ABSTRACT
Transcription and replication complexes can coincide in space and time. Such coincidences may result in collisions that trigger genomic instability. The phosphorylation of Mrc1 by different signaling kinases is part of a general mechanism that serves to delay replication in response to different stresses that trigger a massive transcriptional response in S phase. This mechanism prevents Transcription-Replication Conflicts and maintains genomic integrity in response to unscheduled massive transcription during S phase.
Transcription-Replication Conflicts (TRCs) are a major cause of genomic instability. Citation1 Cells have evolved a wide range of strategies to prevent TRCs, whereby they limit potential head-on collisions between the transcription and replication machineries, which are the events most likely to trigger recombination events and genomic instability. Citation2 Eukaryotic cells focus their strategies for the protection of highly transcribed regions either by the use of non-nucleosomal DNA-bound protein structures that cause a polar block of DNA replication Citation3 or by locating replication and transcription factories in temporarily and spatially separated chromatin domains. Citation4 However, how cells cope with sudden and unscheduled outbursts of transcription during S phase is an unclear question.
Cells are continuously exposed to changing environmental conditions that challenge their survival. Specific signaling pathways are activated to cope with such cellular stresses by the induction of a global cellular response to maximize cell survival. Stress-Activated Protein Kinases (SAPKs) are key for this response to stress. Hog1, the yeast counterpart of the mammalian p38, is a prototypical SAPK. Hog1 is activated upon osmostress and leads to the regulation of many cellular functions including the transcription of hundreds of stress-responsive genes. Citation5 Such massive activation of gene expression, although crucial for the osmoadaptive response, represents an obstacle to the replication machinery during duplication of the genome. Remarkably, the stress-activated Hog1 phosphorylates Mrc1, a protein of the replication complex, to temporarily block DNA replication. The Hog1-mediated phosphorylation sites in Mrc1 (Thr169, Ser215 and Ser229) are located in the N-terminal domain and are different to the Mrc1 sites phosphorylated by Mec1 in response to DNA damage. Citation6 Cells carrying the Hog1-non-phosphorylatable allele of MRC1 (mrc13A ) bypassed the replication block and displayed a dramatic increase in Transcription-Associated Recombination (TAR) and genomic instability. Citation7 Therefore, when cells activate transcription upon osmostress, the concomitant transient block of DNA replication is critical in order to guarantee cell survival.
In addition to its role in osmostress, Mrc1 also plays a key role in maintaining genomic integrity in response to other stresses that trigger the Environmental Stress Response (ESR) transcriptional signature. Citation8 The N-terminal phosphorylation of Mrc1 at the Hog1 sites is essential for blocking S phase upon heat, oxidative or low glucose stresses, which also involve the massive transcriptional response featured by the ESR. Correspondingly, mrc13A cells bypass the S phase block in response to these stresses and display TAR and genomic instability. An unbiased kinome screen identified Mpk1, Psk1 and Snf1 as the kinases that phosphorylate Mrc1 upon heat, oxidative and low glucose stresses respectively. Thus, cells use canonical stress-signaling pathways to phosphorylate a common substrate, Mrc1, to protect genomic integrity during unscheduled massive transcription in S phase. Interestingly, the role of Mrc1 is not restricted to environmental cues and it also responds to internal stresses, such as slow growth or genomic instability, that also trigger the ESR response. Overall, Mrc1 plays a key role in protecting genomic integrity in scenarios that compromise cell survival, either due to environmental insults or as a result of abnormal cell fitness. This “Mrc1 transcription-replication safeguard mechanism (MTR)” protects genomic integrity when outbursts of transcription occur during S phase ().
Figure 1. The “MTR safeguard mechanism” protects genomic integrity in response to stress-induced outbursts of transcription during S phase. Cells induce a conserved transcriptional signature known as the ESR upon environmental stresses such as osmo (NaCl), heat (37ºC), oxidative (H2O2) or low glucose as well as to mutations that reduce cell fitness. (A) Mrc1 is phosphorylated at the N-terminus by multiple signaling kinases (e.g. Hog1, Mpk1, Psk1 or Snf1), which delays replication to maintain genomic integrity upon stress. (B) The unphosphorylatable mutant of Mrc1 (mrc13A ) fails to delay replication and accumulates TAR and genomic instability due to collisions between RNA and DNA polymerases. The role of Mrc1 in the MTR is fully independent of the DNA damage checkpoint pathway.
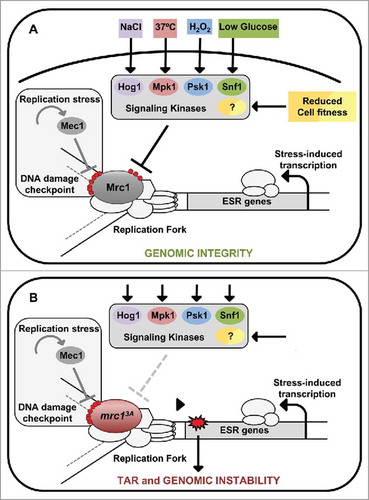
Identification of the MTR highlights the necessity for cells to possess a dedicated mechanism for the prevention of TRCs that can eventually occur in the genome when there is an unscheduled massive induction of transcription. Such unscheduled transcription differs from transcriptions that operate at particular sites in the genome, or at particular genes that are scheduled to be induced during the S phase of the cell cycle. Future work will allow further exploration of whether the function of Mrc1 is relevant beyond stress and whether it plays a pivotal role in preventing collisions and genomic instability when regular transcription takes place during S phase.
Of note, Mrc1 has two independent functions that are necessary for maintaining genomic integrity. First, Mrc1 is a protein of the Replication Complex that is essential for achieving efficient fork speed during DNA replication. Citation9 Second, Mrc1 also has a crucial function in activation of the DNA damage checkpoint in response to replication stress. Citation6 Protection of genomic integrity by N-terminally phosphorylated Mrc1 is a third independent function of Mrc1 since mrc13A cells replicate efficiently under normal conditions and are proficient in activating the DNA damage checkpoint pathway in response to DNA damage and replication stress. Citation7 , Citation8
If the MTR safeguard mechanism were to be conserved in higher eukaryotes, it could be crucial not only for avoiding collisions and genomic instability in cells exposed to environmental changes (e.g., epithelial cells that suffer temperature changes; renal cells that support high osmolarity, etc.) but it might also play an important function in pre-cancerous cells, which display genomic instability that is sensed as an internal stress. Citation10 Furthermore, a high percentage of tumorigenic cells display mutations in the DNA damage checkpoint, resulting in an important obstacle to chemotherapy treatment. Thus, the discovery of a novel regulatory pathway that blocks the cell cycle independently of known checkpoint pathways opens a new landscape of possible new compounds that might block cell cycle progression.
Disclosure of potential conflicts of interest
No potential conflicts of interest were disclosed.
Additional information
Funding
References
- Hamperl S , Cimprich KA. Conflict Resolution in the Genome: How Transcription and Replication Make It Work. Cell. 2016;167:1455–67. doi:10.1016/j.cell.2016.09.053. PMID:27912056
- Prado F , Aguilera A . Impairment of replication fork progression mediates RNA polII transcription-associated recombination. EMBO J. 2005;24:1267–76. doi:10.1038/sj.emboj.7600602. PMID:15775982
- Brewer BJ , Fangman WL . A replication fork barrier at the 3′ end of yeast ribosomal RNA genes. Cell. 1988;55:637-43. doi:10.1016/0092-8674(88)90222-X. PMID:3052854
- Meryet-Figuiere M , Alaei-Mahabadi B , Ali MM , Mitra S , Subhash S , Pandey GK , Larsson E , Kanduri C . Temporal separation of replication and transcription during S-phase progression. Cell Cycle. 2014;13:3241–8. doi:10.4161/15384101.2014.953876. PMID:25485504
- de Nadal E , Posas F . Osmostress-induced gene expression–;a model to understand how stress-activated protein kinases (SAPKs) regulate transcription. FEBS J. 2015;282:3275-85. doi:10.1111/febs.13323. PMID:25996081
- Osborn AJ , Elledge SJ . Mrc1 is a replication fork component whose phosphorylation in response to DNA replication stress activates Rad53. Genes Dev. 2003;17:1755–67. doi:10.1101/gad.1098303. PMID:12865299
- Duch A , Felipe-Abrio I , Barroso S , Yaakov G , Garcia-Rubio M , Aguilera A , de Nadal E. , Posas F . Coordinated control of replication and transcription by a SAPK protects genomic integrity. Nature. 2013;493:116-9. doi:10.1038/nature11675. PMID:23178807
- Duch A , Canal B , Barroso SI , Garcia-Rubio M , Seisenbacher G , Aguilera A , de Nadal E. , Posas F . Multiple signaling kinases target Mrc1 to prevent genomic instability triggered by transcription-replication conflicts. Nat Commun. 2018;9:379. doi:10.1038/s41467-017-02756-x. PMID:29371596
- Yeeles JT , Janska A , Early A , Diffley JF . How the Eukaryotic Replisome Achieves Rapid and Efficient DNA Replication. Mol Cell. 2017;65:105–16. doi:10.1016/j.molcel.2016.11.017. PMID:27989442
- Pfau SJ , Amon A . Chromosomal instability and aneuploidy in cancer: From yeast to man. EMBO Rep. 2012;13:515–27. doi:10.1038/embor.2012.65. PMID:22614003