Abstract
In metazoans, stem cells in developing and adult tissues can divide asymmetrically to give rise to a daughter that differentiates and a daughter that retains the progenitor fate. Although the short-lived nematode C. elegans does not possess adult somatic stem cells, the lateral hypodermal seam cells behave in a similar manner: they divide once per larval stage to generate an anterior daughter that adopts a non-dividing differentiated fate and a posterior daughter that retains the seam fate and the ability to divide further. Wnt signaling pathway is known to regulate the asymmetry of these divisions and maintain the progenitor cell fate in one daughter, but how activation of the Wnt pathway accomplished this was unknown. We describe here our recent work that identified the GATA transcription factor EGL-18 as a downstream target of Wnt signaling necessary for maintenance of a progenitor population of larval seam cells. EGL-18 was previously shown to act in the initial specification of the seam cells in the embryo. Thus the acquisition of a Wnt-responsive cis-regulatory module allows an embryonic fate specification factor to be reutilized later in life downstream of a different regulator (Wnt signaling) to maintain a progenitor cell population. These results support the use of seam cell development in C. elegans as a simple model system for studying stem and progenitor cell biology.
Abbreviations
WBC | = | Wnt/BAR-1 canonical |
WBA | = | Wnt/β-catenin asymmetry |
VPC | = | vulval precursor cell |
β-Catenin Dependent Wnt Signaling in C. elegans
The β-catenin dependent Wnt signaling pathway is one of a core set of extracellular signaling pathways that are used repeatedly throughout the course of metazoan development.Citation1-3 The nematode C. elegans employs Wnt signaling throughout its development, however in this model organism there are 2 β-catenin dependent Wnt signaling pathways. The C. elegans Wnt/BAR-1 canonical (WBC) and Wnt/β-catenin asymmetry (WBA) pathways both culminate in β-catenin dependent transcriptional regulation of target genes, however the manner by which pathway activation accomplishes this differs.Citation4,5 The WBC pathway is activated in the same manner as the ‘canonical’ Wnt signaling pathway in vertebrates; in the absence of Wnt signal the transcription factor β-catenin is subjected to proteasomal degradation, but in the presence of ligand β-catenin is stabilized and forms a complex with a TCF family member to activate target gene expression. The WBA pathway is a nematode specific variant that regulates many asymmetric divisions in C. elegans and its hallmark is the asymmetric distribution of Wnt pathway components in the mother cell and daughter cells of an asymmetric division. In such divisions, in the daughter cell displaying Wnt pathway activation, a kinase cascade causes export of the TCF homolog POP-1 from the nucleus, lowering its level to facilitate formation of a complex with β-catenin which activates target gene expression, causing the Wnt signaled daughter cell to adopt a specific fate. The non-Wnt responding daughter cell continues to have high nuclear POP-1 levels, repressing target gene expression and driving adoption of a fate different from its sister.
Wnt Signaling and the Stem Cell-like Division of the Hypodermal Seam Cells
One process regulated by the WBA pathway is the asymmetric division of the hypodermal seam cells during larval development. The hypodermis of the worm is the single cell layer thick skin, which serves both structural and protective functions.Citation6,7 For example, the hypodermal cells secrete the cuticle, the collagen-rich outer layer of the worm that is resynthesized before each molt.Citation7,8 The seam cells are specialized hypodermal cells arrayed in a single row on both sides of the worm from anterior to the posteriorCitation6 (). Ten seam cells per side are born and specified during embryogenesis but do not divide. Then, during each of the 4 larval stages most seam cells divide in an asymmetric manner to generate a posterior daughter that retains the seam cell fate and the ability to divide, and an anterior daughter that terminally differentiates, in most cases generating a hypodermal cell that fuses with the surrounding skin syncytiumCitation6,9 (). These reiterated asymmetric stem cell-like divisions allow seam cell self renewal while contributing numerous cells to the growing skin of the animal during larval life. After their final division in the mid L4 stage all of the seam cells exit the cell cycle, terminally differentiate, and fuse to form a single long cell that secretes a specialized cuticular structure called the adult alae (). Wnt signaling was first identified to regulate fate specification during the division of individual seam cells; subsequently the WBA pathway was shown to function in the asymmetric divisions of most seam cells during larval life.Citation10-13 For example, we showed that reduction of Wnt pathway activity causes the posterior daughter to adopt the fate of its anterior hypodermal sister thus decreasing terminal seam cell number, while over activation of the Wnt pathway causes both daughters of a seam cell division to adopt the seam fate resulting in too many seam cells 11(). This and earlier results therefore indicate that Wnt signaling is not necessary for seam cell division per se, but is required for the asymmetric nature of the daughter cell fates.
Figure 1. Seam cell division pattern. (A) Diagram of a newly hatched L1 hermaphrodite larva showing the position of 10 seam cells along the lateral midline on the left side (anterior is left, dorsal is up). (B) Diagram showing the division pattern of a representative ‘V’ class seam cell. The seam cell is specified in the embryo but does not divide. During each larval stage the seam cell divides once along the anterior-posterior axis to generate an anterior daughter that differentiates (usually as a hypodermal cell ‘H’ that joins the syncytial hypodermis) and a posterior daughter that retains the seam cell fate and the ability to divide further (blue lineage). In the early L2 stage, the seam cell divides once in a symmetric manner to generate 2 seam daughters that subsequently only divide asymmetrically. After their division in the L4 stage, the seam cells themselves exit the cell cycle and fuse homotypically to form a long syncytial seam cell that secretes the adult cuticle alae (blue lines).
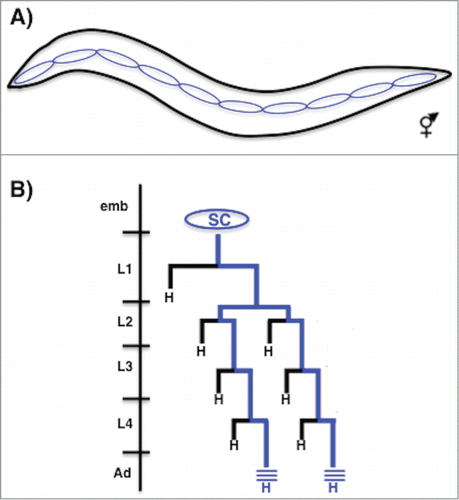
Although the short lived C. elegans does not possess adult somatic stem cells, the behavior of the seam cells during larval development is reminiscent of the behavior of progenitor cells in adult tissues of vertebrates. For example, during larval life, the seam cells are committed to the hypodermal fate but have not terminally differentiated, they have the ability to divide multiple times to generate differentiated hypodermal and neuronal cell types, and during wild type development they exit the cell cycle and terminally differentiate after a finite number of cell divisions.Citation9 Further, Wnt pathway signaling is responsible for the maintenance of the progenitor-like cell fate during the asymmetric divisions of the seam cells, and Wnt signaling is known to regulate the behavior of progenitor cells in vertebrate tissues.Citation14,15 Therefore understanding the genetic architecture regulating the asymmetric cell division of the C. elegans larval seam cells could shed light on questions fundamental to metazoan development and homeostasis.Citation9 Knowing this, a key question becomes what target genes are activated by Wnt signaling to allow the C. elegans larval seam cells to behave in a manner similar to stem and progenitor cells in adult somatic tissues of larger metazoans?
Identifying Wnt Pathway Target Genes in C. elegans
Compared to our knowledge in vertebrates, very little is known about Wnt pathway target genes in C. elegans.Citation16,17 Several approaches can be taken to address the question of target gene identity. For example, molecular and genetic techniques can identify Wnt target genes that give a phenotype similar to Wnt pathway mutants for a given process; this is the manner in which most known C. elegans Wnt pathway targets were identified.Citation18-26 Knowing the preferred binding site of a transcription factor allows use of bioinformatics approaches to identify possible target genes. However, since the POP-1 consensus binding site is predicted to be present roughly once per kilobase in the genome,Citation16 the near ubiquity of POP-1/TCF binding sites in the C. elegans genome makes de novo bioinformatics approaches difficult, however this approach has recently been used to identify Wnt regulated genes.Citation27 Chromatin immunoprecipitation efforts with POP-1 have also been hampered by the ubiquity of binding sites and by sickness caused by transgenic arrays carrying GFP-tagged POP-1 (K. Thompson and D. Eisenmann, unpublished results). As an alternative approach we previously used conditional activation of Wnt signaling and microarray analysis to identify transcripts upregulated in response to Wnt pathway activation in vivo.Citation16 In this technique, the heat shock promoter was used to express a stabilized gain-of-function variant of the C. elegans β-catenin BAR-1 that can cause a Wnt gain-of-function phenotype in both vulval development and seam cell division.Citation11,28 Jackson et al subjected synchronized populations of animals to a heat shock at a defined time in larval life and compared transcription profiles from worms subjected to Wnt pathway activation to those given only the heat shock and used microarray analysis to identify over 100 genes upregulated upon Wnt signaling.Citation16 After further validation in vivo, 14 genes were shown to be bona fide Wnt target genes, although whether they are direct targets could not be determined given the caveats discussed above. Several of these gene encoded cuticle collagens normally expressed in the mid L4, indicating a previously unknown role for Wnt signaling in regulating adult cuticle development.Citation16
Identifying Wnt Pathway Target Genes in the Larval Seam Cells
The analysis of Jackson et al showed that the conditional activation/microarray method could identify previously unknown Wnt pathway targets, however few of the genes found played an obvious role in development of the vulval precursor cells (VPCs) or seam cells, the 2 larval cells types we had shown were dependent on Wnt signaling for proper fate specification. One reason may be that we examined gene expression from whole animals, and identified genes expressed in the most cells or expressed at the highest levels. However, the cells we are interested in make up a tiny fraction of the cells in a larval worm: there are 32 seam cells and 6 VPCs in an early L3 stage worm, out of > 1000 somatic and germ cells.Citation29 One approach that has been used successfully to catalog gene expression in small numbers of embryonic cells is the dissociation of embryos expressing GFP in a defined cell type, followed by sorting and the use of GFP positive cells for gene expression analysis.Citation30,31 Unfortunately the thick cuticle of the larval worm is more difficult to dissociate than the embryonic eggshell, and the cells we are interested in are physically attached to the cuticle, rendering this technique unattractive.
To identify Wnt pathway targets specifically in the larval seam cells we combined our previous conditional Wnt activation approach with a cell type-specific profiling technique called ‘mRNA tagging’.Citation32 In this method an epitope-tagged polyA binding protein is expressed in the cell type of interest, proteins and mRNA are crosslinked in vivo, then transcripts from the cells of interest are isolated following affinity purification of the tagged polyA binding protein and crosslink reversal. This method has been successfully used to characterize the larval transcriptomes of muscle and neuronal cell types.Citation31-35 We believe we are the first to combine this method with the conditional activation of an extracellular signaling pathway, as described below.
In our recent work, we expressed the tagged polyA binding protein using an enhancer active in the larval seam cells and VPCsCitation36 (Gorrepati et al submitted). We conditionally activated the Wnt signaling pathway as before using heat shock expression of the amino-terminally deleted BAR-1 protein at the L2/L3 molt. We also conditionally inhibited Wnt signaling in other animals using heat shock expression of a dominant negative POP-1/TCF variant that lacks the β catenin binding domain; expression of this protein leads to Wnt reduction of function phenotypes in several cell types.Citation11,37 Animals in which Wnt signaling was activated and inhibited along with control heat shock animals were subjected to affinity purification to isolate mRNA pools enriched for transcripts from the seam cells and VPCs and these samples were subjected to microarray analysis to examine gene expression profiles. By comparing transcript levels in Wnt activated versus Wnt inhibited populations, we identified 239 putative Wnt pathway targets differentially expressed in the seam cells and VPCs in response to Wnt signaling (Gorrepati et al submitted). Interestingly, we identified the GATA factor encoding genes egl-18 and elt-6 as putative targets of Wnt signaling.Citation36 These factors seemed like prime candidates to be effectors of Wnt signaling that regulate fate in the larval asymmetric seam cell divisions because of their known role in initial embryonic specification of the seam cells.
Specification of the Embryonic Seam Cells Requires GATA Factors ELT-1, EGL-18 and ELT-6
During embryogenesis, a GATA transcription factor network comprising ELT-1, EGL-18 and ELT-6 is required for the initial specification of the seam cell fate in 10 cells per side.Citation6 While elt-1 is required to generate all epidermal cells (seam cells, P cells and syncytial hypodermal cells),Citation38,39 egl-18 and elt-6 are specifically required for specifying seam cell fate.Citation40 egl-18 and elt-6 are adjacent genes that encode related GATA type transcription factors that are expressed in the embryonic cells fated to become seam cells.Citation40 Loss of function of one or both factors by RNAi causes loss of expression of several seam specific genes, ectopic expression of elt-3, a GATA factor normally expresses in non-seam hypodermal cells, and fusion of the seam-fated cells with the surrounding hypodermis, leading to fewer seam cells in early larval worms. These results led to the model that these GATA factors are required downstream of ELT-1 to specify the seam cell fate initially in the embryo, and may also act in maintenance of the seam cell fate in larval life.Citation40 However, it was not clear how these factors may be regulated during larval life to perform their proposed functions. In addition, there was no evidence for the function of Wnt signaling in embryonic seam cell specification, so the identification of these genes as potential targets downstream of Wnt in the larval seam cells was a novel result.
egl-18 and elt-6 Act Downstream of Wnt Signaling to Maintain the Seam Cell Fate During Larval Asymmetric Cell Divisions
Consistent with a function in larval seam cell asymmetric divisions, we found that an egl-18 transcriptional reporter is expressed strongly in the posterior daughter of seam cell divisions that adopts the seam fate, while fading in the anterior daughter that differentiates.Citation36 Further, reduction of egl-18 and elt-6 function only in larval animals (after embryogenesis is complete) led to a decrease in the number of seam cells in young adults. Consistent with a role downstream of Wnt signaling, we showed that egl-18 reporter expression is increased or decreased after Wnt pathway activation or inhibition respectively, and that egl-18 activity is necessary for the hypodermal to seam cell fate transformations induced by Wnt pathway overactivation. A single POP-1/TCF binding site in an upstream region of egl-18 was required for strong expression of the egl-18 reporter in the larval seam cells, and POP-1 bound to this site in vitro. Finally, over expression of EGL-18 was sufficient to drive the expression of a seam cell marker in other hypodermal cells in wild type larvae, and in anterior hypodermal-fated daughters in a Wnt pathway-sensitized background, suggesting that egl-18 expression may be instructive and not just permissive for seam cell fate specification. Based on these results, we proposed that egl-18 (and elt-6) are reiteratively activated by Wnt signaling during the larval asymmetric seam cell divisions to facilitate the specification of seam cell fate in only one of the daughters of each division thereby allowing the renewal of this progenitor cell population after each cell division 36().
Figure 2. Wnt signaling regulates the asymmetry of the larval seam cell divisions. (A) In wild type animals, each seam cell divides once per larval stage to generate anterior hypodermal daughter (‘hyp’) that differentiates and a posterior daughter that maintains the seam cell fate (‘SC’) and the ability to divide further (vertical arrow). (B) When Wnt signaling pathway activity is reduced, seam cells are seen to divide symmetrically to generate 2 daughters that adopt the anterior ‘differentiated cell’ fate. (C) When Wnt signaling pathway activity is increased, seam cells are seen to divide symmetrically to generate 2 daughters that adopt the posterior 'progenitor cell' fate.
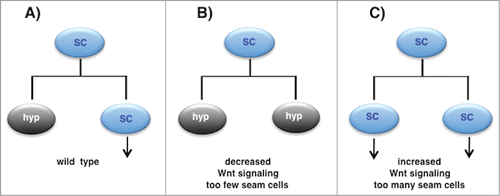
Figure 3. EGL-18 mediates seam cell fate specification in the embryo and larva downstream of different inputs. (A) During embryogenesis, cells in the AB lineage that are fated to become seam cells are first specified to adopt an epidermal fate (‘epi’) via action of the GATA factor ELT-1. Later, expression of CEH-16 in these cells leads to expression of the GATA factor EGL-18 (these cells also express the related GATA factor ELT-6, not shown), leading them to adopt the lateral hypodermal or seam cell fate (‘SC’). These cells do not divide until larval life begins. (B) In larval life, some seam cells divide asymmetrically to generate a posterior daughter that retains the seam cell fate and the ability to divide further. Maintenance of this progenitor cell fate requires activation of the Wnt signaling pathway to maintain expression of egl-18 in only that daughter cell of the division. Thus an embryonic fate specification factor is reutilized to maintain a progenitor cell population in larval life, and egl-18 specifies the seam cell fate both embryonically and post-embryonically, but in response to different input signals.
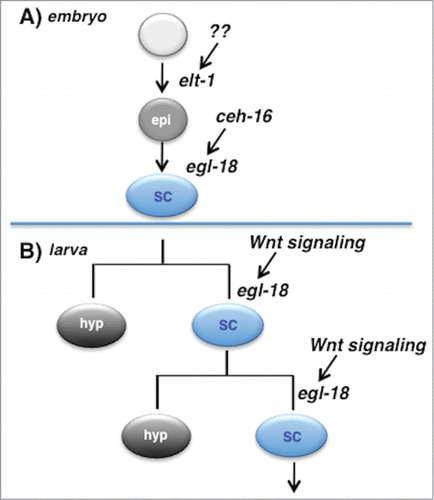
The Reutilization of Embryonic Specification Factors in Postembryonic Development
These data suggested that 2 GATA factors required for seam cell specification in the embryo independent of Wnt signaling, are reused downstream of Wnt signaling during larval life. During metazoan development, it is not uncommon for transcription factors to be re-used during larval or adult life to recapitulate aspects of embryonic development of a specific cell type or tissue. For instance, recent work has shown that the transcriptional cascade comprising Pax6, Neurog1 and Tbr1 and 2 used during embryonic neurogenesis is utilized again during adult neurogenesis.Citation41 Similarly, during mammalian skeletal muscle development, transcription factors Pax3 and Pax7 not only function during myogenesis in the embryo but are also crucial for maintaining the adult skeletal muscle stem cell population and initiating myogenesis in response to injury and muscle wasting.Citation42 In C. elegans, the Hox gene lin-39 is required in early larval life to specify a set of ventral hypodermal blast cells called the Vulval Precursor Cells.Citation43,44 Later in larval life Wnt signaling is required for these cells to maintain expression of lin-39 and their VPC fate,Citation24 however Wnt signaling is not required in the earlier lin-39-dependent fate specification process, and some factors required for initial embryonic expression of lin-39 in the precursors to these cells do not have a role in later larval expression.Citation45 The question that arises is why do seam cells re-use these GATA factors in larval life?
In the C. elegans embryo, the fate choices of embryonic cells are controlled by the asymmetric segregation of transcription factors and the asymmetric activation of signaling pathways at early cell divisions.Citation46 Such processes lead to expression of the transcription factor ELT-1 in the descendants of the founder cell AB that will become the embryonic seam cells, causing them to adopt a general epidermal fate, however the exact factors or signals required for early ELT-1 expression in the prospective seam cells are unknownCitation6 (). Further unknown events lead to expression of the Engrailed homolog CEH-16 in these cells, which is necessary for expression of EGL-18 and ELT-6 and adoption of the seam cell fate.Citation40,47 It is parsimonious to extend this basic mechanism to maintain seam cell identity in larval life as well: there is no need to ‘reinvent the wheel’. However, once embryogenesis is complete, this unique combination of factors and signals is unlikely to be present in the larva. Further, the larval seam cells undergo asymmetric divisions that require the action of the fate specification factors to be restricted to only one daughter of a division (). Given this, it is not unreasonable that the regulation of egl-18 and elt-6 should come under the WBA pathway that controls many asymmetric divisions in C. elegans,Citation5 perhaps through acquisition of POP-1 binding site in the upstream region.Citation36 In this way, these master regulators of seam cell fate that are initially expressed in response to one set of factors in the embryo can be reutilized downstream of Wnt signaling during asymmetric larval cell divisions to maintain the progenitor seam fate in one daughter.
A number of questions remain about the role of Wnt signaling and the GATA factors EGL-18 and ELT-6 in the development and division of the seam cells. For example, it is still not clear what factors lead to the asymmetric activation of the Wnt pathway in just the posterior daughter of each larval seam cell division, when the seam cells themselves are arrayed along the anterior-posterior axis.Citation10,11 Also, it will be interesting to identify the targets of EGL-18 and ELT-6 that allow them to execute and maintain the progenitor-like seam cell fate. egl-18 activity is required for expression of several nuclear hormone receptors in the seam cells, and for repression of the GATA factor ELT-3 and the fusogen EFF-1.Citation40,47 Other factors identified in the conditional Wnt activation array experiments may also function downstream of EGL-18 and ELT-6 (Gorrepati et all, submitted). Considering the crucial role Wnt signaling and GATA factors play in regulating stem cell biology in many mammalian tissues, the C. elegans seam cells should provide an excellent model to elaborate the relationships between these important factors in metazoan development.
Disclosure of Potential Conflicts of Interest
No potential conflicts of interest were disclosed.
Acknowledgments
We thank M. Krause, J. Rothman, H. Korswagen, I. Greenwald, I. Hamza, Y. Budovskaya, H. Zhang and S. Kim for sharing reagents and strains used in the work described here. Some nematode strains were provided by the National BioResource Project of Japan and the Caenorhabditis Genetics Center (CGC), which is funded by the National Institutes of Health National Center for Research Resources (NCRR).
Funding
The work described here was supported by National Science Foundation (NSF) grant [IBN-0131485], National Institutes of Health (NIH) grant [GM65424] and support from the University of Maryland Baltimore County.
References
- Cadigan KM, Peifer M. Wnt signaling from development to disease: insights from model systems. Cold Spring Harb Perspect Biol 2009; 1:a002881; PMID:20066091
- Clevers H, Nusse R. Wnt/β-catenin signaling and disease. Cell 2012; 149:1192-205; PMID:22682243
- MacDonald BT, Tamai K, He X. Wnt/β-catenin signaling: components, mechanisms, and diseases. Dev Cell 2009; 17:9-26; PMID:19619488
- Jackson BM, Eisenmann DM. β-catenin-dependent Wnt signaling in C. elegans: teaching an old dog a new trick. Cold Spring Harb Perspect Biol 2012; 4:a007948; PMID:22745286
- Sawa H, Korswagen HC. Wnt signaling in C. elegans (December 9, 2013). In: Community TCeR, ed. WormBook, ed. The C. elegans Research Community, WormBook, doi:10.1895/wormbook.1.7.2, http://www.wormbook.org
- Chisholm AD, Hsiao TI. The epidermis as a model skin. I: development, patterning, and growth. Wiley Interdiscip Rev Dev Biol 2012; 1:861-78; PMID:23539299
- Chisholm AD, Xu S. The Caenorhabditis elegans epidermis as a model skin. II: differentiation and physiological roles. Wiley Interdiscip Rev Dev Biol 2012; 1:879-902; PMID:23539358
- Page AP, Johnstone IL. The cuticle. he cuticle (March 19, 2007), WormBook, ed. The C. elegans Research Community, WormBook, doi:10.1895/wormbook.1.138.1, http://www.wormbook.org.
- Joshi PM, Riddle MR, Djabrayan NJ, Rothman JH. Caenorhabditis elegans as a model for stem cell biology. Dev Dyn 2010; 239:1539-54; PMID:20419785
- Banerjee D, Chen X, Lin SY, Slack FJ. kin-19/casein kinase Iα has dual functions in regulating asymmetric division and terminal differentiation in C. elegans epidermal stem cells. Cell Cycle 2010; 9:4748-65; PMID:21127398
- Gleason JE, Eisenmann DM. Wnt signaling controls the stem cell-like asymmetric division of the epithelial seam cells during C. elegans larval development. Dev Biol 2010; 348:58-66; PMID:20849842
- Huang X, Tian E, Xu Y, Zhang H. The C. elegans engrailed homolog ceh-16 regulates the self-renewal expansion division of stem cell-like seam cells. Dev Biol 2009; 333:337-47; PMID:19607822
- Ren H, Zhang H. Wnt signaling controls temporal identities of seam cells in Caenorhabditis elegans. Dev Biol 2010; 345:144-55; PMID:20624379
- Holland JD, Klaus A, Garratt AN, Birchmeier W. Wnt signaling in stem and cancer stem cells. Curr Opin Cell Biol 2013; 25:254-64; PMID:23347562
- Lien WH, Fuchs E. Wnt some lose some: transcriptional governance of stem cells by Wnt/β-catenin signaling. Genes Dev 2014; 28:1517-32; PMID:25030692
- Jackson BM, Abete-Luzi P, Krause MW, Eisenmann DM. Use of an activated β-catenin to identify Wnt pathway target genes in Caenorhabditis elegans, including a subset of collagen genes expressed in late larval development. G3 (Bethesda) 2014; 4:733-47.
- http://www.stanford.edu/∼rnusse/wntwindow.html.
- Arata Y, Kouike H, Zhang Y, Herman MA, Okano H, Sawa H. Wnt signaling and a Hox protein cooperatively regulate psa-3/Meis to determine daughter cell fate after asymmetric cell division in C. elegans. Dev Cell 2006; 11:105-15; PMID:16824957; http://dx.doi.org/10.1016/j.devcel.2006.04.020
- Bertrand V, Hobert O. Wnt asymmetry and the terminal division of neuronal progenitors. Cell Cycle 2009; 8:1973-4; PMID:19550137; http://dx.doi.org/10.4161/cc.8.13.9024
- Lam N, Chesney MA, Kimble J. Wnt signaling and CEH-22/tinman/Nkx2.5 specify a stem cell niche in C. elegans. Curr Biol 2006; 16:287-95; PMID:16461282; http://dx.doi.org/10.1016/j.cub.2005.12.015
- Maduro MF, Kasmir JJ, Zhu J, Rothman JH. The Wnt effector POP-1 and the PAL-1/Caudal homeoprotein collaborate with SKN-1 to activate C. elegans endoderm development. Devl Biol 2005; 285:510-23; PMID:16084508; http://dx.doi.org/10.1016/j.ydbio.2005.06.022
- Shetty P, Lo MC, Robertson SM, Lin R. C. elegans TCF protein, POP-1, converts from repressor to activator as a result of Wnt-induced lowering of nuclear levels. Dev Biol 2005; 285:584-92; PMID:16112103; http://dx.doi.org/10.1016/j.ydbio.2005.07.008
- Streit A, Kohler R, Marty T, Belfiore M, Takacs-Vellai K, Vigano M, Schnabel R, Affolter M, Müller F. Conserved regulation of the Caenorhabditis elegans labial/Hox1 gene ceh-13. Dev Biol 2002; 242:96-108; PMID:11820809; http://dx.doi.org/10.1006/dbio.2001.0544
- Eisenmann DM, Maloof JN, Simske JS, Kenyon C, Kim SK. The β-catenin homolog BAR-1 and LET-60 Ras coordinately regulate the Hox gene lin-39 during Caenorhabditis elegans vulval development. Development 1998; 125:3667-80; PMID:9716532
- Jiang LI, Sternberg PW. Interactions of EGF, Wnt and HOM-C genes specify the P12 neuroectoblast fate in C. elegans. Development 1998; 125:2337-47; PMID:9584132
- Maloof JN, Whangbo J, Harris JM, Jongeward GD, Kenyon C. A Wnt signaling pathway controls hox gene expression and neuroblast migration in C. elegans. Development 1999; 126:37-49; PMID:9834184
- Bhambhani C, Ravindranath AJ, Mentink RA, Chang MV, Betist MC, Yang YX, Koushika SP, Korswagen HC, Cadigan KM. Distinct DNA binding sites contribute to the TCF transcriptional switch in C. elegans and Drosophila. PLoS Genet 2014; 10:e1004133; PMID:24516405; http://dx.doi.org/10.1371/journal.pgen.1004133
- Gleason JE, Korswagen HC, Eisenmann DM. Activation of Wnt signaling bypasses the requirement for RTK/Ras signaling during C. elegans vulval induction. Genes Dev 2002; 16:1281-90; PMID:12023306; http://dx.doi.org/10.1101/gad.981602
- Sulston J, Horvitz H. Post-embryonic cell lineages of the nematode, Caenorhabditis elegans. Dev Biol 1977; 56:110-56; PMID:838129; http://dx.doi.org/10.1016/0012-1606(77)90158-0
- Fox RM, Von Stetina SE, Barlow SJ, Shaffer C, Olszewski KL, Moore JH, Dupuy D, Vidal M, Miller DM, 3rd. A gene expression fingerprint of C. elegans embryonic motor neurons. BMC Genomics 2005; 6:42; PMID:15780142; http://dx.doi.org/10.1186/1471-2164-6-42
- Spencer WC, Zeller G, Watson JD, Henz SR, Watkins KL, McWhirter RD, Petersen S, Sreedharan VT, Widmer C, Jo J, et al. A spatial and temporal map of C. elegans gene expression. Genome Res 2011; 21:325-41; PMID:21177967; http://dx.doi.org/10.1101/gr.114595.110
- Roy PJ, Stuart JM, Lund J, Kim SK. Chromosomal clustering of muscle-expressed genes in Caenorhabditis elegans. Nature 2002; 418:975-9; PMID:12214599
- Kunitomo H, Uesugi H, Kohara Y, Iino Y. Identification of ciliated sensory neuron-expressed genes in Caenorhabditis elegans using targeted pull-down of poly(A) tails. Genome Biol 2005; 6:R17; PMID:15693946; http://dx.doi.org/10.1186/gb-2005-6-2-r17
- Takayama J, Faumont S, Kunitomo H, Lockery SR, Iino Y. Single-cell transcriptional analysis of taste sensory neuron pair in Caenorhabditis elegans. Nucleic Acids Res 2010; 38:131-42; PMID:19875417; http://dx.doi.org/10.1093/nar/gkp868
- Von Stetina SE, Watson JD, Fox RM, Olszewski KL, Spencer WC, Roy PJ, Miller DM, 3rd. Cell-specific microarray profiling experiments reveal a comprehensive picture of gene expression in the C. elegans nervous system. Genome Biol 2007; 8:R135; PMID:17612406; http://dx.doi.org/10.1186/gb-2007-8-7-r135
- Gorrepati L, Thompson KW, Eisenmann DM. C. elegans GATA factors EGL-18 and ELT-6 function downstream of Wnt signaling to maintain the progenitor fate during larval asymmetric divisions of the seam cells. Development 2013; 140:2093-102; PMID:23633508; http://dx.doi.org/10.1242/dev.091124
- Korswagen HC, Herman MA, Clevers HC. Distinct β-catenins mediate adhesion and signalling functions in C. elegans. Nature 2000; 406:527-32; PMID:10952315; http://dx.doi.org/10.1038/35020099
- Gilleard JS, McGhee JD. Activation of hypodermal differentiation in the Caenorhabditis elegans embryo by GATA transcription factors ELT-1 and ELT-3. Mol Cell Biol 2001; 21:2533-44; PMID:11259601; http://dx.doi.org/10.1128/MCB.21.7.2533-2544.2001
- Page BD, Zhang W, Steward K, Blumenthal T, Priess JR. ELT-1, a GATA-like transcription factor, is required for epidermal cell fates in Caenorhabditis elegans embryos. Genes Dev 1997; 11:1651-61; PMID:9224715; http://dx.doi.org/10.1101/gad.11.13.1651
- Koh K, Rothman J. ELT-5 and ELT-6 are required continuously to regulate epidermal seam cell differentiation and cell fusion in C. elegans. Development 2001; 128:2867-80; PMID:11532911
- Hodge RD, Hevner RF. Expression and actions of transcription factors in adult hippocampal neurogenesis. Dev Neurobiol 2011; 71:680-9; PMID:21412988; http://dx.doi.org/10.1002/dneu.20882
- Chang NC, Rudnicki MA. Satellite cells: the architects of skeletal muscle. Curr Top Dev Biol 2014; 107:161-81; PMID:24439806; http://dx.doi.org/10.1016/B978-0-12-416022-4.00006-8
- Clark S, Chisholm A, Horvitz H. Control of cell fates in the central body region of C. elegans by the homeobox gene lin-39. Cell 1993; 74:43-55; PMID:8101475; http://dx.doi.org/10.1016/0092-8674(93)90293-Y
- Wang BB, Muller-Immergluck MM, Austin J, Robinson NT, Chisholm A, Kenyon C. A homeotic gene cluster patterns the anteroposterior body axis of C. elegans. Cell 1993; 74:29-42; PMID:8101474; http://dx.doi.org/10.1016/0092-8674(93)90292-X
- Liu WJ, Reece-Hoyes JS, Walhout AJ, Eisenmann DM. Multiple transcription factors directly regulate Hox gene lin-39 expression in ventral hypodermal cells of the C. elegans embryo and larva, including the hypodermal fate regulators LIN-26 and ELT-6. BMC Dev Biol 2014; 14:17; PMID:24885717; http://dx.doi.org/10.1186/1471-213X-14-17
- Maduro MF. Cell fate specification in the C. elegans embryo. Dev Dyn 2010; 239:1315-29; PMID:20108317
- Cassata G, Shemer G, Morandi P, Donhauser R, Podbilewicz B, Baumeister R. ceh-16/engrailed patterns the embryonic epidermis of Caenorhabditis elegans. Development 2005; 132:739-49; PMID:15659483; http://dx.doi.org/10.1242/dev.01638