Abstract
Objective: To investigate the repair bond strength of fresh and aged nanohybrid and hybrid composite resins using a universal adhesive (UA).
Materials and methods: Fresh and aged substrates were prepared using two nanohybrid (Venus Pearl, Heraus Kulzer; Filtek Supreme XTE, 3 M ESPE) and one hybrid (Z100, 3 M ESPE) composite resin, and randomly assigned to different surface treatments: (1) no treatment (control), (2) surface roughening with 320-grit (SR), (3) SR + UA (iBOND, Heraus Kulzer), (4) SR + Silane (Signum, Ceramic Bond I, Heraeus Kulzer) + UA, (5) SR + Sandblasting (CoJet, 3 M ESPE) + Silane + UA. After surface treatment, fresh composite resin was added to the substrates at 2 mm layer increments to a height of 5 mm, and light cured. Restored specimens were water-stored for 24 h and sectioned to obtain 1.0 × 1.0 mm beams (n = 12), and were either water-stored for 24 h at 37 °C, or water-stored for 24 h, and then thermocycled for 6000 cycles before microtensile bond strength (µTBS) testing. Data were analyzed with ANOVA and Tukey’s HSD tests (p = .05).
Results: Combined treatment of SR, sandblasting, silane and UA provided repair bond strength values comparable to the cohesive strength of each tested resin material (p < .05). Thermocycling significantly reduced the cohesive strength of the composite resins upto 65% (p < .05). Repair bond strengths of UA-treated groups were more stable under thermocycling.
Conclusions: Universal adhesive application is a reliable method for composite repair. Sandblasting and silane application slightly increases the repair strength for all substrate types.
Introduction
Improvements in restorative composite resin formulations as well as improved clinical techniques increased the predictability of composite resin restorations, making it an indispensable option both for anterior and posterior regions. Even though performance of the materials are satisfactory, maintenance procedures are required for discolorations, microleakage and partial fractures of the restorations [Citation1,Citation2]. Since every replacement would lead to larger cavity preparations, repair procedure is preferable to avoid further damage to sound dental hard tissues and reduce the overall cost of dental treatment to the public health care system [Citation1–4].
Successful repair procedure requires a durable bond between the old restoration and the new composite resin. Bonding to aged composite resin restorations can be highly challenging due to the water absorption over time and the diminished number of accessible C = C bonds to react with the new resin material [Citation4,Citation5]. The surface of old restoration serving as bonding substrate should be modified with proper treatment methods [Citation1–7]. Many techniques have been tested previously to improve the repair bond strength of composite resins including etching with phosphoric or hydrofluoric acids, tribochemical silica coating, diamond bur-roughening and silane treatment followed by the application of etch-and-rinse or self-etch adhesive resins [Citation1,Citation7,Citation7–12]. However, there are limited information regarding the use of these techniques with different type of composite resin materials.
Nanohybrid composite resins offer the advantages of durability, low polymerization shrinkage, high polishability, easy handling and superior esthetic properties due to their nano-sized filler particles and higher filler content [Citation13–15]. Previous studies reported that the repair bond strength of nanohybrid composites fell behind the cohesive strength of the original material itself [Citation7,Citation7,Citation16]. Pretreatment applications such as mechanical roughening and silane treatment followed by adhesive resin application were found to be quite efficient in the repair of microhybrid resins, however, similar improvements could not be achieved in the repair of nanohybrid composites [Citation1,Citation12]. This has been explained with the high conversion degree of the polymerized resin, limiting the number of available unreacted C = C bonds. Repair bond strength of nanohybrid composites may mainly rely on the micro-mechanical retention between old and new composite layers [Citation16].
Universal adhesives (UA) have been developed to be used in a wide variety of direct and indirect restorative applications including bonding to composite resins, dental ceramics and alloys [Citation17,Citation18]. It has been claimed that stable and reliable bond strength to enamel and dentin can be achieved in etch-and-rinse, self-etch or selective-etch modes [Citation19–21]. In principle, this would enable bonding to various surfaces without the need for dedicated and separately placed primers such as silanes, or other surface primers. Considering that defective composite restorations may contain dentin, enamel and composite borders, these adhesives may be more user-friendly in repair procedures. Thus, this study aimed to investigate the efficacy of different surface treatments and UA application on the strength and durability of repair procedures of fresh and aged nano or microhybrid composite resins.
Materials and methods
The materials used in this study and their application techniques are listed in . The schematic diagram of study protocol is shown in . A total of 300 composite resin blocks (8 mm × 8 mm × 5 mm) were prepared as bonding substrates using three different composite resins (Venus Pearl [VP]; Z100; Filtek Supreme XTE [FS]) by light-curing (Elipar S10, 3 M ESPE 1186Mw/cm2 measured with CheckMARC, Blue Light Analytics, Halifax, Nova Scotia, Canada) in silicone molds according to the manufacturers recommendations. Considering the time required for final adjustments while building up a composite resin restoration, polymerizing the last layer of resin approximately within 5 min can be regarded as reasonable, which was also previously reported to give the same bond strength values as the immediate layering [Citation22]. Therefore, for the fresh substrates, the oxygen inhibition layer was maintained, and the repair specimens were built 5 min after the polymerization of final layer. In the aged substrates, the oxygen inhibition layer was prevented by covering the top surface with a mylar strip during polymerization. After polymerization, the blocks were first water-stored at 37 °C for 48 h, boiled for 8 h in water, and again water-stored at 37 °C for 3 weeks according to the aging method described previously [Citation8]. Before aging, the substrate surfaces were wet-ground with 320-grit abrasive paper corresponding to the roughness obtained by diamond bur grinding in order to remove the superficial resin-rich layer and create standardized repair surfaces [Citation23,Citation24].
Table 1. The materials used in the study.
The fresh and aged substrates were randomly allocated to the groups according to the surface treatment: (1) no treatment (control), (2) surface roughening with 320-grit (SR), (3) SR + UA (iBOND), (4) SR + Silane (Signum, Ceramic Bond I) + UA, (5) SR + Sandblasting (CoJet Sand, 3 M ESPE) + Silane + UA. In the fresh no treatment control groups, new composite layers were directly placed over the substrate surfaces after 5 min of polymerization. For all the groups, fresh resin layers were applied incrementally up to 5 mm using the same resin with the substrate, and light-cured. Build-ups were sectioned into beams (1 mm ×1 mm ×10 mm) under water cooling by using a low-speed saw (Isomet saw, Buehler Ltd., Lake Bluff, IL) to obtain microtensile bond strength (µTBS) specimens (n = 24/group) following water storage at 37 °C for 24 h. After sectioning, the beams were randomly divided into two subgroups. The first group was water-stored for 24 h at 37 °C and tested. The second group was additionally thermocycled between 5–55 °C for 6000 cycles with a dwell time of 30 s. The µTBS was measured using a microtensile tester (Bisco Microtensile Tester; Bisco, Schaumburg, IL) at a crosshead speed of 0.5 mm/min.
The fractured specimens were gold sputter-coated and examined under scanning electron microscope (SEM) at different magnifications using Phenom ProX (Phenom-World, Eindhoven, The Netherlands) operating at 10 kV to detect the fracture types as cohesive in composite, adhesive in interface or mixed. In addition, one representative specimen from each group was polished with 180-, 320-, 600- and 1200-grit SiC papers and 1 µm (MetaDi Ultra, Buehler, Düsseldorf, Germany) 0.1 µm (MetaDi Supreme, Buehler, Düsseldorf, Germany) and 0.05 µm (MasterPrep, Buehler, Düsseldorf, Germany) polishing suspensions, respectively, and gold sputter-coated. Then, the morphological distribution of filler particles, and their Si-Zr concentration ratios were analyzed on the repair surfaces by SEM and EDS analysis.
The µTBS data were analyzed for normality (Kolmogorov–Smirnov test) and homoscedasticity (modified Levine test) and since these were not violated, a multivariate (three-way) ANOVA was used for the parameters ‘treatment type’, ‘composite resin type’ and ‘storage conditions’. Further for interactions, two-way ANOVA and Tukey Post-hoc analysis were performed. (IBM SPSS v0.23 (NY) at p = .05.)
Results
The interactions among composite resin type, surface treatment and aging procedure were found to be significant for µTBS (p < .05). Therefore, two-way ANOVA was performed. The mean µTBS of (±SD) fresh control composite samples were 79.38 (± 10.60) MPa for VP, 79.20 (± 15.09) for Z100 and 45.89 (± 10.89) MPa for FS as shown in . In the fresh groups of VP, Z100 and FS without thermocycling, the decrease in bond strength due to the repair procedure ranged between 9 and 28%, 30 and 49%, and 18 and 66%, respectively. Aging significantly decreased the cohesive strength of all the tested composite resins approximately up to 65% (p < .05). Thermocycling also reduced the cohesive strength of fresh resins; however, the difference was only significant for VP (p < .05). Compared to the fresh controls, the bond strength of the aged controls had significantly lower µTBS with and without thermocycling (p < .05).
Figure 2. Repair bond strengths and fracture analysis of fresh and aged VP groups before and after thermocycling. The same uppercase letter shows that there is no significant difference between the same substrate groups subjected to the different surface treatments. The same lowercase letter indicates no significant difference among substrate groups subjected to the same treatment technique (p >.05).
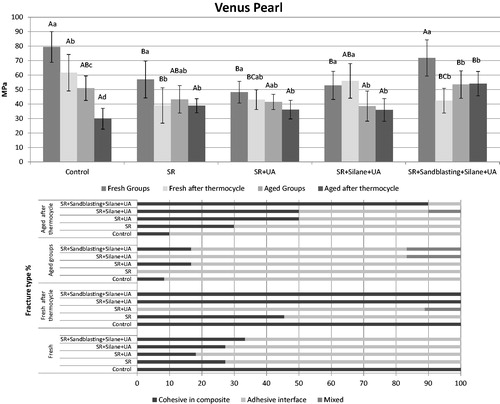
Figure 3. Repair bond strengths and fracture analysis of fresh and aged Z100 groups before and after thermocycling. The same uppercase letter shows that there is no significant difference between the same substrate groups subjected to the different surface treatments. The same lowercase letter indicates no significant difference among substrate groups subjected to the same treatment technique (p >.05).
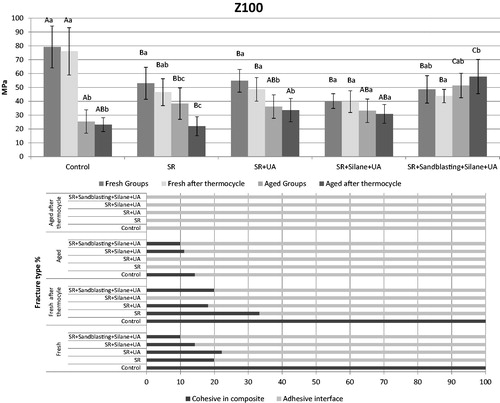
Figure 4. Repair bond strengths and fracture analysis of fresh and aged FS groups before and after thermocycling. The same uppercase letter shows that there is no significant difference between the same substrate groups subjected to the different surface treatments. The same lowercase letter indicates no significant difference among substrate groups subjected to the same treatment technique (p >.05).
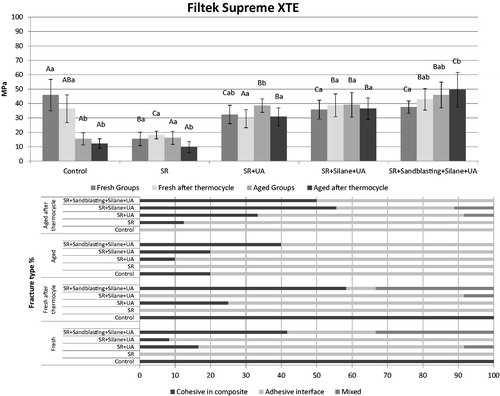
Figure 5. Representative SEM images from the fracture analysis of VP groups after µTBS testing; (a) cohesive failure in the fresh control group, (b) adhesive interface failure in the abrasive paper aged subgroup, (c) mixed failure in the SR + UA aged subgroup, (d) mixed failure in the SR + Silane + UA aged subgroup, (e) mixed failure in the SR + Sandblasting + Silane + UA aged subgroup. Resin matrix and filler distributions on the surface of (f) VP, (g) Z100, (h) FS specimens.
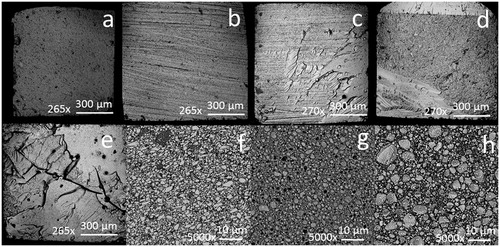
Regarding the effect of surface treatments on µTBS, SR treatment alone was not adequate to improve the repair bond strength for none of the aged + theymocycled subgroups. Multiple comparisons showed that SR + sandblasting + silane + UA provided comparable bond strength with the control. In addition, there were no significant differences between the treatments of SR + UA and SR + silane + UA (p < .05). For FS, SR + UA treatment showed significant improvement in the repair bond strength compared to the SR alone.
Representative SEM images of fractured VP specimens are shown in . It was observed that the percentage of ‘cohesive in composite’ type fractures increased when the higher repair bond strength values were achieved. Similar fracture types were also seen if the cohesive strength of the composite resin significantly dropped with aging and thermocycling. On the repaired resin surfaces, the atomic concentration ratios (%) of Si and Zr were 98.21 and 1.79 for VP, 69.25 and 30.75 for Z100, and 79 and 21 for FS, respectively.
Discussion
Longevity of composite resin repair mainly depends on the strength and durability of the repair interface. Up to date, there is no consensus regarding a standard treatment protocol of aged composite resin surface during repair procedures. Some studies have stated that macro- or micro-mechanical surface roughening of substrate surface is more important than the application of an adhesive agent [Citation9,Citation25], while the others have recommended an intermediate resin [Citation8,Citation26]. However, most clinicians prefer simpler procedures. Therefore, the feasibility of using a UA only was evaluated with or without micro-mechanical surface treatment and additional silane primer for different types of composite resins in this study.
Aging is one of the important factors limiting the longevity of adhesive restorations. During aging, water molecules absorbed by the resin structure both plasticize the polymer matrix and also diffuse into silane-treated filler-matrix interface, resulting in the hydrolysis within the resin matrix and/or at the resin-filler interface [Citation27–29]. Moreover, emerging stresses caused by the recurring thermal expansion and contractions undermine the durability of adhesive interfaces [Citation30]. Therefore, the effects of aging on both restorative resin structure and the repair interface should be taken into account while assessing the overall success of repair procedures. However, in in vitro studies, the acceleration of the aging process in composite resin structure can be challenging, the results of which could be seen after years of clinical use. Since there is currently no consensus on an aging method completely imitating the clinical conditions, the present study employed two substrate conditions (fresh and aged) and two aging regimes (testing after 24 h and after thermocycling). With the fresh groups, immediate repair procedures were simulated while the aged substrate groups subjected to thermocycling represented the long-term performance of a repaired, aged restoration. In accordance with a previous study [Citation6], the repair bond strength of the aged control groups were lower than the fresh ones in the present study. Among the fresh subgroups, the bond strengths of repair specimens, in which the oxygen inhibition layer was removed by SR, were also significantly lower than the corresponding controls. These findings show the necessity of surface conditioning techniques during resin repair procedures.
Present study investigated four different surface treatments systematically from only macro-mechanical roughening (SR) to the combination of macro- and micro-mechanical roughening (SR + sandblasting), chemical conditioning (silane) and intermediate resin application (UA). It was found that SR treatment alone did not provide any improvement in the repair bond strength of tested resin materials. When additionally combined with UA, only FS, nanofilled resin showed an increase in the repair bond strength, which was also stable after thermocycling. In comparison to SR + UA treatment, the silanization of the bonding surface did not show any significant improvement effect. On the other hand, the combined treatment of SR + sandblasting + silane + UA was found effective in improving the repair bond strength for all tested resins, almost reaching to the cohesive strength of the aged resin material itself. Hence, it can be suggested that, considering the average immediate bond strength values to enamel and dentin, such bond strength values could result in a reliable restoration that will likely to survive as long as the bonding interface to enamel and dentin stays sound.
Three different composite resins were tested in this study with variations in their resin matrix and filler compositions. Nanofilled resin FS containing mainly bis-GMA and UDMA monomers in the matrix composition showed the lowest cohesive and repair bond strength. A recent study showed that Bis-GMA- and UDMA-based resin formulations showed the highest water sorption compared to the TEGDMA-based formulations [Citation31]. Therefore, it was thought that the hydrolyzation and softening of resin matrix in FS subgroups impaired the chemical bond between the filler particles and resin matrix [Citation32]. If one assumes that the fresh composite resin either chemically or mechanically retains to the aged repair surface mainly by means of filler particles, the loose particles in hydrolyzed FS resin matrix could easily detach, which might explain the obtained low bond strength results with FS. Z100 mainly contains Bis-GMA and TEGDMA monomers with zirconia/silica fillers ranging from 3.5 to 0.01 micron in size. Therefore, it can be assumed that water sorption level of Z100 could be less than FS. Moreover, the bigger particle sizes can provide higher degree of conversion, filler percentage by weight, dynamic and static modulus of elasticity, and Vickers microhardness in comparison to FS [Citation33]. It can be also suggested that, the bigger filler particles of Z100 may provide extra retentive areas after SR treatment, thereby the new composite layer can be easily retained.
MDP monomers can chemically bond to zirconia surface [Citation34]. Considering the zirconia content of fillers in both FS and Z100, it could be assumed that MDP monomers within UA would be beneficial for the promotion of the repair bond strength by providing additional chemical bonding. Accordingly, the enhanced repair strength of the aged FS subgroups following UA application could have been attributed to such a bonding mechanism, rather than tighter mechanical interlocking. However, similar results could not be obtained with Z100, although the relative zirconia quantities of both composite resins were within the same range as detected by EDS. In line with the previous studies [Citation35,Citation36], these comparisons showed that the main role of intermediate resin application was to provide better mechanical interlocking between substrate surface and new resin layer, rather than a possible chemical interaction between MDP monomers and zirconia fillers.
Silane treatment during resin repair procedures basically aims to promote chemical bonding by forming siloxane bonds between silicate-containing filler particles exposed on the repair surface and the resin matrix of fresh resin layer. It is well-known that surface wettability is also increased, which can improve the micro-mechanical bonding in the repair interface [Citation37,Citation38]. For the repair procedure of a nanofilled resin, it has been recently reported that a silane-containing UA combined with a separate silane application and air abrasion treatment provided the highest repair bond strength, and the efficacy of the UA varied depending on the used silane type [Citation38]. In accordance with this study, the combined treatment of SR, sandblasting, silane and UA was the most efficient surface treatment method in our study.
According to the fracture type analysis, fresh controls demonstrated 100% ‘cohesive in composite’ type fractures while the predominant failure type was ‘adhesive interface’ in the aged ones. This finding indicates an adhesive interface bonding which is weaker than the cohesive strength of the material in all the aged subgroups. After thermocycling, there were shifts in the fracture types from ‘adhesive interface’ to ‘cohesive in composite’, which were most probably related with the decreasing cohesive strength of the resin materials due to aging, rather than a significant increase in the strength of adhesive interface. With the SR + sandblasting + silane + UA treatment, VP and FS showed a higher percentage of ‘cohesive in composite’ type fractures as compared to the other surface treatments. In Z100, mainly fractures occurred at the adhesive interface since the strength of the adhesive interface was not higher than the high cohesive strength of the material.
Conclusions
Within the limitations of this study, following conclusions were drawn:
For the fresh and aged nanofilled resin (FS), macro-mechanical roughening of the repair surface alone was not adequate for a durable repair bonding, and adhesive resin application was required to improve the repair bond strength.
Repair bond strength of aged nanohybrid (VP) and hybrid (Z100) resins could be improved only with SR, sandblasting, silane and adhesive resin application.
Only the combined treatment of macro- and micro-mechanical roughening, silane and UA provided repair bond strength values comparable to the cohesive strength of each tested resin.
Universal adhesive with MDP monomer content may improve the mechanical interlocking to the repair surfaces similar to conventional adhesive resins, rather than providing noticeable additional chemical bonding.
Acknowledgements
The materials used in the present study were donated by the manufacturers, and is highly appreciated.
Disclosure statement
The authors declare no conflict of interest.
Additional information
Funding
References
- Staxrud F, Tveit AB, Rukke HV, et al. Repair of defective composite restorations. A questionnaire study among dentists in the Public Dental Service in Norway. J Dent. 2016;52:50–54.
- Gordan VV, Garvan CW, Richman JS, DPBRN Collaborative Group, et al. How dentists diagnose and treat defective restorations: evidence from the dental practice-based research network. Oper Dent. 2009;34:664–673.
- Loomans BA, Cardoso MV, Roeters FJ, et al. Is there one optimal repair technique for all composites? Dent Mater. 2011;27:701–709.
- Baur V, Ilie N. Repair of dental resin-based composites. Clin Oral Investig. 2013;17:601–608.
- Eliasson ST, Tibballs J, Dahl JE. Effect of different surface treatments and adhesives on repair bond strength of resin composites after one and 12 months of storage using an improved microtensile test method. Oper Dent. 2014;39: E206–E216.
- Rinastiti M, Özcan M, Siswomihardjo W, et al. Effects of surface conditioning on repair bond strengths of non-aged and aged microhybrid, nanohybrid, and nanofilled composite resins. Clin Oral Invest. 2011;15:625–633.
- Loomans BA, Cardoso MV, Opdam NJ, et al. Surface roughness of etched composite resin in light of composite repair. J Dent. 2011;39:499–505.
- Tezvergil A, Lassila LV, Vallittu PK. Composite-composite repair bond strength: effect of different adhesion primers. J Dent. 2003;31:521–525.
- Rinastiti M, Ozcan M, Siswomihardjo W, et al. Immediate repair bond strengths of microhybrid, nanohybrid and nanofilled composites after different surface treatments. J Dent. 2010;38:29–38.
- Celik EU, Ergücü Z, Türkün LS, et al. Tensile bond strength of an aged resin composite repaired with different protocols. J Adhes Dent. 2011;13:359–366.
- da Costa TR, Serrano AM, Atman AP, et al. Durability of composite repair using different surface treatments. J Dent. 2012;40:513–521.
- Jafarzadeh Kashi TS, Erfan M, Rakhshan V, et al. An in vitro assessment of the effects of three surface treatments on repair bond strength of aged composites. Oper Dent. 2011;36:608–617.
- Turssi CP, Ferracane JL, Ferracane LL. Wear and fatigue behavior of nano-structured dental resin composites. J Biomed Mater Res Part B Appl Biomater. 2006;78:196–203.
- Jung M, Sehr K, Klimek J. Surface texture of four nanofilled and one hybrid composite after finishing. Oper Dent. 2007;32:45–52.
- Ergücü Z, Türkün LS, Aladag A. Color stability of nanocomposites polished with one-step systems. Oper Dent. 2008;33:413–420.
- Staxrud F, Dahl JE. Role of bonding agents in the repair of composite resin restorations. Eur J Oral Sci. 2011;119:316–322.
- McLean DE, Meyers EJ, Guillory VL, et al. Enamel bond strength of new universal adhesive bonding agents. Oper Dent. 2015;40:410–417.
- Rosa WL, Piva E, Silva AF. Bond strength of universal adhesives: a systematic review and meta-analysis. J Dent. 2015;43:765–776.
- Suzuki T, Takamizawa T, Barkmeier WW, et al. Influence of etching mode on enamel bond durability of universal adhesive systems. Oper Dent. 2016;41:520–530.
- Takamizawa T, Barkmeier WW, Tsujimoto A, et al. Influence of different etching modes on bond strength and fatigue strength to dentin using universal adhesive systems. Dent Mater. 2016;32:e9–e21.
- Yesilyurt C, Kusgoz A, Bayram M, et al. Initial repair bond strength of a nano-filled hybrid resin: effect of surface treatments and bonding agents. J Esthet Restor Dent. 2009;21:251–260.
- Mutlu O, Gurel P. Effect of delay in layering on the incremental adhesion of indirect dental composite resins. Int J Adhes Adhes.2012;39:15–20.
- Anusavice KJ. Philips’ science of dental materials. 10th ed. Pennsylvania: W.B. Saunders Company; 1996. p. 298–299.
- Eliasson ST, Dahl JE. Effect of curing and silanizing on composite repair bond strength using an improved micro-tensile test method. Acta Biomater Odontol Scand. 2017;3:21–29.
- Hamano N, Chiang YC, Nyamaa I, et al. Effect of different surface treatments on the repair strength of a nanofilled resin-based composite. Dent Mater J. 2011;30:537–545.
- Wendler M, Belli R, Panzer R, et al. Repair bond strength of aged resin composite after different surface and bonding treatments. Materials. 2016;9:547.
- Sideridou ID, Karabela MM, Bikiaris DN. Aging studies of light cured dimethacrylate-based dental resins and a resin composite in water or ethanol/water. Dent Mater. 2007;23:1142–1149.
- Øysaed H, Ruyter IE. Composites for use in posterior teeth: mechanical properties tested under dry and wet conditions. J Biomed Mater Res. 1986;20: 261–271.
- Tarumi H, Torii M, Tsuchitani Y. Relationship between particle size of barium glass filler and water sorption of light-cured composite resin. Dent Mater J. 1995;14:37–44.
- Imbery TA, Gray T, DeLatour F, et al. Evaluation of flexural, diametral tensile, and shear bond strength of composite repairs. Oper Dent. 2014;39: E250–E260.
- Fonseca AS, Labruna Moreira AD, de Albuquerque PP, et al. Effect of monomer type on the CC degree of conversion, water sorption and solubility, and color stability of model dental composites. Dent Mater. 2017;33:394–401.
- Tezvergil A, Lassila LV, Yli-Urpo A, et al. Repair bond strength of restorative resin composite applied to fiber-reinforced composite substrate. Acta Odontol Scand. 2004;62:51–60.
- Zhao L, Jian YT, Wang XD, et al. Bond strength of primer/cement systems to zirconia subjected to artificial aging. J Prosthet Dent. 2016;116:790–796.
- Baena E, Vignolo V, Fuentes MV, et al. Influence of repair procedure on composite-to-composite microtensile bond strength. Am J Dent. 2015;28:255–260.
- Souza MO, Leitune VC, Rodrigues SB, et al. One-year aging effects on microtensile bond strengths of composite and repairs with different surface treatments. Braz Oral Res. 2017;31:e4
- El-Askary FS, El-Banna AH, van Noort R. Immediate vs delayed repair bond strength of a nanohybrid resin composite. J Adhes Dent. 2012; 14:265–274.
- Staxrud F, Dahl JE. Silanising agents promote resin-composite repair. Int Dent J. 2015;65:311–315.
- Fornazari IA, Wille I, Meda EM, et al. Effect of surface treatment, silane, and universal adhesive on microshear bond strength of nanofilled composite repairs. Oper Dent. 2017;42:367–374.