ABSTRACT
Starvation causes reductions in plasma leptin concentrations, but the physiologic impact of this observation had not been documented in the starved state. Here we discuss our recent work demonstrating that hypoleptinemia activates the hypothalamic-pituitary-adrenal (HPA) axis, increasing white adipose tissue (WAT) lipolysis and mediating a shift from glucose to fat metabolism to maintain euglycemia in fasted rats.
Throughout history, frequent feast-and-famine cycles have required humans and animals to evolve mechanisms to store energy when food is plentiful, and mobilize energy efficiently when food is scarce, thereby maintaining euglycemia and permitting survival. In the fed state, the body primarily metabolizes glucose, whereas in the fasted state, fat and ketone metabolism prevailCitation1; however the signals that regulate this transition have been the subject of debate for decades. In a classic study published in Nature, Ahima et al. demonstrated hypoleptinemia in fasted mice and performed bolus injections of high doses of leptin to interrogate the physiologic impact of hypoleptinemia. They concluded that leptin mediates the role of the reproductive, thyroid, and adrenal axes to starvation, but did not observe a significant impact of leptin on glycemia or ketosis.Citation2 However, we have recently shown that in diabetic ketoacidosis, hypoleptinemia causes hypothalamic-pituitary-adrenal (HPA) axis activation and resultant increases in white adipose tissue (WAT) lipolysis, hepatic acetyl-CoA content, and hepatic gluconeogenesis.Citation3,Citation4 We hypothesized that the experimental conditions employed (a bolus administration of very high doses of leptin, as opposed to a continuous infusion increasing leptin concentrations to the physiologic range in starved rodents) could explain the absence of an effect of leptin on glycemia in the study by Ahima et al. To test this hypothesis, we re-examined the physiologic role of hypoleptinemia in starvation using stable isotope infusions including Positional Isotopomer Nuclear Magnetic Resonance Tracer AnalysisCitation5 to examine the impact of hypoleptinemia on lipolysis, hepatic acetyl-CoA content, and rates of glycogenolysis, pyruvate carboxylase flux, and gluconeogenesis from glycerol.Citation6
In our recent study, we examined two key transitions: from the recently fed state (0–8 hr fasted) to moderate-term fasting (16 hr), and from moderate- (16 hr) to long-term starvation (48 hr). In the recently fed to moderate-term fasted transition, reductions in plasma leptin increased plasma corticosterone, thereby increasing WAT lipolysis, hepatic acetyl-CoA content, and hepatic pyruvate carboxylase flux, allowing rats to maintain euglycemia despite exhibiting marked reductions in rates of hepatic glycogenolysis (). We then demonstrated that glycogen depletion, with resultant reductions in plasma glucose and insulin concentrations and adipocyte glucose uptake, were the signal to the fat cell to lower plasma leptin concentrations: treatment of 8 and 16 hr fasted rats with an inhibitor of glycogen phosphorylase and thus of glycogenolysis reduced plasma glucose, insulin, and leptin concentrations and increased plasma corticosterone to levels measured in glycogen depleted, 48 hr fasted rats. These data demonstrate that plasma glucose and insulin concentrations provide a systemic barometer for hepatic glycogen reserves and the resulting reductions in plasma glucose and insulin signal the adipocyte to mobilize energy due to reductions in leptin and increases in HPA axis activity generating increased WAT lipolysis and ketogenesis. The increases in fatty acid and ketone concentrations that then occur due to increased rates of WAT lipolysis and ketogenesis provide fuel for critical organs such as the brain and heart, even as the supply of glucose is declining.
Figure 1. Proposed mechanism of a glucose-fatty acid cycle permitting the maintenance of adequate plasma glucose concentrations in the short-term and prolonged fasted states. WAT, white adipose tissue; HPA, hypothalamic-pituitary-adrenal axis. Liver, adrenal, and muscle images were obtained from Servier Medical Art.
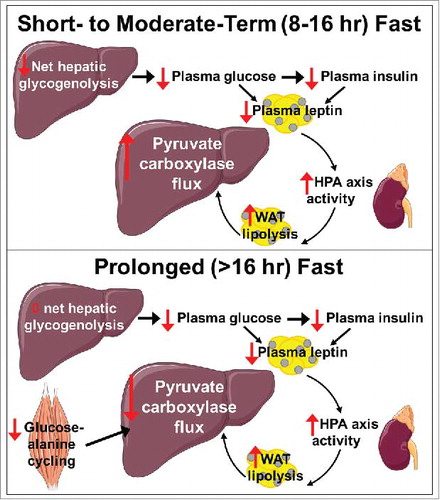
These data beg the question of why prior studies had not observed an effect of leptin on plasma glucose in the fastedCitation2 or insulinopenic type 1 diabetic (T1D) state.Citation7 However, prior studies have administered large boluses of leptin resulting in plasma leptin concentrations 10–100 times normal. Given the ability of leptin to stimulate catecholamine synthesis,Citation8 we hypothesized that these supraphysiologic leptin concentrations could activate sympathetic nervous system (SNS) activity, obfuscating the effect of leptin to suppress glucocorticoid-driven WAT lipolysis. Consistent with this hypothesis, we found that infusion of leptin to achieve normal physiologic concentrations in 48 hr fasted and T1D rats markedly reduced plasma corticosterone, WAT lipolysis, and hepatic gluconeogenesis, such that the former group required glucose infusion to avoid symptomatic hypoglycemia.Citation6 However, infusion of supraphysiologic leptin to increase plasma leptin concentrations to those observed in a prior study in T1D ratsCitation7 stimulated SNS activity, increasing WAT lipolysis and hepatic gluconeogenesis and reversing the effect of leptin to lower plasma glucose concentrations in fasted or T1D rats.Citation6 These data highlight the critical importance of interrogating the role of leptin in mediating glycemia using physiologic leptin infusions rather than treatment with high doses of this agent.
Surprisingly, in the prolonged (48 hr) fasted state, despite increased WAT lipolysis and increased hepatic acetyl-CoA due to reductions in plasma leptin and increases in corticosterone concentrations, rats exhibited 50% reductions in hepatic pyruvate carboxylase flux and hepatic gluconeogenesis.Citation6 Consistent with prior studies demonstrating reduced alanine turnover in fasted humans,Citation9 we observed a 65% reduction in whole-body alanine turnover after a 48 hr fast, which resulted in suppression of hepatic mitochondrial anaplerosis (pyruvate carboxylase flux) and oxidation (hepatic citrate synthase flux) (Figure). However, infusion of alanine to restore alanine turnover to rates measured in recently fed rats increased both hepatic pyruvate carboxylase flux and hepatic citrate synthase flux rates similar to those measured in fed animals.Citation6 Thus these data demonstrate that anaplerotic substrate limitation, even in the setting of increases in WAT lipolysis and hepatic acetyl-CoA content, limit hepatic gluconeogenesis during a prolonged fast.
Taken together, our recent study demonstrates that in addition to reductions in plasma insulin concentrations, hypoleptinemia is also necessary to maintain euglycemia during starvation in awake rodents. We also describe a novel glucose-fatty acid cycle whereby reductions in hepatic glycogen content lead to reductions in leptin production due to reduced WAT glucose uptake, thereby stimulating the HPA axis, WAT lipolysis, hepatic acetyl-CoA content, and hepatic pyruvate carboxylase flux in the short-term fasted state. However, in prolonged starvation, substrate limitation resulting from reduced muscle glucose-alanine cycling impairs both hepatic pyruvate carboxylase flux and hepatic mitochondrial oxidation. These data provide an evolutionary explanation for hepatic insulin resistance with starvation: with prolonged starvation, increased hepatic lipid accumulation due to increased WAT lipolysis minimize hepatic glucose uptake and synthesis of glycogen, thereby shuttling any available glucose to critical organs such as the heart and brain. Future studies will be required to demonstrate whether this glucose-fatty acid cycle is similarly important to maintain euglycemia in the starved state in humans.
Disclosure of potential conflicts of interest
No potential conflicts of interest were disclosed.
Additional information
Funding
References
- Rothman DL, Magnusson I, Katz LD, Shulman RG, Shulman GI. Quantitation of hepatic glycogenolysis and gluconeogenesis in fasting humans with 13C NMR. Science. 1991;254(5031):573–6. PubMed. doi:10.1126/science.1948033. PMID:1948033.
- Ahima RS, Prabakaran D, Mantzoros C, Qu D, Lowell B, Maratos-Flier E, Flier JS. Role of leptin in the neuroendocrine response to fasting. Nature. 1996;382(6588):250–2. PubMed doi:10.1038/382250a0. PMID:8717038.
- Perry RJ, Zhang XM, Zhang D, Kumashiro N, Camporez JP, Cline GW, Rothman DL, Shulman GI. Leptin reverses diabetes by suppression of the hypothalamic-pituitary-adrenal axis. Nat Med. 2014. Epub 2014/06/16. PubMed. doi:10.1038/nm.3579
- Perry RJ, Peng L, Abulizi A, Kennedy L, Cline GW, Shulman GI. Mechanism for leptin's acute insulin-independent effect to reverse diabetic ketoacidosis. J Clin Invest. 2017;127(2):657–69. PubMed; PMCID: PMC5272181. doi:10.1172/JCI88477. PMID:28112679
- Perry RJ, Peng L, Cline GW, Butrico GM, Wang Y, Zhang XM, Rothman DL, Petersen KF, Shulman GI. Non-invasive assessment of hepatic mitochondrial metabolism by positional isotopomer NMR tracer analysis (PINTA). Nat Commun. 2017;8(1):798. PubMed doi:10.1038/s41467-017-01143-w. PMID:28986525
- Perry RJ, Wang Y, Cline GW, Rabin-Court A, Song JD, Dufour S, Zhang XM, Petersen KF, Shulman GI. Leptin Mediates a Glucose-Fatty Acid Cycle to Maintain Glucose Homeostasis in Starvation. Cell. 2018;172(1–2):234–48 e17. PubMed; PMCID: PMC5766366. doi:10.1016/j.cell.2017.12.001
- Morton GJ, Meek TH, Matsen ME, Schwartz MW. Evidence against hypothalamic-pituitary-adrenal axis suppression in the antidiabetic action of leptin. J Clin Invest. 2015;2015:4587–4591. PubMed; PMCID: PMC4665796.
- Shibuya I, Utsunomiya K, Toyohira Y, Ueno S, Tsutsui M, Cheah TB, Ueta Y, Izumi F, Yanagihara N. Regulation of catecholamine synthesis by leptin. Ann N Y Acad Sci. 2002;971:522–7. PubMed. doi:10.1111/j.1749-6632.2002.tb04517.x. PMID:12438173
- Felig P, Pozefsky T, Marliss E, Cahill GF, Jr. Alanine: key role in gluconeogenesis. Science. 1970;167(3920):1003–4. PubMed. doi:10.1126/science.167.3920.1003. PMID:5411169