters: person (standing), body mass 75 kg, height 1.80 m, metabolic heat production between 100–1200 W (based on categorization, estimates of sports with low/medium/high metabolic heat production), assuming that athletes were fully heat acclimated, allowed to freely ingest fluid (i.e., maintain hydration), dressed in clothing with low insulation (0.4 Clo units; This CLO value was kept constant for the analyses illustrating the importance of exercise duration and timing of the event (), as well as the difference between indoor and outdoor setting (). For unique disciplines where special sport clothing is required (e.g., protective helmets and clothing or safety-west in sailing) the value may be higher and considered in the qualitative consideration for the sport), static moister permeability index of 0.38, fraction covered by reflective clothing equal to 0.54, emissivity of reflective clothing set to 0.97.
Table 1. Meteorological input parameters for the predicted heat strain model (outdoor assuming maximal radiation and indoor no radiation or wind)
Table 2. Metabolic heat production, environmental conditions, event duration and motor-cognitive complexity for each sport
With the specified assumption the simulations to predict hyperthermia (core/rectal temperature responses) and levels of dehydration for different scenarios and durations were run with a modified version of the PHS model (PHS, ISO7933 [Citation78]) that allows metabolic heat production to exceed 400 W/m2 as relevant for most of the sports of interest.
The potential motor-cognitive impact, as discussed in the introduction, is dependent on the extent of hyperthermia the athlete may develop, as well as the presence and strength of any exacerbating factors, such as dehydration or superimposed radiation [i.e., direct sunlight exposure [Citation61,Citation66,Citation70];]. Precise thresholds have not been established, but based on the available evidence [i.e., how motor-cognitive performance are negatively affected [Citation61,Citation66,Citation73]], together with the work of Morrison and colleagues [Citation79], showing that gradual increase in core temperature have an inverse relationship with voluntary activation and psychological strain]. Based on the aforementioned, we believe it is reasonable to expect a graded transition from values (dehydration level, solar radiation exposure of the head and level of hyperthermia) with no impact on motor-cognitive function to values for each parameter that will provoke high/maximal negative effects. For the estimation of the integrated motor-cognitive impact level (MCIL; that subsequently is considered in combination with the complexity of the activity – i.e., the discipline’s vulnerability to MCIL) the following graded function was assumed:
Where is body rectal temperature and
(W/m2) is direct solar radiation (see ).
Figure 1. Relation between rectal core temperature, solar radiation and the risk categories for motor-cognitive impact (MCIL). X-axis rectal temperature (°C) and Y-axis solar radiation level (W/m2). Green i.e. low risk (MCIL≤0.5), Yellow for moderate risk (0.5< MCIL <1.5) and red for high risk (MCIL >1.5) for impact on motor-cognitive performance
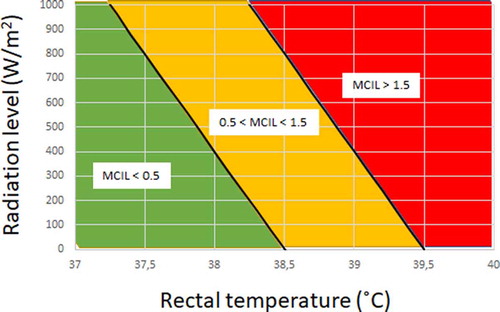
The motor-cognitive impact level (MCIL) can be interpreted as follows:
Low MCIL ≤ 0.5
full shade conditions and
[Citation46–56].
With increasing solar radiation low cognitive impact can appear at lower
Moderate 0.5 < MCIL < 1.5
graded impact between low and high cognitive impact level.
full shade conditions starting from
°C [Citation47–50,Citation52,Citation56–59,Citation61,Citation80]
with increasing solar radiation moderate cognitive impact can occur at
[Citation12,Citation13,Citation73]
High MCIL
1.5
full shade conditions and
[Citation47–50,Citation52,Citation56–59,Citation61,Citation80]
with increasing solar radiation levels high cognitive impact can occur at
for high solar radiation
800 W/m2, the
[Citation73]
The above model is based on numerous observations, where baseline conditions are compared with moderate hyperthermia (references # 47, 55, 61) or baseline is compared to acute exposure to radiation (but with a normal core temperature; ref #73), where we observe no impairment in motor-cognitive [green level]. Whereas, severe hyperthermia per se [Citation47,Citation55,Citation61] or moderate hyperthermia (core temperature in the range 38–38.5°C) in combination with exposure to high levels of solar radiation [Citation73] provoke a marked effect on motor-cognitive performances [red level in ]. Clear thresholds have not been experimentally established and the transition from one level to the next is most likely graded. We therefore indicate the likelihood of motor-cognitive impact (presented in ) as graded change in color over a range of activity levels, times of day, and activity durations. This part of the analysis is intended as an overview that allows the athletes and sports coaches to estimate risk for their sport/activity, and it may depend on timing of the event. This can be done by drawing a line vertical (intensity) and horizontal (time of day) will indicate the magnitude of impact (green, yellow, and red) on motor-cognitive performance and CNS fatigue.
Figure 2. Motor-cognitive Impact Level with varying levels of metabolic heat production across the day for event with a) duration of 15 min. panel b) 30 min. exposure, panel c) 45 min. exposure and panel d) 120 min. exposure. X-axis time of day, Y-axis (right) is metabolic heat production in watts and Y-axis (left) motor-cognitive impact level. Green (no impact), Yellow (moderate impact) and red (high impact) on motor-cognitive performance
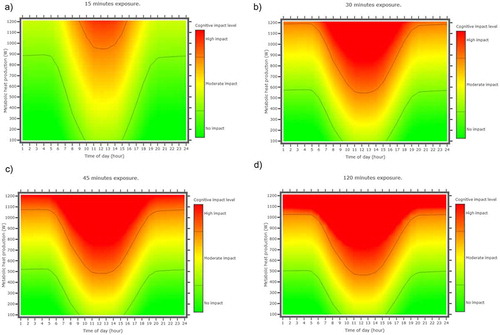
Combining PHS-predictions with motor-cognitive complexity
The PHS-simulations serve to predict the potential risk for motor-cognitive impairments, but the impact on performance will also depend on the complexity of the motor-cognitive tasks of the given discipline, i.e. the sport/task vulnerability to heat stress impacts. Therefore, all sports were evaluated on the following four parameters: 1) the environment (indoor/outdoor – time of day), 2) the mean metabolic heat production for the above-specified “representative athlete” 3) the duration of the competition (single match or series of competitions where relevant), and DL i.e. 4) the complexity categorization of the sport/discipline (see and description below).
Categorization of sports “motor-cognitive” complexity
Under the umbrella term “motor-cognitive complexity”, we attempt to provide an overarching metric that encompasses the demands for sensory acuity, motor precision and movement difficulty, as well as the extent of decision-making. The overall evaluation is based on categorical scores on these parameters, adapted with the purpose of providing the readers and practitioners with an easy-to-interpret metric that can aid in pinpointing which disciplines may be particularly affected by heat stress during the 2021 Summer Olympics. The scoring parameters chosen for our categorization system were selected to encompass the complex processes entailing sensory processing and predictions, cognitive involvement, and motor execution. We chose these categories since they outline the factors that may render performance of a given sport susceptible to the impeding effects of hyperthermia, dehydration, and solar exposure. It is important to note that despite the below introduced parametrization of the various Olympic discipline, we fully acknowledge that these parameters are not independent. As an example, a street skater that early in the spin trick makes a precise (implicit) prediction of where and how she/he will land, enables an earlier preparation leading to improved execution of the landing, which frees attentional and mental resources to decide how to approach the next obstacle [Citation81].
An archetypal discipline with a vulnerable combined sensory-cognitive-motor profile is a sport that calls for early, precise sensory predictions based on high spatial and temporal acuity in the sensory processing, quickly choosing between several possible actions and finally carrying out movements with complex kinematic patterns with high velocity and accuracy. Moreover, disciplines that involved rapid changes in involved sensory, cognitive and motor components would be more susceptible to performance decrements than one that call for uniform thinking and moving.
Predictability of sensory inputs and events
The first parameter termed Predictability of inputs and events spans from 1) Completely predictable, closed motor skills that are carried out without paying attention to external influence, 2) Highly Predictable closed motor skills that may be impacted by extrinsic factors, 3) Partly unpredictable open motor skills that are at least in part subject to feedback control and finally 4) open motor skills that to a large extent rely on sensorimotor reactions to a rapidly changing and largely unpredictable sensory environment. Specifically, a 100 m dash (score 1) can be carried out in a similar manner regardless of the direction of the wind, whereas an archer (score 2) needs to attend to changes in wind direction and velocity. During a cycling (road) race the athlete needs to continuously adjust her/his pace to match the terrain and accommodate the actions of teammates and opponents (score 3), whereas the strain on acute, fast and accurate sensory predictions and reactions is less than that of a tennis player (score 4). Obtaining a good position to return a serve is contingent on early and fast computation of the likelihood for the serve to end up high or low in the for- or backhand but also for last (milli)second adjustments to account for the unexpected spin of the ball [Citation82].
Decision-making
The second parameter describes the need for decision-making in the broadest interpretation of the term including continuous ad hoc decisions in interceptive sports but also major tactical changes. The 4 categories of the Decision-making parameter spans from disciplines with 1) no need for pre hoc tactics and no demand for decision-making over 2) Few tactical deviations with minor impact may occur and 3) Updating of tactics during competition without significant time distress to 4) continuous high demands on updating tactics and/or fast decision making.
Lifting a weight in the context of a competition is carried out with feedforward control and only affected by gravity and internal force. The athlete can visualize the lift from start to end and if the coordination and power suffice, the lift will be successful. The tactical, cognitive, and decision-making components in the execution of a lift are marginal at best (score 1). A marathon runner may change the pre-race plan if a rival chose to break away from the group (score 2). A golf player has ample time between two holes to decide whether to attempt a high risk, high gain shot or playing it safe. The original plan may have been to aim for a par but the boogie on the previous hole may call for a riskier option to stay in run for the medals (score 3). The captain of a 49er (Sailing) follows a pre-race strategy but encounters emergent decision including both the starting position and the continued decisions on trajectories based on interactions between environmental constraints and interaction with competing boats (score 3; see ref [Citation83]). A football player is faced with a multitude of decisions during a game. E.g. should the ball be passed (if so where to?) or should the player attempt a goal on his/her own (which type of kick and which in side/corner of the goal to direct the shot?). These decisions are often made, within a fraction of second, as an opponent is closing in or the window of opportunity may be closing and they are based on the position of the ball, opponents, teammates but also the weight distribution and perceived balance of the player (score 4).
Movement skills and their difficulty
Even the simplest movements can be difficult if the need for precision is high. Reversely, relatively complex kinetic patterns such as human gait can be considered easy, as they are commonly used in everyday life and mastered early in life. Therefore, we have reserved the lowest scoring category for disciplines where the movement is uniform, and the kinematic patterns of experts and novices are somewhat comparable. The four categories distinguish between 1) disciplines solely involving rhythmic movement patterns such as walking or running, 2) some non-basic technical element such as swimming and 3) disciplines contingent on high spatial precision and small errors without significant time constraints as well as disciplines combining sequences of movements that in isolation have simple kinetic patterns. Finally, 4) movements characterized by either complex kinematics sequences and/or shifting between different effectors and kinetic demands.
As examples: running and biking comprises basic mainly rhythmic movement (score 1), whereas swimming, and rowing have higher technical demands imposed on the uniform rhythmic movements and steeplechase adds a simple technical element on top of the basic movement pattern (score 2). Performance in the Olympic shooting disciplines depends on motor precision and consistency with little room for errors (score 3). Although long jumping is a technical athletic discipline, each of the movement in the sequence are relatively simple (score 3) whereas e.g. pole vault or skating disciplines involves rotation around multiple axes, inter-segment coordination and different kinematic patterns exemplified by the difference between stabilizing the skateboard and body during a slide versus high velocity rotation during spins (score 4).
All authors independently evaluated each sport, and the average overall categorization is presented in . For simplicity this presented as grouping of sports into 3-sub groups (based on the summarized score of predictability, decision-making and movement-difficulty) with low representing a score below 6, moderate [for scores 7–9] and high complexity [Citation10–12]) as this grouping was considered relevant for the MCIL risk assessment – i.e. if tasks are likely to have low, moderate or high vulnerability to heat stress impacts on motor-cognitive performance for a given MCIL (see Appendix 1 for overview of individual sport scores). In addition, in Appendix 1 mean and range the scores given by the seven authors are displayed (see numbers between brackets) and interclass correlation coefficient (ICC) for the average motor-cognitive complexity score were calculated in accordance with Koo and Li [Citation84] to 0.92 indicating a high level of agreement.
Results
Importance of duration, intensity and event timing (time of day) for outdoor disciplines
illustrates how the risk for motor-cognitive impairments are influenced by duration of the discipline (competition time), with high risk (“red alert”) for short events only relevant for intense disciplines (high endogenous heat production) scheduled for midday, but with the risk of heat-impact increasing and spreading across the day as discipline duration increases from 15 to 45 min, but no further increase in MCIL-risk by prolonging the duration to 2 hours (assuming similar hydration levels). The figure also illustrates the importance of event timing with high impact in the middle of the day where the environmental conditions both via impact on overall heat-balance (risk of hyperthermia for given metabolic heat production) and via the separate impact/risk related to sunlight exposure (radiation) is high. As discussed later the risk-levels should be considered in combination with the sport complexity and athlete’s ability to prevent dehydration or mitigate direct exposure of the head (see and for illustration of radiation/shading effects).
Table 3. Overview of the potential motor-cognitive impacts across all Olympic disciplines with specific analyses/evaluations of risk “directly” related to hyperthermia, risk for developing dehydration (BW-loss exceeding 2%) and exposure to solar radiation. With consideration for each factor and evaluation of the integrated scenario and combined risk effect on motor-cognitive performance and/or central fatigue
Figure 3. Motor-cognitive Impact Level of metabolic heat production vs. Time of day – Maximum radiative exposure and Full shade. Panel a) maximal solar radiation [120 min exposure] and panel b) full shade condition. X-axis time of day, Y-axis (right) is metabolic heat production in watts and Y-axis (left) motor-cognitive impact level. Green (no impact), Yellow (moderate impact) and red (high impact) on motor-cognitive performance
![Figure 3. Motor-cognitive Impact Level of metabolic heat production vs. Time of day – Maximum radiative exposure and Full shade. Panel a) maximal solar radiation [120 min exposure] and panel b) full shade condition. X-axis time of day, Y-axis (right) is metabolic heat production in watts and Y-axis (left) motor-cognitive impact level. Green (no impact), Yellow (moderate impact) and red (high impact) on motor-cognitive performance](/cms/asset/27ef7017-e359-42df-9199-6b770ccbface/ktmp_a_1957367_f0003_oc.jpg)
illustrates the effect of open sun exposure (panel a; assuming solar radiation as outlined in ; i.e. no shading) versus shaded outdoor or indoor conditions (assuming full shade, but no air conditioning or other artificial cooling; panel b) for the longest calculated exposure duration (120 min). Comparing panel a) and b) illustrates that with full shade (even during hours with peak air temperature; midday) high risk for impairments in motor-cognitive performance (red area) will only be relevant in sports with very high metabolic heat production.
From general risk to impact on specific sport disciplines
Based on the general MCIL assessment (presented in ), combined with the risk for dehydration levels exceeding 2% and the previously described complexity categorization ( and Appendix 1) we outline the level of risks for each sport and sub-disciplines in (see below).
As summarized in the last column of , there are a range of sports were no (or very low/limited) impact is expected; either because the heat exposure is low (indoor or short duration events) and the athlete’s risk for hyperthermia in Tokyo 2021 will not be different from competitions in cooler conditions or sport disciplines where hyperthermia has little (or no) detrimental influence on performance. The latter include sprinting disciplines and sports involving short-explosive effort, as they benefit from faster contraction velocities related to higher muscle temperatures without suffering from motor-cognitive impacts (as negative effects related to motor-cognitive factors or CNS fatigue are mainly seen during prolonged efforts).
Amongst the sports at the other range of the risk scale (i.e., potential high influence from elevated heat stress) are the prolonged outdoor ball-games, e.g., field hockey and football where the “complexity” is high as performance rely on both tactical decision-making and continuous adjustment of the motor-activation to varying situations. In those sports, the long duration and elevated metabolic heat production markedly increase the susceptibility for developing hyperthermia and the exposure to solar radiation or hazard for developing dehydration adds additional risk.
In the middle range risk category (moderate/potential effects expected, but partly preventable) are sports where the athletes either have a lower metabolic rate (e.g., golf) or better potential to seek shadow and hydrate during breaks (e.g. tennis and beach volleyball) or where the task complexity is considered to be lower (sports dominated by closed skills).
Discussion
The framework and associated analyses presented in this paper allow for short and long-term projections of the environmental settings to be combined with physiological considerations relevant for impact on motor-cognitive performance and how this may influence various sport disciplines in specific events such as the Summer Olympic Games. The “general model” should of cause be interpreted and used as a conservative “rough risk-level assessment” that integrates the intensity, duration, and scheduling (time of day) with the forecasted environmental settings (for Tokyo 2021 or any future events with expected heat stress). The event organizers may use such information for planning purposes, while participants and coaches may identify the risk and importance of specific parameters for their competition. The general risk assessment should be combined with specific considerations for the sport and in the combined evaluation (), we therefore provide quantitative and detailed considerations for the different sports and their vulnerability to the four main risk factors – i.e., risk of developing severe hyperthermia, being exposed to high levels of radiation or moderate levels of hypohydration and the complexity of the given sport. As illustrated in , motor-cognitive impairments are likely to be negligible in short-duration sports, however, the intensity, setting and scheduling of the events are important in sports lasting longer than 30 min. From this perspective, it is interesting that the women’s football finale is pre-scheduled to be played around midday (Friday the 6th August at 11am [Citation85]), when temperatures are at their hottest [Citation1]. As outlined in , football is a highly vulnerable sport, due to the high-level decision-making requirements, elevated metabolic rate rates, prolonged playing time, exposure to solar radiation, and risk of dehydration. Indeed, the average dehydration-level in football players is ~2% following matches in both moderate and severe heat stress scenarios [Citation86]. Moreover, women maybe at greater risk for developing hyperthermia [Citation87,Citation88] or forced to lower the pace to maintain heat balance as there is some evidence suggest that women have a lower evaporative heat loss capacity [Citation89,Citation90], particularly at high exercise intensities, which further increases their risk for motor-cognitive impairments and brings into question the logic behind scheduling the event for this time.
For indoor sports (e.g., basketball and handball) or outdoor sports (e.g., tennis and beach volleyball) with better access to resources (such as water and shaded rest areas) and/or have regular breaks in play, the risk for impairments may be reduced – especially if athletes adopt appropriate mitigation measures (see discussion below). Endurance sports with low complexity scores (closed tasks or fewer changes in the motor activation pattern) such as distance running, rowing, time trial cycling etc. (see and Appendix 1) are heat resilient toward effects on motor-cognitive performance, but they may still be affected by the development of fatigue. Athletes exposed to heat and sun for prolonged periods are at high risk for becoming hyperthermic and/or developing dehydration [Citation22,Citation24,Citation26,Citation27,Citation42], with associated effects on central fatigue in addition to the effects on cardiovascular factors relevant for performance in these sports [Citation7,Citation8,Citation31,Citation91,Citation92].
In strength disciplines and short-term power dominated sports (e.g., sprinting, jumping, throwing, weightlifting, and gymnastics), performance may benefit from faster cross-bridge cycle rates or other chemical processes positively affected by the Q10-effect, associated with an elevated muscle temperature (see ref [Citation7] for detailed discussion). Furthermore, these sports will most likely not suffer from negative effects on fine motor coordination or maximal activation (CNS fatigue) as they are less complex (mainly closed motor tasks) and of short duration where adequate motor activation in contrast to prolonged/sustained efforts seems to be preserved [Citation8]. Likewise, indoor sports (such as aquatics, track cycling etc.) where the thermal conditions may be controlled will be much less likely to experience heat stress and associated decrements in motor-cognitive performance. Participants in indoor sports (where the athletes have high metabolic rates for prolonged periods, such as badminton and the prolonged track cycling disciplines) may experience heat stress regardless of the outdoor environment, but the lack of solar radiation and typically better opportunity to avoid very adverse environmental conditions, put participants in these sports at lower risk.
Mitigation strategies
Based on the risk assessment, it seems very important that coaches and athletes have the knowledge about efficient mitigation strategies [Citation93–96] “on-hand”, especially during midday where solar radiation, air temperature, humidity and risk for dehydration are highest [Citation1]. For comprehensive overview of availability, feasibility and the effectiveness of mitigation methods, we refer to Morris and colleagues [Citation96], but here we highlight potential methods/solutions targeting the specific factors of relevance against deterioration in motor-cognitive performance. Specifically, we introduce mitigations methods that provide the most efficient protection against severe hyperthermia, direct solar radiation on the head and dehydration.
One mitigations method athletes and coaches should consider when preparing for the Olympics is heat acclimation/acclimatization. Heat acclimation/acclimatization has been repeatedly shown to mitigate and/or postpone the negative effects of heat stress on physical performance [Citation97–103], albeit heat acclimation may take longer due to the environmental conditions in Tokyo (e.g., high humidity) [Citation95] as well as being limited due to evaporative heat loss [Citation104] compared to dry heat [Citation105]. In addition, it is worth noticing that high humidity may increase amounts of dripping sweat (e.g., increases the likelihood of dehydration). Furthermore, hydration may need careful consideration, as humid heat seems to hampers exercise performance compared to dry heat with similar water intake [Citation9]. In relation to motor-cognitive performance, there is some discrepancy between findings. For instance, some studies have shown a protective effect of heat acclimation on aspects of cognition [Citation106,Citation107], whereas we (Piil and colleagues 2019) and others [Citation108,Citation109] have shown that if participants reach their maximal tolerable core temperature, fine motor coordination and cognitively-dominated performance may be compromised. However, as is evident from that paper, participants could exercise for ~30% longer, before reaching max levels of core temperature post-heat acclimation [Citation109], which indeed underlines the importance of being heat acclimated/acclimatized before coming to the Olympics. Furthermore, sufficient heat acclimation/acclimatization can be achieved within one to two weeks [Citation97].
Athletes and coaches also need to consider hydration and re-hydrations strategies. Thermoregulatory sweating is associated with loss of both water and electrolytes [Citation29]) and as dehydration may aggravate cardiovascular stress [Citation22,Citation24,Citation26,Citation27,Citation42] and impair motor-cognitive performance [Citation66,Citation68,Citation70–72,Citation110] it is extremely important to limit these homeostatic disturbances. This is in general of importance, but particularly for athletes competing within a weight-category (i.e. for athletes that may needs to cut excess weight – due to dehydration strategies) or if multiple matches/competitions are scheduled within one day.
Other potential mitigation strategies that can be utilized during the Olympics includes pre-cooling (e.g. cold-water submersion, water dosing, cold fluid ingestion, ice vests etc [Citation93,Citation111–114].) and per-cooling (e.g. ice slurry ingestion, increase/facilitate airflow [fan], ice vests and shading as examples [Citation93,Citation111,Citation115–118]). In particular, precooling can be highly beneficial for events lasting less than an hour, as the lowered starting core temperature may blunt or delay the rise in core temperature at the start of exercise and benefit the athletic performance [Citation119]. For events lasting longer than 1 h, the effect of precooling will likely wear off and therefore methods for per-cooling can be prioritized to mitigate heat strain [Citation119]. Clothing optimization (more breathable, less evaporative resistance, wearing a cap [may not be applicable/feasible in all scenarios, depending on in-game rules]) can help mitigate thermal strain. Furthermore, the possibility of shading also seems to be of importance based on our model (see ), as it shows that removing solar radiation from the equation substantially reduces the heat stress, especially during midday.
Conclusion
Based on the analyses of risk scenarios presented in this paper, it seems evident that several sport performances in the upcoming Summer Olympics games will be affected by heat-related impacts on motor-cognitive performances such as decision-making, maximal motor-activation or precision. Especially, sports characterized as “complex” and with the competitors exercising with an intensity and duration that makes them susceptible for developing hyperthermia and this will be highly relevant for outdoor events scheduled midday where superimposed solar radiation both elevates the overall heat stress and direct exposure of the head further elevates the motor-cognitive impact level. The present work should highlight and create awareness on the interaction between sport complexity, heat exposure and the subsequent impact on identified physiological factors of importance (heat homeostasis and hydration). The proposed framework is not intended for calculation of a specific (individual) score that may predict the precise impact and future studies or field observations from specific sport events and refinement of the ability to test impact in ecological setting, may indeed improve knowledge on the importance environmental heat stress and motor-cognitive performances.
However, based on the available knowledge appropriate mitigations strategies such as precooling, per cooling (for sports with frequent breaks) or at least avoiding overheating during the preparation phase seems relevant. Also, maintenance of hydration and protection of the head against direct sunlight seems to be of great importance for the sports characterized as complex and therefore high-heat vulnerable. In contrast, athletes competing in short explosive or less complex sports should not worry and may in fact benefit from elevated body (muscle) temperatures providing muscular benefits (e.g. on contraction velocities and anaerobic metabolic processes) without negative effects on CNS function.
Abbreviations
CNS | = | central nervous system |
MVC | = | maximal voluntary contraction |
UV | = | ultra violet |
PHS | = | predictive heat strain model |
Tglobe | = | black globe temperature |
Vair | = | air velocity |
MCIL | = | motor-cognitive impact level |
Trectal | = | rectal core temperature |
Qsolar | = | direct solar radiation |
BW | = | body weight |
Disclosure statement
No potential conflict of interest was reported by the author(s).
Additional information
Funding
References
- Gerrett N, Kingma BRM, Sluijter R, et al. Ambient conditions prior to tokyo 2020 olympic and paralympic games: considerations for acclimation or acclimatization strategies. Front Physiol. 2019;10.
- Kakamu T, Wada K, Dr S, et al. Preventing heat illness in the anticipated hot climate of the Tokyo 2020 summer olympic games. Environ Health Prev Med. 2017;22(1):68.
- Vanos JK, Kosaka E, Iida A, et al. Planning for spectator thermal comfort and health in the face of extreme heat: the Tokyo 2020 Olympic marathons. SciTotal Environ. 2019;657:904–917.
- Vanos JK, Thomas WM, Grundstein AJ, et al. A multi-scalar climatological analysis in preparation for extreme heat at the Tokyo 2020 olympic and paralympic games. Temperature. 2020;7(2):191–214. doi: https://doi.org/10.1080/23328940.2020.1737479.
- Pachauri RK, Allen MR, Barros VR, et al. Climate change 2014: synthesis report. Contribution of Working Groups I, II and III to the fifth assessment report of the Intergovernmental Panel on Climate Change. Ipcc; 2014. Geneva, Switzerland.
- Moss RH, Edmonds JA, Hibbard KA, et al. The next generation of scenarios for climate change research and assessment. Nature. 2010;463(7282):747–756.
- Nybo L, Rasmussen P, Sawka MN Performance in the heat—physiological factors of importance for hyperthermia-induced fatigue.
- Nybo L, Nielsen B. Hyperthermia and central fatigue during prolonged exercise in humans. J Appl Physiol. 2001;91(3):1055–1060.
- Che Muhamed AM, Yusof HA, Stannard SR, et al. The efficacy of ingesting water on thermoregulatory responses and running performance in a warm-humid condition. Front Physiol. 2019;10.
- Lei T-H, Schlader ZJ, Che Muhamed AM, et al. Differences in dry-bulb temperature do not influence moderate-duration exercise performance in warm environments when vapor pressure is equivalent. Eur J Appl Physiol. 2020;120(4):841–852.
- Nielsen B, Kassow K, Aschengreen FE. Heat balance during exercise in the sun. Eur J Appl Physiol. 1988;58:189–196.
- Otani H, Kaya M, Tamaki A, et al. Exposure to high solar radiation reduces self-regulated exercise intensity in the heat outdoors. Physiol Behav. 2019;199:191–199.
- Otani H, Kaya M, Tamaki A, et al. Effects of solar radiation on endurance exercise capacity in a hot environment. Eur J Appl Physiol. 2016;116(4):769–779.
- Cheung SS. Neuromuscular response to exercise heat stress. Thermoregulation Hum Perform. 2008;53:39–60.
- Racinais S, Ihsan M, Périard JD. Neural and Muscular Function in the Heat. In: Périard JD, Racinais S, editors. Heat stress in sport and exercise: thermophysiology of health and performance. Cham, Switzerland: Springer International Publishing. 2019; p. 67–88.
- González‐Alonso J, Crandall CG, Johnson JM. The cardiovascular challenge of exercising in the heat. J Physiol. 2008;586:45–53.
- Crandall CG, Wilson TE. Human cardiovascular responses to passive heat stress. Compr Physiol. 2015;5:17–43.
- Crandall CG, González-Alonso J. Cardiovascular function in the heat-stressed human. Acta Physiol (Oxf). 2010;199(4):407–423.
- Junge N, Jørgensen R, Flouris AD, et al. Prolonged self-paced exercise in the heat – environmental factors affecting performance. Temperature. 2016;3(4):539–548. doi: https://doi.org/10.1080/23328940.2016.1216257.
- Racinais S, Alonso J-M, Coutts AJ, et al. Consensus recommendations on training and competing in the heat. Sports Med. 2015;45(7):925–938.
- Racinais S, Cocking S, Périard JD. Sports and environmental temperature: from warming-up to heating-up. Temperature. 2017;4(3):227–257. doi: https://doi.org/10.1080/23328940.2017.1356427.
- Cheuvront SN, Kenefick RW, Montain SJ, et al. Mechanisms of aerobic performance impairment with heat stress and dehydration. J Appl Physiol. 2010;109(6):1989–1995.
- Sawka MN, Wenger CB, Young AJ, et al. Physiological responses to exercise in the heat 1993.
- Trangmar SJ, Heat G-AJ. Hydration and the human brain, heart and skeletal muscles. Sports Med. 2019;49(S1):69–85.
- Trangmar SJ, González-alonso J. New insights into the impact of dehydration on blood flow and metabolism during exercise. Exerc Sport Sci Rev. 2017;45(3):146–153.
- Maughan RJ. Impact of mild dehydration on wellness and on exercise performance. Eur J Clin Nutr. 2003;57(S2):S19–S23.
- Nielsen B, Kubica R, Bonnesen A, et al. Physical work capacity after dehydration and hyperthermia. Scandinavian j sports sci 3: 1981:2–10.
- Drust B, Rasmussen P, Mohr M, et al. Elevations in core and muscle temperature impairs repeated sprint performance. Acta Physiol Scand. 2005;183(2):181–190.
- Mohr M, Nybo L, Grantham J, et al. Physiological responses and physical performance during football in the heat. PLOS ONE. 2012;7(6):e39202.
- González-Alonso J, Teller C, Andersen SL, et al. Influence of body temperature on the development of fatigue during prolonged exercise in the heat. J Appl Physiol. 1999;86(3):1032–1039.
- Nybo L. CNS fatigue provoked by prolonged exercise in the heat. Front Biosci (Elite Ed). 2010;2(2):779–792.
- Matsuura R, Arimitsu T, Yunoki T, et al. Effects of heat exposure in the absence of hyperthermia on power output during repeated cycling sprints. Bio sport Ins Sport. 2015;32(1):15–20.
- Downs NJ, Axelsen T, Schouten P, et al. Biologically effective solar ultraviolet exposures and the potential skin cancer risk for individual gold medalists of the 2020 Tokyo Summer Olympic Games. Temperature. 2019;7(1):89–108. doi: https://doi.org/10.1080/23328940.2019.1581427.
- Cheuvront SN, Kenefick RW. (2014). Dehydration: Physiology, Assessment, and Performance Effects. In Terjung R, editor. Comprehensive Physiology; 2014.
- Montain SJ. Hydration Recommendations for Sport 2008. Curr Sports Med Rep. 2008;7(4):187.
- Sawka MN, Burke LM, Eichner ER, et al. Exercise and Fluid Replacement. Med Sci Sports Exercise. 2007;39:377–390.
- Sawka MN, Montain SJ. Fluid and electrolyte supplementation for exercise heat stress. Am J Clin Nutr. 2000;72(2):564S–572S.
- Craig EN, Cummings EG. Dehydration and muscular work. J Appl Physiol. 1966;21(2):670–674.
- González-Alonso J. Separate and combined influences of dehydration and hyperthermia on cardiovascular responses to exercise. Int J Sports Med. 1998;19(Suppl 2):S111–114.
- Gonzalez-Alonso J, Mora-Rodriguez R, Below PR, et al. Dehydration reduces cardiac output and increases systemic and cutaneous vascular resistance during exercise. J Appl Physiol. 1995;79(5):1487–1496.
- González -Alonso J, Mora-Rodríguez R, Below PR, et al. Dehydration markedly impairs cardiovascular function in hyperthermic endurance athletes during exercise. J Appl Physiol. 1997;82(4):1229–1236.
- Nybo L, Jensen T, Nielsen B, et al. Effects of marked hyperthermia with and without dehydration onV˙o onV˙o2 kinetics during intense exercise. J Appl Physiol. 2001;90(3):1057–1064.
- Trangmar SJ, Chiesa ST, Llodio I, et al. Dehydration accelerates reductions in cerebral blood flow during prolonged exercise in the heat without compromising brain metabolism. Am J Physiol Heart Circ Physiol. 2015;309(9):H1598–H1607.
- Trangmar SJ, Chiesa ST, Stock CG, et al. Dehydration affects cerebral blood flow but not its metabolic rate for oxygen during maximal exercise in trained humans. J Physiol. 2014;592(14):3143–3160.
- Walton CC, Keegan RJ, Martin M, et al. The potential role for cognitive training in sport: more research needed. Front Psychol. 2018;9.
- Clarke ND, Duncan MJ, Smith M, et al. Pre-cooling moderately enhances visual discrimination during exercise in the heat. J Sports Sci. 2017;35(4):355–360.
- Gaoua N, Racinais S, Grantham J, et al. Alterations in cognitive performance during passive hyperthermia are task dependent. Int J Hyperthermia. 2011;27(1):1–9.
- Hocking C, Silberstein RB, Lau WM, et al. Evaluation of cognitive performance in the heat by functional brain imaging and psychometric testing. Comp Biochem Physiol Part A. 2001;128(4):719–734.
- Lee JKW, Koh ACH, Koh SXT, et al. Neck cooling and cognitive performance following exercise-induced hyperthermia. Eur J Appl Physiol. 2014;114(2):375–384.
- Liu K, Sun G, Li B, et al. The impact of passive hyperthermia on human attention networks: an fMRI study. Behav Brain Res. 2013;243:220–230.
- McMorris T, Swain J, Smith M, et al. Heat stress, plasma concentrations of adrenaline, noradrenaline, 5-hydroxytryptamine and cortisol, mood state and cognitive performance. Int J Psychophysiol. 2006;61(2):204–215.
- Racinais S, Gaoua N, Grantham J. Hyperthermia impairs short-term memory and peripheral motor drive transmission. J Physiol. 2008;586(19):4751–4762.
- Schlader ZJ, Gagnon D, Adams A, et al. Cognitive and perceptual responses during passive heat stress in younger and older adults. Am J Physiol Regul Integr Comp Physiol. 2015;308(10):R847–R854.
- Schlader ZJ, Lucas RAI, Pearson J, et al. Hyperthermia does not alter the increase in cerebral perfusion during cognitive activation. Exp Physiol. 2013;98(11):1597–1607.
- Schmit C, Hausswirth C, Le Meur Y, et al. Cognitive functioning and heat strain: performance responses and protective strategies. Sports Med. 2017;47(7):1289–1302.
- Sun G, Yang X, Jiang Q, et al. Hyperthermia impairs the executive function using the Attention Network Test. Int J Hyperthermia. 2012;28(7):621–626.
- Bandelow S, Maughan R, Shirreffs S, et al. The effects of exercise, heat, cooling and rehydration strategies on cognitive function in football players. Scand J Med Sci Sports 20 Suppl. 2010;3:148–160.
- Gaoua N, Herrera CP, Périard JD, et al. Effect of passive hyperthermia on working memory resources during simple and complex cognitive tasks. Front Psychol. 2017;8:2290.
- Qian S, Li M, Li G, et al. Environmental heat stress enhances mental fatigue during sustained attention task performing: evidence from an ASL perfusion study. Behav Brain Res. 2015;280:6–15.
- Simmons SE, Saxby BK, McGlone FP, et al. The effect of passive heating and head cooling on perception, cardiovascular function and cognitive performance in the heat. Eur J Appl Physiol. 2008;104(2):271–280.
- Piil JF, Lundbye-Jensen J, Trangmar SJ, et al. Performance in complex motor tasks deteriorates in hyperthermic humans. Temperature. 2017;4(4):420–428. doi: https://doi.org/10.1080/23328940.2017.1368877.
- D’Anci KE, Constant F, Rosenberg IH. Hydration and cognitive function in children. Nutr Rev. 2006;64(10):457–464.
- Goodman SPJ, Moreland AT, Marino FE. Maintaining euhydration preserves cognitive performance, but is not superior to hypohydration. Journal of Cognitive Enhancement. 2019;3:338–348.
- Gopinathan MA, Pichan G, Sharma VM. Role of dehydration in heat stress-induced variations in mental performance. Arch Environ Health. 1988;43(1):15–17.
- Lindseth PD, Lindseth GN, Petros TV, et al. Effects of Hydration on Cognitive Function of Pilots. Mil Med. 2013;178(7):792–798.
- Piil JF, Lundbye-Jensen J, Christiansen L, et al. High prevalence of hypohydration in occupations with heat stress—Perspectives for performance in combined cognitive and motor tasks. PLoS One. 2018;13(10):e0205321.
- Sharma VM, Sridharan K, Pichan G, et al. Influence of heat-stress induced dehydration on mental functions. Ergonomics. 1986;29(6):791–799.
- Smith MF, Newell AJ, Baker MR. Effect of acute mild dehydration on cognitive-motor performance in golf. J Strength Cond Res. 2012;26(11):3075.
- Watson P, Whale A, Mears SA, et al. Mild hypohydration increases the frequency of driver errors during a prolonged, monotonous driving task. Physiol Behav. 2015;147:313–318.
- Wittbrodt MT, Sawka MN, Mizelle JC, et al. Exercise‐heat stress with and without water replacement alters brain structures and impairs visuomotor performance. Physiol Rep. 2018;6(16):e13805.
- Goodman SPJ, Moreland AT, Marino FE. The effect of active hypohydration on cognitive function: a systematic review and meta-analysis. Physiol Behav. 2019;204:297–308.
- Wittbrodt M, Millard-Stafford M. Dehydration Impairs Cognitive Performance: a Meta-analysis. Med Sci Sports Exercise. 2018;50(11):2360–2368.
- Piil JF, Christiansen L, Morris NB, et al. Direct exposure of the head to solar heat radiation impairs motor-cognitive performance. Sci Rep. 2020;10(1):7812.
- Griggs KE, Stephenson BT, Price MJ, et al. Heat-related issues and practical applications for Paralympic athletes at Tokyo 2020. Temperature. 2019;7(1):37–57. doi: https://doi.org/10.1080/23328940.2019.1617030.
- Malchaire J, Piette A, Kampmann B, et al. Development and validation of the predicted heat strain model. Ann Work Expo Health. 2000;45(2):123–135.
- Liljegren JC, Carhart RA, Lawday P, et al. Modeling the wet bulb globe temperature using standard meteorological measurements. J Occup Environ Hyg. 2008;5(10):645–655.
- Ramsey JD, Bernard TE. Heat Stress. In Harris R, editor. Patty’s Industrial Hygiene; 2001.
- Ioannou, Tsoutsoubi L, Mantzios K, et al. A free software to predict heat strain according to the ISO 7933: 2018. Ind Health. 2019;57(6):711–720.
- Morrison S, Sleivert GG, Cheung SS. Passive hyperthermia reduces voluntary activation and isometric force production. Eur J Appl Physiol. 2004;91(5–6):729–736.
- Simmons SE, Mündel T, Jones DA. The effects of passive heating and head-cooling on perception of exercise in the heat. Eur J Appl Physiol. 2008;104(2):281–288.
- Wolpert DM, Landy MS. Motor control is decision-making. Curr Opin Neurobiol. 2012;22(6):996–1003.
- Yarrow K, Brown P, Krakauer JW. Inside the brain of an elite athlete: the neural processes that support high achievement in sports. Nat Rev Neurosci. 2009;10(8):585–596.
- Araújo D, Davids K, Diniz A, et al. Ecological dynamics of continuous and categorical decision-making: the regatta start in sailing. Eur J Sport Sci. 2015;15(3):195–202.
- Koo TK, Li MY. A guideline of selecting and reporting intraclass correlation coefficients for reliability research. J Chiropr Med. 2016;15(2):155–163.
- FIFA.com. Women’s Olympic Football Tournament Tokyo 2020 - FIFA.com [Online]. www.fifa.com:[dateunknown].https://www.fifa.com/womensolympic/ [2021 Mar 18].
- Nybo L, Flouris AD, Racinais S, et al. Football facing a future with global warming: perspectives for players health and performance. Br J Sports Med. 2021;55(6):297–298.
- Lei T-H, Mündel T. Humid heat stress affects trained female athletes more than does their menstrual phase. Temperature. 2018;5(3):202–204. doi: https://doi.org/10.1080/23328940.2018.1436394.
- Lei T, Cotter JD, Schlader ZJ, et al. On exercise thermoregulation in females: interaction of endogenous and exogenous ovarian hormones. J Physiol. 2019;597(1):71–88.
- Gagnon D, Kenny GP. Sex differences in thermoeffector responses during exercise at fixed requirements for heat loss. J Appl Physiol (1985). 2012;113(5):746–757.
- Flouris AD, McGinn R, Poirier MP, et al. Screening criteria for increased susceptibility to heat stress during work or leisure in hot environments in healthy individuals aged 31–70 years. Temperature. 2017;5(1):86–99. doi: https://doi.org/10.1080/23328940.2017.1381800.
- Nybo L. Hyperthermia and fatigue. J Appl Physiol. 2008;104(3):871–878.
- Nybo L, Secher NH. Cerebral perturbations provoked by prolonged exercise. Prog Neurobiol. 2004;72:223–261.
- Bongers CCWG, Hopman MTE, Eijsvogels TMH. Cooling interventions for athletes: an overview of effectiveness, physiological mechanisms, and practical considerations. Temperature. 2017;4(1):60–78. doi: https://doi.org/10.1080/23328940.2016.1277003.
- Costa RJS, Gaskell SK, McCubbin AJ, et al. Exertional-heat stress-associated gastrointestinal perturbations during Olympic sports: management strategies for athletes preparing and competing in the 2020 Tokyo Olympic Games. Temperature. 2020;7(1):58–88. doi: https://doi.org/10.1080/23328940.2019.1597676.
- Lei T-H, Wang F. Looking ahead of 2021 tokyo summer olympic games: how does humid heat affect endurance performance? Insight into physiological mechanism and heat-related illness prevention strategies. J Therm Biol. 2021;99:102975.
- Morris NB, Jay O, Flouris AD, et al. Sustainable solutions to mitigate occupational heat strain – an umbrella review of physiological effects and global health perspectives. Environ Health. 2020;19(1):95.
- Daanen HAM, Racinais S, Périard JD. Heat acclimation decay and re-induction: a systematic review and meta-analysis. Sports Med. 2018;48(2):409–430.
- Lorenzo S, Halliwill JR, Sawka MN, et al. Heat acclimation improves exercise performance. J Appl Physiol (1985). 2010;109(4):1140–1147.
- Mikkelsen CJ, Junge N, Piil JF, et al. Prolonged Heat Acclimation and Aerobic Performance in Endurance Trained Athletes. Front Physiol. 2019;10.
- Nadel ER, Pandolf KB, Roberts MF, et al. Mechanisms of thermal acclimation to exercise and heat. J Appl Physiol. 1974;37(4):515–520.
- Oberholzer L, Siebenmann C, Mikkelsen CJ, et al. Hematological adaptations to prolonged heat acclimation in endurance- trained males. Front Physiol. 2019;10.
- Périard JD, Racinais S, Sawka MN. Adaptations and mechanisms of human heat acclimation: applications for competitive athletes and sports. Scand J Med Sci Sports. 2015;25(Suppl 1):20–38.
- Sawka MN, Latzka WA, Montain SJ, et al. Physiologic tolerance to uncompensable heat: intermittent exercise, field vs laboratory. Med Sci Sports Exerc. 2001;33(3):422–430.
- Nielsen B, Strange S, Christensen NJ, et al. Acute and adaptive responses in humans to exercise in a warm, humid environment. Pflugers Arch. 1997;434(1):49–56.
- Nielsen B, Hales JR, Strange S, et al. Human circulatory and thermoregulatory adaptations with heat acclimation and exercise in a hot, dry environment. J Physiol. 1993;460(1):467–485.
- Racinais S, Wilson MG, Gaoua N, et al. Heat acclimation has a protective effect on the central but not peripheral nervous system. J Appl Physiol. 2017;123(4):816–824.
- Radakovic SS, Maric J, Surbatovic M, et al. Effects of acclimation on cognitive performance in soldiers during exertional heat stress. Mil Med. 2007;172(2):133–136.
- Brazaitis M, Skurvydas A. Heat acclimation does not reduce the impact of hyperthermia on central fatigue. Eur J Appl Physiol. 2010;109(4):771–778.
- Piil JF, Mikkelsen CJ, Junge N, et al. Heat acclimation does not protect trained males from hyperthermia-induced impairments in complex task performance. Int J Environ Res Public Health. 2019;16(5):716.
- Taylor L, Watkins SL, Marshall H, et al. The impact of different environmental conditions on cognitive function: a focused review. Front Physiol. 2016;6.
- Morris NB, Jay O. To drink or to pour: how should athletes use water to cool themselves? Temperature. 2016;3(2):191–194.doi: https://doi.org/10.1080/23328940.2016.1185206.
- Siegel R, Maté J, Watson G, et al. Pre-cooling with ice slurry ingestion leads to similar run times to exhaustion in the heat as cold water immersion. J Sports Sci. 2012;30(2):155–165.
- Siegel R, Maté J, Brearley MB, et al. Ice slurry ingestion increases core temperature capacity and running time in the heat. Med Sci Sports Exerc. 2010;42(4):717–725.
- Misailidi M, Mantzios K, Papakonstantinou C, et al. Environmental and psychophysical heat stress in adolescent tennis athletes. Int J Sports Physiol Perform. 2021;1–6.
- Bongers CCWG, Thijssen DHJ, Veltmeijer MTW, et al. Precooling and percooling (cooling during exercise) both improve performance in the heat: a meta-analytical review. Br J Sports Med. 2015;49(6):377–384.
- Jay O, Hoelzl R, Weets J, et al. Fanning as an alternative to air conditioning – a sustainable solution for reducing indoor occupational heat stress. Energy Build. 2019;193:92–98.
- Morris NB, English T, Hospers L, et al. The effects of electric fan use under differing resting heat index conditions: a clinical trial.
- Morris NB, Chaseling GK, English T, et al. Electric fan use for cooling during hot weather: a biophysical modelling study. Lancet Planet Health. 2021;5(6):e368–e377.
- Bongers CCWG, Hopman MTE, Eijsvogels TMH. Cooling interventions for athletes: an overview of effectiveness, physiological mechanisms, and practical considerations. Temperature. 2017;4(1):60–78. doi: https://doi.org/10.1080/23328940.2016.1277003.
- Ainsworth BE, Haskell WL, Herrmann SD, et al. Compendium of physical activities: a second update of codes and MET Values. Med Sci Sports Exercise. 2011;438:1575–1581. 2011
- Morris KS, Osborne MA, Shephard ME, et al. Velocity, aerobic power and metabolic cost of whole body and arms only front crawl swimming at various stroke rates. Eur J Appl Physiol. 2016;116(5):1075–1085.
- Pe DP, Botter A, Osgnach C. The energy cost of sprint running and the role of metabolic power in setting top performances. Eur J Appl Physiol. 2015;115(3):451–469.
- Spencer MR, Gastin PB. Energy system contribution during 200- to 1500-m running in highly trained athletes. Med Sci Sports Exercise. 2001;33:157–162.
- Brisswalter J, Fougeron B, Legros P. Variability in energy cost and walking gait during race walking in competitive race walkers. Med Sci Sports Exercise. 1998;30:1451–1455.
- Faude O, Meyer T, Rosenberger F, et al. Physiological characteristics of badminton match play. Eur J Appl Physiol. 2007;100(4):479–485.
- McInnes SE, Carlson JS, Jones CJ, et al. The physiological load imposed on basketball players during competition. J Sports Sci. 1995;13(5):387–397.
- Michael JS, Rooney KB, Smith R. The Metabolic Demands of Kayaking: a Review. J Sports Sci Med. 2008;7:1–7.
- Novak AR, Dascombe BJ. Physiological and performance characteristics of road, mountain bike and BMX cyclists. Journal of Science and Cycling. 2014;3(3):9-16.
- Ebert TR, Martin DT, Stephens B, et al. Power output during a professional men’s road-cycling tour. Int J Sports Physiol Perform. 2006;1(4):324–335.
- Granier C, Abbiss CR, Aubry A, et al. Power output and pacing during international cross-country mountain bike cycling. Int J Sports Physiol Perform. 2018;13(9):1243–1249.
- Craig NP, Norton KI. Characteristics of track cycling. Sports Med. 2001;31(7):457–468.
- Osgnach C, Poser S, Bernardini R, et al. Energy cost and metabolic power in elite soccer: a new match analysis approach. Med Sci Sports Exercise. 2010;42(1):170–178.
- Y M, S K, T H. Heart rate and metabolic responses to participation in golf. J Sports Med Phys Fitness. 1989;29:269–272.
- Banister EW, Ribisl PM, Porter GH, et al. The caloric cost of playing handball. Res Quarterly Am Asso Health, Phyl Edu Recreation. 1964;35(3):236–240.
- Sell KM, Ledesma AB Heart rate and energy expenditure in division i field hockey players during competitive play. Wolters Kluwer: 2016.
- Arseneau E, Mekary S, Léger LA. V2 Requirements of Boxing Exercises. J Strength Cond Res. 2011;25(2):348–359.
- Crisafulli A, Vitelli S, Cappai I, et al. Physiological responses and energy cost during a simulation of a Muay Thai boxing match. Appl Physiol Nutr Metab. 2009;34(2):143–150.
- Horswill CA. Applied Physiology of Amateur Wrestling. Sports Med. 1992;14:114–143.
- Secher NH. The physiology of rowing. J Sports Sci. 1983;1(1):23–53.
- Dubois R, Paillard T, Lyons M, et al. Metabolic demands of elite rugby union assessed using traditional, metabolic power, and heart rate monitoring methods. J Sports Sci Med. 2017;16:84–92.
- Bojsen-Moller J, Larsson B, Magnusson SP, et al. Strength and endurance profiles of elite Olympic class sailors. In: Human performance in sailing conference proceedings: incorporating the 4th European Conference on Sailing Sports Science and Sports Medicine and the 3rd Australian Sailing Science Conference. Palmerston North, New Zealand: Massey University. 2003, p. 97–111.
- Murias JM, Lanatta D, Arcuri CR, et al. Metabolic and functional responses playing tennis on different surfaces. J Strength Cond Res. 2007;21(1):112–117.