Abstract
Objective: To determine the influence of niobium pentoxide (Nb2O5) addition on the physical and chemical properties of glass ionomer cements (GICs).
Materials and methods: Five, 10 or 20 wt.% of Nb2O5 were incorporated into commercial GICs (Maxxion R, Vitro Molar, Vitro Fil R) and one group of each GIC remained without Nb2O5 (control groups). The GICs were evaluated by Knoop hardness, compressive strength, acid erosion, particle size and radiopacity. Data were analyzed by two-way ANOVA followed by Tukey's test.
Results: The addition of 10% and 20% reduced the microhardness of two GICs (p < .05). Compressive strength showed no difference among groups (p > .05). Nb2O5 did not influence Maxxion R and Vitro Fil R regarding the acid erosion test (p > .05). Vitro Molar increased its acid erosion with 10% of Nb2O5 (p < .05). Maxxion R presented 15.78 μm, while Vitro Molar and Vitro Fil R showed 5.14 μm and 6.18 μm, respectively. As the Nb2O5 concentration increased, the radiopacity increased for all groups. Vitro Molar and Vitro Fil R did not present significant difference to at least 1 mm aluminum (p > .05).
Conclusion: The addition of 5 wt.% Nb2O5 did not affect the tested physical and chemical properties of the GICs and improved the radiopacity of one of the cements. These materials are therefore suitable for further testing of biomimetic remineralization properties.
Introduction
Glass ionomer cements (GICs) are widely used in clinical procedures due to its adhesion to dental tissues without prior treatment [Citation1–5] and friendly technique with reduced number of clinical steps.[Citation6] However, GICs present some shortcomings such as high solubility in oral environment and low wear resistance.[Citation4,Citation7] To overcome these issues, the formulation of the glass [Citation8] and the powder:liquid ratio [Citation4,Citation9] have been changed. In addition, inorganic fillers have been incorporated to GICs.[Citation10,Citation11]
The first attempts to increase the filler contents of GICs were the addition of silver–tin amalgam alloy powder [Citation12] with no significant improvement in mechanical properties.[Citation13–15] Fused amalgam was sintered to glass particles,[Citation16] zinc sulfate,[Citation17] zinc oxide,[Citation18] titanium tetrafluoride,[Citation11] ytterbium fluoride, barium sulfate,[Citation19] strontium oxide and strontium fluoride [Citation20] to enhance the mechanical properties and fluoride release. Calcium phosphates were also added to develop GICs with bioactivity. Besides that, the radiopacity of GICs could be improved to overcome diagnostic of recurrent caries under restorations.[Citation21] Nevertheless, any of these fillers could improve physical and chemical properties with bioactivity.
Niobium pentoxide (Nb2O5) is a metal oxide generally presenting a monoclinic form which contributes to enhance the mechanical properties when incorporated to metal alloys.[Citation22] The addition to resin based dental materials has already showed improved the radiopacity [Citation23,Citation24] and microhardness [Citation23] of adhesive systems [Citation23] and root canal sealers.[Citation24] In addition to biocompatibility and to improve chemical and physical properties of materials, Nb2O5 presents bioactivity. In previous studies, Nb2O5 promoted the growth of hydroxyapatite crystals on its surface when it is in contact with simulated and human saliva [Citation25] or in simulated body fluid.[Citation26] The bioactivity of this oxide could enhance dentin remineralization after caries disease via biomimetic remineralization process.[Citation27] The evaluation of GICs with different Nb2O5 concentrations is essential to develop a material with suitable properties to be future tested by biomimetic remineralization approach. However, to the best of our knowledge, there are no reports on the use of Nb2O5 in commercial GICs.
Therefore, the purpose of this study was to determine the influence of different concentrations of Nb2O5 addition to commercial GICs’ surface hardness, compressive strength, acid erosion and radiopacity.
Materials and methods
Three different restorative GICs were used: Maxxion R (FGM Produtos Odontológicos, Joinville, SC, Brazil), Vitro Fil R (DFL Indústria e Comércio S.A., Rio de Janeiro, RJ, Brazil) and Vitro Molar (DFL Indústria e Comércio S.A., Rio de Janeiro, RJ, Brazil). The composition of the GICs is shown in . A high purity Nb2O5 (CBMM, Araxá, MG, Brazil) presenting a mean particle size of 38.16 μm was used as inorganic filler to GICs. The GICs powder and liquid were weighted by analytical balance (AUW220D, Shimadzu, Kyoto, Japan) using the volume according to manufactures’ instructions. The mean masses of powder and liquid were calculated through three measurements to use the same powder:liquid ratio indicated by manufactures. To obtain the experimental groups, 5 wt.%, 10 wt.% and 20 wt.% of GICs powder was replaced by Nb2O5. A group without addition of Nb2O5 was used as control for each commercial GIC. The GICs powder was hand mixed with Nb2O5 and manipulated with the liquid according to manufactures’ instructions.
Table 1. Commercial GICs and the respective composition provided by the manufacturers.
Surface microhardness
Five samples per group with 5.0 ± 0.5 mm in diameter and 1.0 mm ±0.2 thickness were prepared to evaluate GICs’ surface microhardness. The samples were included in a poly (methyl methacrylate) (PMMA) mould and polished with abrasive paper (#600 and #1200 grit SiC) and felt disc saturated with an alumina suspension (Alumina 1.0 μm, Arotec, Cotia, SP, Brazil). The samples were subjected to a microhardness test in a digital microhardness tester (HMV 2, Shimadzu, Tokyo, Japan) by means of three indentations per sample with 50 g per 10 s each one. The length of the larger diagonal was measured and the Knoop hardness was calculated with the following equation:
where 14,228 is a constant; c is the load (g) and d is the length of the larger diagonal (μm). The Knoop hardness was the mean of the three indentations for each sample and the values were expressed in Knoop hardness number (KHN).
Compressive strength
Compressive strength test was conducted according to ISO 9917-1,[Citation28] except for the sample sizes. Five samples per group presenting 6.0 ± 0.1 mm diameter and 12.0 ± 0.1 mm height were produced in a stainless steel mould to compressive strength assay. The samples were stored in a 100% moisture environment in a cabinet at 37 °C for 24 h. The diameter of the samples was calculated by taking the mean of two measurements using a digital caliper. The samples were placed between the plates of mechanical tester and a compressive load along the diametric axis of the sample with a cross head speed of 1 mm/min was applied. The maximum force applied, when the sample fractures were recorded, and the compressive strength (C) were calculated in MPa using the following equation:
where p is the maximum force applied (N) and d is the measured diameter of the sample (mm).
Acid erosion
Acid erosion test was conducted according to ISO 9917-1.[Citation28] Five samples with 5.0 ± 0.1 mm diameter and 2.0 ± 0.1 mm thickness were produced into a PMMA mould and stored for 24 h at 37 °C in a 100% moisture environment. After 24 h, an abrasive paper (#1200 grit SiC), with continuous water irrigation, was used to flat the samples. The initial depth at center of each sample was measured in μm with the mould in a plane reference, with a dial gauge. After the initial measurement, the samples were immersed in an eroding solution prepared also according to ISO 9917-1.[Citation28] Before immersions, the pH was checked at 2.74 ± 0.02. The mould with the samples were immerse in 30 mL of eroding solution face upwards during 24 h at 37 °C. After 24 h immersion, the final depth at the center of each sample was measured by the same way of initial depth. The final result is the difference between the initial and the final depth at the center of each sample in μm.
Radiopacity
Acid erosion test was conducted according to ISO 9917-1 [Citation28] except the diameter of the samples and the aluminum step-wedge thickness. Five samples with 5.0 ± 0.5 mm in diameter and 1.0 mm ±0.2 thickness were prepared. X-ray images were obtained with phosphorous plates in a digital system (VistaScan, Dürr Dental GmbH & Co. KG, Bietigheim-Bissingen, Germany) at 70 kV and 8 mA, with 0.2 s of exposure time and a focus-film distance of 400 mm. One sample from each commercial GIC with the same concentration of Nb2O5 was positioned in each film for a total of five films per concentration. An aluminum step-wedge was exposed simultaneously with the samples in all images. The aluminum step-wedge thickness ranged from 1.0 mm to 8.0 mm in increments of 1.0 mm. The images were saved in TIFF format. Digital images were handled with ImageJ software (Wayne Rasband, National Institutes of Health, EUA) and the means and standard deviations of the gray levels (pixel density) of the aluminum step-wedge and the samples were obtained in a standardized area of 1.5 mm2.
Particle size
The means of particle sizes of the three commercial GICs were evaluated using a laser diffraction particle size analyzer (CILAS 1180 Particle-Size-Analyzer, Compagnie Industrielle des Lasers, Orleans, Loiret, France). The results of the analysis are showed in μm.
Statistical analysis
Data were analyzed by two-way ANOVA (Nb2O5 concentration and cement brand) for Knoop microhardness, compressive strength and acid erosion tests and by one-way ANOVA for radiopacity assay (including the radiopacity of 1 and 2 mm Al in the comparisons), followed by Tukey's post hoc test. All analyses were performed at a 5% level of significance.
Results
shows the Knoop microhardness, the compressive strength and the acid erosion of the GICs. The Knoop microhardness ranged from 39.11 ± 1.08 to 63.09 ± 4.08. Compressive strength ranged from 21.67 ± 4.94 to 31.17 ± 12.45 MPa and it was not influenced by the Nb2O5 addition (p > .05) and there was no difference among the groups (p > .05). The addition of any concentration of Nb2O5 in Maxxion R and in Vitro Fil R did not influence the resistance to acid erosion of these cements (p > .05). However, the addition of 10% of Nb2O5 in Vitro Molar increased the acid degradation (p < .05).
Table 2. Means ± standard deviation of Knoop microhardness, compressive strength (MPa) and acid erosion (μm) as function of concentration of the Nb2O5 added to glass ionomer cements.
The radiopacity values of the experimental groups are presented in . The results showed an increase in radiopacity with the increase of Nb2O5 concentration. The group of Maxxion R with the addition of 10% of Nb2O5 had no statistical difference to 1 mm of aluminum (p > .05) and with the addition of 20% of the Nb2O5, this cement showed no statistical difference to 2 mm of aluminum (p > .05). The group of Vitro Molar without the addition of Nb2O5 showed no statistical difference to 1 mm of aluminum (p > .05) and with the addition of 20%, this cement had no statistical difference to 2 mm of aluminum (p > .05). The group of Vitro Fil R without the addition of Nb2O5 also showed no statistical difference to 1 mm of aluminum (p > .05), while with the addition of 5% of Nb2O5, this cement presented no statistical difference to 2 mm of aluminum (p > .05).
Figure 1. Radiopacity (pixels) as function of concentration of the Nb2O5 added to glass ionomer cements. Equal symbols refer to no difference (p > .05) against references (1 mm Al or 2 mm Al).
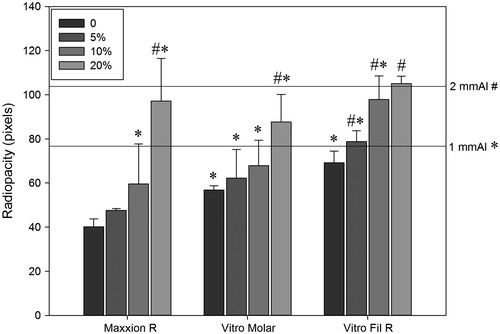
The analyses indicated different particles sizes among GICs brands. Maxxion R showed 15.78 μm, the higher mean size, while Vitro Molar and Vitro Fil R presented 5.14 μm and 6.18 μm, respectively.
Discussion
Conventional GICs can be used to restore and seal caries lesion without the need of electric, rotatory and photocuring devices.[Citation29,Citation30] In the present study, it was observed that the addition of 10 wt.% of Nb2O5 reduced microhardness values (p < .05) of two commercial GICs, while the other cement had it decreased at 20 wt.% of Nb2O5 addition (p < .05). Higher concentrations of Nb2O5 weakened the bulk of the cement and may lead to this result because this oxide is probably not chemically attacked by poly acrylic acid and it is not able to react in acid–basic reaction to take part in GICs setting.
In the current research, there was no statistical difference for compressive strength test (p > .05) among the groups with different Nb2O5 concentrations or different commercial GICs. GICs compressive strength is related to cross-linking of trivalent ions in the matrix and is a mechanical property of brittle materials related to masticatory forces in vivo. The tested filler was not able to interact specially due to its insolubility in the medium. At higher oxide concentrations, the disruption of the glass network [Citation10] or the low wetness of the glass particles by the acid [Citation31] can decrease GICs’ physical properties. However, Nb2O5 did not altered the compressive strength of the experimental cements in any concentration added.
Vitro Molar presented a better result in acid erosion test. Although it is not possible to evaluate in detail the differences among the compositions of the GICs with the information provided by manufactures, Vitro Molar is a high-viscosity GIC, consequently, it presents smaller particles and more amount of them. In this way, the power/liquid proportion is higher providing better physical behavior.[Citation32] In addition, it was showed that Vitro Molar, when compared to other brands, as Maxxion, presented lower roughness.[Citation33] This result can also be related to the better response of Vitro Molar compared to this GIC, since superficial characteristics influence the perform of the materials in front of acid erosion test. Two commercial cements showed no statistical difference (p > .05) between them at the same concentrations of Nb2O5. It may be explain due to their similar particle mean size and, consequently, the same volume ratio of Nb2O5 incorporated into their glass powder. However, at higher concentrations of Nb2O5 (10 wt.% and 20 wt.%), one of the commercial cements showed significant difference (p < .05) to its control group, corroborating with the Knoop microhardness test for the use of Nb2O5 5 wt.% concentration. Besides the oxide was not able to react in gelation process, the usual agglomeration of fillers can act as stress-concentrating defect sites, which decrease material properties.[Citation34] Surface hardness and resistance to acid erosion are specially related to this event due to agglomerates debond and surface wear. In the present study, for one GIC, higher amounts than 5 wt.% may have increased the chances of agglomerates formation.
Researches are focused in the improvement of bioactivity of restorative materials besides other properties.[Citation35] GICs have shown to be effective in the remineralization process of dental tissues in ART and it is possible to treat patients with this material based on the philosophy of minimally intervention dentistry. Thus, there is a decrease of the risk of accidental mechanical pulp exposure particularly in young patients.[Citation36] To improve the bioactivity of GICs, calcium phosphates are used [Citation37,Citation38] such as nanocrystals of calcium fluorapatite and hydroxyapatite [Citation39] to act as nuclei to remineralization process after the ionic saturation of the liquid medium.[Citation40] However, it was shown that hydroxyapatite is consumed during GIC setting [Citation39] and may not be available for bioactivity and the release of calcium and phosphate ions lasts a few months.[Citation37] Low concentration of Nb2O5 can be an alternative filler for this purpose, since it was observed in this study that higher amounts of Nb2O5 decreased the properties of GICs as it could not react during setting. Furthermore, it is well known that the local ion-rich alkaline environ favors apatite formation and matrix phosphorylation.[Citation40] Nb2O5 can enhance the environ pH, as it was showed when this oxide was added to bioglass,[Citation41] change the acidic environ of ionomer based materials and turn it more favorable to tooth remineralization.
Materials more easily distinguished from adjacent structures are those with radiopacity slightly higher than enamel.[Citation42] By increasing the radiopacity of the restorative material, it is possible to improve the accuracy of recurrent caries and marfinal defects diagnosis.[Citation21] Nb2O5 can enhance radiopacity values of dental materials [Citation23,Citation24] due to its higher atomic number (41) compared to the components around it, as fluorine (9), aluminum (13) and silicon (14). In this study, none of the three GICs tested without Nb2O5 reached to 2 mm of Al, corresponding less to 1 mm of enamel (p < .05) and all three GICs increased their radiopacity to 2 mm of Al (p > .05) at 20 wt.% of Nb2O5 incorporation. Nevertheless, Vitro Fil presented the best radiopacity result because it showed no statistical difference (p > .05) to 2 mm of Al at low Nb2O5 incorporation. Thus, at 5 wt.% of Nb2O5 addition, it is possible to increase Vitro Fil radiopacity similar to enamel’s value without affecting the other evaluated properties.
The development of GICs with different Nb2O5 concentrations was necessary to make it possible a better understanding about the influence of this oxide on the commercial cements. By means of the tests proposed in this study, it was possible to evaluate some of the chemical (acid erosion) and physical (surface hardness, compressive strength and radiopacity) behavior of the developed materials, which can contribute to predict the in vivo performance and to reject some of the tested concentrations of Nb2O5 addition. It was necessary to create a material with suitable properties to be future tested by biomimetic remineralization approach. The addition of 5 wt.% Nb2O5 did not affect the tested physical and chemical properties of the GICs and improved the radiopacity of one of the cements. These materials are therefore suitable for further testing of biomimetic remineralization properties.
Disclosure statement
The authors report no conflicts of interest. The authors alone are responsible for the content and writing of this article.
References
- Ana ID, Matsuya S, Ohta M, et al. Effects of added bioactive glass on the setting and mechanical properties of resin-modified glass ionomer cement. Biomaterials. 2003;24:3061–3067.
- Bertolini MJ, Zaghete MA, Gimenes R, et al. Determination of the properties of an experimental glass polyalkenoate cement prepared from niobium silicate powder containing fluoride. Dent Mater. 2008;24:124–128.
- Czarnecka B, Kruszelnicki A, Kao A, et al. Adhesion of resin-modified glass-ionomer cements may affect the integrity of tooth structure in the open sandwich technique. Dent Mater. 2014;30:e301–e305.
- Mitsuhashi A, Hanaoka K, Teranaka T. Fracture toughness of resin-modified glass ionomer restorative materials: effect of powder/liquid ratio and powder particle size reduction on fracture toughness. Dent Mater. 2003;19:747–757.
- Schwendicke F, Tu YK, Hsu LY, et al. Antibacterial effects of cavity lining: a systematic review and network meta-analysis. J Dent. 2015;43:1298–1307.
- Pinto Gdos S, Oliveira LJ, Romano AR, et al. Longevity of posterior restorations in primary teeth: results from a paediatric dental clinic. J Dent. 2014;42:1248–1254.
- Mese A, Burrow MF, Tyas MJ. Sorption and solubility of luting cements in different solutions. Dent Mater J. 2008;27:702–709.
- El-Negoly SA, El-Fallal AA, El-Sherbiny IM. A new modification for improving shear bond strength and other mechanical properties of conventional glass-ionomer restorative materials. J Adhes Dent. 2014;16:41–47.
- Kunzelmann KH, Burkle V, Bauer C. Two-body and three-body wear of glass ionomer cements. Int J Paediatr Dent. 2003;13:434–440.
- Kim DA, Abo-Mosallam HA, Lee HY, et al. Development of a novel aluminum-free glass ionomer cement based on magnesium/strontium-silicate glasses. Mater Sci Eng C Mater Biol Appl. 2014;42:665–671.
- Pamir T, Sen BH, Celik A. Mechanical and fluoride release properties of titanium tetrafluoride-added glass-ionomer cement. Dent Mater J. 2005;24:98–103.
- Simmons JJ. The miracle mixture. Glass ionomer and alloy powder. Tex Dent J. 1983;100:6–12.
- Sarkar NK. Metal–matrix interface in reinforced glass ionomers. Dent Mater. 1999;15:421–425.
- Xu X, Burgess JO. Compressive strength, fluoride release and recharge of fluoride-releasing materials. Biomaterials. 2003;24:2451–2461.
- Yap AU, Cheang PH, Chay PL. Mechanical properties of two restorative reinforced glass-ionomer cements. J Oral Rehabil. 2002;29:682–688.
- McLean JW, Gasser O. Glass-cermet cements. Quintessence Int. 1985;16:333–343.
- Osinaga PW, Grande RH, Ballester RY, et al. Zinc sulfate addition to glass-ionomer-based cements: influence on physical and antibacterial properties, zinc and fluoride release. Dent Mater. 2003;19:212–417.
- Boyd D, Towler MR. The processing, mechanical properties and bioactivity of zinc based glass ionomer cements. J Mater Sci Mater Med. 2005;16:843–850.
- Prentice LH, Tyas MJ, Burrow MF. The effect of ytterbium fluoride and barium sulphate nanoparticles on the reactivity and strength of a glass-ionomer cement. Dent Mater. 2006;22:746–751.
- Shahid S, Hassan U, Billington RW, et al. Glass ionomer cements: effect of strontium substitution on esthetics, radiopacity and fluoride release. Dent Mater. 2014;30:308–313.
- Hitij T, Fidler A. Radiopacity of dental restorative materials. Clin Oral Investig. 2013;17:1167–1177.
- Saroja S, Vijayalakshmi M, Raj B. Physical metallurgy of a Ti–5%Ta–1.8%Nb alloy. Trans Indian Inst Met. 2008;61:389–398.
- Leitune VC, Collares FM, Takimi A, et al. Niobium pentoxide as a novel filler for dental adhesive resin. J Dent. 2013;41:106–113.
- Leitune VC, Takimi A, Collares FM, et al. Niobium pentoxide as a new filler for methacrylate-based root canal sealers. Int Endod J. 2013;46:205–210.
- Karlinsey RL, Hara AT, Yi K, et al. Bioactivity of novel self-assembled crystalline Nb2O5 microstructures in simulated and human salivas. Biomed Mater. 2006;1:16–23.
- Karlinsey RL, Yi K. Self-assembly and bioactive response of a crystalline metal oxide in a simulated blood fluid. J Mater Sci Mater Med. 2008;19:1349–1354.
- Zainuddin N, Karpukhina N, Law RV, et al. Characterisation of a remineralising Glass Carbomer® ionomer cement by MAS-NMR spectroscopy. Dent Mater. 2012;28:1051–1058.
- ISO 9917-1. Dentistry – water-based cements; 2007.
- Hilgert LA, de Amorim RG, Leal SC, et al. Is high-viscosity glass-ionomer-cement a successor to amalgam for treating primary molars? Dent Mater. 2014;30:1172–1178.
- Taifour D, Frencken JE, Beiruti N, et al. Effectiveness of glass-ionomer (ART) and amalgam restorations in the deciduous dentition: results after 3 years. Caries Res. 2002;36:437–444.
- De Caluwe T, Vercruysse CW, Fraeyman S, et al. The influence of particle size and fluorine content of aluminosilicate glass on the glass ionomer cement properties. Dent Mater. 2014;30:1029–1038.
- Santos MMPR, Mathias IF, Diniz MB, et al. Evaluation of surface hardness of glass ionomer reinforced cements by carbon nanotubes. Rev Odontol UNESP. 2015;44:108–112.
- Lima RBW, Oliveira JA, Andrade AKM, et al. Influence of topical applications of fluoride of gels of the surface roughness of glass ionomer cements. Rev Bras Odontol. 2015;72:41–46.
- Belli R, Kreppel S, Petschelt A, et al. Strengthening of dental adhesives via particle reinforcement. J Mech Behav Biomed Mater. 2014;37:100–108.
- Chen S, Mestres G, Lan W, et al. Cytotoxicity of modified glass ionomer cement on odontoblast cells. J Mater Sci Mater Med. 2016;27:116.
- Ngo HC, Mount G, Mc Intyre J, et al. Chemical exchange between glass-ionomer restorations and residual carious dentin in permanent molars: an in vivo study. J Dent. 2006;34:608–613.
- Zhang L, Weir MD, Chow LC, et al. Novel rechargeable calcium phosphate dental nanocomposite. Dent Mater. 2016;32:285–293.
- Valanezhad A, Odatsu T, Udoh K, et al. Modification of resin modified glass ionomer cement by addition of bioactive glass nanoparticles. J Mater Sci Mater Med. 2016;27:3.
- Zainuddin N, Karpukhina N, Law RV, et al. Characterization of a remineralising Glass Carbomer® ionomer cement by MAS-NMR spectroscopy. Dent Mater. 2012;10:1051–1058.
- Dorozhkin SV. Calcium orthophosphates in dentistry. J Mater Sci Mater Med. 2013;24:1335–1363.
- Lopes JH, Magalhães A, Mazali IO, et al. Effect of niobium oxide on the structure and properties of melt-derived bioactive glasses. J Am Chem Soc. 2014;97:3843–3852.
- Espelid I, Tveit AB, Erickson RL, et al. Radiopacity of restorations and detection of secondary caries. Dent Mater. 1991;7:114–117.