ABSTRACT
The Egyptian water crisis has encouraged the exploration of innovative solutions for wastewater treatment. One promising approach is using treated agricultural drainage water for its large amount per year and low pollution. There are many methods used for the treatment of wastewater, but this research focused on a new one which is the use of microalgae for in-situ treatment of wastewater, due to its ability to uptake and adsorb pollutants, aerate water, and produce biomass for potential use as a biofuel or fertilizer. Microalgae have shown great potential for removing various pollutants from wastewater, including organic matter, nutrients, heavy metals, and pathogens. Moreover, the use of microalgae for wastewater treatment can be more cost-effective and sustainable than traditional wastewater treatment methods, which often require large and expensive infrastructure. This study aimed to investigate the effectiveness of using microalgae for in-situ treatment of agricultural drainage wastewater of Bahr El-Baqar drain. The results revealed that the removal efficiencies were 29% for BOD (biochemical oxygen demand), 46.9% for COD (chemical oxygen demand), 56.1% for TSS (total suspended solids), 31% for HM (Heavy Metal) removal, and 86% increment in DO (Dissolved Oxygen) concentration. This experiment provided evidence that microalgae could be a promising and cost-effective solution for in-situ treatment of agricultural drainage wastewater, with high removal efficiencies for pollutants in wastewater and the potential to reduce the need for constructing large and expensive wastewater treatment plants.
Introdution
The water scarcity issue in Egypt is becoming more severe due to the increasing population, higher standards of living, and expanding urban and industrial activities. As a result, the demand for water has surpassed the available conventional water resources such as the Nile River, groundwater, and rain precipitation, which can produce roughly 62 BCM/y of water. Therefore, non-conventional water resources are needed such as treated municipal and agricultural drainage water, seawater desalination, and brackish groundwater in the delta region (Ashour et al., Citation2009).
The strategy of agricultural drainage water reuse is considered one of the most important unconventional water resources, and it can contribute to overcoming the shortage of water problem in Egypt, saving fresh water, and improving the Physico-chemical properties of soil. It’s an economical and effective method that could produce high-quality water instead of more expensive methods such as municipal wastewater treatment or seawater desalination (AbdEllah, Citation2020).
The agriculture drains were originally made to collect irrigation drainage but due to increasing population, and seepage of sewerage facilities. These drains now carry different types of polluted water because factories and people discharge all their types of wastes in these drains. This led to several adverse impacts such as depletion of dissolved oxygen levels in drains, which may affect the aquatic life in drains. Also, self-purification ability will decrease. As a result, direct reuse of water from agricultural drains becomes very risky and requires treatment for the drains’ water to improve its quality for reuse purposes (Abd-Elhamid et al., Citation2017).
Constructing open drain systems was established at the end of the 19th century. It helped in getting rid of soil problems that were raised due to high water table and increasing salinity. A strong open drainage system was constructed in Egypt along the Nile to transfer excess water to the Mediterranean Sea and lakes. (MWRI, Citation2005) But reuse of agricultural drainage water has been threatened by many factors as discussed below:
Agricultural drains in Egypt face multiple pollutant sources, threatening the ecosystem and water quality for irrigation and crop consumption. Factors influencing water quality include irrigation method, with surface runoff carrying sediments, nutrients (especially phosphorus), and agricultural chemicals, subsurface drainage water contains dissolved salts, minerals, and fewer sediments, Pollution loads in drains have increased due to the excessive use of agrochemicals, animal feeds, and drugs to boost productivity. Factories contribute to pollution by directly discharging untreated wastewater into agricultural drains. Additionally, domestic wastewater discharge into drainage canals further adds to the organic compound content (Fleifle & Allam, Citation2016).
Problems facing the agricultural soil due to reusing agricultural wastewater are accumulation of salts in the root zone which decreases the plants’ ability to absorb water and decreases the soil permeability which decreases the water seepage into the soil, some chemical constituents of agricultural drainage water as boron, sodium, and chloride are toxic to plants, elevated amounts of carbonate and pH in irrigation water affect vegetable leaves, especially in sprinkler irrigation systems (Fleifle & Allam, Citation2016). All the mentioned factors showed that the reuse of agricultural drainage water without proper treatment would exert a serious problem.
Agricultural drainage water treatment
The reuse of agricultural drainage water for irrigation purposes is considered the most suitable and economic solution to overcome the water shortage problem all over the world. As a result, the need for agriculture drainage water treatment appeared either for reuse or to conform to regulatory disposal requirements. Many criteria should be taken into consideration when it comes to choosing a treatment procedure for agriculture drainage water. Some of these criteria are capital cost, land availability, and labor intensity whether in operation or maintenance. Presence of sufficient drain length to execute the treatment process, impact on the local population, ability to remove pollutants from water as (toxins, heavy metals, and nutrients) capability to respond to (variation in flow, pollutant loading, change in chemical properties in water as PH and salinity), the complexity of harvesting requirements, the commercial potential to produce animal feed or fuels from harvested biomass, the commercial potential for producing construction materials, biomass, and sludge disposal methods (Rashed, Citation2002).
Several scenarios in agricultural water treatment can be used according to treatment procedure and location. This research will focus on treatment inside the stream body itself as a cost-effective and easy construction method for agricultural wastewater treatment. In this method, the whole volume of drain is treated inside the stream body to enhance the level of water quality and accelerate the self-purification effect. The cost of this method is negligible, unlike the two previous methods (Ateia, Citation2016).
There are many techniques used for in-situ treatment of agricultural drainage wastewater such as Hydraulic, Mechanical, and Biological Methods.
This research will focus on the biological methods specially Bioremediation, which involves using living organisms such as aquatic weeds, floating plants, algae, fungi, and bacteria to break down organic matter, heavy metals, and toxins. This process can be carried out with minimum construction, operation, and maintenance costs, making it cost-effective (Saeed et al., Citation2022).
There are many types of wastewater such as municipal, agricultural, and industrial wastewater in the agricultural drains. Therefore, water can be highly polluted with many organic and inorganic pollutants such as microplastics, xenobiotics, heavy metals, and high concentrations of nitrates, phosphates, and carbon (C) compounds. Therefore, no single treatment could manage these various types and scales of pollutants.
Conventional WWTP works on the removal of suspended solids, and BOD by biological treatment. This leads to the breaking down of organic and inorganic pollutants such as N and P. But the degradation capacity of these conventional wastewater treatment technologies is limited especially with the treatment of heavy metals, high nutrient load, and xenobiotics. If these contaminants aren’t removed from wastewater, they will accumulate in groundwater leading to its pollution. However, using micro-algae in wastewater treatment shows promising results because they can deal with various types of pollutants in wastewater. This owes to their metabolic flexibility and their ability to do photoautotrophic, mixo-trophic, or heterotrophic metabolism. Also, biomass generated from using micro-algae in treatment can be used to produce useful products such as proteins, pigments, animal feed, fertilizers (Wollmann et al., Citation2019).
Algae-based techniques for wastewater treatment have been extensively researched since the 1950s, owing to their ability to effectively remove inorganic nitrogen and phosphorus from water. Microalgae play a pivotal role in this process by directly absorbing pollutants through adsorption or ion exchange, transforming other pollutants, and providing oxygen to bacteria for the aerobic degradation of pollutants. This feature reduces the cost of wastewater treatment compared to other methods that require artificial aeration.
However, the use of microalgae in wastewater treatment can be challenging due to the complex and variable nature of different types of wastewaters. Despite this, microalgae offer many advantages, including reducing biochemical and biological oxygen demand, removing nutrients such as N and P, eliminating harmful bacteria and heavy metals, consuming CO2 from the atmosphere, producing oxygen, generating uniform biomass, and producing high-value products. These benefits make microalgae a promising option for sustainable wastewater treatment (Castellanos-Estupiñan et al., Citation2022).
Microalgae have a wide range of species of range 200,000–800,000. Their extraordinary adaptogenic capability enables them to reproduce and increase in different environmental conditions. This makes them a perfect alternative in WWT and biofuel production (Koul et al., Citation2022).
Microalgae treatment methodology inside stream body
The removal action of microalgae for pollutants exist in wastewater is described below:
Bioadsorption
Bioadsorption is a process where compounds passively adsorb on the cell wall or extracellular polysaccharides (EPS). Many processes are involved in bioadsorption and discussed as follows: Cation/Anion Exchange where algal cell walls utilize their functional groups for efficient cation/anion exchange, enabling effective removal of heavy metals from wastewater and potential metal recovery. Absorption where microalgae absorb inorganic nitrogen and phosphorus, converting them into organic compounds for metabolism and the synthesis of essential biomolecules. Nitrogen is converted to ammonium, while phosphorus is utilized as H2PO4− and HPO4−2, undergoing phosphorylation to form organic compounds. Precipitation where algae in wastewater release organic compounds that lower pH, facilitating pollutant precipitation. Metal cations adhere to cell surfaces at higher pH, reducing their bioavailability and aiding in pollutant removal (Koul et al., Citation2022).
Bioaccumulation
Bioaccumulation, measured by the bio-concentration factor (BCF), is influenced by various factors such as chemical availability, metabolism, physical barriers, ionization, and dissolved organic matter, determining the concentration of contaminants in organisms relative to their environment (El-Sheekh et al., Citation2022).
Intracellular degradation
Contaminants enter the cell through the cell wall during bio-uptake, where they bind to intracellular proteins and other substances. Contrary to adsorption, bio-uptake of pollutants into the cell takes place over hours to days and only happens in living microalgal cells (Sutherland & Ralph, Citation2019).
Microalgae can remove organics from wastewater through biodegradation, consumption, and biosorption mechanisms. The cell wall of microalgae provides sorption zones for organic contaminants. Some microalgae can use organics in a mixotrophic or heterotrophic manner, allowing for the treatment of organic-rich streams (Al-Jabri et al., Citation2021).
Microalgae can work with bacteria or fungi to degrade organic materials in wastewater. Microalgae produce oxygen through photosynthesis that bacteria use to break down organics, while microalgae use the produced CO2 for growth. Bacteria also produce valuable substances and can regenerate nutrients for microalgae (Al-Jabri et al., Citation2021).
In 2010, HydroMentia made an algal turf scrubber. It is a flow-way system that utilizes periphytic algae to fix excess nutrients and CO2 from treated wastewater (Wollmann et al., Citation2019).
In 2015, Mohd et al. conducted an experiment using Chlorella species in the bioremediation of aquaculture wastewater. The experiment showed good results in the efficiency of Chlorella in removing nutrients, with an optimal inoculation rate of 30% (v/v). The removal of N-NH4 was 98.5% and p-PO4 was 92.24% (Mohd et al., Citation2015).
In 2016, a US company developed a low-cost, floating offshore photo bioreactor that utilizes natural light and CO2. It demonstrated high removal efficiency in wastewater treatment, with 75% removal of total N, 93% removal of total P, and 93% removal of BOD (Wollmann et al., Citation2019).
In 2017, research was carried out to cultivate microalgae in raw institutional effluent for wastewater treatment. Scenedesmus sp. and Chlorella sp. were isolated from Kallar Kahar Lake in Pakistan and were used. Microalgae cells were injected into a wastewater sample in a mixotrophic mode. By the seventh day of operation, the pollutants in the wastewater had been effectively eliminated from the original sample. Scenedesmus sp. was used to eliminate COD 95%, nitrate 99.7%, and phosphate 80.5%. Meanwhile, Chlorella sp. decreased COD by 84.86%, nitrate by 98.2%, and phosphate by 70% (Ansari et al., Citation2017).
In 2017, a study was done by Dr Mohamed Elhosseiny and Dr Omar Elhosseiny using algae ponds for the desalination of the agriculture drainage water of Baloza drain to be suitable for reuse in irrigation of crops or for applying in fish cultivation ponds. The solution succeeded to decrease the water salinity by 70% that lower its TDS than 2000 ppm which is suitable for the two targeted purposes (El Nadi et al., Citation2017).
Aim of study
This study was conducted to develop a new technology for the instream treatment of agricultural wastewater using a cost-effective, easy to construct, requires minimal maintenance and operation expenses, with the advantage of minimum pollution to the surrounding environment, and production of valuable by-products using a layer of Scenedesmus Obliquus algae species.
Materials and methods
The study was conducted near Bahr El-Baqar drain on a pilot-scale plant built by the EU research project named “drains water quality enhancement.” The pilot was placed nearby the drain at Faqous Wastewater Treatment Plant, Sharkia Governorate, Egypt. The pilot was operated under the natural conditions of temperature, sunlight, and humidity according to the study period. The applied pilot is shown in (Ali et al., Citation2020)
.Pilot description
The pilot consisted of two parallel channels that simulate the drain. These channels were of dimensions 40 cm width, 70 cm depth, and length of 10 m. They were fed and drained by two crossing channels one at the beginning and the second at the end of the five channels each with 70 cm depth and 1 m width and have a feeding pipe of diameter 4-inch from a submersible pump of 10 l/s and head 10 m erected inside the drain, and a disposal pipe of diameter 6-inch at its ends to return water back to the drain.
The applied biomass as a treatment procedure was placed in channel 2 after 50 cm from its beginning with average thicknesses 10 mm (7–12.5 mm) and length 50 cm that was fixed by PVC plates, leaving channel 1 without any treatment media to simulate the drain. A plan, sectional elevation, and side view for the pilot plant unit are shown in . The microalgae used in the study was the species Scenedesmus Obliquus that grew naturally in open stream canals and drains. The choice of these species owes to their ability to effectively remove contaminants from wastewater (Ansari et al., Citation2017; Castellanos-Estupiñan et al., Citation2022; Moustafa et al., Citation2023).
Operation procedure
The operation procedures for the practical work were made as follows:
The submersible pump was operated to deliver raw wastewater from the drain to the pilot.
The outlet valve was closed until the pilot was filled with water and then opened for continuous flow.
Before starting the treatment runs, one preparation week was made to allow for the establishment of favorable growth conditions, adaptation of the microalgae to the system, and the development of a stable and functional algal biomass for subsequent treatment runs, ensuring the biomass stability with the flow, and the continuity of operation.
After the one-week preparation, a run was made which spent six weeks in its operation to assess the effectiveness of the system. This duration allowed for a thorough evaluation of the treatment process and its ability to treat the wastewater over an extended period.
Samples were taken from the inlet crossing channel, after 0.5 m from algae layer, from the middle of the two longitudinal channels, and at the disposing crossing channel at three times daily rate for six weeks of run period. The daily average value was used to represent the current drain’s water quality criteria.
Measured parameters were DO, BOD, COD, TSS, and HM as they are considered fundamental parameters that serve as baseline indicators for wastewater treatment. By focusing on these indicators, we can assess the initial efficacy of the treatment approach in reducing organic matter and solids, which are often the primary concerns in wastewater treatment processes.
(DO is the dissolved oxygen in water and is measured by the digital dissolved oxygen meter made by AMTAST in USA, Model no. AMT08, BOD is typically quantified as the quantity of oxygen consumed by aerobic bacteria during the oxidation process of biodegradable organic substances, under aerobic conditions and at a temperature of 20°C, over a period of five days. The measurement of BOD was performed using a BOD measuring instrument, following the standardized method for analyzing water and wastewater samples, (Standard Methods Committee of the American Public Health Association,1966) COD represents the quantity of oxygen consumed during the chemical oxidation process of all organic compounds in wastewater, regardless of their biodegradability, utilizing a powerful oxidizing agent over a duration of three hours, at a temperature of 200°C. The measurement of COD was conducted in accordance with the standardized method for analyzing water and wastewater samples (Standard Methods Committee of the American Public Health Association, Citation2023), TSS refers to particles in water that are larger than 2 microns. These particles consist mainly of inorganic materials, but they can also include algae and bacteria. TSS measurement was conducted following the standard method for water and wastewater analysis. The process involved filtering the water sample through filter paper using a filtration apparatus and suction flask. The filter paper was then dried in an oven at 103°C to 105°C for one hour, cooled in a desiccator, and weighed using an analytical balance. The difference in weight before and after drying represents the TSS content in the sample (APHA-AWWA-WPCF, Citation1998), and HM is the heavy metal concentration in water, and were measured by a spectrophotometer Model No. 19 made by Cole-Palmar co. USA).
Results and discussion
Microalgae showed excellent innovation in the removal of pollutants such as BOD, COD, &TSS, HM from wastewater with very low cost and high removal efficiency as mentioned before (Koul et al., Citation2022). (El-Sheekh et al., Citation2022) (Al-Jabri et al., Citation2021)
Do removal results discussion
The average values of DO daily measurements and the increasing ratios of DO compared to channel 1 are illustrated in and .
Figure 3. Average DO concentrations along the two channels.
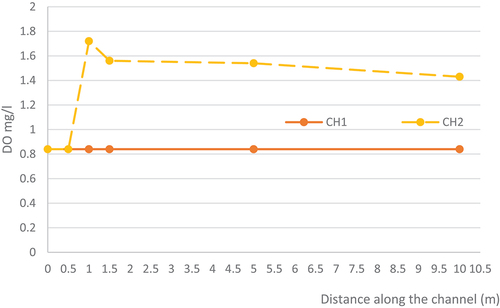
Table 1. Average DO results and increasing ratios.
In the buffer channel, DO concentration remained constant due to insufficient water velocity inside the channel which is needed for effective air-water mixing, which would increase the DO concentration. Additionally, the DO concentration didn’t decrease as it didn’t reach the necessary threshold to initiate bacterial oxidation activity (Šaulys et al., Citation2020).
DO concentration reached its peak in channel two immediately after the microalgae layer. This phenomenon can be attributed to the photosynthetic activity of the microalgae, which resulted in the production of oxygen and subsequently elevated the DO concentration compared to the buffer channel (Sánchez Zurano et al., Citation2021).
After 0.5 m from the microalgae layer, DO concentration began to decrease due to the consumption of DO by bacteria during the oxidation process. However, the DO concentration remained significantly higher compared to the buffer channel with an increase of 86% above the buffer channel.
After 5&10 m from the beginning of the channel, the DO concentration continued to decrease as it was consumed by bacteria in its oxidation action with noticing that it was higher than the buffer channel by 83%, and 70%.
BOD Removal results discussion
The average values of BOD daily measurements and the removal ratios of BOD compared with channel one are illustrated in and .
Table 2. Average BOD results and removal ratios.
The constant concentration of BOD in channel one along its 10-m length can be attributed to the limited availability of dissolved oxygen (DO) within the channel. The low DO concentration hinders bacterial activity in degrading organic matter. The self-purification process, which relies on bacterial action, requires a significant distance to occur effectively. This distance allows for an increase in DO through water velocity and air friction, facilitating the bacterial activity necessary for self-purification (Šaulys et al., Citation2020).
After the microalgae layer, the concentration of BOD decreased by 29%, this reduction can be attributed to the presence of an adequate concentration of dissolved oxygen (DO) resulting from the photosynthetic activity of microalgae. And this agreed with the study that proved the success of microalgae in the removal of 51% of BOD from wastewater (Marella et al., Citation2018). The increased DO levels activated the bacteria, initiating their oxidation process. The high availability of DO facilitates efficient degradation between the bacteria and organic matter, leading to BOD removal within the channel. However, this removal was at declining rates. At the middle and the end of the channel, the water velocity was insufficient to replenish the consumed DO. As a result, the degradation process weakened, causing BOD concentrations to decrease by 39% and 45%, respectively, when compared to channel 1. Once the DO concentration decreased to match that of the influent, the bacterial degradation will return to be very slow as happened in the buffer channel (Kumar, R., Citation2023)
COD Removal results discussion
The average values of COD daily measurements and the removal percentages of COD in channel 2 compared with channel 1 are illustrated in and .
Table 3. Average COD results and removal ratios.
In the buffer channel, the concentration of COD remained relatively constant. This can be attributed to the insufficient concentration of dissolved oxygen (DO) necessary to initiate the oxidation process. The short length of the pilot channel, which is only 10 m, was inadequate for the self-purification process to occur. This process relies on the increase of DO concentration in water through water velocity and friction with air, which facilitates oxidation and COD removal. However, due to the limited channel length, these conditions were not met, resulting in a consistent COD concentration in the buffer channel (Šaulys et al., Citation2020).
After the layer of microalgae, a noticeable improvement in the removal ratio was observed, with a decrease of 46.9% in COD concentration. And this agreed with the study that proved the success of microalgae in the removal of 91% of COD from wastewater (Marella et al., Citation2018). The removal of COD continued within the channel following the microalgae layer, as there was sufficient dissolved oxygen (DO) available for the oxidation of both organic and inorganic substances. This increased the COD removal ratios, but with declining rates. This is primarily due to the high production of oxygen by the microalgae near the layer, which elevates the DO concentration in the water. Consequently, the oxidation of organic and inorganic substances is facilitated by the presence of sufficient DO. However, as the distance from the microalgae layer increased, the DO concentration decreased, leading to a decrease in the oxidation rate. Specifically, the oxidation rate decreased by 49.5% after 5 m and 51.3% after 10 m, compared to channel 1. It is expected that the oxidation rate will continue to decrease until it becomes linear similar to the buffer channel, where the DO concentration matches that of the influent.
TSS Removal results discussion
The average values of TSS daily measurements and the removal percentages of TSS in channel two compared with channel one are illustrated in and .
Table 4. Average TSS results and removal ratios.
TSS removal efficiency depends on the oxidation reaction that results in settleable substances, and as the rate of oxidation action increases this will result in more settleable substances which settle under the effect of gravity in a hindered settling way that sweeps and blocks small suspended solids and forces them to settle-down (Ugya et al., Citation2021).
TSS removal in channel one almost remained constant as no oxidation process happened due to the insufficiency of DO concentration so no settleable matters were formed and due to the short distance of the pilot’s channel the time for precipitating the existing suspended solids was too short (Šaulys et al., Citation2020; Ugya et al., Citation2021).
After the microalgae layer, the removal ratio reached 56.1% as the oxidation action was high, as shown in BOD & COD removal which resulted in the formation of a large amount of settleable matter. TSS removal continued inside the channel away from the microalgae layer but with declining rates until it reached 61.2% after 5 m and 63.5% after 10 m. This happened because the remaining DO concentration continued to decrease and that was obvious in decreasing the rate of BOD and COD removal along the length of the channels resulted in the precipitation of less produced settleable matter.
HM Removal results discussion
The average values of HM daily measurements and the removal percentages of HM in channel two compared with channel one are illustrated in and .
Table 5. Average HM results and removal ratios.
The concentration of HM decreases along the length of the channel as they were oxidized by DO and adsorbed to the surface of the microalgae layer or absorbed inside its cell wall, so it decreases in each sampling location more than the previous one (N. Kumar et al., Citation2020).
The HM concentration remained constant in the buffer channel as the DO concentration wasn’t enough to oxidize the existing HM, and due to the short length of the channel which wasn’t enough for self-purification action to take place with the absence of any source of aeration, in addition to absence of the microalgae adsorbent layer.
Just after the microalgae layer. The removal of HM reached its peak, due to the photosynthetic activity of the microalgae which produce oxygen which raises the DO level that helped in the oxidation of the existing HM. This agrees with many experiments that proved the efficiency of microalgae in the removal of HM from wastewater (Agamy et al., Citation2020; Villanueva et al., Citation2013).
After 0.5 m from the microalgae layer, the concentration of HM continued to decrease due to the effect of oxidation action and by adsorption to the surface of the microalgae layer with removal ratio 31%.
At 5&10 m from the beginning of the channel, the removal of HM continued but with declining rates due to decreasing the available DO for oxidation that was consumed by bacteria in its oxidation action and used in the oxidation of HM. The removal ratios for HM were 70%, and 78% respectively.
Conclusion
The study’s main target was to ensure the suitability to apply microalgae for treating wastewater inside the agricultural drain and its possibility to improve the drain water quality to meet reuse purposes.
The findings of this study offer valuable insights into the potential of microalgae for treating agricultural drainage wastewater. It demonstrates the efficiency of this approach in pollutant removal.
First, the concentration of DO increase just after the microalgae layer due to the photosynthetic activity of the microalgae layer, and it decreased along the length of the channel as a result of bacterial activity with the absence of high-water velocity to replenish the consumed DO.
As evidenced the BOD was reduced by 29%. This reduction can be attributed to the microalgae’s ability to convert organic compounds into less harmful or completely mineralized substances, while also providing oxygen to support bacterial oxidation of biodegradable compounds which continued after the microalgae layer raising the BOD removal to 45% at the channel end after 9 m from microalgae layer end.
Similarly, COD reduction was clear after the microalgae layer by 46.9% and increased along the channel with bacterial action to 51.3%.
The TSS decreased after microalgae layer by 56.1% which can be attributed to the oxidation process that resulted in the formation of settleable and stable particles, leading to a decrease in TSS levels with the rising in their removal ratio till channel end up to 63.5%.
The HM decreased after the microalgae layer by 31% which can be attributed to the adsorption action on the surface of microalgae in addition to the oxidation of the HM as a result of elevating the DO level due to the photosynthetic activity of the microalgae layer, and it continued to decrease at the end of the channel by 78%.
The promising results encourage the need for more research for this application. Also depending on the study results, the repeat of microalgae layers at interval distances could determine the optimum treatment results with very low-cost solution and minimal environmental hazards, also raising the possibility to reuse the cultivated microalgae in several industries as fertilizers, animal food and pharmaceutical industries.
Authors’ contributions
AMM (Aya Moustafa Moustafa): participate by 25% by doing the experimental work of this research.
MH (Mohamed El-Hosseiny): participate by 5% by suggesting research ideas and general supervision.
AMN (Amira Mohamed Nagy): participate by 35% by analysis of results and writing and editing of the manuscript.
MM (Mahmoud Mohamed): participate by 35% by analysis of results and revising this manuscript.
All authors read and approved the final manuscript.
Availability of data and material
Enquiries about data availability should be directed to the authors.
Contribution
What does this article add to previous knowledge in this subject area?
1- previous researches focused on using agricultural wastes in the treatment of agricultural drainage wastewater
2- The novelty of this work is that microalgae (living organism) was experimented in the treatment of agricultural drainage wastewater. The utilization of microalgae was based on their natural ability to restore the surrounding environment to its original state in order to ensure their own survival and it proved its ability in the removal of pollutants such as BOD, COD, & TSS, HM
As a corresponding author I affirm the submission of the author agreement form. I assert that all co- authors agreed to and signed the document.
Signature (Aya Moustafa)
Submission date 1 June 2024.
Acknowledgments
I would like to acknowledge the following individuals and organizations for their contributions to this research project. I would like to express my deepest gratitude to my research supervisors, Dr Mohamed, Dr Amira, and Dr Mahmoud for their guidance, support, and encouragement throughout this study.
Disclosure statement
No potential conflict of interest was reported by the author(s).
Additional information
Funding
References
- Abd-Elhamid, H., El-Gohary, E., & Elnag, Z. A. (2017). Safe reuse of treated wastewater for agriculture in Egypt. Conference: Exceed-Swindon Expert Workshop, Water Efficient Cities, Morocco.
- AbdEllah, R. G. (2020). Water resources in Egypt and their challenges, lake Nasser case study. The Egyptian Journal of Aquatic Research, 46(1), 1–12. https://doi.org/10.1016/j.ejar.2020.03.001
- Agamy, S., El Saadi, A., & Galal, M. M. (2020). Application of algae to free surface wetlands for effluent reuse. Water and Environment Journal, 35(2), 748–758. https://doi.org/10.1111/wej.12667
- Ali, S. S., El-Nadi, M. H., Mohamed, S. R., & Hassan, N. A. (2020). Simple treatment of agricultural drains wastewater [ M.Sc. Thesis]. Faculty of Engineering. AIN SHAMS UNIVERSITY.
- Al-Jabri, H., Das, P., Khan, S., Thaher, M., & AbdulQuadir, M. (2021). Treatment of wastewaters by microalgae and the potential applications of the produced biomass—a review. Water, 13(1), 27. https://doi.org/10.3390/w13010027
- American Public Health Association American Water Works Association, Water Environment Federation,(1998)Standard methods for the examination of water and wastewaterAndrew D. Eaton, Lenore S. Clesceri, Arnold E. Greenberg19thAmerican Public Health Association
- Ansari, A. A., Khoja, A. H., Nawar, A., Qayyum, M., & Ali, E. (2017). Wastewater treatment by local microalgae strains for CO2 sequestration and biofuel production. Applied Water Science, 7(7), 4151–4158. https://doi.org/10.1007/s13201-017-0574-9
- Ashour, M., El Attar, A., S, T., & Rafaat, Y. M. (2009). Water resources management in Egypt. Journal of Engineering Sciences, Assiut University, 37(2), 269–279. https://doi.org/10.21608/jesaun.2009.121215
- Ateia, M. (2016). In-situ biological water treatment technologies for environmental remediation: a review. Journal of Bioremediation & Biodegradation, 7(3), 1–5. ht tps://1 0.4172/2155-6199.1000348
- Castellanos-Estupiñan, M. A., Carrillo-Botello, A. M., Rozo-Granados, L. S., Becerra-Moreno, D., García-Martínez, J. B., Urbina-Suarez, N. A., López Barrera, G. L., Barajas-Solano, A. F., Bryan, S. J., & Zuorro, A. (2022). Removal of nutrients and pesticides from agricultural runoff using microalgae and cyanobacteria. Water, 14(4), 558. https://doi.org/10.3390/w14040558
- El Nadi, M. H., El Hosseiny, O. M., & Ashmawy, H. H. A. (2017). Treat agricultural drain water for reuse needs using algae ponds. Journal of Applied Science and Research, 5(6), 6–14.
- El-Sheekh, M., El-Dalatony, M. M., Thakur, N., Zheng, Y., & Salama, E. S. (2022). Role of microalgae and cyanobacteria in wastewater treatment: Genetic engineering and omics approaches. International Journal of Environmental Science and Technology, 19(3), 2173–2194. https://doi.org/10.1007/s13762-021-03270-w
- Fleifle, A., & Allam, A. (2016). Remediation of agricultural drainage water for sustainable reuse. In A. Negm (Ed.), The Nile Delta. The handbook of environmental chemistry (Vol. 55). Springer 297–324.
- Koul, B., Sharma, K., & Shah, M. P. (2022). Phycoremediation: A sustainable alternative in wastewater treatment (WWT) regime. Environmental Technology & Innovation, 25(1), 1092–1105. https://doi.org/10.1016/j.eti.2021.102040
- Kumar, N., Hans, S., Verma Srivastava, R., & Srivastava, A. (2020). Acclimatization of microalgae Arthrospira platensis for treatment of heavy metals in Yamuna River. Water Science & Engineering, 13(3), 214–222, ISSN 1674-2370. https://doi.org/10.1016/j.wse.2020.09.005
- Kumar, R. (2023). Self-purification of natural streams | sewage treatment |waste management. Environmental pollution. https://www.environmentalpollution.in/sewage-treatment/self-purification-of-natural-streams-sewage-treatment-waste-management/5310
- Marella, T. K., Parine, N. R., & Tiwari, A. (2018). Potential of diatom consortium developed by nutrient enrichment for biodiesel production and simultaneous nutrient removal from waste water. Saudi Journal of Biological Sciences, 25(4), 704–709. https://doi.org/10.1016/j.sjbs.2017.05.011
- Mohd, N. N., Abu, B. N. S., Lananan, F., Abdul, H. S. H., Lam, S. S., & Jusoh, A. (2015). Treatment of African catfish, carias gariepinus wastewater utilizing phytoremediation of microalgae, Chlorella sp. with aspergillus niger bio-harvesting. Bioresource Technology, 190, 492–498. https://doi.org/10.1016/j.biortech.2015.03.023
- Moustafa, A. M., El Nadi, M. E., Abdelmomen, M. M., & Nagy, A. M. (2023). Impact of microalgae layer thickness on the treatment performance of drain water. Scientific Reports, 13(1), 20785. https://doi.org/10.1038/s41598-023-48129-x
- MWRI. (2005). National water resources plan for Egypt 2017 Ministry of water resources and irrigation. http://extwprlegs1.fao.org/docs/pdf/egy147082.pdf
- Rashed, A. (2002). Biological studies on the snail intermediate hosts of schistosomiasis with a special emphasis on using larval echinostomes as biocontrol agent against larval schistosomes and snails. Journal of the Egyptian Society of Parasitology, 32(3), 775–784. https://doi.org/10.13140/RG.2.1.2483.3368
- Saeed, M., Hussain, U., Sumrin, N., Shahbaz, A., Noor, A., Bilal, S., Aleya, M., & Iqbal, L. (2022). Microbial bioremediation strategies with wastewater treatment potentialities – a review. Science of the Total Environment, 818, 151754. https://doi.org/10.1016/j.scitotenv.2021.151754
- Sánchez Zurano, A., Gómez Serrano, C., Acién-Fernández, F. G., Fernández-Sevilla, J. M., & Molina-Grima, E. (2021). Modeling of photosynthesis and respiration rate for microalgae–bacteria consortia. Biotechnology and Bioengineering, 118(2), 952–962. https://doi.org/10.1002/bit.27625
- Šaulys, V., Survilė, O., & Stankevičienė, R. (2020). An assessment of self-purification in streams. Water, 12(1), 87. https://doi.org/10.3390/w12010087
- Standard Methods Committee of the American Public Health Association, American Water Works Association, and Water Environment Federation. (1966). 5210 biochemical oxygen demand (BOD). In W. Lipps, T. Baxter, E. Braun-Howland (Eds.), Standard methods for the examination of water and wastewater. APHA Press. https://doi.org/10.2105/SMWW.2882.102
- Standard Methods Committee of the American Public Health Association, American Water Works Association, and Water Environment Federation. (2023). 5220 chemical oxygen demand (COD). In W. Lipps, T. Baxter, E. Braun-Howland (Eds.), Standard methods for the examination of water and wastewater. APHA Press. https://doi.org/10.2105/SMWW.2882.103
- Sutherland, D. L., & Ralph, P. J. (2019). Microalgal bioremediation of emerging contamin ants - opportunities and challenges. Water Research, 164, 114921. article no.114921. https://doi.org/10.1016/j.watres.2019.114921
- Ugya, A. Y., Ajibade, F. O., & Hua, X. (2021). ‘The efficiency of microalgae biofilm in the phycoremediation of water from river kaduna’. Journal of Environmental Management, 295, 113109. ISSN 0301-4797. https://doi.org/10.1016/j.jenvman.2021.113109
- Villanueva, R. C., Horcasitas, M., Del, C. M., & Vela, H. G. P. (2013). Las microalgas en la biorremediación acuática: Una alternativa biotecnológica. In F.-C. Alarcón (Ed.), Biorremediación de suelos y aguas (Trillas Publishing Company S.A. de).
- Wollmann, F., Dietze, S., Ackermann, J., Bley, T., Walther, T., Steingroewer, J., & Krujatz, F. (2019). Microalgae wastewater treatment: Biological and technological approaches. Engineering in Life Sciences, 19(12), 860–871. https://doi.org/10.1002/elsc.201900071