ABSTRACT
The present comprehensive review (i) summarizes the current knowledge on the impacts of occupational heat stress on outdoor workers, (ii) provides a historical background on this issue, (iii) presents a meta-analysis of published data, (iv) explores inter-individual and intra-individual factors, (v) discusses the available heat mitigation strategies, (vi) estimates physical work capacity, labour productivity, and metabolic rate for the year 2030, and (vii) provides an overview of existing policy and legal frameworks on occupational heat exposure. Meta-analytic findings from 38 field studies that involved monitoring 2,409 outdoor workers across 41 jobs in 21 countries suggest that occupational heat stress increases the core (r = 0.44) and skin (r = 0.44) temperatures, as well as the heart rate (r = 0.38) and urine specific gravity (r = 0.13) of outdoor workers (all p < 0.05). Moreover, it diminishes the capacity of outdoor workers for manual labour (r = −0.82; p < 0.001) and is responsible for more than two thirds of the reduction in their metabolic rate. Importantly, our analysis shows that physical work capacity is projected to be highly affected by the ongoing anthropogenic global warming. Nevertheless, the metabolic rate and, therefore, labour productivity are projected to remain at levels higher than the workers’ physical work capacity, indicating that people will continue to work more intensely than they should to meet their financial obligations for food and shelter. In this respect, complementary measures targeting self-pacing, hydration, work-rest regimes, ventilated garments, and mechanization can be adopted to protect outdoor workers.
Introduction
The global challenge faced by rising temperatures places workers at an increasingly greater risk of heat-related morbidity and mortality. Literature suggests the existence of a thermally neutral ambient temperature zone between ~15°C and ~25°C (clothed individuals), where heat exchange () and body core temperature regulation is achieved primarily by control of dry heat loss [Citation1]. Any human activity outside this temperature range is exponentially affected, leading workers to experience elevated physiological heat strain [Citation2–5] accompanied by diminished cognitive [Citation2,Citation5–10] and physical performances [Citation2–4,Citation8,Citation11–13]. Of course, it is beyond any doubt that a thermally neutral zone of only 10°C is proportionately small compared to the wide range of ambient temperatures where human beings are expected to survive and perform their daily activities. In fact, people can be currently found fishing in the frozen lakes of Finland, as well as walking camels in the hot Sahara Desert. This broad range of environments where people perform their daily duties is, unfortunately, expected to get warmer due to the ongoing anthropogenic global warming, affecting the billions of people who work outdoors [Citation14]. In addition to this, most industrialized countries are concurrently faced with the challenge of an aging workforce [Citation15,Citation16], which is accompanied by chronic diseases and a deterioration of human physiological capacity for heat dissipation [Citation17–22]. Currently, despite the increasing awareness of the effects of occupational heat stress on health and well-being, tens of crop workers are dying every year due to occupational heat exposure in the United States [Citation23], while one fatal case of occupational heat stress is expected to occur every 14 to 24 minutes worldwide by 2030 [Citation24]. Furthermore, by the end of the decade more than 2 % of working hours across the globe are estimated to be lost every year, because it will be too hot to work and/or because people will work at a slower pace [Citation14]. Outdoor workers such as those who work in agriculture and construction will be the most affected, accounting for 60 % and 19 % of global working hours lost to heat stress, respectively [Citation14]. Similarly, the ongoing climate change is becoming a “threat multiplier” which increases the occurrence of fires [Citation25] and conflicts [Citation26] all around the globe, multiplying the exposure of first responders and soldiers to increased occupational heat stress. Countries have begun to refine their policies and guidelines to address the rising threat to worker health and safety caused by the anthropogenic global warming, and these efforts must be presented and discussed to provide guidance to countries wishing to adopt relevant policies in the future.
Figure 1. Heat transfer between human body and the surrounding thermal environment in occupational settings.
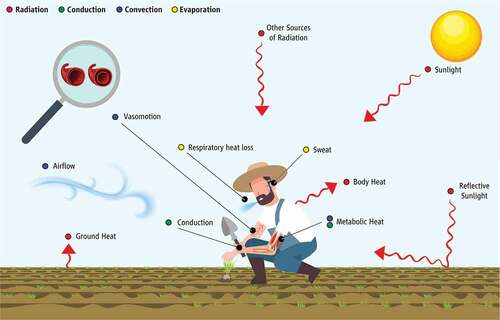
The present comprehensive review summarizes current knowledge and understanding on the impacts of occupational heat stress on outdoor workers. It provides a historical background on the work performed since the early scientific research and includes meta-analyses of recently published ecological data on the impact of occupational heat stress on indicators of physiological heat strain (heart rate, skin temperature, core temperature, hydration status, metabolic rate). It also discusses inter-individual (e.g. sex, age, chronic disease) and intra-individual (modifiable within an individual over relatively short periods) factors both within (e.g. hydration, fitness, acclimation) and beyond an individual’s control (e.g. work shift duration, number of consecutive days of work), and presents the available heat mitigation strategies for outdoor workers. Finally, the article concludes with a novel presentation of projected physical work capacity (see subsection “capacity or productivity?”), labour productivity, and metabolic rate for the year 2030 based on climate change models, and provides a brief overview of existing policy and legal frameworks on occupational heat exposure.
Historical background
Occupational heat stress is also linked with a broad spectrum of deleterious effects on human health and wellbeing. It is fair to say that workplace heat exposure is a problem as old as humans. After all, it has been speculated that we have been evolutionary adapted for hunting in the heat [Citation27], which was for several millennia our preeminent occupation. Although historical observations are not strictly gathered according to modern research criteria and guidelines, several archaic sources reported incidents of occupational heat strain in ancient combats. For instance, in the history of Peloponnesian war, Thucydides reported that Spartan and Athenian soldiers were suffering from thirst under the heat of the sun [Citation28]. Similar stories, by the same historian, reported that Syracusans forced Athenian captives to work in hot quarries without any roof to cover them from the sun, making them ill [Citation29]. In a different incident, Archimedes used mirrors and reflective sunlight as an anti-personnel weapon to defend Syracuse from the Roman fleet [Citation30]. Although, these stories of occupational heat stress/strain were reported more than two millennia ago, it was the first time in history where ambient conditions were described as being capable to affect humans in occupational settings. Several centuries later, an article published in The Lancet, in June 1865, reported that “Commanding Officers of volunteers are very apt to err in this particular; and the spirit of their men is such that they shrink from complaint, and persevere in efforts which may easily, under a burning sun, become dangerous to life.”[Citation31]. With the invention of high-speed presses and the facilitation of more frequent publication of newspaper articles, this historical knowledge became more apparent to the world. An article published by Stamford Mercury in August 1871 reported: “The intense heat has for some days made it very difficult for labourers to work in the harvest fields in Devon and Cornwall, and on Monday a man named Rogers expired very suddenly while at work near Kingsbridge.” Also, incidents of occupational heat stroke during military operations and work in firerooms were only some of the examples that filled out the pages of newspapers every so often.
The idea of describing the heat stress experienced by someone was also incubated in the early scientific research era. Aristotle, the father of thermoregulation [Citation32,Citation33], was the first to state that the brain regulates body temperature within an optimum range for comfort (in ancient Greek, “Ὁ μὲν οὖν ἐγκέφαλος εὔκρατον ποιεῖ τὴν ἐν τῇ καρδίᾳ θερμότητα καὶ ζέσιν”) [Citation34]. He also wrote on the physics of temperature and moisture, stimulating the development of the first instruments for quantifying heat stress, more than two millennia ago [Citation33]. Over the next two millennia, knowledge on human thermoregulation advanced sporadically through contributions of individual scientists and physicians such as Galileo Galilei [Citation33] and Santorio Santori [Citation35] who developed the first instruments to assess ambient and body temperature, while it was during the late 18th century when the scientific community worked collectively on the topic. In 1777, in an early attempt to explain metabolic heat production, Lavoisier formulated his respiration theory in which oxygen in lungs reacts with carbon from blood producing carbon dioxide and heat [Citation36–38]. A few years later, in 1789, following his hypothesis that heat is a substance which he called “caloric”, he came up with the term “calorimeter” [Citation38,Citation39]. In 1908, Haldane published first on the effects of occupational heat stress on the physiological heat strain experienced by workers [Citation40]. In 1912, Isenschmid and Krehl, reported first on the importance of hypothalamus on temperature regulation [Citation41]. Six years later, in 1918, an editorial article reported in the Journal of the American Medical Association suggested that workplace heat stress is an industrial health hazard [Citation42]. Soon thereafter, Houghten and Yaglou made an early attempt to characterize the “comfort zone” at the laboratories of the American Society of Heating and Ventilating Engineers [Citation43]. In Europe, Vernon and Warner [Citation44] and, later, Bedford [Citation45] conducted empirical studies among factory workers. Analytical work started few years prior to the second World War in the United States, where Winslow and his colleagues [Citation46] made significant contributions. During the second World War, research activity on the topic intensified and many disciplines including engineering, physiology, medicine, and meteorology became involved. After the War, Yaglou and Minard developed instructions for the United States Army, introducing for the first time the Wet-Bulb Globe Temperature (WBGT) [Citation47]. During the 1960s, the work of Wyndham and colleagues at the Chamber of Mines of South Africa developed the foundations of modern “occupational environmental physiology” [Citation48–50]. At the same time, in England, Lind conducted laboratory experiments aiming to identify a physiological criterion for setting environmental limits during work in the heat [Citation51], and soon thereafter his findings were adopted by the World Health Organization [Citation52].
Coming to the most recent times, in 1986, the first International Conference on Environmental Ergonomics was held in Whistler, Canada. This meeting became a hub for researchers wanting to present their work on the impacts of different environmental factors on working people. In 2015, the European Commission funded the “HEAT-SHIELD” project which aimed to expand knowledge on the impact of occupational heat stress on worker health and productivity [Citation53]. The “Operation Heat Shield Canada” project was recently funded by the Canadian Foundation for Innovation to develop population-specific mitigation strategies reducing heat-related morbidity and mortality. At the same time, the “HeatSafe” project in Southeast Asia seeks to protect people who work in tropical countries, such as Singapore, Vietnam, and Cambodia. Also, reputable organizations such as the International Labour Organization [Citation54] and the Department of Labor of the United States [Citation55] take initiatives to safeguard the health and wellbeing of people who work under significant heat stress. The recent attention of both scientists and funding bodies is a result of the increasing realization that outdoor workers are significantly affected by ambient conditions, experiencing increased physiological heat strain [Citation2–5,Citation11,Citation18,Citation53,Citation54,Citation56–58] and reduced capacity for manual labour [Citation2,Citation11,Citation13]. This is timely because the ongoing global warming indicates that advanced and focused research is needed now more than ever before.
Occupational heat strain in outdoor workers: A meta-analysis
As discussed throughout this article, heat exposure in outdoor workplaces is accompanied by an inescapable alteration of human physiological function and capacity for work. Numerous laboratory experiments and ecological studies have been conducted to investigate the associations between occupational heat stress and physiological heat strain. In an attempt to provide a holistic view on the impact of occupational heat stress on outdoor workers, this section synthesizes the available evidence on the topic and provides quantitative information, by means of meta-analysis, on the impact of workplace heat exposure on the physiology and labour capacity of those who work outdoors. The following subsections provide detailed information on all the steps and procedures involved in the present meta-analysis.
Inclusion criteria
In this meta-analysis we included studies that involved monitoring outdoor (people who typically work under the sun or under partially shaded conditions) workers who carry out their duties in warm-to-hot environments. To eliminate the effect of automation and mechanization that has drastically increased over the last two decades resulting in reduced metabolic rate and physiological heat strain during work in the heat [Citation2,Citation59–61], our meta-analysis included only studies conducted since the year 2000. This is because, the present meta-analysis aims at investigating the heat strain experienced by modern workers employing contemporary work practices, where their work effort and metabolic rates are significantly reduced due to automation and mechanization. Additionally, only studies presenting numerical (means and standard deviations/medians and ranges) or graphical (illustrations and figures) information (pre-, per-, and post-shift data, or comparisons between neutral/cold and warm/hot environments) were included in the present meta-analysis.
Exclusion criteria
We excluded laboratory experiments since they do not usually consider solar radiation which is known to increase the physiological heat strain experienced by outdoor workers [Citation5,Citation57,Citation62–67], and self-pacing which is known to reduce the work effort and therefore metabolic heat production and subsequently mitigate the physiological heat strain experienced by workers [Citation2,Citation3,Citation11,Citation68] (). Also, we excluded studies incorporating only indoor workers, such as those who work in the manufacturing, education, and health industries, since they were deemed to be out of scope of the present review which examines the impact of occupational heat stress on outdoor workers. We also excluded studies that involved monitoring outdoor workers but did not provide numerical or graphical information describing the effect of heat exposure, throughout the work-shift, on the monitored workers.
Methods
Our search was performed manually over seven databases (PubMed, Web of Science, Scopus, Embase, Medline, Google Scholar, and Agricultural and Environmental Science Collection) for studies that investigated the impacts (variables listed below) of occupational heat stress on people who work under the sun. In each study, we extracted the average work-shift WBGT as well as pre-, per-, and post-shift data for body core temperature as measured with ingestible capsules or rectal probes (:A), mean skin temperature (:B), heart rate (:C), dehydration as expressed by urine specific gravity (:D), and labor productivity as measured by time-motion analysis (:E). WetPlotDigitizer was used to extract data from studies presenting only graphical information [Citation69]. Detailed information on all the procedures involved when assessing physiological heat strain in ecological settings is presented in a subsequent section entitled “assessing physiological heat strain in occupational settings”.
Figure 3. Effect of occupational heat stress as expressed by the Wet-Bulb Globe Temperature (WBGT) on the physiological heat strain experienced by outdoor workers. Information was extracted from 38 studies investigating the impact of occupational heat stress on outdoor workers. Shaded areas represent the 95 % confidence interval around the fitted line. Each circle represents a study, while circle size portrays study weight. Data are presented as delta differences from baseline. Equations can be solved as: α + β × WBGT.
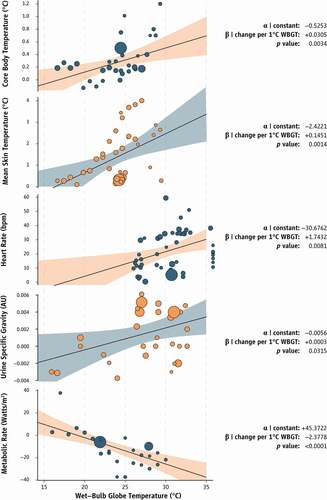
In studies where precise WBGT values were not available, we used the reported environmental data (usually air temperature and relative humidity) to calculate the indicator using the approach proposed by Liljegren [Citation70], which is the recommended methodology for calculating workplace WBGT from meteorological data [Citation71,Citation72]. When no solar radiation and/or wind speed data were reported, we assumed 800 W/m2 and 1 m/s, respectively, which are representable ambient conditions during work outdoors under partially cloudy sky [Citation2,Citation11]. In a study that took place over multiple months [Citation73], average daily environmental data were obtained from the nearest weather station (www.wunderground.com) and were subsequently used to compute mean WBGT values, following the methods described above. In this case, the retrieved air velocity values were adjusted for height and air friction following previous methodology [Citation74–76].
All environmental and physiological data were extracted manually from the original publications. In studies where only median and quartiles/ranges were reported, the extracted data were converted to means and standard deviations following previous methodology [Citation77,Citation78]. Thereafter, all WBGT values together with the relevant physiological data (means and standard deviations) were analyzed using the “metafor” package [Citation79] in R language (Rstudio, Version 1.3.1093, PBC, Boston, Massachusetts, United States).
Results and discussion
Our search resulted in 38 eligible ecological studies that involved monitoring experienced and acclimatized outdoor workers carrying out 41 jobs across 21 countries: Australia [Citation64,Citation66,Citation80–82], Brazil [Citation83], China [Citation84,Citation85], Cyprus [Citation11,Citation58,Citation86], El Salvador [Citation87], Greece [Citation2,Citation58], Cambodia [Citation88], Guatemala [Citation67], Hong Kong [Citation89], India [Citation90,Citation91], Indonesia [Citation92], Iran [Citation65,Citation93–96], Japan [Citation97], Malaysia [Citation98], Mexico [Citation99], South Africa [Citation100], South Korea [Citation101], Spain [Citation2,Citation58], Thailand [Citation102,Citation103], United Arab Emirates [Citation73], and United States of America [Citation104–107]. Of these, 60.7 % worked in agriculture, 16.1 % in construction, 15.4 % in mining, 2.8 % in utilities, 2.4 % in public services, 1.9 % in shipping, 0.5 % in oil and gas production, and 0.2 % in commerce industries (), according to the classification of industries and sectors of the International Labour Organization.
Table 1. | Jobs carried out by the workers in the eligible studies
Core temperature data were extracted from 10 studies that involved monitoring 385 outdoor workers [Citation2,Citation64,Citation66,Citation81–83,Citation86,Citation101,Citation104,Citation105]. Skin temperature data were extracted from 10 studies that involved monitoring 391 outdoor workers [Citation2,Citation11,Citation81,Citation82,Citation86,Citation91,Citation94,Citation95,Citation101,Citation105]. Heart rate data were extracted from 24 studies that involved monitoring 1023 outdoor workers [Citation63,Citation64,Citation66,Citation81–86,Citation88–98,Citation101–103,Citation105]. Urine specific gravity data were extracted from 17 studies that involved monitoring 1452 outdoor workers [Citation58,Citation63–67,Citation73,Citation80,Citation81,Citation87,Citation99,Citation100,Citation104–108]. Metabolic rate data were extracted from 2 studies that involved monitoring 57 outdoor workers [Citation2,Citation11].
Overall, our analyses involved 2,409 outdoor workers who performed moderate intensity labour (metabolic rate: 197.2 ± 49.3 W/m2; mean ± SD) for prolonged duration (420.6 ± 168.9 minutes). On average these workers were 35.3 years old (n = 1,987) having a body mass index of 25.0 kg/m2 (n = 1,763) and a body surface area of 1.81 m2 (n = 689). The ambient conditions which we were able to extract data from ranged between 15.9°C WBGT during apple harvesting [Citation106] and 35.8°C WBGT during work in a construction site [Citation85]. Our analyses revealed statistically significant associations between WBGT and differences from baseline in core temperature (r = 0.4444, p = 0.0034), skin temperature (r = 0.4373, p = 0.0014), heart rate (r = 0.3784, p = 0.0081), urine specific gravity (r = 0.1288, p = 0.0315), and metabolic rate (r = −0.8171, p < 0.0001) (). Yet, these associations explained only a fraction of the variance in core (R2 = 0.1975) and skin (R2 = 0.1912) temperatures, heart rate (R2 = 0.1432), and urine specific gravity (R2 = 0.0166) experienced by outdoor workers. The remaining amount of variance may be explained by inter- and intra-individual differences in outdoor workers (discussed in a subsequent section). On the other hand, WBGT explained more than two thirds of the variance in metabolic rate (R2 = 0.6677), in a way that the higher the occupational heat stress, the lower the metabolic rate of a worker.
Assessing physiological heat strain in occupational settings
Measuring occupational heat stress in the field was severely limited in previous years due to the technical difficulties to measure even the most fundamental physiological parameters. While technological advances over the past decade have improved the ability to perform field research, they do not allow for full spectrum evaluation of physiological variables known to be impaired during work in the heat [Citation2]. It is therefore fair to state that, despite the important steps forward seen in the last decade, our understanding of occupational heat stress and strain is still far from being complete, especially in context of heat vulnerable workers (e.g. older adults, individuals with common chronic disease) [Citation21,Citation109,Citation110]. Nevertheless, the ongoing technological progress is anticipated to facilitate new capabilities for heat-health measurements in occupational settings. Soon, wireless sweat rate [Citation111] and blood flow [Citation112] sensors along with wearable technology [Citation113] could uncover further aspects of the physiological heat strain experienced by outdoor workers. Of course, this presupposes having a good understanding of the impact of occupational heat stress on the physiological responses experienced by different groups of workers, including older individuals and those with chronic diseases.
A methodology for assessing the physiological heat strain experienced by outdoor workers was recently introduced by the HEAT-SHIELD project and adopted by the International Labour Organization [Citation54]. To assess physiological parameters of primary relevance, this methodology includes using wireless skin temperature sensors, telemetric core temperature capsules, wireless heart rate monitors, as well as urine specific gravity and urine colour assessments. To evaluate occupational heat stress, portable weather stations are used, while time-motion analysis is used to assess metabolic rate (see section “assessing physical work capacity and labour productivity”).
Assessing physical work capacity and labour productivity
Capacity or productivity?
The first issue is whether the question is related to worker capacity, or productivity; each has a unique definition, and they have important differences. Labor capacity has been defined as “the maximum physical work output that can be reasonably expected from an individual performing moderate to heavy work over an entire shift”[Citation13]. Thus, work capacity relates specifically to the potential to perform work under a unique set of conditions i.e. the climate [Citation13], or the physical condition of the worker [Citation114,Citation115]. In contrast, productivity has been defined as the “effectiveness of productive effort, especially in industry, as measured in terms of the rate of output per unit of input”. Productivity is determined not only by worker capacity, but also psychomotor factors and operational logistics, and is therefore difficult to model/predict reliably. Consequently, work that has assessed reductions in labor capacity in the heat [Citation13] might underestimate reductions in productivity, since a) heat stress also increases central fatigue [Citation116,Citation117] and can decrease cognitive performance [Citation7,Citation9,Citation118–120], and b) performing at the maximum capacity requires daily and sustained high levels of motivation. The benefits of assessing capacity are a) reduced variance from unknown sources, b) can be completed in a lab environment, and c) the findings are more generalizable across different industrial sectors. Productivity has generally been assessed in the field using methods such as time-motion analysis [Citation11] and rice bundle collection output in agriculture [Citation90], or rock carts filled in mining [Citation12]. These methods provide more valid estimates of real-world productivity losses induced by heat, but their generalizability to different industrial sectors is unknown. Ultimately, studies should be explicit whether they are assessing physical work/labor capacity, or productivity. The following section will focus primarily on heat induced reductions in labor capacity, unless stated otherwise.
There exists several different methods to assess/estimate labor capacity. Since these have been discussed previously [Citation13,Citation121], an in-depth discussion on each approach was not the primary focus of either of these papers. This section will address the methods available to assess labor capacity/ productivity, and their strengths and weaknesses.
Work/rest ratios
The International Organization for Standardization (ISO), the American Conference of Governmental Industrial Hygienists (ACGIH), and the National Institute for Occupational Safety and Health (NIOSH) have each published Threshold Limit Values (TLVs) relating to safe work durations at different levels of WBGT exposure. Safe work durations are expressed as the percent of work time that should be spent working or resting (typically in a 1-hour bout). The advice can be further modified based on expected work intensity (expressed in Watts or Watts per square meter of body surface area) and clothing [Citation122]. Higher work rates and clothing insulation values necessitate a reduction in safe allowable work durations for a given WBGT. The advice is intended to limit core temperature of an average group of workers from exceeding 38°C, such that those at the upper end of the distribution would be prevented from exceeding 41°C, a risk for heat stroke development. The advice from the ACGIH and ISO standard have been used to form predictions of labor capacity [Citation123,Citation124]. For example, Dunne and colleagues [Citation123] combine ACGIH TLVs for light, moderate and heavy labor into a single metric, such that % workability can be determined against the same WBGT axis along a continuum ranging from 25 to 32.2°C. A similar approach has been used by Bröde and colleagues [Citation124], who compared results using the TLVs from ISO:7243 or NIOSH.
Regardless of the specific approach, relying on TLVs to form predictions results in extremely steep reductions in labor capacity, which may be unrealistic. At a WBGT of 30°C, labor capacity is predicted to fall by 70 % based on Dunne’s model [Citation123], whereas empirical data shows only a 15 to 40 % reduction in labor capacity depending on worker’s aerobic fitness [Citation54,Citation115]. The advice using TLVs is, by intention, highly conservative since its aim is grounded in protecting most workers from exceeding a core temperature of 38°C (which in and of itself is conservative). Therefore, the models presented show that 0 % work is possible when WBGT >32°C, which is inconsistent with observations of continued labor in hot regions where that climatic heat value is typically exceeded [Citation54]. A further issue is that the models predict 100 % labor capacity at WBGT 26°C, even during heavy work. Physiological studies consistently show reduced physical work output in these environments, compared with a cooler reference condition [Citation13,Citation125,Citation126], such that the effect of heat is underestimated using a model based on TLVs. Finally, these exposure limits do not consider individual factors (e.g. age of the worker, health status, etc) that alter heat tolerance during work in the heat and therefore work output/capacity.
Fixed heart rate studies
Field observations demonstrate that self-pacing promotes a steady reduction in work output as the heat stress intensity increases, and such a reduction in work rate results in stable core temperature and heart rate responses [Citation68,Citation127]. A variety of other studies have also demonstrated that work output in hot conditions is intrinsically linked to heart rate, such that average working heart rate will not exceed a daily acceptable limit [Citation128–130]. Such outcomes are only likely in industries whereby self-pacing is possible, which is not the case in some occupations where the work may be a fixed pace, an emergency scenario, for those working on commission who may be highly motivated. Nonetheless, self-pacing approaches are observed across major industries such as in construction [Citation130], agriculture [Citation11], and manufacturing [Citation131], accounting for millions of workers worldwide. The precise upper acceptable working heart rate likely varies between individuals, yet little information from the field is available in this regard. Data on typical work heart rate variation with age, fitness, and sex, would be a useful addition to the literature. However, a good estimate of maximum acceptable average heart rate in the work periods across the day is ~ 130 b/min. This level sits at the borderline between moderate and high intensity occupational physical work domains (regardless of metabolic rate), as dictated by the World Health Organisation [Citation132].
During physical work in which individuals can self-pace, heart rate does not appreciably change throughout the heat stress spectrum (due to reductions in metabolic rate). Thus, using a protocol whereby heart rate is fixed should accurately capture the decrease in work output in response to heat stress. Importantly, however, it is not clear how various physiological (dehydration, cardiac fatigue, poor sleep quantity/quality) and psychological (motivation, anxiety) states impact upon what a worker deems as an acceptable working heart rate. Nonetheless, a major benefit of the fixed heart rate approach is that it is highly sensitive to heat, because as the heat stress intensity increases, a growing proportion of the cardiac output is used to delivered to cutaneous tissue to help promote surface heat loss from the body [Citation133,Citation134]. Consequently, at a fixed heart rate and with increasing heat stress, less cardiac output can be used to fuel muscular work, resulting in decreased total work output [Citation133,Citation134].
Previous studies have used fixed heart rate protocols to determine various human physiological responses to exercise [Citation135–137]. Furthermore, Jay and colleagues [Citation126] used a 1-hour fixed heart rate protocol in the heat to determine the impact of different cooling solutions, demonstrating that, at a fixed heart rate, total work output is reduced in warm compared with cooler conditions, and that electric fan use can rescue ~50 % of work output in the heat. Building on this study, the fixed heart rate approach has since been used to develop highly accurate prediction models of labor capacity in the heat [Citation13], with further refinements based on solar radiation [Citation138], wind speed [Citation139], aerobic fitness [Citation115], and work duration [Citation140]. Major strengths of this approach are that (i) it is highly sensitive to adjustments in the thermal climate and individual human factors, (ii) sources of unknown variability in typical self-paced studies (such as time trials or time to exhaustion tests) are minimized, and (iii) labor capacity can be assessed in a laboratory environment, allowing researchers to also determine the most effective cooling solutions in specific environments. A weakness of the approach is that it relies on the assumption that, in the field, heart rate is stable throughout a typical work period, which may be an oversimplification [Citation90]. Nonetheless, fixing the heart rate throughout an exercise protocol in the lab is preferable as it allows for generalisation across most industries involving physical work. Adopting a temporal heart rate response pattern based on a specific job type is likely preferable if the findings are applied to one task. It should also be carefully considered if absolute heart rate, or % heart rate maximum should be used to best reflect self-pacing behaviors across the age spectrum. For example, while a fixed heart rate of 130 beats/min has been used in young adults [Citation13,Citation115], such a prescription is likely to be inappropriate for older adults, who would be working at a greater % heart rate maximum.
American time use survey
Data from the American Time Use Survey (ATUS), coupled with meteorological data, has been used to determine the impact of hot weather on labor productivity [Citation141], and subsequent economic losses with climate change [Citation142,Citation143]. Importantly, the models were developed only for jobs in which air conditioning is not available, such as construction and agriculture. ATUS was analyzed from 2003–2006, which is useful because 2006 included a heat wave that produced very high temperatures across much of the United States, allowing estimates of the labor productivity across both ends of the temperature distribution [Citation141]. ATUS broadly describes how and where Americans spend their time, based on a 24-hour time diary which details any activities undertaken, duration of the activity, and where it took place. Data were analyzed from individuals who were considered at high risk of heat exposure during work (i.e. those working predominantly outdoors), and those at low risk (i.e. those who predominantly work indoors).
The models of ATUS merged with weather station data indicate up to 1-hour lost labor time when air temperature reaches 40°C, and almost no lost labor time at 30°C. Importantly, this only indicates that 1-hour less time is spent on labor overall, and offers no insight into how heat impacts productivity during the active working period. Therefore, the ATUS approach likely underestimates the impact of heat on labor productivity. Interestingly, the predictions were apparently insensitive to high humidity, despite its well-known negative impact on performance [Citation144]. One reason may be that maximum humidity was expressed as a percentage (i.e. relative humidity), and was likely to occur in the early morning due to the lower air temperature. Reporting absolute humidity at a time that corresponds with the maximum air temperature would, in our opinion, be a less biased approach. Moreover, ATUS cannot account for solar radiation effects, which can have a substantial impact on the heat stress severity. Nonetheless, the ATUS approach provides only mild effects of heat stress on labor productivity since it does not consider work output during labor, which is well known to be highly sensitive to heat and humidity [Citation13,Citation127,Citation145]. For example, ATUS only captures total time spent working, and cannot measure how heat impacts pacing (i.e. metabolic work rate), or the ratio of work/rest breaks. A strength of modeling labor productivity from the ATUS survey is the very large sample sizes used in the analysis (> 40,000 respondents). A weakness is its questionable sensitivity to heat and humidity which may underestimate current and future climate impacts.
Time-motion analysis
Workplace heat exposure can trigger a reallocation of the time devoted to specific work activities [Citation2]. Work activities characterized by increased metabolic demands tend to be performed at cooler times within the work-shift, and vice versa. Similarly, outdoor workers allocate more time to work breaks when the heat increases [Citation2,Citation11]. Based on this phenomenon, detailed video recordings can be used as a scientific means to investigate the effect of occupational heat stress on the metabolic rate and labour productivity of outdoor workers. This technique is known as “time-motion analysis” and is performed either through video recordings or in real-time [Citation2,Citation11,Citation105,Citation146–148]. In each case, the technique involves four interconnected stages: (i) concealing the presence of cameras and/or observers at the work site, (ii) conducting a sham measurement one day before the actual experiment, (iii) conducting the actual experiment without interfering with workers’ normal workflow, as well as (iv) informing everyone involved about the true purpose of the study, and analyze the data [Citation11].
The first two stages of time-motion analysis are dedicated to mitigating the so-called “Hawthorne effect”, also known as “observer effect”, which indicates that workers change aspects of their behaviour as a result of their awareness of being studied. A subsequent analysis of the data included in Hawthrone experiments [Citation149] suggested that the observer effect accounts for roughly 10 % of the variance in productivity, and thus it should always be considered. The third stage of time-motion analysis is the actual experiment. In this phase, all the procedures involved should be executed carefully, having in mind that workers should not be interrupted at any time of their work. The cameras and/or observers should be located in a proximity ensuring that all work activities are viewed clearly, yet without interfering with workers’ normal workflow. The fourth stage of time-motion analysis requires analysing the video recordings, on a second-by-second basis, in a way that every second matches an activity out of a list of >10 predefined work- (e.g. harvesting) and non-work-related activities (e.g. unplanned breaks) [Citation2]. These activities are then paired with known metabolic equivalents [Citation150,Citation151] to calculate the impact of heat stress on workers’ metabolic rate [Citation2], and they are used to calculate the amount of time spent on non-work-related activities as an indicator of labour loss [Citation2,Citation11,Citation54,Citation152]. In cases where the time-motion analysis is performed in real-time via observers, these features must be defined prior to commencing stage three.
Major strengths of the time-motion analysis approach are that (i) it involves monitoring actual experienced and acclimatized workers during their normal work routine in natural ambient conditions, (ii) it considers behavioural thermoregulation (e.g. self-pacing and varying clothing insulation), (iii) it considers the need for workers to meet their financial obligations for survival (e.g. the worker faces income loss when less is achieved within the same period of time), and (vi) it distinguishes productivity as expressed by the amount of goods produced [Citation90,Citation153–157] from labour productivity [Citation2,Citation11,Citation54,Citation152,Citation158] which is determined by the actual effort put into producing goods. In agriculture, for instance, expressing labour productivity as the amount of crop picked implies constant availability and equal distribution of crop across the tested piece of land, which is known to be untrue [Citation11]. It is a thought-provoking paradox to assume that a miner who spent an entire day searching for gold, in a barren mine, without success, was not productive because of the heat. The same applies to all outdoor occupations.
The main weaknesses of time-motion analysis are that (i) it requires significant resources to be performed (for example, ~12 person-hours are needed to analyse a single 8-hour work shift for one worker, not including study set-up, sham measurement, and video recording), (ii) it is sensitive to the Hawthorne effect, (iii) it can be difficult to make assessments of labour productivity when the work is mechanized (e.g. driving tractors) across different locations, and (iv) the outcome is not necessarily linked with the traditional notion of productivity (i.e. production of goods/services) which makes the findings less translational.
Inter- and intra-individual factors affecting work in the heat
Workplace heat stress does not affect all individuals to the same extent and/or manner (inter-individual differences), and its impacts may change at different times even for the same person (intra-individual differences). To date, 20 different factors have been reported to modulate the physiological heat strain experienced by workers () [Citation159]. Eight of these factors refer to inter-individual differences: age [Citation18,Citation160–163], anthropometrics [Citation18,Citation159,Citation164], cultural habits [Citation165], disabilities [Citation166,Citation167], drugs and other addictions [Citation159], ethnicity [Citation168,Citation169], medical conditions [Citation110,Citation170,Citation171], and sex [Citation18,Citation172]. The remaining 12 factors are connected to intra-individual differences: acclimatization [Citation173–176], clothing [Citation5,Citation177–179], consecutive shifts [Citation163,Citation180–182], environmental conditions [Citation2,Citation5,Citation11,Citation183], heat mitigation strategies [Citation2,Citation5,Citation184–186], metabolic demands [Citation187,Citation188], nutritional diet [Citation189], physical fitness [Citation18,Citation164,Citation176], sleep deprivation [Citation190–193], water consumption [Citation58,Citation176,Citation194], work duration [Citation159], and work experience [Citation159]. Detailed information on each of these factors is provided elsewhere [Citation16,Citation109,Citation159]. This general categorization across inter- and intra-individual factors is used for context, as most of these factors can vary both between different workers and for the same worker across different times/situations [Citation109,Citation159]. In this categorization scheme, factors that are within and beyond a worker’s control were considered intra-individual, while the remaining factors that cannot be changed or need longer time to change were considered inter-individual.
Figure 4. Inter- and intra-individual factors affecting the risk for experiencing increased physiological heat strain during work. Plus and minus signs indicate factors that positively or negatively affect the risk for experiencing increased physiological heat strain during work in the heat.18
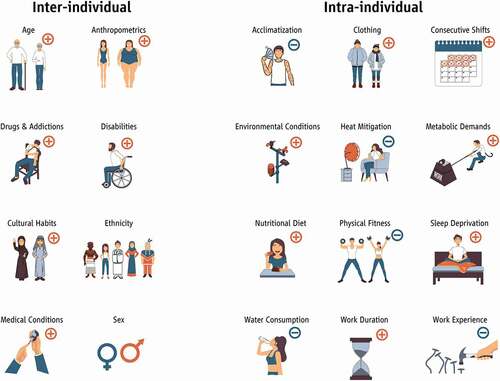
In light of the rapid technological advances, there is growing number of studies suggesting the transition from a more centralized heat stress management to the development of more individualized guidelines considering inter- and intra-individual factors [Citation18,Citation109,Citation113]. Recent technological advances can be adopted as means toward the development of more effective heat mitigation planning and strategies [Citation195]. Moreover, in an attempt to modernize the generic guidelines published by ACGIH which have been adopted by different governmental bodies all around the globe (see section “policy and current legal framework worldwide”), artificial intelligence along with machine learning techniques can be adopted to intelligently analyze large databases incorporating outdoor workers. These analyses should focus on identifying useful patterns and thresholds where work limits should be put to effectively safeguard those who work in the heat, taking into consideration intra- and inter-individual factors.
Heat mitigation strategies
To date, there have been a plethora of investigations into different methods for improving human health and performance in the heat [Citation184]. However, many of these methods have been conducted without necessarily considering cost, feasibility, and sustainability of these interventions within occupational contexts [Citation184]. Also typically negated from investigations into the quality of cooling interventions is how they will be perceived by the actual stakeholders (e.g. industry managers versus labour unions) who will be impacted by the implementation of these interventions [Citation56]. Importantly, stakeholder buy-in must include the workers, employers, safety officers and policy makers for maximum effectiveness [Citation56,Citation196]. In particular, lack of employee training and awareness regarding heat stress, and lack of employer support, are regularly found to be primary barriers to cooling intervention adoption [Citation56,Citation196]. Accordingly, the following cooling intervention recommendations are those we have assessed to meet acceptable standards for effectiveness, cost, feasibility and sustainability, and have been approved by stakeholders themselves [Citation56].
Although humans can physiologically acclimatize to performing physical tasks in the heat, these adaptations take at least four days to occur [Citation175] and are hindered by environmental, occupational, and lifestyle factors. Accordingly, a period of reduced work intensity and/or duration should be given to workers when first exposed to heat stress [Citation197].
Taking additional rest breaks in cooled areas is an effective mitigation method, however, employers might be apprehensive due to perceptions that additional breaks will cut into company productivity. Therefore, employers should be advised that if extra breaks are not provided, workers will work less efficiently (presenteeism) [Citation2,Citation11,Citation198] and may miss work due to illness (absenteeism) [Citation198]. Ultimately, the worker faces income loss when less is achieved within the same period of time, or a loss of leisure/family time if more work is required.
Breaks should be taken in relatively cool areas with access to drinking water. Small indoor air-conditioned or well-ventilated rooms can be used, whereas outdoors, temporary rest stations can be created using portable parasols and sun tents to limit solar heating [Citation199]. Other effective cooling solutions during breaks include submerging limbs in cold water [Citation200], or, in hot and dry environments with sufficient air flow, wetting the skin [Citation201], and/or wearing wetting clothing [Citation202] (especially in front of a fan [Citation203]). Using electric fans while working [Citation126] or resting [Citation185] is beneficial and effective in most geographical areas across the globe, even in extreme heat [Citation186]. If indoor resting/cooling areas are available, evaporative coolers have been reported to lower room temperature by as much as 20.6°C in hot (35°C) and dry environments [Citation204]. However, in small rooms and humid environments, the effectiveness of the coolers are limited, will likely interfere with natural evaporative heat loss from sweating and may create an unsafe work space due to the condensation of water in the room. For more intense, short-duration work (e.g. spraying chemicals in a greenhouse), cooling vests can be effective [Citation205].
As outdoor workers often arrive to work dehydrated and remain dehydrated throughout the day [Citation58,Citation105,Citation180], they should be encouraged to drink regularly before, during and after work. In industries where workers have limited access to water (e.g. agriculture), workers should be provided with portable methods of carrying water (e.g. backpacks containing water bladders) [Citation199], have water brought to them periodically, or have water caches established around the work area. Very cold water and ice slushies have limited effectiveness during work [Citation206] and are less palatable resulting in less consumption. Therefore, water temperature should ideally be maintained at 10°C [Citation207]. As a final consideration, many women do not take rest breaks because they do not wish to appear weak or their hourly income is lower [Citation208]. Moreover, women have been demonstrated to be at greater risk for dehydration, due to voluntary fluid restriction to avoid having to use unhygienic (or non-existent) at-work toilet facilities [Citation209]. Relative to males, young females have a reduced capacity to dissipate heat during a heat stress. Irrespective of age, whole-body heat loss (is ~5% lower in females relative to males [Citation210]. Further, the body’s capacity to dissipate heat declines with increasing age in both males (~3% per decade) and females (~4% per decade) [Citation210]. Consequently, relative to young adults, older adults experience higher increases in body temperature and a greater cardiovascular burden during a heat stress, which is potentially worse in females. In this light, it is important to consider that sex is a key modulator of the body’s physiological capacity to dissipate heat [Citation211], and therefore, sufficient support, both in the form of administrative policy and resource availability, are required to support female workers.
Protective clothing, which is highly insulative and reduces heat loss [Citation212,Citation213], must often be worn but can be ameliorated by incorporating ventilation patches into less exposed areas, such as the groin, underarms, inside the elbows and behind the knees. For outdoor workers, the need for heat loss and ultraviolet radiation protection must be balanced. As such, workers should be encouraged to wear hats and long, loose-fitting clothing made of light-weight breathable materials made of light colours or reflective fabrics to reduce solar radiation [Citation5].
If possible, the work shift can be started earlier to avoid hot afternoon hours or mid-day breaks or “siestas” can be taken to avoid peak temperatures [Citation214]. Alternatively, the most physically demanding activities can be moved to cooler times of the day. However, these strategies can interfere with private life or fixed work schedules and may be unfeasible in some jobs.
For cooling interventions to be most effective, employers/health officers need to prepare a concrete heat action plan, have all the required equipment for this plan in place, and have all their workers trained on how to use this plan before periods of hot weather occur. Subsequently, weather forecasts should be checked regularly or employers/health officers should subscribe to weather warning systems, to ensure they are not caught off guard by hot weather events [Citation215]. Additionally, “Buddy systems” and ensuring all workers are aware of the signs and symptoms of heat illness can help prevent illness and injury [Citation53].
In addition to not performing cost/feasibility/sustainability analyses [Citation184] and verifying the implementability of cooling interventions with stakeholders [Citation56], many cooling interventions have yet to be tested in the field. To this end a recent series of studies, led by the International Labour Organization and the HEAT-SHIELD project, was conducted to examine the capacity of 10 different heat mitigation strategies in reducing the physiological heat strain experienced by people who work across four countries with hot climates [Citation2]. The study found that self-pacing should be recommended as the basis of heat mitigation, while strategies targeting hydration, work-rest regimes, ventilated garments, and mechanization can lessen the physiological heat strain experienced by outdoor workers () [Citation2]. In addition to these findings, a different study led by the same organizations identified that light-coloured clothes are capable of reducing the skin temperature of those who work outdoors under the sun [Citation5].
Figure 5. Heat mitigation strategies tested in a recent study led by the International Labour Organization and the HEAT-SHIELD project (illustration modified with permission from a previous publication [Citation2]). Top left graph: black, blue, red, and grey colors represent “business as usual”, mechanical fruit cart, planned breaks, and ventilated garments scenarios, respectively. Top right graph: black, blue, red, and grey colors represent “business as usual”, hydration, evaporative garments, and planned breaks scenarios, respectively. Bottom left graph: black, blue, red, and grey colors represent “business as usual”, hydration, evaporative garments, and planned breaks scenarios, respectively. Bottom right graph: black, blue, red, and grey colors represent “business as usual”, hydration, ice slurry, and planned breaks scenarios, respectively. Precise values and effect sizes can be found in the original source [Citation2]. Tcore, Tsk, HR, and MET represent core temperature, mean skin temperature, heart rate, and metabolic rate, respectively. Asterisks indicate statistically significant differences between “business as usual” and the tested heat mitigation strategies.
![Figure 5. Heat mitigation strategies tested in a recent study led by the International Labour Organization and the HEAT-SHIELD project (illustration modified with permission from a previous publication [Citation2]). Top left graph: black, blue, red, and grey colors represent “business as usual”, mechanical fruit cart, planned breaks, and ventilated garments scenarios, respectively. Top right graph: black, blue, red, and grey colors represent “business as usual”, hydration, evaporative garments, and planned breaks scenarios, respectively. Bottom left graph: black, blue, red, and grey colors represent “business as usual”, hydration, evaporative garments, and planned breaks scenarios, respectively. Bottom right graph: black, blue, red, and grey colors represent “business as usual”, hydration, ice slurry, and planned breaks scenarios, respectively. Precise values and effect sizes can be found in the original source [Citation2]. Tcore, Tsk, HR, and MET represent core temperature, mean skin temperature, heart rate, and metabolic rate, respectively. Asterisks indicate statistically significant differences between “business as usual” and the tested heat mitigation strategies.](/cms/asset/aaebd415-6795-4c2b-92c2-ce41049c2e64/ktmp_a_2030634_f0005_oc.jpg)
Considerations for firefighters
The ongoing climate change is becoming a “threat multiplier” increasing the occurrence of fires all around the globe [Citation25] and this may increase the exposure of firefighters to increased occupational heat stress. Unlike industry, where heat-health complications occur in the interest of productivity and profit, firefighters are willing to risk their lives, often working long hours in unbearable environmental conditions, to help others in need [Citation216]. It is, therefore, not surprising that much of the literature on the impact of occupational heat stress on human health and well-being is focused on these people. As a consequence of the nature of their profession, firefighters are at a high risk for heat-related injuries and inflammation [Citation217]. A study in India [Citation218] showed that one out of five firefighters reports significant occurrence of heat exhaustion, while one in twenty reports events of heat syncope, heat pyrexia, and heat cramps. This is not surprising since even relatively brief sessions of physical work while wearing protective clothing in the heat can cause significant increase in physiological heat strain [Citation212,Citation219]. Things may be worse for older firefighters, who can be more susceptible to heat-related injuries while on duty, in comparison to their younger counterpars [Citation220].
The frequent heat exposure and/or high physical fitness of Canadian firefighters has been shown to generate protective effects during work in the heat [Citation221]. This is in line with data from the United States suggesting that the long-term heat exposure accrued by firefighters is sufficient to induce heat acclimatization [Citation221,Citation222]. In addition to the natural heat acclimatization which plays a catalytic role in reducing the physiological heat strain experienced by firefighters, complementary heat mitigation strategies should be always taken to ensure adequate protection while on duty. For instance, to maintain firefighters’ muscle functionality it is recommended to provide one hour recovery time after performing a heavy work bout in actual occupational settings [Citation223]. Similarly, active cooling methods can be also used in mitigating the physiological heat strain experienced by firefighters before re-entering a hot environment [Citation224]. The importance of these mitigation strategies becomes more apparent when considering that those performing self-paced firefighting in the heat, and provided with adequate recovery periods and unlimited access to water, may be as effective as during duty under temperate conditions [Citation225,Citation226].
Considerations for military personnel
Occupational heat stress is a major challenge for military personnel and there is a potential, with the ongoing global warming, to escalate into an even larger threat in the years to come [Citation227]. The problem of heat illnesses in military settings is not recent. It was described over two millennia ago, as discussed in a previous section (see “historical background”). In 1954 the first instructions on how to prevent heat illnesses in the army were issued, recommending (i) acclimatization for freshmen, (ii) special training for obese soldiers, (iii) trial of air-conditioned barracks, and (iv) adoption of a new thermal stress indicator at the time, the WBGT [Citation47]. Even after adopting these measures, in the period from 1980 through 2002, more than five thousand soldiers were hospitalized for heat-related illnesses in the United States, of whom 37 lost their lives [Citation228]. In early 2000s, there were 14.5 soldiers hospitalized due to heat stroke for every 100,000 troops, presenting symptoms such as dehydration, rhabdomyolysis, and acute renal failure [Citation228]. These numbers can be increased by ~16 times among those who previously experienced mild heat injuries, as well as ~2 times among overweight and ~3 times among obese individuals [Citation229]. On the other hand, the number of heat stroke cases can be reduced significantly when soldiers are adequately acclimatized to hot conditions. For instance, a study in Serbia showed that acclimated soldiers do not suffer any detrimental effects of exertional heat stress, in comparison to their unacclimated counterparts [Citation230]. Similarly, a study in the British Army found that, along with body composition and insufficient briefing about casualty evacuation, inadequate time for heat acclimatization was the most frequent risk factor for experiencing heat illnesses among soldiers who perform in the heat [Citation231]. It is important to note that, although heat acclimatization is of vital importance for soldiers who operate in the heat, literature suggests that it should not be reviewed in isolation, but instead it should be measured in the context of warfighting, considering exogenous factors such as wounding, protective equipment, dehydration, and febrile illnesses [Citation227].
In addition to the acclimatization strategies adopted by armies around the world, physical fitness is a crucial factor for human thermoregulatory capacity [Citation18]. For this reason, encouraging military personnel to engage in physical training, especially when ambient conditions are thermally comfortable or in air-conditioned facilities, is of vital importance [Citation232]. Moreover, complementary cooling techniques and devices can be adopted as heat mitigating strategies to increase heat dissipation during duty, yet, these techniques should be carefully selected since many of them are impractical for use in the field [Citation233]. Taking everything into account, when organizing a military operation several factors should be considered, including soldiers’ physical condition, the characteristics of the mission (duration, intensity, clothing, and water availability), and the climatic conditions in which the expedition is planned to take place [Citation234].
Climate change and future directions
The adoption of the Paris agreement on climate change, which aims to keep global warming well below 2°C above pre-industrial levels, constitutes a political success and it is widely recognized as an important win of climate diplomacy [Citation235]. Yet, even if stronger climate change measures are adopted, the evidence for a severe global warming is getting more and more apparent worldwide. The recent Intergovernmental Panel on Climate Change report [Citation236] indicated that the earth is warming at a much faster pace than previously thought. For workers, the threat of rising temperatures is omnipresent as industry worldwide will face more frequent and intense heat events, which are predicted to be more deadly over the next decade. While, temperatures all over the planet are projected to increase by up to 4°C within this century [Citation237], affecting the billions of people who work outdoors on a daily basis [Citation14]. In this light, evaluating the implications on the health and well-being of those who spend their day working outdoors in the heat, as well as examining the economic consequences of heat-induced labour loss are of paramount importance.
With the current ambient conditions in the United States, more than four hundred workers lost their lives to heat stress over the period between 1992 and 2006 [Citation23], which is translated into more than one fatal occupational injury every two weeks. However, when considering that people do not work consecutive days, as well as that heat-related occupational deaths occur mostly during months with excessive heat stress, the picture changes drastically, indicating that there is up to one fatal occupational injury every two days of work in the heat. Global warming is, unfortunately, projected to increase these mortality rates, but most importantly, it is also expected to diminish the quality of life of those who will survive. In hot countries people are already affected by working in environments which are hotter than what their thermoregulatory system can cope [Citation214,Citation238]. For instance, a study in Nigeria which involved mainly outdoor workers with no or low formal education, found that currently one out of five workers strongly agreed with the statement that heat-related injuries are common at work and that they are aware of heat-related deaths in their workplace [Citation239]. These heat-related morbidity and mortality risks are most prevalent in agriculture as well as other sectors where people work outdoors, such as construction and firefighting [Citation240]. Increased prevalence of acute occupational hazards such as fatigue, exhaustion, and psychological stress, as well as chronic illnesses of the cardiovascular and respiratory systems, cancers, and kidney diseases are often linked to global warming [Citation241–243]. Though climate change may increase the prevalence of these occupational hazards, the likelihood of new or previously unknown hazards should not be excluded, since there is a risk for interaction between known hazards and new conditions leading to the development of new hazards [Citation244]. In general, the frequency and intensity of extreme heat events is expected to increase occupational heat stress, primarily affecting the people who work in countries situated close to the equator [Citation245].
The increased risk of health complications due to climate change is accompanied by important socioeconomic impacts, such as reduced income driven by the impaired capacity of people to work in the heat, and other spillover effects at a community level [Citation246]. Warm and hot regions are expected to be the most impaired in the face of climate change, presenting an inverse relationship between ambient heat and labour productivity [Citation247]. For instance, in Nigeria [Citation239], Australia [Citation248], as well as countries situated in southern Europe [Citation72] the climate is projected to get warmer and workers are likely to experience increased occupational heat stress that will diminish their capacity for manual labour. It is important to note that this issue is not limited only to hot regions, as it also affects countries with relatively temperate climates such as Canada, which currently loses about 1 % of annual work hours due to increased occupational heat stress [Citation249]. This is because, in cold regions, workers performing physically demanding occupations are likely to face a deterioration in their ability to perform their duties [Citation247]. This is in line with recent findings from Alaska where social workers report that they are already aware of climate change, as well as over three quarters of them believe that climate change is currently dangerous for their clients or will be dangerous in a decade from now [Citation250].
To further explore the impact of climate change on the capacity of people to work at different parts of the world, we conducted a novel analysis to predict the magnitude of heat-induced loss in physical work capacity (), labour productivity (), and metabolic rate () of workers by year 2030. It is important to note that, since our analysis focuses on outdoor workers who commonly work in hot environments and would be acclimatized to the heat, we used models developed based only on acclimatized workers, and thus our findings may not reflect the impact of occupational heat stress on unacclimatized individuals. Physical work capacity is projected to be markedly reduced due to the ongoing anthropogenic global warming, especially in regions situated close to the equator. Nevertheless, the metabolic rate and, therefore, labour productivity are projected to remain at levels higher than the workers’ physical work capacity, indicating that people will continue to work more intensely than they should, in an attempt to meet their financial obligations for food and shelter. These estimations were conducted by using projected ambient temperatures from the Hadley Centre Global Environmental Model version 2 – Earth System (HadGEM2-ES) [Citation251,Citation252] general circulation model with the Representative Concentration Pathway 6.0 climate change scenario. Relative humidity and air velocity data were assumed to be constant at 40 % and 1 m/s, respectively, which are representative values for outdoor workplaces [Citation2]. Solar radiation was calculated for each grid cell (resolution: 0.5° × 0.5°), assuming work under clear sky at 14:00 [Citation253]. Thereafter, WBGT values were estimated based on the methods described by Liljegren [Citation70], and physical work capacity [Citation31] along with labour productivity [Citation152] were computed based on published models. The impact of occupational heat stress on workers’ metabolic rate was calculated based on the above-described regression derived from our meta-analysis.
Figure 6. Predicted physical work capacity in 2030 depending on the level of occupational heat stress. These predictions are based on estimated WBGT values for the year 2030. Air temperature was obtained from the HadGEM2-ES general circulation model with the Representative Concentration Pathway 6.0 climate change scenario. Solar radiation was calculated for each grid cell [Citation253], assuming work under clear sky [Citation291] at 14:00. WBGT values were estimated based on the methods described by Liljegren [Citation70]. The impact of occupational heat stress on outdoor workers was computed using a published physical work capacity model [Citation13].
![Figure 6. Predicted physical work capacity in 2030 depending on the level of occupational heat stress. These predictions are based on estimated WBGT values for the year 2030. Air temperature was obtained from the HadGEM2-ES general circulation model with the Representative Concentration Pathway 6.0 climate change scenario. Solar radiation was calculated for each grid cell [Citation253], assuming work under clear sky [Citation291] at 14:00. WBGT values were estimated based on the methods described by Liljegren [Citation70]. The impact of occupational heat stress on outdoor workers was computed using a published physical work capacity model [Citation13].](/cms/asset/7e65553e-706e-4ef5-a1fc-7e57204ce79e/ktmp_a_2030634_f0006_oc.jpg)
Figure 7. Predicted labour productivity in 2030 depending on the level of occupational heat stress. These predictions are based on estimated WBGT values for the year 2030. Air temperature was obtained from the HadGEM2-ES general circulation model with the Representative Concentration Pathway 6.0 climate change scenario. Solar radiation was calculated for each grid cell [Citation253], assuming work under clear sky [Citation291] at 14:00. WBGT values were estimated based on the methods described by Liljegren [Citation70]. The impact of occupational heat stress on outdoor workers was computed using a published labour productivity model [Citation152].
![Figure 7. Predicted labour productivity in 2030 depending on the level of occupational heat stress. These predictions are based on estimated WBGT values for the year 2030. Air temperature was obtained from the HadGEM2-ES general circulation model with the Representative Concentration Pathway 6.0 climate change scenario. Solar radiation was calculated for each grid cell [Citation253], assuming work under clear sky [Citation291] at 14:00. WBGT values were estimated based on the methods described by Liljegren [Citation70]. The impact of occupational heat stress on outdoor workers was computed using a published labour productivity model [Citation152].](/cms/asset/03deb5d3-4ddd-4ba2-9e1a-fadba22382c9/ktmp_a_2030634_f0007_oc.jpg)
Figure 8. Predicted reduction in workers’ metabolic rate during 2030 due to occupational heat stress. These predictions are based on estimated WBGT values for the year 2030. Air temperature was obtained from the HadGEM2-ES general circulation model with the Representative Concentration Pathway 6.0 climate change scenario. Solar radiation was calculated for each grid cell [Citation253], assuming work under clear sky [Citation291,Citation292] at 14:00. WBGT values were estimated based on the methods described by Liljegren [Citation70]. The impact of occupational heat stress on outdoor workers was computed using the regression derived from our meta-analysis [reduction in metabolic rate (W/m2) = 45.3722 − 2.3778 × WBGT in °C; ].
![Figure 8. Predicted reduction in workers’ metabolic rate during 2030 due to occupational heat stress. These predictions are based on estimated WBGT values for the year 2030. Air temperature was obtained from the HadGEM2-ES general circulation model with the Representative Concentration Pathway 6.0 climate change scenario. Solar radiation was calculated for each grid cell [Citation253], assuming work under clear sky [Citation291,Citation292] at 14:00. WBGT values were estimated based on the methods described by Liljegren [Citation70]. The impact of occupational heat stress on outdoor workers was computed using the regression derived from our meta-analysis [reduction in metabolic rate (W/m2) = 45.3722 − 2.3778 × WBGT in °C; Figure 3].](/cms/asset/011cb164-7d6d-4b3a-99ab-1b26a46a3c82/ktmp_a_2030634_f0008_oc.jpg)
Climate change currently affects or is expected to affect a broad range of the United Nations Sustainable Development Goals, including “SDG3: health and well-being”, as well as “SDG8: decent work and economic growth”. Lessons in the fight against global warming can be taken by Tobacco use, another important global threat, which has many similarities with climate change, since both threats cause enormous damage to the health and socioeconomic status of the population [Citation254]. The main difference between them arises from the fact that climate change has consequences on planet’s entire population [Citation254]. The most important lesson learnt from tobacco control is that any further delay in reaching agreement on climate change policy as well as poor implementation of the approved actions will cost countless lives [Citation254]. In this respect, cautious science-driven messages on the health consequences of global warming and the benefits of implementing climate change policies may act as a key component for convincing policy-makers and people worldwide of the urgency of climate actions [Citation255]. The collaboration between scientists and policy-makers on the recommendation of heat mitigation strategies and heat action plans is considered a necessary step towards developing legislative incentives [Citation53]. These heat mitigation measures should be planned in a way that safeguards human rights, supports social justice, as well as prevents the rise of new problems or intensification of existing ones [Citation256]. Also, the efficacy of managing occupational heat stress in the context of global warming depends heavily on early warning systems [Citation215,Citation257,Citation258], as well as valid and reliable ways to quantify heat exposure at work [Citation183,Citation184,Citation259].
Existing policy and legal frameworks on occupational heat exposure
Climate change is real, and industries worldwide are feeling its impacts. The science is clear and demonstrates that rising ambient temperatures have grown into a major threat for societies all over the globe [Citation260–262]. Extreme heat events, such as prolonged heatwaves, are currently increasing in frequency, intensity, and duration [Citation263], causing workers to experience high physiological heat strain and reduced capacity for manual and mental labour [Citation3], as well as resulting in higher occupational morbidity and mortality rates [Citation4,Citation264–266]. As discussed in a previous section, the problem has been known for centuries and the long-term financial and health benefits from adopting heat-health legislations have been under consultation for many years [Citation56,Citation255]. However, despite the history and evidence on the topic, at the time of writing this review, only a small number of countries enacted official legislation to protect their workforce from the heat.
Recently, some cities in the United States took positive steps toward developing legislations to protect pet from the heat [Citation267]. In Indianapolis, for instance, when ambient temperature is higher than 26.5°C pet shelters must be shaded by trees, and when it rises above 32°C pets must be brought into a temperature controlled facility [Citation267]. Although this is undoubtedly a step in the right direction, it is oxymoron that there are no similar standards for their owners who work in the heat under the United States Code of Federal Regulations, and uniform occupational heat-health policies do not exist [Citation268]. Only California [Citation269] and Washington [Citation270] have standards for outdoor heat exposure, while according to ACGIH [Citation271] there is a new law requiring the Maryland Commissioner of Labour and Industry to develop and embrace an occupational heat-health regulation in the near future. In California, the employer shall implement heat mitigation measures when ambient temperature is equal or higher than 35°C (WBGT = 31.9°C; assuming solar radiation = 800 W/m2, relative humidity = 40 %, and wind speed = 1 m/s), including a minimum of 10 minutes of cool-down rest period every two hours of work, which is interestingly more than five times less than what is suggested for light-intensity work by the ACGIH [Citation272]. On the other hand, the state of Washington adopted more drastic measures requiring the employers in the state to take steps toward protecting outdoor workers from heat illness, starting from May through September every year [Citation270,Citation273]. Yet, in neither of those states there are clear temperature thresholds at which outdoor work activities must terminate. It is important, however, to note that, the United States Department of Labor announced enhanced heat mitigation measures to protect people who work in the heat, including outdoor workers [Citation55]. In Canada, the Centre for Occupational Health and Safety [Citation274] guides Canadian jurisdictions to adopt the TLVs for heat stress as published by the ACGIH [Citation272,Citation275]. Yet, according to the same organization, legislation does not always specify a maximum temperature at which work must stop, especially when working outdoors [Citation274,Citation275].
On the other side of the Atlantic, European countries do not perform better in protecting their workforce from heat illnesses. Specifically, at the time of writing the present manuscript only the island nation of Cyprus, which is one of the warmest places for a European citizen to work, is known to have enacted heat-health legislation [Citation276], following the work-rest cycles recommended by the ACGIH [Citation272]. Work to develop such legislation has been ongoing in Greece, yet no official Ministerial Decision has been announced to date. Collaborations between Ministries and Universities have been forming across several South European countries, and it is hoped that effective heat-health legislations will emerge during the current decade. This southern European effort is in line with the most recent scientific evidence which indicate that even if stronger greenhouse gas emission actions are implemented, high heat risk is expected for large parts of Southern Europe within the next decades [Citation72]. On the other hand, some Northern European countries, such as the United Kingdom, adopted more generic regulations requiring the employer to arrange outdoor workstations, so far as is reasonably practicable, to provide protection from adverse ambient conditions [Citation277].
In Africa, the South African legislation requires the employers to ensure that their employees work in a safe environment, but there is no particular provision for outdoor workers in terms of reducing exposure to high ambient temperatures [Citation278]. On the other hand, countries of the Gulf region are often criticized for the adverse ambient conditions where their workforces carry out their duties, though it seems that these countries have taken the lead in the development of heat-health policies. The Ministry of Labour of the United Arab Emirates estimates that there are days when more than half of country’s workforce works outdoors in conditions that typically exceed 45°C coupled with 90 % relative humidity [Citation279,Citation280]. These conditions, are unfortunately projected to worsen, becoming intolerable to humans, if urgent measures are not adopted [Citation281]. In this light, the United Arab Emirates launched the “Safety in the Heat” programme targeting 800,000 people who work in the heat [Citation279,Citation280]. They also enacted a legislation prohibiting outdoor work between 12:30 and 15:00, during the hot months of summer [Citation282]. Until recently, similar legislation was in force in the state of Qatar, which was updated in 2020 after the collaborative effort between the University of Thessaly, the International Labour Organization, and the Qatari Ministry of Administrative Development, Labour and Social Affairs [Citation54]. The new Qatari legislation [Citation283] introduces a significant expansion of summertime working hours during which outdoor work is prohibited, it includes a work stoppage when WBGT rises beyond 32.1°C, prescribes requirements for annual health checks for workers, and requires mandatory risk assessments to be prepared by enterprises [Citation272].
In the Asia and the Pacific region, although some heat-health legislations are in force, they are likely insufficient to safeguard worker’s health and wellbeing. The Chinese Ministry of Health in 2010 announced a guideline to protect Chinese workforce from environmental hazards [Citation284]. The governmental regulation requires the employer to pay high-temperature subsidies to workers when ambient temperature climbs above 35°C [Citation285]. Additionally, when daily maximum temperature ranges between 35 and 37°C the amount of time spent doing continuous labour should be limited: at temperatures between 35 and 37°C outdoor working hours should be less than six, and when temperatures exceed 40°C (calculated WBGT: 36.0°C; assuming solar radiation = 800 W/m2, relative humidity = 40 %, and wind speed = 1 m/s) all outdoor work activities should be suspended [Citation286]. However, the implementation of the regulation is left to local provinces in practice and applies differently to different occupations [Citation285]. In contrast, in Malaysia, the Department of Occupational Safety and Health of the Ministry of Human Resources published a guideline on heat stress management at the workplace [Citation287], suggesting the adoption of the TLVs for heat stress as published by the ACGIH [Citation272]. On the other hand, in Australia, there are no regulations specifying standards for maximum temperatures in the workplace, however, employers have a duty under the Victorian Occupational Health and Safety Act to provide and maintain a safe, and without risks to health, work environment for their employees [Citation288].
Concluding remarks and future directions
Occupational heat stress is a problem as old as humankind and will continue to be for the years to come. Global warming currently impacts the billions of people who work outdoors, and is projected to worsen the working conditions of those who will choose to do so in the foreseeable future, starting from our children and grandchildren. In this respect, meta-analytic findings from countries all around the world suggest that occupational heat stress impacts significantly the physiology and labour capacity of those who work outdoors. In particular, heat exposure impacts physiological factors including body core and skin temperatures, as well as the heart rate and hydration state of outdoor workers. Yet, the majority of the variance in these factors may be explained through inter- and intra-individual differences among workers. On the other hand, occupational heat stress diminishes the capacity of outdoor workers for manual labour and is responsible for more than two thirds of the variance in their metabolic rate. In this light, many methods have been proposed to investigate the effect of heat on the capacity of workers for manual labour, including lab studies looking at physical work capacity, and time-motion analysis looking at labour productivity. Both of these methods have benefits and drawbacks, and there is a continuous need to explore new techniques for assessing this important issue that affects billions of people worldwide, particularly in the face of climate change.
Taken together, the evidence presented in this review suggests that physical work capacity is projected to be highly affected due to the ongoing global warming, especially in regions situated close to the equator. On the other hand, labour productivity is projected to remain at much higher levels, indicating that workers will probably continue to work at fast pace even though their physical work capacity will be diminished. These contradictions suggest that the physiological heat strain experienced by workers will probably increase within the next few decades unless urgent measures are adopted. However, an inconvenient truth, is that even if human greenhouse gas emissions stopped tomorrow, their lengthy lifetime in the atmosphere would ensure continued warming for decades [Citation289], while people will pursue work in a warming planet in an attempt to meet their financial obligations for food and shelter. In this respect, complementary measures targeting self-pacing, hydration, work-rest regimes, ventilated garments, and mechanization can be adopted to protect those who work outdoors [Citation2]. In addition to these measures, a collective global effort is deemed necessary to bring the voice of experts to the surface. That is to say, consensus recommendations by experts [Citation259,Citation290] and structured communication with policy-makers [Citation56] could be utilized as a mean to deliver strong messages towards adopting heat-health policies. This is because any further delay in reaching agreement on climate change policy or poor implementation of the approved actions will cost countless lives. It is probably the time to realize that for some people it may be too hot to work today, but tomorrow will be even hotter.
List of abbreviations
Disclosure statement
No potential conflict of interest was reported by the authors.
Additional information
Notes on contributors
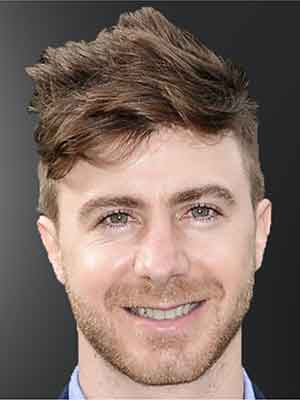
Leonidas G. Ioannou
Leonidas Ioannou earned a dual PhD degree in environmental exercise physiology from the University of Thessaly and the University of Copenhagen. He is currently a postdoctoral research fellow at FAME lab and his research focuses on the effects of environmental factors on human health and performance. He has participated in several projects conducting research across many countries worldwide, leading to the development of national and international legislations. He has also collaborated with European armed forces, as well as other reputable organizations, including the World Health Organization and the International Labour Organization, on projects aiming to enhance human health and wellbeing.
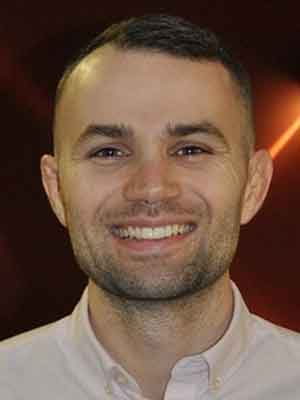
Josh Foster
Josh Foster completed his first Postdoctoral Fellowship at the Environmental Ergonomics Research Centre (Loughborough University, UK). He is completing a second Postdoctoral Fellowship at the Institute for Exercise and Environmental Medicine (University of Texas Southwestern Medical Center, USA). He has contributed to several large projects funded by the European Union, The USA’s National Institute of Health, and the USA’s Department of Defense. Overall, his work aims to better understand the impact of heat and global warming on human health, physical performance, and wellbeing. He was the 2021 recipient of the TROMP award for the highest impact paper in Biometeorology.
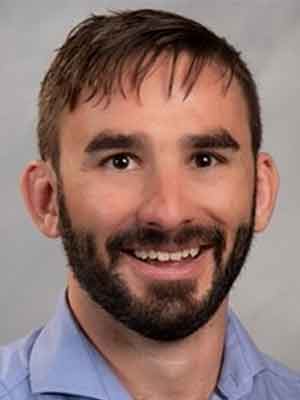
Nathan B. Morris
Nathan Morris is an Assistant Professor in the Hybl Sports Medicine and Performance Center at the University of Colorado at Colorado Springs, USA. The bulk of his research focuses on improving health and performance in the heat, within the contexts of public and occupational health, as well as athletics. Prior to his current position, he was a postdoctoral fellow at the University of Copenhagen, Denmark as part of the HEAT-SHIELD project. Earlier, he completed his PhD at the University of Sydney, Australia in the Thermal Ergonomics Laboratory.athan Morris is an Assistant Professor in the Hybl Sports Medicine and Performance Center at the University of Colorado at Colorado Springs, USA. The bulk of his research focuses on improving health and performance in the heat, within the contexts of public and occupational health, as well as athletics. Prior to his current position, he was a postdoctoral fellow at the University of Copenhagen, Denmark as part of the HEAT-SHIELD project. Earlier, he completed his PhD at the University of Sydney, Australia in the Thermal Ergonomics Laboratory.
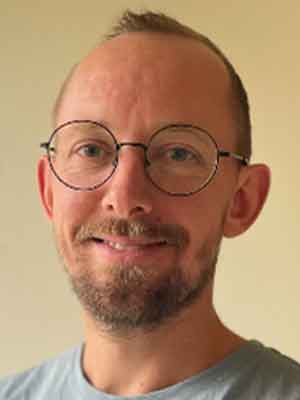
Jacob F. Piil
Jacob F. Piil is currently a Postdoctoral research fellow at the University of Copenhagen, Denmark. The focus of his research is on improving health and performance in the heat, within the contexts of public and occupational health, as well as athletics. He obtained his PhD in environmental heat stress and motor-cognitive performance at the University of Copenhagen, Denmark in 2020 as part of the HEAT-SHIELD project.
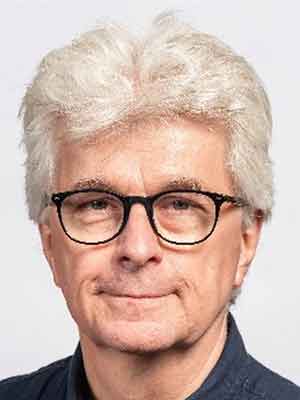
George Havenith
Professor George Havenith (FACSM, FCIEHF) is presently working at Loughborough University in the UK, where he has a Chair in Environmental Physiology and Ergonomics and is director of the Environmental Ergonomics Research Centre (www.lboro.ac.uk/eerc). His research covers three main topic areas: -Human Thermal Physiology/Environmental Ergonomics, -Heat and mass (vapour) transfer through clothing, and -Athlete exercise performance in relation to clothing. His special contribution to these fields is the multidisciplinary integration of physiology, physics, textile science and ergonomics. His work spans experimental studies, to the development of theoretical frameworks, and subsequently to the final application of the knowledge in the field.
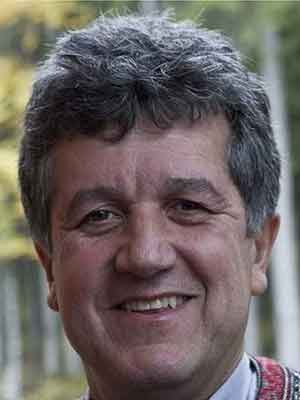
Igor B. Mekjavic
Igor B. Mekjavic is Professor of Environmental Physiology, and scientific counsellor at the Jozef Stefan Institute (Slovenia). He graduated from the Salford University (UK) where he also pursued his MSc studies. He completed his doctoral studies on human thermoregulation at the University of British Columbia (Canada), and his post-doctoral studies at the Karolinska Institutet (Sweden). He has served in various academic positions: University of British Columbia (Canada), University of Portsmouth (UK), and University of Ljubljana (Slovenia). He is currently also Adjunct Professor at Simon Fraser University (Canada). He conducts research on the effects of different environmental stressors on human health and performance.
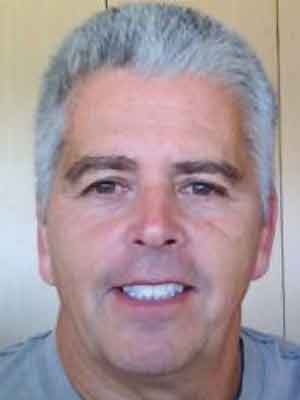
Glen P. Kenny
Dr. Kenny is a Professor at the University of Ottawa and holds a University and Industry Research Chair. He has led numerous studies evaluating the human heat stress response including projects directed at creating heat protection solutions tailored to the worker (e.g., exposure limits defined by the person’s age, sex, fitness, health). He is working with industry to understand the high-risk work demands and environments that pose a threat to worker health and safety. This includes advancing workplace heat prevention programs encompassing modifications to workplace design and interventions to reduce the negative impacts to health and safety caused by heat.
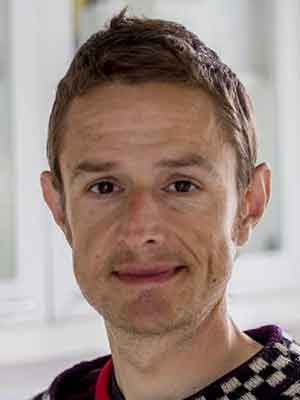
Lars Nybo
Professor Lars Nybo has an educational background in Human Physiology and has conducted research for more than 20 years in Thermal Physiology with particular focus on occupation heat stress and health as well as mechanistic research in cerebral factors of importance for motor-cognitive performance and fatigue during prolonged exercise in the heat. As invited speaker to large international conferences and author of more than 140 peer-reviewed papers he has a broad experience in conducting highly advanced laboratory experiments and field studies. He has led a number of national and international projects and contributed to WHO reports on heat-health and guidance documents.
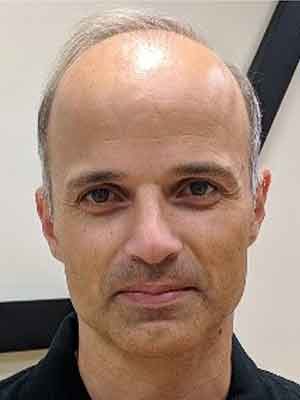
Andreas D. Flouris
Andreas Flouris is an Associate Professor at the University of Thessaly, in Greece, and an Adjunct Professor at the University of Ottawa, Canada. He is contributing to a series of large international research projects in Europe and North America, and he has published widely on the effects of different environmental factors on human health, productivity, and performance. He is currently participating in several Working Groups tasked to develop prevention measures to reduce the impacts of environmental factors for workers, athletes, and the general population, including the World Health Organization, the International Labour Organization, and the Greek Ministry of Labour.
REFERENCES
- Kingma BR, Frijns AJ, Schellen L, van Marken Lichtenbelt WD. Beyond the classic thermoneutral zone: Including thermal comfort. Temperature. 2014;1(2):142–9. doi: https://doi.org/10.4161/temp.29702
- Ioannou LG, Mantzios K, Tsoutsoubi L, et al. Occupational heat stress: multi-country observations and interventions. Int. J. Environ. Res. 2021;18(12):6303. doi: https://doi.org/10.3390/ijerph18126303
- Ioannou LG, Mantzios K, Tsoutsoubi L, et al. Effect of a Simulated Heat Wave on Physiological Strain and Labour Productivity. Int. J. Environ. Res. 2021;18(6):3011. doi: https://doi.org/10.3390/ijerph18063011
- Flouris AD, Dinas PC, Ioannou LG, et al. Workers’ health and productivity under occupational heat strain: a systematic review and meta-analysis. Lancet Planet. Health. 2018;2(12):e521–e531. doi: https://doi.org/10.1016/s2542-5196(18)30237-7
- Ioannou LG, Tsoutsoubi L, Mantzios K, et al. The impacts of sun exposure on worker physiology and cognition: multi-country evidence and interventions. Int. J. Environ. Res. 2021;18(14):7698. doi:https://doi.org/10.3390/ijerph18147698
- Pilcher JJ, Nadler E, Busch C. Effects of hot and cold temperature exposure on performance: a meta-analytic review. Ergonomics. 2002;45(10):682–98. doi:https://doi.org/10.1080/00140130210158419
- Taylor L, Watkins SL, Marshall H, Dascombe BJ, Foster J. The Impact of Different Environmental Conditions on Cognitive Function: A Focused Review. Front Physiol. 2015;6:372. doi:https://doi.org/10.3389/fphys.2015.00372
- Parsons K. Human thermal environments: the effects of hot, moderate, and cold environments on human health, comfort, and performance. CRC press, Boca Raton, Florida, USA; 2014.
- Piil JF, Lundbye-Jensen J, Trangmar SJ, Nybo L. Performance in complex motor tasks deteriorates in hyperthermic humans. Temperature. 2017;4(4):420–428. doi: https://doi.org/10.1080/23328940.2017.1368877
- Kingma BRM, Roijendijk LMM, Van Maanen L, Van Rijn H, Van Beurden MHPH. Time perception and timed decision task performance during passive heat stress. Temperature. 2021;8(1):53–63. doi: https://doi.org/10.1080/23328940.2020.1776925
- Ioannou LG, Tsoutsoubi L, Samoutis G, et al. Time-motion analysis as a novel approach for evaluating the impact of environmental heat exposure on labor loss in agriculture workers. Temperature. 2017;4(3):330–340. doi:https://doi.org/10.1080/23328940.2017.1338210
- Wyndham CH. Adaptation to heat and cold. Environ. Res. 1969;2(5):442–469. doi:https://doi.org/10.1016/0013-9351(69)90015-2
- Foster J, Smallcombe JW, Hodder S, et al. An advanced empirical model for quantifying the impact of heat and climate change on human physical work capacity. Int. J. Biometeorol. 2021;65(7):1215–1229. doi:https://doi.org/10.1007/s00484-021-02105-0
- Kjellstrom T, Maître N, Saget C, Otto M, Karimova T. Working on a warmer planet: The impact of heat stress on labour productivity and decent work. 2019. Publications Production Unit, International Labour Organization. Geneva, Switzerland. ISBN: 978-92-2-132968-8. https://www.ilo.org/global/publications/books/WCMS_712011/lang--en/index.htm
- Kenny GP, Notley SR, Flouris AD, Grundstein A. Climate Change and Heat Exposure: Impact on Health in Occupational and General Populations. In: Adams WM, Jardine JF, eds. Exertional Heat Illness: A Clinical and Evidence-Based Guide. Springer International Publishing, Cham, Switzerland; 2020:225–261.
- Kenny GP, Groeller H, McGinn R, Flouris AD. Age, human performance, and physical employment standards. Appl Physiol Nutr Metab. 2016;41(6 Suppl 2):S92–S107. doi:https://doi.org/10.1139/apnm-2015-0483
- Kenny GP, Sigal RJ, McGinn R. Body temperature regulation in diabetes. Temperature. 2016;3(1):119–145. doi:https://doi.org/10.1080/23328940.2015.1131506
- Flouris AD, McGinn R, Poirier MP, et al. Screening criteria for increased susceptibility to heat stress during work or leisure in hot environments in healthy individuals aged 31–70 years. Temperature. 2018;5(1):86–99. doi:https://doi.org/10.1080/23328940.2017.1381800
- Kenney WL, Munce TA. Invited Review: Aging and human temperature regulation. J. Appl. Physiol. 2003;95(6):2598–2603. doi:https://doi.org/10.1152/japplphysiol.00202.2003
- Meade RD, Notley SR, Kenny GP. Aging and human heat dissipation during exercise-heat stress: an update and future directions. Curr. Opin. Physiol. 2019;10:219–225. doi:https://doi.org/10.1016/j.cophys.2019.07.003
- Notley SR, Akerman AP, Friesen BJ, et al. Heat tolerance and occupational heat exposure limits in older men with and without type 2 diabetes or hypertension. Med Sci Sports Exerc. 2021;53(10):2196–2206. doi:https://doi.org/10.1249/mss.0000000000002698
- Notley SR, Poirier MP, Sigal RJ, et al. Exercise heat stress in patients with and without type 2 diabetes. Jama. 2019;322(14):1409–1411. doi:https://doi.org/10.1001/jama.2019.10943
- Centers for Disease Control and Prevention. Heat-related deaths among crop workers--United States, 1992-2006. MMWR. 2008;57(24):649–653.
- Kjellstrom T, Lemke B, Otto M, Hyatt O, Dear K. Occupational heat stress: contribution to WHO project on “Global assessment of the health impacts of climate change”, which started in 2009. Mapua: Health and Environment International Trust. 2014;4.
- Flannigan M, Stocks B, Turetsky M, Wotton M. Impacts of climate change on fire activity and fire management in the circumboreal forest. Glob. Change Biol. 2009;15(3):549–560. doi:https://doi.org/10.1111/j.1365-2486.2008.01660.x
- Selby J, Dahi OS, Fröhlich C, Hulme M. Climate change and the Syrian civil war revisited. Polit Geogr. 2017;60:232–244. doi:https://doi.org/10.1016/j.polgeo.2017.05.007
- Halsey LG, Bryce CM. Are humans evolved specialists for running in the heat? Man vs. horse races provide empirical insights. Exp. Physiol. 2021;106(1):258–268. doi:https://doi.org/10.1113/EP088502
- Thucydides. History of the Peloponnesian war. Greece, Book 4 , chapter 35. 425–424 BC.
- Thucydides. History of the Peloponnesian war. Greece, Book 6, chapter 87. 414–413 BC.
- Mills AA, Clift R. Reflections of the’Burning mirrors of Archimedes’. With a consideration of the geometry and intensity of sunlight reflected from plane mirrors. Eur. J. Phys. 1992;13(6):268.
- Porter AM. Heat Illness and Soldiers. Mil. Med. 1993;158(9):606–609. doi:https://doi.org/10.1093/milmed/158.9.606
- Flouris AD. A unifying theory for the functional architecture of endothermic thermoregulation. Temperature. 2014;1(3):162–3. doi:https://doi.org/10.4161/23328940.2014.980138
- Barnett MK. The development of thermometry and the temperature concept. Osiris. 1956;12:269–341.
- Aristotle. On the parts of animals (in Greek: Περί ζώων μορίων, Greece). 322–384 BC.
- Bigotti F. The Weight of the Air: Santorio’s Thermometers and the Early History of Medical Quantification Reconsidered. J Early Mod Stud (Bucur). 2018;7(1):73–103. doi:https://doi.org/10.5840/jems2018714
- Hoff HE, Guillemin R, Sakiz E. Claude Bernard on Animal Heat--an Unpublished Manuscript and Some Original Notes. Perspect Biol Med. 1965;8:347–68. doi:https://doi.org/10.1353/pbm.1965.0033
- Morris RJ. Lavoisier and the Caloric Theory. Br J Hist Sci. 1972;6(1):1–38. doi:https://doi.org/10.1017/S000708740001195X
- Frankenfield DC. On heat, respiration, and calorimetry. Nutr. 2010;26(10):939–950. doi:https://doi.org/10.1016/j.nut.2010.01.002
- Roberts L. A word and the world: the significance of naming the calorimeter. Isis. 1991;82(2):198–222. doi:https://doi.org/10.1086/355725
- Haldane JS. Work under pressure and in great heat. Science Progress in the Twentieth Century (1906-1916). 1908;2(7):378–398.
- Alpers BJ. Hyperthermia due to lesions in the hypothalamus. AMA Arch. Neurol. 1936;35(1):30–42. doi:https://doi.org/10.1001/archneurpsyc.1936.02260010040003
- Editorial. Heat as an industrial health hazard. JAMA. 1918;70(3):166–167. doi:https://doi.org/10.1001/jama.1918.02600030030014
- Houghton FC, Yaglou CP. Determining lines of equal comfort. ASHVE Transactions. 1923;29:163–176.
- Vernon HM, Warner CG. The Influence of the Humidity of the Air on Capacity for Work at High Temperatures. J. Hyg. 1932;32(3):431–462. doi:https://doi.org/10.1017/S0022172400018167
- Bedford T. The warmth factor in comfort at work: a physiological study of heating and ventilation. Industrial Health Research Board Report Medical Research Council. 1936 ;No.76
- Winslow C-EA, Herrington LP, Gagge AP. Physiological reactions of the human body to various atmospheric humidities. Am. J. Physiol-Legacy Content. 1937;120(2):288–299. doi:https://doi.org/10.1152/ajplegacy.1937.120.2.288
- Yaglou C, Minaed D. Control of heat casualties at military training centers. Arch Indust Health. 1957;16(4):302–16.
- Wyndham CH, Strydom NB, Morrison JF, et al. Criteria for physiological limits for work in heat. J. Appl. Physiol. 1965;20(1):37–45. doi:https://doi.org/10.1152/jappl.1965.20.1.37
- Williams CG, Bredell GAG, Wyndham CH, et al. Circulatory and metabolic reactions to work in heat. J. Appl. Physiol. 1962;17(4):625–638. doi:https://doi.org/10.1152/jappl.1962.17.4.625
- Wyndham CH, Strydom NB, Morrison JF, Toit FDd, Kraan JG. Responses of Unacclimatized Men Under Stress of Heat and Work. J. Appl. Physiol. 1954;6(11):681–686. doi:https://doi.org/10.1152/jappl.1954.6.11.681
- Lind AR. A physiological criterion for setting thermal environmental limits for everyday work. J. Appl. Physiol. 1963;18(1):51–56. doi:https://doi.org/10.1152/jappl.1963.18.1.51
- World Health Organization. Technical Report Series No. 412: Health factors involved in working under conditions of heat stress. 1969: 32.
- Morris NB, Piil JF, Morabito M, et al. The HEAT-SHIELD project — Perspectives from an inter-sectoral approach to occupational heat stress. J Sci Med Sport. 2021. doi:https://doi.org/10.1016/j.jsams.2021.03.001
- Flouris AD, Ioannou LG, Dinas PC, et al. Assessment of occupational heat strain and mitigation strategies in Qatar. 2019. International Labour Organization, Doha, Qatar.
- United States Department of Labor - Occupational Safety and Health Administration. OSHA National News Release: US Department of Labor announces enhanced, expanded measures to protect workers from hazards of extreme heat, indoors and out. United States Department of Labor. Accessed September 27, 2020. www.osha.gov/news/newsreleases/national/09202021
- Morris NB, Levi M, Morabito M, et al. Health vs. wealth: Employer, employee and policy-maker perspectives on occupational heat stress across multiple European industries. Temperature. 2020;8(3):284–301. doi:https://doi.org/10.1080/23328940.2020.1852049
- Piil JF, Christiansen L, Morris NB, et al. Direct exposure of the head to solar heat radiation impairs motor-cognitive performance. Sci. Rep. 2020;10(1):7812. doi:https://doi.org/10.1038/s41598-020-64768-w
- Piil JF, Lundbye-Jensen J, Christiansen L, et al. High prevalence of hypohydration in occupations with heat stress—Perspectives for performance in combined cognitive and motor tasks. PLOS ONE. 2018;13(10):e0205321. doi:https://doi.org/10.1371/journal.pone.0205321
- Isaak M, Yahya A, Razif M, Mat N. Mechanization status based on machinery utilization and workers’ workload in sweet corn cultivation in Malaysia. Comput Electron Agric. 2020;169:105208. doi:https://doi.org/10.1016/j.compag.2019.105208
- Smith LA, Dennis Wilson G, Sirois DL. Heart-rate response to forest harvesting work in the south-eastern United States during summer. Ergonomics. 1985;28(4):655–664. doi:https://doi.org/10.1080/00140138508963179
- Hachiya M, Amano T, Yamagata M, Kojima M. Development and Utilization of a New Mechanized Cabbage Harvesting System for Large Fields. Japan Agricultural Research Quarterly: JARQ. 2004;38(2):97–103. doi:https://doi.org/10.6090/jarq.38.97
- Foster J, Smallcombe JW, Hodder S, et al. The impact of heat on human physical work capacity; part III: the impact of solar radiation varies with air temperature, humidity, and clothing coverage. bioRxiv. 2021:2021.07.01.449782. doi:https://doi.org/10.1101/2021.07.01.449782
- Boonruksa P, Maturachon T, Kongtip P, Woskie S. Heat Stress, Physiological Response, and Heat-Related Symptoms among Thai Sugarcane Workers. Int. J. Environ. Res. 2020;17(17):6363.
- Brearley M, Harrington P, Lee D, Taylor R. Working in hot conditions--a study of electrical utility workers in the northern territory of Australia. J Occup Environ Hyg. 2015;12(3):156–62. doi:https://doi.org/10.1080/15459624.2014.957831
- Farshad A, Montazer S, Monazzam MR, Eyvazlou M, Mirkazemi R. Heat Stress Level among Construction Workers. Iran J Public Health. 2014;43(4):492–498.
- Hunt AP, Parker AW, Stewart IB. Heat strain and hydration status of surface mine blast crew workers. J Occup Environ Med. 2014;56(4):409–14. doi:https://doi.org/10.1097/jom.0000000000000114
- Sorensen CJ, Butler-Dawson J, Dally M, et al. Risk Factors and Mechanisms Underlying Cross-Shift Decline in Kidney Function in Guatemalan Sugarcane Workers. J. Occup. Environ. Med. 2019;61(3):239–250. doi:https://doi.org/10.1097/JOM.0000000000001529
- Kalkowsky B, Kampmann B. Physiological strain of miners at hot working places in German coal mines. Ind Health. 2006;44(3):465–73. doi:https://doi.org/10.2486/indhealth.44.465
- Rohatgi A. WebPlotDigitizer: version 4.5. Updated August, 2021. Accessed September 01, 2021. www.automeris.io/WebPlotDigitizer
- Liljegren JC, Carhart RA, Lawday P, Tschopp S, Sharp R. Modeling the Wet Bulb Globe Temperature Using Standard Meteorological Measurements. J Occup Environ Hyg. 2008;5(10):645–655. doi:https://doi.org/10.1080/15459620802310770
- Lemke B, Kjellstrom T. Calculating Workplace WBGT from Meteorological Data: A Tool for Climate Change Assessment. Ind. Health. 2012;50(4):267–278. doi:https://doi.org/10.2486/indhealth.MS1352
- Casanueva A, Kotlarski S, Fischer AM, et al. Escalating environmental summer heat exposure—a future threat for the European workforce. Reg. Environ. Change. 2020;20(2):40. doi:https://doi.org/10.1007/s10113-020-01625-6
- Bates GP, Miller VS, Joubert DM. Hydration Status of Expatriate Manual Workers During Summer in the Middle East. Ann Occup Hyg. 2009;54(2):137–143. doi:https://doi.org/10.1093/annhyg/mep076
- Masters GM. Renewable and efficient electric power systems. John Wiley & Sons, Hoboken, New Jersey, USA; 2013.
- Misailidi M, Mantzios K, Papakonstantinou C, Ioannou LG, Flouris AD. Environmental and Psychophysical Heat Stress in Adolescent Tennis Athletes. Int J Sports Physiol Perform. 2021:1–6. doi:https://doi.org/10.1123/ijspp.2020-0820
- Mantzios K, Ioannou LG, Panagiotaki Z, et al. Effects of weather parameters on endurance running performance: Disipline-specific analysis of 1258 races. Med Sci Sports Exerc. 2021 ;in press. doi:https://doi.org/10.1249/MSS.0000000000002769
- Luo D, Wan X, Liu J, Tong T. Optimally estimating the sample mean from the sample size, median, mid-range, and/or mid-quartile range. Stat Methods Med Res. 2018;27(6):1785–1805. doi:https://doi.org/10.1177/0962280216669183
- Wan X, Wang W, Liu J, Tong T. Estimating the sample mean and standard deviation from the sample size, median, range and/or interquartile range. BMC Med. Res. Methodol. 2014;14(1):135. doi:https://doi.org/10.1186/1471-2288-14-135
- Viechtbauer W. Conducting meta-analyses in R with the metafor package. J. Stat. Softw. 2010;36(3):1–48.
- Miller V, Bates G. Hydration of outdoor workers in north-west Australia. J. Occup. Health Saf. Aust. N. Z. 2007;23(1):79.
- Maté JE. Industrial heat stress: using ice slurry ingestion as a practical approach to reducing heat strain in workers. Edith Cowan University, Joondalup, Australia; 2010. https://ro.ecu.edu.au/theses/141
- Sinclair WH, Brownsberger JC. Wearing long pants while working outdoors in the tropics does not yield higher body temperatures. Aust N Z J Public Health. 2013;37(1):70–5. doi:https://doi.org/10.1111/1753-6405.12013
- Ruas AC, Maia PA, Roscani RC, Bitencourt DP, Amorim FT. Heat stress monitoring based on heart rate measurements. Rev Bras Med Trab. 2020;18(2):232–240. doi:https://doi.org/10.47626/1679-4435-2020-449
- Wong del PL, Chung JW, Chan AP, Wong FK, Yi W. Comparing the physiological and perceptual responses of construction workers (bar benders and bar fixers) in a hot environment. Appl Ergon. 2014;45(6):1705–11. doi:https://doi.org/10.1016/j.apergo.2014.06.002
- Li L, Liu H, Chen L, He J. Investigation on heat stress of construction workers in summer in Chongqing, China. E3S Web Conf. 2019;80:03005.
- Ioannou LG, Tsoutsoubi L, Mantzios K, Flouris AD. A free software to predict heat strain according to the ISO 7933:2018. Ind Health. 2019;57(6):711–720. doi:https://doi.org/10.2486/indhealth.2018-0216
- García-Trabanino R, Jarquín E, Wesseling C, et al. Heat stress, dehydration, and kidney function in sugarcane cutters in El Salvador – A cross-shift study of workers at risk of Mesoamerican nephropathy. Environ. Res. 2015;142:746–755. doi:https://doi.org/10.1016/j.envres.2015.07.007
- Hashim Z, Shafii S, Phan K, Rao V, Hashim HJ. The impact of heat on the health and productivity of cassava farmers in kratie, Cambodia. Malaysian J. Med. Health Sci. 2019;15(3):139-142.
- Yi W, Chan APC. Effects of Heat Stress on Construction Labor Productivity in Hong Kong: A Case Study of Rebar Workers. Int. J. Environ. Res. 2017;14(9):1055.
- Sahu S, Sett M, Kjellstrom T. Heat Exposure, Cardiovascular Stress and Work Productivity in Rice Harvesters in India: Implications for a Climate Change Future. Ind. Health. 2013. doi:https://doi.org/10.2486/indhealth.2013-0006
- Ashtekar S, Mishra S, Kapadia V, Nag P, Singh G. Workplace Heat Exposure Management in Indian Construction Workers Using Cooling Garment. Workplace Health Saf. 2019;67(1):18–26. doi:https://doi.org/10.1177/2165079918785388
- Santiana IMA, Lokantara WD, Yusuf M, Sutapa IN. Workload Analysis of Rice Field Workers in Bali. International Conference on Science and Technology (ICST 2018) Bali, Indonesia, Atlantis Press; 2018:504–507.
- Assilian-Mahabadi H, Jafari Mohammad J, Gholami A, Teimori Gholam H, Khodakarim S. Heat stress level and physiological parameters among an open-pit mine workers in Razavi Khorasan, Iran. Ann Med Health Sci Res. 2017;7:54–49.
- Golbabaei F, Monazzam MR, Yazdani Aval M, Allahyari T, Taban E, Rostami Aghdam Shendi M. Investigation of heat stress and heat strain in outdoor workers: a case study in Iran. AAB. 2016;7(4):30–38. doi:https://doi.org/10.22037/jps.v7i4.14494
- Nassiri P, Monazzam MR, Golbabaei F, et al. Application of Universal Thermal Climate Index (UTCI) for assessment of occupational heat stress in open-pit mines. Ind. Health. 2017;advpubdoi:https://doi.org/10.2486/indhealth.2017-0018
- Arman Z, Nikooy M, Tsioras PA, Heidari M, Majnounian B. Physiological workload evaluation by means of heart rate monitoring during motor-manual clearcutting operations. J. For. Eng. 2021;32(2):91–102. doi:https://doi.org/10.1080/14942119.2021.1868238
- Morioka I, Miyai N, Miyashita K. Hot environment and health problems of outdoor workers at a construction site. Ind Health. 2006;44(3):474–80. doi:https://doi.org/10.2486/indhealth.44.474
- Nurlzzate S, Bahri MTS, Karmegam K, Guan NY. Study on physiological effects on palm oil mill workers exposed to extreme heat conditions. J Sci Ind Res. 2015;74:406–410.
- Wagoner RS, López-Gálvez NI, de Zapien JG, Griffin SC, Canales RA, Beamer PI. An Occupational Heat Stress and Hydration Assessment of Agricultural Workers in North Mexico. Int. J. Environ. Res. 2020;17(6):2102.
- Biggs C, Paterson M, Maunder E. Hydration status of South African forestry workers harvesting trees in autumn and winter. Ann Occup Hyg. 2011;55(1):6–15. doi:https://doi.org/10.1093/annhyg/meq068
- Choi J-W, Kim M-J, Lee J-Y. Efficacy of cooling vests for alleviating heat strain of farm workers in summer. J Korean Soc Cloth Text. 2005;29(8):1176–1187.
- Yang YC, Wei MC, Hong SJ. Evaluation of occupation hot exposure in industrial workplaces in a subtropical country. Article. Int J Occup Med Environ Health. 2017;30(3):379–395. doi:https://doi.org/10.13075/ijomeh.1896.00761
- Phanprasit W, Rittaprom K, Dokkem S, et al. Climate Warming and Occupational Heat and Hot Environment Standards in Thailand. Saf Health Work. 2021;12(1):119–126. doi:https://doi.org/10.1016/j.shaw.2020.09.008
- Mac V, Elon L, Mix J, et al. Risk Factors for Reaching Core Body Temperature Thresholds in Florida Agricultural Workers. J. Occup. Environ. Med. 2021;63(5):395–402. doi:https://doi.org/10.1097/jom.0000000000002150
- Meade RD, Lauzon M, Poirier MP, Flouris AD, Kenny GP. An evaluation of the physiological strain experienced by electrical utility workers in north America. J Occup Environ Hyg. 2015;12(10):708–20. doi:https://doi.org/10.1080/15459624.2015.1043054
- Spector JT, Krenz J, Calkins M, et al. Associations between heat exposure, vigilance, and balance performance in summer tree fruit harvesters. Appl Ergon. 2018;67:1–8. doi:https://doi.org/10.1016/j.apergo.2017.09.002
- Mix J, Elon L, Vi Thien Mac V, et al. Hydration Status, Kidney Function, and Kidney Injury in Florida Agricultural Workers. J. Occup. Environ. Med. 2018;60(5):e253–e260. doi:https://doi.org/10.1097/JOM.0000000000001261
- Brake DJ, Bates GP. Fluid losses and hydration status of industrial workers under thermal stress working extended shifts. Occup. Environ. Med. 2003;60(2):90–96. doi:https://doi.org/10.1136/oem.60.2.90
- Notley SR, Flouris AD, Kenny GP. Occupational heat stress management: Does one size fit all? American Am. J. Ind. Med. 2019;62(12):1017–1023. doi:https://doi.org/10.1002/ajim.22961
- Notley SR, Akerman AP, Friesen BJ, et al. Exercise-heat tolerance in middle-aged-to-older men with type 2 diabetes. Acta Diabetol. 2021. doi:https://doi.org/10.1007/s00592-021-01684-z
- Song Y, Min J, Yu Y, et al. Wireless battery-free wearable sweat sensor powered by human motion. Sci. Adv. 2020;6(40):eaay9842. doi:https://doi.org/10.1126/sciadv.aay9842
- Boutry CM, Beker L, Kaizawa Y, et al. Biodegradable and flexible arterial-pulse sensor for the wireless monitoring of blood flow. Nat. Biomed. Eng. 2019;3(1):47–57. doi:https://doi.org/10.1038/s41551-018-0336-5
- Notley SR, Flouris AD, Kenny GP. On the use of wearable physiological monitors to assess heat strain during occupational heat stress. Appl Physiol Nutr Metab. 2018;43(9):869–881. doi:https://doi.org/10.1139/apnm-2018-0173 %M 29726698
- Foster J, Hodder SG, Lloyd AB, Havenith G. Individual Responses to Heat Stress: Implications for Hyperthermia and Physical Work Capacity. Review. Front. Physiol. 2020;11(1147). doi:https://doi.org/10.3389/fphys.2020.541483
- Foster J, Smallcombe JW, Hodder SG, et al. Aerobic fitness as a parameter of importance for labour loss in the heat. J Sci Med Sport. 2021;24(8):824–830. doi:https://doi.org/10.1016/j.jsams.2021.05.002
- Nybo L, Nielsen B. Hyperthermia and central fatigue during prolonged exercise in humans. J. Appl. Physiol. 2001;91(3):1055–1060. doi:https://doi.org/10.1152/jappl.2001.91.3.1055
- Nielsen B, Nybo L. Cerebral Changes During Exercise in the Heat. Sports Med. 2003;33(1):1–11. doi:https://doi.org/10.2165/00007256-200333010-00001
- Gaoua N, Racinais S, Grantham J, El Massioui F. Alterations in cognitive performance during passive hyperthermia are task dependent. Int J Hyperthermia. 2011;27(1):1–9. doi:https://doi.org/10.3109/02656736.2010.516305
- Gaoua N. Cognitive function in hot environments: a question of methodology. Scandinavian J Sci Med Sport. 2010;20(s3):60–70. doi:https://doi.org/10.1111/j.1600-0838.2010.01210.x
- Racinais S, Gaoua N, Grantham J. Hyperthermia impairs short-term memory and peripheral motor drive transmission. J Physiol. 2008;586(19):4751–4762. doi:https://doi.org/10.1113/jphysiol.2008.157420
- Axelson O. Influence of heat exposure on productivity. Work Environ Health. 1974;11(2):94–9.
- Fiala D, Havenith G. Modelling Human Heat Transfer and Temperature Regulation. In: Gefen A, Epstein Y, eds. The Mechanobiology and Mechanophysiology of Military-Related Injuries. Springer International Publishing, Switzerland; 2016:265–302.
- Dunne JP, Stouffer RJ, John JG. Reductions in labour capacity from heat stress under climate warming. Nat. Clim. Change. 2013;3(6):563–566. doi:https://doi.org/10.1038/nclimate1827
- Bröde P, Fiala D, Lemke B, Kjellstrom T. Estimated work ability in warm outdoor environments depends on the chosen heat stress assessment metric. Int J Biometeorol. 2018;62(3):331–345. doi:https://doi.org/10.1007/s00484-017-1346-9
- Galloway SD, Maughan RJ. Effects of ambient temperature on the capacity to perform prolonged cycle exercise in man. Med Sci Sports Exerc. 1997;29(9):1240–9. doi:https://doi.org/10.1097/00005768-199709000-00018
- Jay O, Hoelzl R, Weets J, et al. Fanning as an alternative to air conditioning – A sustainable solution for reducing indoor occupational heat stress. Energy Build. 2019;193:92–98. doi:https://doi.org/10.1016/j.enbuild.2019.03.037
- Wyndham CH. The effects of heat stress upon human productivity. Arch Sci Physiol (Paris). 1973;27(4):491–7.
- Vogt JJ, Libert JP, Candas V, Daull F, Mairiaux P. Heart rate and spontaneous work-rest cycles during exposure to heat. Ergonomics. 1983;26(12):1173–85. doi:https://doi.org/10.1080/00140138308963453
- Mairiaux P, Malchaire J. Workers self-pacing in hot conditions: a case study. Appl Ergon. 1985;16(2):85–90. doi:https://doi.org/10.1016/0003-6870(85)90209-1
- Miller V, Bates G, Schneider JD, Thomsen J. Self-Pacing as a Protective Mechanism against the Effects of Heat Stress. Ann Occup Hyg. 2011;55(5):548–555. doi:https://doi.org/10.1093/annhyg/mer012
- Pogačar T, Casanueva A, Kozjek K, et al. The effect of hot days on occupational heat stress in the manufacturing industry: implications for workers’ well-being and productivity. Int J Biometeorol. 2018;62(7):1251–1264. doi:https://doi.org/10.1007/s00484-018-1530-6
- World Health Organization. Regional office for Europe, Andersen KL, Masironi R, et al. Habitual physical activity and health. WHO regional publications European series;6. World Health Organization. Regional Office for Europe; 1978. https://apps.who.int/iris/handle/10665/272957.
- Crandall CG, Wilson TE. Human cardiovascular responses to passive heat stress. Compr Physiol. 2015;5(1):17–43. doi:https://doi.org/10.1002/cphy.c140015
- Rowell LB. Cardiovascular Adjustments to Thermal Stress. In Rowell LB, editor., Handbook of Physiology, The Cardiovascular System. Bethesda, MD, USA: American Physiological Society. 1983. 967–1024.
- Nag PK. Predicting maximal oxygen uptake of workers engaged in agricultural tasks. J Hum Ergol. 1981;10(1):25–33. doi:https://doi.org/10.11183/jhe1972.10.25
- Margaria R, Aghemo P, Rovelli E. Indirect determination of maximal O2 consumption in man. J. Appl. Physiol. 1965;20(5):1070–1073. doi:https://doi.org/10.1152/jappl.1965.20.5.1070
- Wingo JE, Cureton KJ. Maximal oxygen uptake after attenuation of cardiovascular drift during heat stress. Aviat Space Environ Med. 2006;77(7):687–94.
- Foster J, Smallcombe JW, Hodder S, et al. Quantifying the impact of heat on human physical work capacity; part III: the impact of solar radiation varies with air temperature, humidity, and clothing coverage. Int J Biometeorol. 2022;66(1):175–188. doi:https://doi.org/10.1007/s00484-021-02205-x
- Foster J, Smallcombe JW, Hodder S, Jay O, Flouris AD, Havenith G. Quantifying the impact of heat on human physical work capacity; part II: the observed interaction of air velocity with temperature, humidity, sweat rate, and clothing is not captured by most heat stress indices. Int J Biometeorol. 2021. doi:https://doi.org/10.1007/s00484-021-02212-y
- Smallcombe JW, Foster J, Hodder S, Jay O, Flouris AD, Havenith G. Quantifying physical work capacity in the heat: one hour versus full-day heat exposure. presented at: International Conference of Environmental Ergonomics; 2019; Amsterdam, Netherlands.
- Zivin JG, Neidell M. Temperature and the Allocation of Time: Implications for Climate Change. J Labor Econ. 2014;32(1):1–26. doi:https://doi.org/10.1086/671766
- Hsiang S, Kopp R, Jina A, et al. Estimating economic damage from climate change in the United States. Science. 2017;356(6345):1362–1369. doi:https://doi.org/10.1126/science.aal4369
- Morrissey MC, Brewer GJ, Williams WJ, Quinn T, Casa DJ. Impact of occupational heat stress on worker productivity and economic cost. Am. J. Ind. Med. 2021;64 (12):981–988. doi:https://doi.org/10.1002/ajim.23297
- Maughan RJ, Otani H, Watson P. Influence of relative humidity on prolonged exercise capacity in a warm environment. Eur. J. Appl. Physiol. 2012;112(6):2313–2321. doi:https://doi.org/10.1007/s00421-011-2206-7
- Nybo L, Rasmussen P, Sawka MN. Performance in the heat-physiological factors of importance for hyperthermia-induced fatigue. Compr Physiol. 2014;4(2):657–89. doi:https://doi.org/10.1002/cphy.c130012
- Bongers CCWG, Eijsvogels TMH. Time-motion analysis in the big data era: A promising method to assess the effects of heat stress on physical performance. Temperature. 2018;5(3):197–198. doi:https://doi.org/10.1080/23328940.2018.1462656
- Kenny GP, Vierula M, Maté J, Beaulieu F, Hardcastle SG, Reardon F. A field evaluation of the physiological demands of miners in Canada’s deep mechanized mines. J Occup Environ Hyg. 2012;9(8):491–501. doi:https://doi.org/10.1080/15459624.2012.693880
- Meade RD, Lauzon M, Poirier MP, Flouris AD, Kenny GP. The physical demands of electrical utilities work in North America. J Occup Environ Hyg. 2016;13(1):60–70. doi:https://doi.org/10.1080/15459624.2015.1077966
- Franke RH, Kaul JD. The Hawthorne Experiments: First Statistical Interpretation. Am. Sociol. Rev. 1978;43(5):623–643. doi:https://doi.org/10.2307/2094540
- Ainsworth BE, Haskell WL, Leon AS, et al. Compendium of physical activities: classification of energy costs of human physical activities. Med Sci Sports Exerc. 1993;25(1):71–80. doi:https://doi.org/10.1249/00005768-199301000-00011
- Poulianiti KP, Havenith G, Flouris AD. Metabolic energy cost of workers in agriculture, construction, manufacturing, tourism, and transportation industries. Ind. Health. 2018;57(3):283–305. doi:https://doi.org/10.2486/indhealth.2018-0075
- Ioannou LG, Liang J, Tsoutsoubi L, et al. The impact of climate change on agricultural labour productivity: implications for human mobility and poverty. under review. 2021.
- Dally M, Butler-Dawson J, Krisher L, et al. The impact of heat and impaired kidney function on productivity of Guatemalan sugarcane workers. PLoS One. 2018;13(10):e0205181. doi:https://doi.org/10.1371/journal.pone.0205181
- Langkulsen U, Vichit-Vadakan N, Taptagaporn S. Health impact of climate change on occupational health and productivity in Thailand. Glob Health Action. 2010;3(1):5607. doi:https://doi.org/10.3402/gha.v3i0.5607
- Quiller G, Krenz J, Ebi K, et al. Heat exposure and productivity in orchards: Implications for climate change research. Arch Environ Occup Health. 2017;72(6):313–316. doi:https://doi.org/10.1080/19338244.2017.1288077
- Sadiq LS, Hashim Z, Osman M. The Impact of Heat on Health and Productivity among Maize Farmers in a Tropical Climate Area. J Environ Public Health. 2019; 2019:9896410. doi:https://doi.org/10.1155/2019/9896410
- Gun RT, Budd GM. Effects of thermal, personal and behavioural factors on the physiological strain, thermal comfort and productivity of Australian shearers in hot weather. Ergonomics. 1995;38(7):1368–84. doi:https://doi.org/10.1080/00140139508925195
- Chinnadurai J, Venugopal V, P K, R P. Influence of occupational heat stress on labour productivity – a case study from Chennai, India. Int. J. Product. Perform. 2016;65(2):245–255. doi:https://doi.org/10.1108/IJPPM-08-2014-0121
- Ioannou LG, Gkikas G, Mantzios K, Tsoutsoubi L, Flouris AD. Chapter 32 - Risk assessment for heat stress during work and leisure. In: Tsatsakis AM, ed. Toxicological Risk Assessment and Multi-System Health Impacts from Exposure. Academic Press, Amsterdam, Netherlands; 2021:373–385. doi: https://doi.org/10.1016/B978-0-323-85215-9.00004-0
- Stapleton JM, Poirier MP, Flouris AD, et al. Aging impairs heat loss, but when does it matter? J. Appl. Physiol. 2015;118(3):299–309. doi:https://doi.org/10.1152/japplphysiol.00722.2014
- Notley SR, Poirier MP, Hardcastle SG, et al. Aging Impairs Whole-Body Heat Loss in Women under Both Dry and Humid Heat Stress. Med Sci Sports Exerc. 2017;49(11):2324–2332. doi:https://doi.org/10.1249/mss.0000000000001342
- Carrillo AE, Flouris AD, Herry CL, et al. Age-related reductions in heart rate variability do not worsen during exposure to humid compared to dry heat: A secondary analysis. Temperature. 2019;6(4):341–345. doi:https://doi.org/10.1080/23328940.2019.1684791
- Notley SR, Meade RD, D’Souza AW, McGarr GW, Kenny GP. Cumulative effects of successive workdays in the heat on thermoregulatory function in the aging worker. Temperature. 2018;5(4):293–295. doi:https://doi.org/10.1080/23328940.2018.1512830
- Notley SR, Lamarche DT, Meade RD, Flouris AD, Kenny GP. Revisiting the influence of individual factors on heat exchange during exercise in dry heat using direct calorimetry. Exp. Physiol. 2019;104(7):1038–1050. doi:https://doi.org/10.1113/EP087666
- Manjunath G, Aravindhakshan R, Varghese S. Effect of fasting during Ramadan on thermal stress parameters. East Mediterr Health J. 2019;25(1):34–39. doi:https://doi.org/10.26719/emhj.18.013
- Webb LH, Parsons KC. Case studies of thermal comfort for people with physical disabilities. Transactions-American Society of Heating Refrigerating and Air Conditioning Engineers. 1998;104:883–895.
- Price MJ, Trbovich M. Chapter 50 - Thermoregulation following spinal cord injury. In: Romanovsky AA, ed. Handbook of Clinical Neurology. Elsevier, Amsterdam, Netherlands; 2018:799–820.
- Jablonski NG, Chaplin G. The Evolution of Skin Pigmentation and Hair Texture in People of African Ancestry. Dermatol. Clin. 2014;32(2):113–121. doi:https://doi.org/10.1016/j.det.2013.11.003
- Havenith G, Griggs K, Qiu Y, Dorman L, Kulasekaran V, Hodder S. Higher comfort temperature preferences for anthropometrically matched Chinese and Japanese versus white-western-middle-European individuals using a personal comfort / cooling system. Build Environ. 2020;183:107162. doi:https://doi.org/10.1016/j.buildenv.2020.107162
- Stansberry KB, Shapiro SA, Hill MA, McNitt PM, Meyer MD, Vinik AI. Impaired Peripheral Vasomotion in Diabetes. Diabetes Care. 1996;19(7):715–721. doi:https://doi.org/10.2337/diacare.19.7.715
- Carter MR, McGinn R, Barrera-Ramirez J, Sigal RJ, Kenny GP. Impairments in local heat loss in type 1 diabetes during exercise in the heat. Med Sci Sports Exerc. 2014;46(12):2224–2233. doi:https://doi.org/10.1249/mss.0000000000000350
- Kaciuba-Uscilko H, Grucza R. Gender differences in thermoregulation. Curr. Opin. Clin. Nutr. Metab. Care. 2001;4(6)
- Périard JD, Racinais S, Sawka MN. Adaptations and mechanisms of human heat acclimation: Applications for competitive athletes and sports. Scand J Med Sci Sports. 2015;25(S1):20–38. doi:https://doi.org/10.1111/sms.12408
- Périard JD, Travers GJS, Racinais S, Sawka MN. Cardiovascular adaptations supporting human exercise-heat acclimation. Auton Neurosci. 2016;196:52–62. doi:https://doi.org/10.1016/j.autneu.2016.02.002
- Tyler CJ, Reeve T, Hodges GJ, Cheung SS. The Effects of Heat Adaptation on Physiology, Perception and Exercise Performance in the Heat: A Meta-Analysis. Sports Med. Nov 2016;46(11):1699–1724. doi:https://doi.org/10.1007/s40279-016-0538-5
- Cheung SS, McLellan TM. Heat acclimation, aerobic fitness, and hydration effects on tolerance during uncompensable heat stress. J. Appl. Physiol. 1998;84(5):1731–1739. doi:https://doi.org/10.1152/jappl.1998.84.5.1731
- Shkolnik A, Taylor CR, Finch V, Borut A. Why do Bedouins wear black robes in hot deserts? Nature. 1980;283(5745):373–375. doi:https://doi.org/10.1038/283373a0
- Havenith G. Interaction of clothing and thermoregulation. Exog. dermatol. 2002;1(5):221–230.
- Havenith G. Clothing and thermoregulation. Textiles and the Skin Curr Probl Dermatol Basel, Karger. 2003;31:35–49.
- Meade RD, D’Souza AW, Krishen L, Kenny GP. The physiological strain incurred during electrical utilities work over consecutive work shifts in hot environments: A case report. J Occup Environ Hyg. 2017;14(12):986–994. doi:https://doi.org/10.1080/15459624.2017.1365151
- Notley SR, Meade RD, Friesen BJ, D’Souza AW, Kenny GP. Does a prolonged work day in the heat impair heat loss on the next day in young men? Med Sci Sports Exerc. 2018;50(2):318–326. doi:https://doi.org/10.1249/mss.0000000000001444
- Notley SR, Meade RD, D’Souza AW, Friesen BJ, Kenny GP. Heat loss is impaired in older men on the day after prolonged work in the heat. Med Sci Sports Exerc. 2018;50(9):1859–1867. doi:https://doi.org/10.1249/mss.0000000000001643
- Ioannou LG, Mantzios K, Tsoutsoubi L, et al. Indicators to assess physiological heat strain - Part 1: Systematic review. Temperature. 2022. doi: https://doi.org/10.1080/23328940.2022.2037376
- Ioannou LG, Tsoutsoubi L, and Mantzios K, et al. Indicators to assess physiological heat strain - Part 3: Multi-country field evaluation and consensus recommendations. Temperature. 2022. doi: https://doi.org/10.1080/23328940.2022.2044739
- Morris NB, Jay O, Flouris AD, et al. Sustainable solutions to mitigate occupational heat strain – an umbrella review of physiological effects and global health perspectives. J. Environ. Health. 2020;19(1):95. doi:https://doi.org/10.1186/s12940-020-00641-7185.
- Morris NB, English T, Hospers L, Capon A, Jay O. The Effects of Electric Fan Use Under Differing Resting Heat Index Conditions: A Clinical Trial. Ann. Intern. Med. 2019;171(9):675–677. doi:https://doi.org/10.7326/M19-0512186.
- Morris NB, Chaseling GK, English T, et al. Electric fan use for cooling during hot weather: a biophysical modelling study. Lancet Planet. Health. 2021;5(6): e368–e377. doi:https://doi.org/10.1016/S2542-5196(21)00136-4
- Fiala D. Dynamic simulation of human heat transfer and thermal comfort. De Montfort University Leicester, UK; 1998.
- Flouris AD, Schlader ZJ. Human behavioral thermoregulation during exercise in the heat. Scand J Med Sci Sports. 2015;25 (S1):52–64. doi:https://doi.org/10.1111/sms.12349
- Westerterp KR. Diet induced thermogenesis. Nutr. Metab. 2004;1(1):5. doi:https://doi.org/10.1186/1743-7075-1-5
- Sawka MN, Gonzalez RR, Pandolf KB. Effects of sleep deprivation on thermoregulation during exercise. American Journal of Physiology-Regulatory, Integrative and Comparative Physiology. 1984;246(1):R72–R77. doi:https://doi.org/10.1152/ajpregu.1984.246.1.R72
- Keramidas ME, Botonis PG. Short-term sleep deprivation and human thermoregulatory function during thermal challenges. Exp. Physiol. 2021;106 (5):1139–1148. doi:https://doi.org/10.1113/EP089467
- Tokizawa K, Sawada S-i, Tai T, et al. Effects of partial sleep restriction and subsequent daytime napping on prolonged exertional heat strain. Occup. Environ. Med. 2015;72(7):521–528. doi:https://doi.org/10.1136/oemed-2014-102548
- Legault G, Clement A, Kenny GP, Hardcastle S, Keller N. Cognitive consequences of sleep deprivation, shiftwork, and heat exposure for underground miners. Appl Ergon. 2017;58:144–150. doi:https://doi.org/10.1016/j.apergo.2016.06.007
- Sawka MN, Montain SJ, Latzka WA. Hydration effects on thermoregulation and performance in the heat. Comparative Biochemistry and Physiology Part A: Molecular & Integrative Physiology. 2001;128(4):679– 690. doi:https://doi.org/10.1016/S1095-6433(01)00274-4
- Wang Z, de Dear R, Luo M, et al. Individual difference in thermal comfort: A literature review. Build Environ. 2018;138:181–193. doi:https://doi.org/10.1016/j.buildenv.2018.04.040
- Xiang J, Hansen A, Pisaniello D, Bi P. Perceptions of Workplace Heat Exposure and Controls among Occupational Hygienists and Relevant Specialists in Australia. PLOS ONE. 2015;10(8):e0135040. doi:https://doi.org/10.1371/journal.pone.0135040
- Bain AR, Jay O. Does summer in a humid continental climate elicit an acclimatization of human thermoregulatory responses? Eur. J. Appl. Physiol. 2011;111(6):1197–1205. doi:https://doi.org/10.1007/s00421-010-1743-9
- Zander KK, Botzen WJW, Oppermann E, Kjellstrom T, Garnett ST. Heat stress causes substantial labour productivity loss in Australia. Nat. Clim. Change. 2015;5(7):647–651. doi:https://doi.org/10.1038/nclimate2623
- Bodin T, García-Trabanino R, Weiss I, et al. Intervention to reduce heat stress and improve efficiency among sugarcane workers in El Salvador: Phase 1. Occup Environ Med. 2016;73(6):409–16. doi:https://doi.org/10.1136/oemed-2016-103555
- DeGroot DW, Gallimore RP, Thompson SM, Kenefick RW. Extremity cooling for heat stress mitigation in military and occupational settings. J. Therm. Biol. 2013;38(6):305–310. doi:https://doi.org/10.1016/j.jtherbio.2013.03.010
- Morris NB, Gruss F, Lempert S, et al. A Preliminary Study of the Effect of Dousing and Foot Immersion on Cardiovascular and Thermal Responses to Extreme Heat. JAMA. 2019;322(14):1411–1413. doi:https://doi.org/10.1001/jama.2019.13051
- Cramer MN, Huang M, Moralez G, Crandall CG. Keeping older individuals cool in hot and moderately humid conditions: wetted clothing with and without an electric fan. J. Appl. Physiol. 2020;128 (3):604–611. doi:https://doi.org/10.1152/japplphysiol.00786.2019
- Muñoz CX, Carney KR, Schick MK, Coburn JW, Becker AJ, Judelson DA. Effects of oral rehydration and external cooling on physiology, perception, and performance in hot, dry climates. Scand J Med Sci Sports. 2012;22(6):e115–24. doi:https://doi.org/10.1111/j.1600-0838.2012.01510.x
- Watt J. Evaporative air conditioning handbook. 2nd ed. Chapman and Hall, New York, USA; 1986.
- Chan AP, Song W, Yang Y. Meta-analysis of the effects of microclimate cooling systems on human performance under thermal stressful environments: potential applications to occupational workers. J Therm Biol. 2015;49-50:16–32. doi:https://doi.org/10.1016/j.jtherbio.2015.01.007
- Jay O, Morris NB. Does Cold Water or Ice Slurry Ingestion During Exercise Elicit a Net Body Cooling Effect in the Heat? Sports Med. 2018;48(Suppl 1):17–29. doi:https://doi.org/10.1007/s40279-017-0842-8
- Burdon CA, Johnson NA, Chapman PG, O’Connor HT. Influence of Beverage Temperature on Palatability and Fluid Ingestion During Endurance Exercise: a systematic review. Int J Sport Nutr Exerc Metab. 15, 2012.
- United Nations Development Programme. Climate Change and Labor: Impacts of Heat in the Workplace. U.N. Dev Program. Accessed October 7, 2020. www.undp.org/content/undp/en/home/librarypage/climate-and-disaster-resilience-/tackling-challenges-of-climate-change-and-workplace-heat-for-dev.html
- Venugopal V, Rekha S, Manikandan K, et al. Heat stress and inadequate sanitary facilities at workplaces – an occupational health concern for women? Glob. Health Action. 2016;9(1): 31945. doi:https://doi.org/10.3402/gha. v9.31945
- D’Souza AW, Notley SR, Kenny GP. The Relation between Age and Sex on Whole-Body Heat Loss during Exercise-Heat Stress. Med Sci Sports Exerc. 2020;52 (10):2242–2249. doi:https://doi.org/10.1249/mss.0000000000002373
- Gagnon D, Kenny GP. Does sex have an independent effect on thermoeffector responses during exercise in the heat? J Physiol. 2012;590(23):5963– 5973. doi:https://doi.org/10.1113/jphysiol.2012.240739
- Poirier MP, Meade RD, McGinn R, et al. The Influence of Arc-Flash and Fire-Resistant Clothing on Thermoregulation during Exercise in the Heat. J Occup Environ Hyg. 2015;12(9):654–67. doi:https://doi.org/10.1080/15459624.2015.1029615
- Stapleton JM, Hardcastle SG, Kenny GP. The influence of activewear worn under standard work coveralls on whole-body heat loss. J Occup Environ Hyg. 2011;8(11):652–661. doi:https://doi.org/10.1080/15459624.2011.616814
- Kjellstrom T, Holmer I, Lemke B. Workplace heat stress, health and productivity – an increasing challenge for low and middle-income countries during climate change. Glob. Health Action. 2009;2(1):2047. doi:https://doi.org/10.3402/gha.v2i0.2047
- Morabito M, Messeri A, Noti P, et al. An Occupational Heat-Health Warning System for Europe: The HEAT- SHIELD Platform. Int J Environ Res Public Health. 2019;16(16). doi:https://doi.org/10.3390/ijerph16162890
- Scandella F. Firefighters: feeling the heat. European Trade Union Institute, Brussels, Belgium; 2012.
- Wright-Beatty HE, McLellan TM, Larose J, Sigal RJ, Boulay P, Kenny GP. Inflammatory responses of older Firefighters to intermittent exercise in the heat. Eur. J. Appl. Physiol. 2014;114 (6):1163–1174. doi:https://doi.org/10.1007/s00421-014-2843-8
- Patel H, Rao NM, Saha A. Heat exposure effects among firefighters. Case Report. Indian J. Occup. Environ. Med. 2006;10 (3):121–123. doi:https://doi.org/10.4103/0019-5278.29572
- Petruzzello SJ, Gapin JI, Snook E, Smith DL. Perceptual and physiological heat strain: Examination in firefighters in laboratory- and field-based studies. Ergonomics. 2009;52(6):747–754. doi:https://doi.org/10.1080/00140130802550216
- Kenny GP, Larose J, Wright-Beatty HE, Boulay P, Sigal RJ, Flouris AD. Older firefighters are susceptible to age-related impairments in heat dissipation. Med Sci Sports Exerc. 2015;47(6):1281–90. doi:https://doi.org/10.1249/mss.0000000000000537
- Wright HE, Larose J, McLellan TM, Miller S, Boulay P, Kenny GP. Do older firefighters show long-term adaptations to work in the heat? J Occup Environ Hyg. 2013;10(12):705–715. doi:https://doi.org/10.1080/15459624.2013.821574
- Lui B, Cuddy JS, Hailes WS, Ruby BC. Seasonal heat acclimatization in wildland firefighters. J. Therm. Biol. 2014;45:134–140. doi:https://doi.org/10.1016/j.jtherbio.2014.08.009
- Oksa J, Rintamäki H, Takatalo K, et al. Firefighters muscular recovery after a heavy work bout in the heat. Appl Physiol Nutr Metab. 2013;38(3):292–9. doi:https://doi.org/10.1139/apnm-2012-0180
- Walker A, Argus C, Driller M, Rattray B. Repeat work bouts increase thermal strain for Australian firefighters working in the heat. Int J Occup Environ Health. 2015;21 (4):285–293. doi:https://doi.org/10.1179/2049396715Y.0000000006
- Larsen B, Snow R, Vincent G, Tran J, Wolkow A, Aisbett B. Multiple Days of Heat Exposure on Firefighters’ Work Performance and Physiology. PloS one. 2015;10(9):e0136413–e0136413. doi:https://doi.org/10.1371/journal.pone.0136413
- Larsen B, Snow R, Aisbett B. Effect of heat on firefighters’ work performance and physiology. J. Therm. Biol. 2015;53:1–8. doi:https://doi.org/10.1016/j.jtherbio.2015.07.008
- Parsons IT, Stacey MJ, Woods DR. Heat Adaptation in Military Personnel: Mitigating Risk, Maximizing Performance Review. Front. Physiol. 2019;10 (1485)doi:https://doi.org/10.3389/fphys.2019.01485
- Carter III R, Cheuvront SN, Williams JO, et al. Epidemiology of hospitalizations and deaths from heat illness in soldiers. 2005.
- Nelson DA, Deuster PA, O’Connor FG, Kurina LM. Sickle Cell Trait and Heat Injury Among US Army Soldiers. Am. J. Epidemiol. 2017;187 (3):523–528. doi:https://doi.org/10.1093/aje/kwx285
- Radakovic SS, Maric J, Surbatovic M, et al. Effects of Acclimation on Cognitive Performance in Soldiers during Exertional Heat Stress. Mil. Med. 2007;172 (2):133–136. doi:https://doi.org/10.7205/milmed.172.2.133
- Moore AC, Stacey MJ, Bailey KGH, et al. Risk factors for heat illness among British soldiers in the hot Collective Training Environment . J. R. Army Med.Corps. 2016;162(6):434–439. doi:https://doi.org/10.1136/jramc-2015-000427
- Rintamäki H, Kyröläinen H, Santtila M, et al. From the Subarctic to the Tropics: Effects of 4-Month Deployment on Soldiers’ Heat Stress, Heat Strain, and Physical Performance. J. Strength Cond. Res. 2012;26:S45–S52. doi:https://doi.org/10.1519/JSC.0b013e31825d817e
- O’Hara R, Eveland E, Fortuna S, Reilly P, Pohlman R. Current and Future Cooling Technologies Used in Preventing Heat Illness and Improving Work Capacity for Battlefield Soldiers: Review of the Literature. Mil. Med. 2008;173(7):653–657. doi:https://doi.org/10.7205/milmed.173.7.653
- Epstein Y, Druyan A, Heled Y. Heat Injury Prevention —A Military Perspective. J. Strength Cond. Res. 2012;26:S82–S86. doi:https://doi.org/10.1519/JSC.0b013e31825cec4a
- Dimitrov RS. The Paris Agreement on Climate Change: Behind Closed Doors. Glob. Environ. Politics. 2016;16(3):1–11. doi:https://doi.org/10.1162/GLEP_a_00361
- Arias P, Bellouin N, Coppola E, et al. Technical Summary. In: Masson-Delmotte V, Zhai P, Pirani A, et al, eds. Climate Change 2021: The Physical Science Basis Contribution of Working Group I to the Sixth Assessment Report of the Intergovernmental Panel on Climate Change. Cambridge University Press, Geneva, Switzerland (In Press); 2021:4–101.
- Thuiller W. Climate change and the ecologist. Nature. 2007;448(7153):550–552. doi:https://doi.org/10.1038/448550a
- Bitencourt DP, Muniz Alves L, Shibuya EK, de Ângelo da Cunha I, Estevam de Souza JP. Climate change impacts on heat stress in Brazil—Past, present, and future implications for occupational heat exposure. Int. J. Climatol. 2021;41(S1): E2741–E2756. doi:https://doi.org/10.1002/joc.6877
- Olu J, Majekodunmi AS. Climate change impact on workers’ health, safety and productivity. Clim. Change. 2020;6(21):156–167.
- Applebaum KM, Graham J, Gray GM, et al. An Overview of Occupational Risks From Climate Change. Curr Environ Health Rep. 2016;3(1):13–22. doi:https://doi.org/10.1007/s40572-016-0081-4
- Ansah EW, Ankomah-Appiah E, Amoadu M, Sarfo JO. Climate change, health and safety of workers in developing economies: A scoping review. The Journal of Climate Change and Health. 2021;3:100034. doi:https://doi.org/10.1016/j.joclim.2021.100034
- Levy BS, Roelofs C. Impacts of Climate Change on Workers’ Health and Safety. Oxford University Press, Oxford, England, UK; 2019.
- Harari Arjona R, Piñeiros J, Ayabaca M, Harari Freire F. Climate change and agricultural workers’ health in Ecuador: occupational exposure to UV radiation and hot environments. Ann Ist Super Sanita. 2016;52 (3):368–373. doi:https://doi.org/10.4415/ann_16_03_08
- Schulte PA, Chun H. Climate Change and Occupational Safety and Health: Establishing a Preliminary Framework. J Occup Environ Hyg. 2009;6(9):542–554. doi:https://doi.org/10.1080/15459620903066008
- Moda HM, Filho WL, Minhas A. Impacts of Climate Change on Outdoor Workers and their Safety: Some Research Priorities. Int. J. Environ. Res. 2019;16(18):3458. doi:https://doi.org/10.3390/ijerph16183458
- Kjellstrom T, Oppermann E, Lee JKW. Climate Change, Occupational Heat Stress, Human Health, and Socioeconomic Factors. In: Theorell T, ed. Handbook of Socioeconomic Determinants of Occupational Health: From Macro-level to Micro-level Evidence. Springer International Publishing, Cham, Switzerland; 2020:71–89.
- Amini Mastaneh, Ghavamabadi LI, Rangkooy H, Dehaghi BF. Climate change and its effects on farm workers. Environ Eng Manag J. 2021;8(3)doi:https://doi.org/10.34172/EHEM.2021.xx
- Hanna EG, Kjellstrom T, Bennett C, Dear K. Climate Change and Rising Heat: Population Health Implications for Working People in Australia. Asia Pac J Public Health. 2011;23 ((2_suppl)):14S–26S. doi:https://doi.org/10.1177/1010539510391457
- Vanos J, Vecellio DJ, Kjellstrom T. Workplace heat exposure, health protection, and economic impacts: A case study in Canada. Am. J. Ind. Med. 2019;62(12):1024–1037. doi:https://doi.org/10.1002/ajim.22966
- Allen MD. Climate change in Alaska: Social workers’ attitudes, beliefs, and experiences. Int. J. Soc. Welf. 2020;29(4):310–320. doi:https://doi.org/10.1111/ijsw.12443
- Jones CD, Hughes JK, Bellouin N, et al. The HadGEM2-ES implementation of CMIP5 centennial simulations. Geosci Model Dev. 2011;4(3):543–570. doi:https://doi.org/10.5194/gmd-4-543-2011
- Collins W, Bellouin N, Doutriaux-Boucher M, et al. Evaluation of the HadGEM2 model. HCTN. 74; 2008.
- Khatib T, Elmenreich W. Modeling of photovoltaic systems using Matlab: Simplified green codes. John Wiley & Sons, Hoboken, New Jersey, USA; 2016.
- Nilsson M, Beaglehole R, Sauerborn R. Climate policy: lessons from tobacco control. The Lancet. 2009;374 (9706):1955–1956. doi:https://doi.org/10.1016/S0140-6736(09)61959-0
- Sauerborn R, Kjellstrom T, Nilsson M. Health as a crucial driver for climate policy. Glob. Health Action. 2009;2(1):2104. doi:https://doi.org/10.3402/gha.v2i0.2104
- Levy BS, Patz JA. Climate Change, Human Rights, and Social Justice. Ann. Glob. Health. 2015;81(3):310–322. doi:https://doi.org/10.1016/j.aogh.2015.08.008
- Gao C, Kuklane K, Östergren P-O, Kjellstrom T. Occupational heat stress assessment and protective strategies in the context of climate change. Int. J. Biometeorol. 2018;62 (3):359–371. doi:https://doi.org/10.1007/s00484-017-1352-y
- Casanueva A, Burgstall A, Kotlarski S, et al. Overview of Existing Heat-Health Warning Systems in Europe. Int. J. Environ. Res. 2019;16(15):2657. doi:https://doi.org/10.3390/ijerph16152657
- Ioannou LG, Dinas PC, and Notley SR, et al. Indicators to assess physiological heat strain - Part 2: Delphi exercise. Temperature. 2022. doi: https://doi.org/10.1080/23328940.2022.2044738
- Organization WH. Quantitative risk assessment of the effects of climate change on selected causes of death, 2030s and 2050s. 2014.
- Flouris AD, Kenny GP. Heat remains unaccounted for in thermal physiology and climate change research. F1000Research. 2017;6. doi:https://doi.org/10.12688/f1000research.10554.2
- Nybo L, Kjellstrom T, Bogataj LK, Flouris AD. Global heating: Attention is not enough; we need acute and appropriate actions. Temperature. 2017;4(3):199–201. doi:https://doi.org/10.1080/23328940.2017.1338930
- Perkins SE, Alexander LV, Nairn JR. Increasing frequency, intensity and duration of observed global heatwaves and warm spells. Geophys. Res. Lett. 2012;39(20)doi:https://doi.org/10.1029/2012GL053361
- Gubernot DM, Anderson GB, Hunting KL. Characterizing occupational heat-related mortality in the United States, 2000–2010: An analysis using the census of fatal occupational injuries database. Am. J. Ind. Med. 2015;58 (2):203–211. doi:https://doi.org/10.1002/ajim.22381
- Bonauto D, Anderson R, Rauser E, Burke B. Occupational heat illness in Washington State, 1995– 2005. Am. J. Ind. Med. 2007;50 (12):940–950. doi:https://doi.org/10.1002/ajim.20517
- Tawatsupa B, Yiengprugsawan V, Kjellstrom T, Berecki-Gisolf J, Seubsman S-A, Sleigh A. Association between Heat Stress and Occupational Injury among Thai Workers: Findings of the Thai Cohort Study. Ind. Health. 2013;51(1):34–46. doi:https://doi.org/10.2486/indhealth.2012-0138
- Animal control board. Sec. 531-401. General requirements for animal care and treatment. MC. Accessed September 09, 2021. https://library.municode.com/in/indianapolis_-_marion_county/codes/code_of_ordinances?nodeId=TITIIIPUHEWE_CH531AN_ARTIVCATR_S531-401GEREANCATR
- Gubernot DM, Anderson GB, Hunting KL. The epidemiology of occupational heat exposure in the United States: a review of the literature and assessment of research needs in a changing climate. Int. J. Biometeorol. 2014;58(8):1779–1788. doi:https://doi.org/10.1007/s00484-013-0752-x
- California Code of Regulations. Title 8, section 3395 - Heat Illness Prevention in Outdoor Places of Employment. Department of Industrial Relations. Accessed September 02 2020. www.dir.ca.gov/title8/3395.html
- Washington State Legislature. WAC 296-62-095: Outdoor heat exposure. Accessed September 02, 2020. www.leg.wa.gov/
- American Industrial Hygiene Association. Media alert: Maryland Enacts AIHA-Supported Bill Protecting Workers from Heat Stress. Accessed September 02, 2021. www.aiha.org/media-alerts/media-alert-mary land-enacts-aiha-supported-bill-protecting-workers- from-heat-stress
- American Conference of Governmental Industrial Hygienists. Cincinnati, Ohio, USA, TLVs and BEIs: Based on the Documentation of the Threshold Limit Values for Chemical Substances and Physical Agents & Biological Exposure Indices. 2012:130. 978-607260-48-6.
- Jackson LL, Rosenberg HR. Preventing heat-related illness among agricultural workers. J. Agromedicine. 2010;15(3):200–215. doi:https://doi.org/10.1080/1059924X.2010.487021
- Safety CCfOHa. Temperature Conditions - Hot. Accessed September 04, 2021. www.ccohs.ca/oshanswers/phys_agents/max_temp.html
- Jay O, Kenny GP. Heat exposure in the Canadian workplace. Am J Ind Med. 2010;53(8):842–53. doi:https://doi.org/10.1002/ajim.20827
- Ministry of Labor Welfare and Social Insurance: Department of Labor Inspection. Occupational heat strain. 4789: 291. Cyprus: Official Gazette of the Republic of Cyprus; 2014.
- Health and Safety Executive. Workplace health, safety and welfare: Workplace (Health, Safety and Welfare) Regulations 1992 - Approved Code of Practice and guidance. 2013.
- Mathee A, Oba J, Rose A. Climate change impacts on working people (the HOTHAPS initiative): findings of the South African pilot study. Glob. Health Action. 2010;3(1):5612. doi:https://doi.org/10.3402/gha.v3i0.5612
- Grivna M, Aw T-C, El-Sadig M, et al. The legal framework and initiatives for promoting safety in the United Arab Emirates. Int J Inj Contr Saf Promot. 2012;19(3):278–289. doi:https://doi.org/10.1080/17457300.2012.696660
- Joubert D, Thomsen J, Harrison O. Safety in the Heat: A Comprehensive Program for Prevention of Heat Illness Among Workers in Abu Dhabi, United Arab Emirates. Am. J. Public Health. 2011;101 (3):395–398. doi:https://doi.org/10.2105/ajph.2009.189563
- Schär C. The worst heat waves to come. Nat. Clim. Change. 2016;6(2):128–129. doi:https://doi.org/10.1038/nclimate2864
- Embassy of the United Arab Emirates. Labor rights in the United Arab Emirates, Washington, DC, USA. 2010.
- Yousef bin Mohamed Al-Othman Fakhroo. Specifying measures to protect workers from heat stress: No. (17) for the year 2021. Qatar Ministry of Administrative Development Labour and Social Affairs, Doha, Qatar; 2020.
- Zhou Z. Understanding the administrative regulation on occupational health and trend in China. J Occup Health. 2018;60(2):126–131. doi:https://doi.org/10.1539/joh.17-0229-RA
- Zhao Y, Sultan B, Vautard R, Braconnot P, Wang HJ, Ducharne A. Potential escalation of heat-related working costs with climate and socioeconomic changes in China. Proceedings of the National Academy of Sciences. 2016;113(17):4640–4645. doi:https://doi.org/10.1073/pnas.1521828113
- Seyfarth. China employment law alert: Summer is hotL Don’t get burned by inadvertent breach of statutory obligations! Seyfarth Shaw LLP. Accessed September 16, 2021. https://www.seyfarth.com/print/content/13610/china-employment-law-alert-summer-is-hot-don-t-get-burned-by-inadvertent-breach-of-statutory-obligations.pdf
- Guidlines on heat stress management (Ministry of Human Resources) 52 (2016).
- Authorised Version No. 35: Occupational Health and Safety Act 2004; No. 107 of 2004 (Authorised by the Chief Parliamentary Counsel) (2021).
- Wheeler SM. State and Municipal Climate Change Plans: The First Generation. J Am Plann Assoc. 2008;74(4):481–496. doi:https://doi.org/10.1080/01944360802377973
- Morrissey MC, Casa DJ, Brewer GJ, et al. Heat Safety in the Workplace: Modified Delphi Consensus to Establish Strategies and Resources to Protect the US Workers. GeoHealth. 2021;5(8):e2021GH000443. doi:https://doi.org/10.1029/2021GH000443
- Kasten F, Czeplak G. Solar and terrestrial radiation dependent on the amount and type of cloud. Sol Energy. 1980;24(2):177–189. doi:https://doi.org/10.1016/0038-092X(80)90391-6